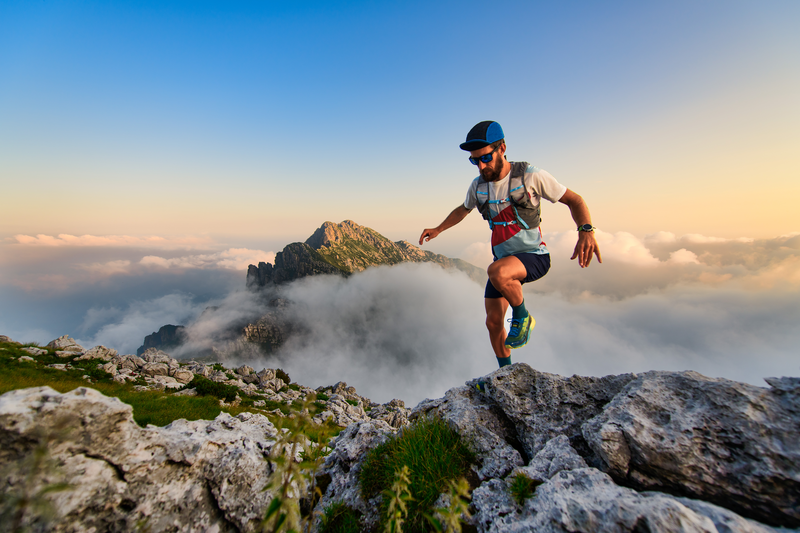
94% of researchers rate our articles as excellent or good
Learn more about the work of our research integrity team to safeguard the quality of each article we publish.
Find out more
REVIEW article
Front. Neurosci.
Sec. Neurodegeneration
Volume 19 - 2025 | doi: 10.3389/fnins.2025.1502417
This article is part of the Research Topic Exercise-Induced Neuroplasticity in Neurodegeneration Diseases Volume II View all 4 articles
The final, formatted version of the article will be published soon.
You have multiple emails registered with Frontiers:
Please enter your email address:
If you already have an account, please login
You don't have a Frontiers account ? You can register here
This review aimed to elucidate the mechanisms through which (i) physical activity (PA) enhances neuroplasticity and cognitive function in neurodegenerative disorders, and (ii) identify specific PA interventions for improving cognitive rehabilitation programs. We conducted a literature search in PubMed, Medline, Scopus, Web of Science, and PsycINFO, covering publications from January 1990 to August 2024. The search strategy employed key terms related to neuroplasticity, physical exercise, cognitive function, neurodegenerative disorders, and personalized physical activity. Inclusion criteria included original research on the relationship between PA and neuroplasticity in neurodegenerative disorders, while exclusion criteria eliminated studies focusing solely on pharmacological interventions. The review identified multiple pathways through which PA may enhance neuroplasticity, including releasing neurotrophic factors, modulation of neuroinflammation, reduction of oxidative stress, and enhancement of synaptic connectivity and neurogenesis. Aerobic exercise was found to increase hippocampal volume by 1-2% and improve executive function scores by 5-10% in older adults. Resistance training enhanced cognitive control and memory performance by 12-18% in elderly individuals. Mind-body exercises, such as yoga and tai-chi, improved grey matter density in memory-related brain regions by 3-5% and enhanced emotional regulation scores Biçimlendirdi: Vurgulu Biçimlendirilmiş: Aralık Sonra: 0 nk, Satır aralığı: tek Biçimlendirdi: Vurgulu Biçimlendirdi: Vurgulu 2 by 15-20%. Dual-task training improved attention and processing speed by 8-14% in individuals with neurodegenerative disorders. We also discuss the potential role of AI-based exercise and AI cognitive training in preventing and rehabilitating neurodegenerative illnesses, highlighting innovative approaches to personalized interventions and improved patient outcomes. PA significantly enhances neuroplasticity and cognitive function in neurodegenerative disorders through various mechanisms. Aerobic exercise, resistance training, mind-body practices, and dual-task exercises each offer unique cognitive benefits. Implementing these activities in clinical settings can improve patient outcomes. Future research should focus on creating personalized interventions tailored to specific conditions, incorporating personalized physical exercise programs to optimize cognitive rehabilitation.
Keywords: Cognitive rehabilitation, hippocampal volume, neurotrophic factors, Oxidative Stress, Personalized interventions, synaptic connectivity Aralık Önce: 0 nk, Sonra: 0 nk Aralık Önce: 0 nk Biçimlendirdi: Yazı tipi: (Varsayılan) Times New Roman, Vurgulu Biçimlendirilmiş: Aralık Önce: 0 nk Biçimlendirdi: Yazı tipi: (Varsayılan) Times New Roman
Received: 26 Sep 2024; Accepted: 04 Feb 2025.
Copyright: © 2025 Ezzdine¹, Dhahbi, Dergaa, CEYLAN, Guelmami, BEN SAAD, Chamari, Stefanica and El Omri. This is an open-access article distributed under the terms of the Creative Commons Attribution License (CC BY). The use, distribution or reproduction in other forums is permitted, provided the original author(s) or licensor are credited and that the original publication in this journal is cited, in accordance with accepted academic practice. No use, distribution or reproduction is permitted which does not comply with these terms.
* Correspondence:
Halil Ibrahim CEYLAN, Physical Education of Sports Teaching Department, Faculty of Kazim Karabekir Education, Ataturk University, Erzurum, Türkiye
Valentina Stefanica, Polytechnic University of Bucharest, Bucharest, Romania
Disclaimer: All claims expressed in this article are solely those of the authors and do not necessarily represent those of their affiliated organizations, or those of the publisher, the editors and the reviewers. Any product that may be evaluated in this article or claim that may be made by its manufacturer is not guaranteed or endorsed by the publisher.
Research integrity at Frontiers
Learn more about the work of our research integrity team to safeguard the quality of each article we publish.