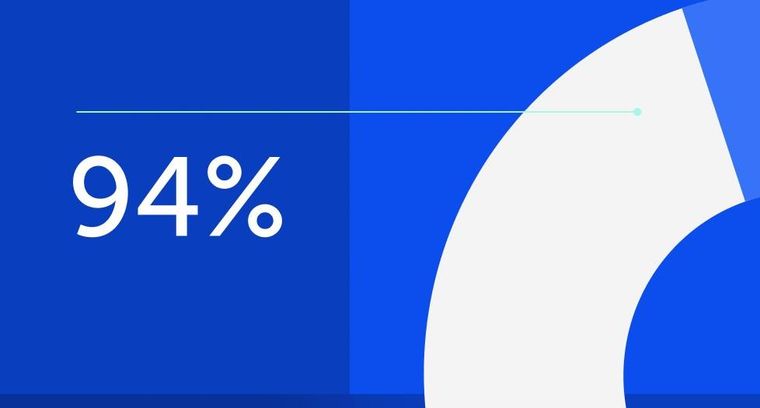
94% of researchers rate our articles as excellent or good
Learn more about the work of our research integrity team to safeguard the quality of each article we publish.
Find out more
PERSPECTIVE article
Front. Neurosci., 22 January 2025
Sec. Neurodevelopment
Volume 19 - 2025 | https://doi.org/10.3389/fnins.2025.1453025
Attention-deficit/hyperactivity disorder (ADHD) is a heterogenous disorder, commonly described for presenting difficulties in sustained attention, response inhibition, and organizing goal-oriented behaviors. However, along with its traditionally described executive dysfunction, more than half of the children diagnosed with ADHD have been reported to show difficulties with gross and fine motor skills, albeit motor impairments in ADHD continue to be a neglected area of clinical attention. The rapidly growing field of the clinical cognitive neuroscience of the cerebellum has begun to relate cerebro-cerebellar circuits to neurodevelopmental disorders. While the cerebellum’s role in motor function, such as balance, motor coordination, and execution, is well recognized, ongoing research has evidenced its additional and fundamental role in neurocognitive development and executive function, including attention and social cognition, which are all areas of impairment commonly found in ADHD. Interestingly, neuroimaging studies have consistently shown differences in cerebellar volume and functional connectivity between ADHD and typically developing children. Furthermore, methylphenidate is known to act at the cerebellar level, as intrinsic cerebellar dopaminergic systems involved in attention and motor function have been identified. This article reviews some of the main findings linking cerebellar dysfunction to ADHD behavioral symptoms and incorporates the cerebellum as a possible neurological basis and differentiating indicator within the condition. We suggest considering more rigorous assessments in future ADHD studies, including cerebellar-associated skill evaluations to correlate with symptom severity and other detected outcomes, such as executive dysfunction, and study possible associative patterns that may serve as more objective measures for this diagnosis.
Attention-deficit/hyperactivity disorder (ADHD) is one of the most prevalent conditions in pediatric psychopathology, estimated to affect approximately 3 to 9.5% of the global population (Polanczyk et al., 2007; Thomas et al., 2015), predominantly affecting school-aged children. Its characteristic symptoms, inattention, hyperactivity, and impulsivity (American Psychiatric Association, 2022) are often explained due to impairments in neuropsychological executive functions such as response inhibition, working memory, and inhibitory control (Barkley, 1997; Martinussen et al., 2005; Willcutt et al., 2005). Another prominent feature of ADHD is that it is often accompanied by a high percentage of comorbid disorders across the lifespan. In adulthood, between 65 and 89% of patients with ADHD suffer from one or more additional psychiatric disorders, such as mood and anxiety disorders, substance use disorders, and personality disorders (Sobanski, 2006). In younger populations, ADHD is frequently associated with psychiatric comorbidities, including learning disorders, conduct disorders, anxiety, and depression (Kessler et al., 2006). ADHD has been frequently associated with motor deficiencies, with 45–70% of children diagnosed with ADHD reported to show difficulties in both gross and fine motor skills (Kaiser et al., 2015; Pitcher et al., 2003; Sayal et al., 2018). Balance and bilateral coordination (including manual dexterity) are the most commonly reported areas of motor function impairment (Buderath et al., 2009; Fliers et al., 2008; Ghanizadeh, 2010; Goulardins et al., 2013; Karaokur et al., 2017; Konicarova et al., 2014).
