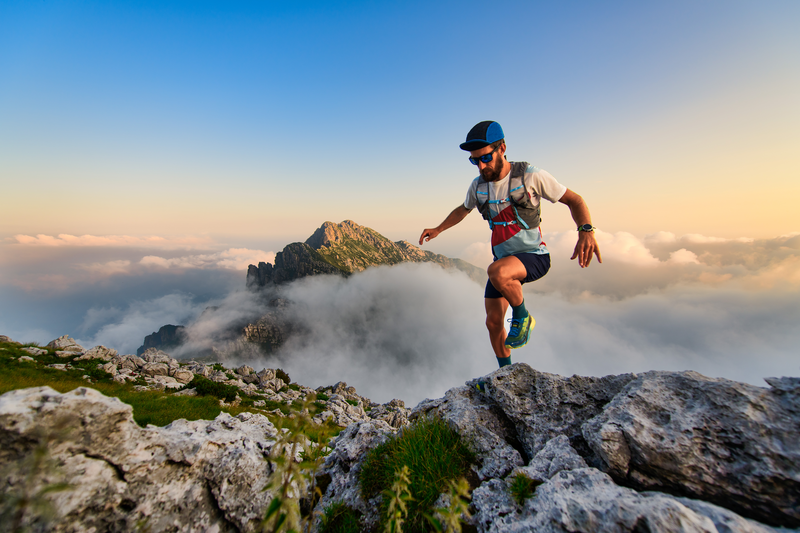
94% of researchers rate our articles as excellent or good
Learn more about the work of our research integrity team to safeguard the quality of each article we publish.
Find out more
ORIGINAL RESEARCH article
Front. Neurosci.
Sec. Perception Science
Volume 19 - 2025 | doi: 10.3389/fnins.2025.1534296
This article is part of the Research Topic Exploring the Neural Mechanisms of Sensory-Cognitive Associations: Bridging Sensory Perception and Higher Cognitive Functions View all articles
The final, formatted version of the article will be published soon.
You have multiple emails registered with Frontiers:
Please enter your email address:
If you already have an account, please login
You don't have a Frontiers account ? You can register here
This study introduces an advanced gustometer to record Gustatory Event-Related Potentials (GERPs) in healthy young adults. We aimed to validate its functionality and reliability. The gustometer includes a programmable controller, a human-machine interface, a modular pump system, and supporting hardware. The Neuro-Audio EEG platform recorded EEG data from 46 volunteers. Psychophysical gustatory tests assessed gustatory function. GERPs were tested using distilled water as a control and sodium chloride solutions (0.3% and 0.6%) as tastants. Tetracaine anesthetized the tongue surface to observe waveform changes and confirm GERP specificity. GERP responses were recorded at the Fz and Cz sites, focusing on the latency and amplitude of GERP P1 and P2 waves and their correlation with psychophysical test results. No stable waveforms were recorded with distilled water. All subjects displayed stable GERP waveforms following salty stimulation. These waveforms disappeared post-anesthesia, confirming GERP specificity. The recorded GERP comprised P1-N1-P2 components. The latency of P1 and P2 waves decreased with increasing salt concentration (p < 0.05). No significant differences in latency were observed between the Pz and Cz sites. Additionally, 48% of subjects showed increased P1-N1 and P2-N2 amplitudes with higher salty stimulation. The latency of P1-N1 and P2-N2 positively correlated with psychophysical test results. This novel gustometer effectively evoked reliable GERP waveforms. The study validated the consistency of GERP amplitude and latency with psychophysical tests, highlighting the gustometer's potential for clinical and research applications in gustatory system.
Keywords: gustatory event-related potentials, Gustometer, Taste, Salty stimulation, Psychophysical tests
Received: 25 Nov 2024; Accepted: 10 Mar 2025.
Copyright: © 2025 Chen, Zhou, Li and Liu. This is an open-access article distributed under the terms of the Creative Commons Attribution License (CC BY). The use, distribution or reproduction in other forums is permitted, provided the original author(s) or licensor are credited and that the original publication in this journal is cited, in accordance with accepted academic practice. No use, distribution or reproduction is permitted which does not comply with these terms.
* Correspondence:
Jianfeng Liu, China-Japan Friendship Hospital, Beijing, China
Disclaimer: All claims expressed in this article are solely those of the authors and do not necessarily represent those of their affiliated organizations, or those of the publisher, the editors and the reviewers. Any product that may be evaluated in this article or claim that may be made by its manufacturer is not guaranteed or endorsed by the publisher.
Research integrity at Frontiers
Learn more about the work of our research integrity team to safeguard the quality of each article we publish.