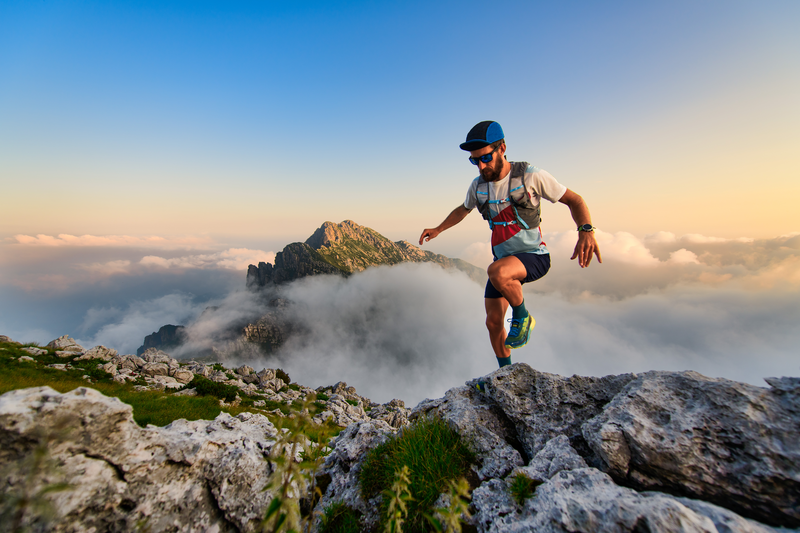
94% of researchers rate our articles as excellent or good
Learn more about the work of our research integrity team to safeguard the quality of each article we publish.
Find out more
PERSPECTIVE article
Front. Neurosci.
Sec. Auditory Cognitive Neuroscience
Volume 19 - 2025 | doi: 10.3389/fnins.2025.1534425
This article is part of the Research Topic Impact of Acoustic Environments and Noise on Auditory Perception View all 6 articles
The final, formatted version of the article will be published soon.
You have multiple emails registered with Frontiers:
Please enter your email address:
If you already have an account, please login
You don't have a Frontiers account ? You can register here
Listening to speech in the presence of irrelevant sounds is ubiquitous in the modern world, but is generally acknowledged to be both effortful and unpleasant.Here we argue that this problem arises largely in circumstances that our human auditory system has not evolved to accommodate. The soundscapes of the Anthropocene are frequently characterized by an overabundance of sound sources, the vast majority of which are functionally irrelevant to a given listener. The problem of listening to speech in such environments must be solved by an auditory system that is not optimized for this task. Building on our previous work linking attention to effortful listening and incorporating an active inference approach, we argue that the answers to these questions have implications not just for the study of human audition. They are also significant for the development and broad awareness of hearing aids and cochlear implants, as well as other auditory technologies such as earbuds, immersive auditory environments, and systems for human-machine interaction.
Keywords: Hearing, evolution, Noise, Free Energy Principle, Attention, listening effort
Received: 25 Nov 2024; Accepted: 19 Feb 2025.
Copyright: © 2025 Strauss, Francis, Schäfer, Latzel, Corona-Strauss and Launer. This is an open-access article distributed under the terms of the Creative Commons Attribution License (CC BY). The use, distribution or reproduction in other forums is permitted, provided the original author(s) or licensor are credited and that the original publication in this journal is cited, in accordance with accepted academic practice. No use, distribution or reproduction is permitted which does not comply with these terms.
* Correspondence:
Daniel J. Strauss, Saarland University, Saarbrücken, Germany
Disclaimer: All claims expressed in this article are solely those of the authors and do not necessarily represent those of their affiliated organizations, or those of the publisher, the editors and the reviewers. Any product that may be evaluated in this article or claim that may be made by its manufacturer is not guaranteed or endorsed by the publisher.
Research integrity at Frontiers
Learn more about the work of our research integrity team to safeguard the quality of each article we publish.