Historically, ADHD has been associated with disturbances in the maturation of executive function (EF) due to frontostriatal and frontoparietal dopamine-signaling deficits (Aboitiz et al., 2014), while motor disturbances were not initially considered part of its diagnostic criteria. Past hypotheses explaining the motor difficulties often found in individuals with ADHD have taken two main approaches: The first attributes these motor difficulties to the basic symptomatic triad of ADHD, influenced by inattention (Ghanizadeh, 2010) and/or impulsivity (Tseng et al., 2004). The severity of inattentiveness score has been found to correlate with and predict poor motor performance, such as coordination problems (Fliers et al., 2008), as well as deficits in fine motor skills or writing (Ghanizadeh, 2010; Kaiser et al., 2015). Piek et al. (2004) explored the link between inattention, motor ability, and aspects of EF in children with ADHD (6–15 years of age) and reported strong correlations between poor motor coordination and inattention but weaker correlations with EF, despite the fact that research has established an undisputed association between EF and ADHD (Willcutt et al., 2005). Tseng et al. (2004) found that measures of sustained attention, along with additional impulse control, were good concurrent predictors of fine and gross motor skills in 6- to 11-year-olds with ADHD, with attention and impulse control being the two best predictors of fine motor skill performance.
The second hypothesis explaining motor problems in ADHD suggests these may be secondary to a comorbidity, particularly developmental coordination disorder (DCD), defined as severe impairment in learning and execution of coordinated motor skills (American Psychiatric Association, 2022), where an estimated 50% of children diagnosed with ADHD have been found to meet the criteria for DCD (Montes-Montes et al., 2021). Notwithstanding, even when ADHD children do not meet the criteria of DCD, they continue to show weaker motor skills than typically developing children when evaluated (Klupp et al., 2021; Manicolo et al., 2016; Poblano et al., 2014).
A few decades ago, a line of research emerged suggesting cerebellar dysfunction to ADHD symptoms after Castellanos et al. reported significantly smaller cerebellar hemispheric volumes in ADHD children using quantitative brain magnetic resonance imaging (Castellanos, 2002; Castellanos et al., 1996). Following neuroimaging studies describing structural differences in the brain of ADHD children when compared to typical peers continued to establish atypical cerebellar volume as one of the most consistent findings in the neuroimaging literature on ADHD (Pereira-Sanchez and Castellanos, 2021; Valera et al., 2007) particularly with gray matter reductions in the posterior-inferior lobules, VIII to X (Berquin et al., 1998; Bledsoe et al., 2011; Mostofsky et al., 1998), and functional cerebellar dysconnectivity (Feng et al., 2023; Tomasi and Volkow, 2012; Wang et al., 2022).
The cerebellum is considered a vital component in the human brain that has traditionally been studied for its central role in motor movement regulation and balance control. It is known to integrate different sensory inputs such as visual, vestibular, and somatosensory information responsible for motor coordinated movements, postural control, and the planning of sequenced actions (Horak and Macpherson, 1996), contributing to the quality of movement in conjunction with the prefrontal cortex (PFC) and basal ganglia (Welniarz et al., 2021). The cerebellum is also known to play a pivotal role in fine motor such as bilateral manual dexterity (Soteropoulos and Baker, 2008), speech articulation (Salman and Tsai, 2016), and the realization of coordinated eye movements including the control of fixed eye movements through projections from the frontal eye field (Pretegiani et al., 2018). While the cerebellum is often described as a structure mainly involved in motor coordination, it has a well-established role in numerous facets of cognitive, emotional, and behavioral functioning (Koziol et al., 2014) which are all areas reported as dysfunctional in ADHD. To date, there is enough evidence to sustain that the cerebellum could be playing a central role in ADHD outcomes and symptomatic behaviors, particularly in children. In this study, we will review some main findings that link the cerebellum directly to all symptoms commonly reported in ADHD.
One of the most currently accepted hypotheses of the underlying physiopathology in ADHD is the deficit in dopamine-signaling mechanisms, associated with genetic factors encoding for dopamine receptor DRD4 and dopamine transporter DAT1, affecting PFC, basal ganglia, thalamus, and amygdala circuits, which participate in EF, suggesting a reduction of executive control associated with abnormalities in frontoparietal and frontostriatal network function (Antshel et al., 2014) which would directly impact working memory, the ability to sustain attention to goal-directed tasks, and self-regulate social–emotional behaviors. Studies using neuropsychological measures reported that overall cognitive abilities are significantly lower among ADHD subjects, including significant impairment in all EF tasks (Frazier et al., 2004).
While the cerebellum’s role in motor function is well recognized, a substantial portion of the cerebellum is associated with non-motor functions connected to different large-scale networks in the neocortex, including frontal and parietal regions typically involved in high-order cognitive processing, providing the means through which association areas and the cerebellum may influence each other’s operation (Ramnani, 2012). Resting-state functional connectivity MRI studies show that cerebral networks involved in movement, attention, and limbic valence, as well as frontoparietal and default systems associated with creativity and imagination, map onto the cerebellum with topographic specificity (Buckner et al., 2011). The dorsal attention, ventral attention, frontoparietal, default mode, and salience networks map onto focal areas within the posterior lobe of the cerebellum. Lobules VI and Crus I are engaged in language and verbal working memory; lobule VI in spatial tasks; lobules VI, Crus I, and VIIB are activated by executive functions such as working memory, planning, organizing, and strategy formation; and vermal lobules VI and VII are involved in emotional processing. Language is heavily right lateralized and spatial functions left-lateralized, reflecting crossed cerebro-cerebellar projections (Schmahmann, 2019). Studies have demonstrated the close relationship between cerebellar function and working memory (Stein, 2021), attention modulation (Mannarelli et al., 2019), and higher order executive functioning (Pretegiani et al., 2018) through cortico-cerebellar circuits (Zhu et al., 2023), including bilateral projections between the neocerebellum and dorsolateral PFC (Habas, 2021) as well as the basal ganglia (Caligiore et al., 2017). These cortico-cerebellar networks have been described as critical during participation in tasks that are difficult and novel, especially those requiring unpredictable and quick adjustments, demanding sustained attention (Diamond, 2000), directed visual attention and working memory processes (Brissenden and Somers, 2019), and during social interactions (Li et al., 2023).
Studies with patients with cerebellar lesions have been found to show deficits in the ability to shift attention (Akshoomoff and Courchesne, 1994) and direct goal-oriented behavior (Beuriat et al., 2022; Botez et al., 1989). Cerebellar symptoms have also been associated with difficulties in spatial working memory in children and adolescents with ADHD (Ferrin and Vance, 2012). Therefore, the cortico-cerebellar network allows the cerebellum not only to contribute to the coordination of movements, as once thought, but also to the modulation and integration of all higher cortical functions, including those compromised in ADHD.
Children with ADHD have consistently shown poor performance on motor skill tests when compared to typically developing children (Brossard-Racine et al., 2012; Goulardins et al., 2013), particularly in activities requiring fluent coordinated movements (Fliers et al., 2008; Kaiser et al., 2015), handwriting (Brossard-Racine et al., 2011), bimanual coordination (Piek et al., 1999), and gait, balance, and postural control (Buderath et al., 2009; Mao et al., 2014; Simmons et al., 2020). All of these areas of motor performance are directly associated with cerebellar function. Even DCD, a common comorbidity to ADHD, that specifically impairs a child’s ability to coordinate movement and learn motor skills to perform everyday activities, has also been associated with cerebellar dysfunction (Gill et al., 2022; Mariën et al., 2010). The cerebellum has been considered essential during motor learning and is strongly implicated in detecting and correcting motor errors (Popa et al., 2016). These motor errors are defined as the discrepancy between the predicted consequences of motor commands and the sensory feedback, which are encoded in the cerebellar cortex in the context of a forward internal model that generates predictions about the upcoming movement and drives learning and adaptation. Cerebellar Purkinje cells have been found not only to drive motor learning but also to expand the capacity of motor circuits (Nguyen-Vu et al., 2013).
The cerebellum is responsible for the complex integration of multiple postural information coming from vestibular, visual, and proprioceptive inputs. Cerebellar circuitry regulates static and dynamic parameters playing a key role in establishing balance stability and steady gait (Dijkstra et al., 2020). Postural instability and sway abnormalities are commonly reported in children with ADHD, and studies have previously shown a relationship between postural control alterations and the cerebellum in ADHD (Hove et al., 2015). During static balance assessments, ADHD-diagnosed children show impaired postural control evidenced in an increased sway of their center of pressure (Cheng and Wang, 2007) and significantly smaller loss of stability area (Isaac et al., 2017) than their typical peers. In dynamic motor control, existing evidence in relation to gait (Bucci et al., 2016; Buderath et al., 2009; Kim et al., 2017; Shum and Pang, 2009) shows that children with ADHD walk with less consistency of speed (Meachon et al., 2023), less rhythmic and less automatic gait (Leitner et al., 2007), greater variability of cadence and step time, and greater variability for stride length when walking at an increased stepping rate (Simmons et al., 2020).
Cerebellar involvement in complex manual tasks has also been documented (Kühn et al., 2012; Rau et al., 2024), including studies of neural correlates in handwriting during childhood (Palmis et al., 2021; Zhang et al., 2022). Regarding upper extremity motor control, children with ADHD have been found to show less fluidity in arm movement (Van and Thomas, 2002), reduced coordination in manual dexterity (Brossard-Racine et al., 2012), and slower performance in fine motor tasks (Scharoun et al., 2013). Studies further find that children with ADHD have less legible handwriting (Mokobane et al., 2019; Shen et al., 2012), with a tendency to be less accurate in keeping letter size within boundaries, spacing, and alignment (Tucha and Lange, 2001).
In addition to gross and fine motor, poor eye movement performance has frequently been reported in children with ADHD (Goto et al., 2010) when compared to typically developing children. Children with ADHD show a larger number of errors during anti-saccade tasks as well as frequent intrusive saccades during smooth pursuit and fixation (Fried et al., 2014; Maron et al., 2021), less precise and stable movements during visual tracking (Munoz et al., 2003; Slaats-Willemse et al., 2005), and need more time to complete oculomotor tasks than those without ADHD (Sherigar et al., 2023). The cerebellum, in particular, is a structure of utmost importance in optimizing goal-directed eye movements such as saccadic and smooth pursuit eye movements to stabilize the images of moving targets (Prsa and Their, 2022). Animal and human studies evidenced that damage to specific parts of the cerebellum causes imprecise visually guided eye movements and smooth pursuit deficits, such as those of the flocculus/paraflocculus or those of the vermis including lobule VI, VII, the uvula, and the deep cerebellar nuclei (Baier et al., 2009).
Timing of motor actions is another aspect that has been found to be impaired in ADHD (Zelaznik et al., 2012), showing slower timing of movements and greater variability between sequences of actions (Chen et al., 2013; Noreika et al., 2013; Shiels Rosch et al., 2013; Slater and Tate, 2018), especially when movements require more planning and complex motor coordination (Kaiser et al., 2015). Along with the motor cortices and basal ganglia, the cerebellum plays a key role in regulating the timing of self-initiated movements and predicting the timing of periodic events (Tanaka et al., 2021) which allows for the execution of synchronized movements such as spontaneous dance and clapping to music (Bo et al., 2008). The cerebellum appears to enable these controls by linking sensory inputs and motor timing, particularly in the range from a few hundred milliseconds to a second (Mauk and Buonomano, 2004). Abnormalities in reproducing temporal durations, with intervals as brief as 400 ms, have been documented in children and adolescents with ADHD, showing impairments in duration discrimination, and the precision and reliability with which they reproduced the intervals (Toplak et al., 2006). Castellanos and Tannock (2002) proposed a temporal processing candidate endophenotype for ADHD linked to deficits in time estimation, emphasizing that intra-individual variability in task performance is “the most striking clinical characteristic in ADHD.” These authors further state that temporal variability in symptom expression in ADHD is linked to cerebellar dysfunction. Timing deficits in ADHD are furthermore supported by functional neuroimaging studies that show dysfunctions in the key inferior fronto-striato-cerebellar and frontoparietal networks that mediate timing functions (Noreika et al., 2013).
In addition to executive dysfunction and motor impairment, it is widely acknowledged that social functioning and social cognition are also altered in children (Arango-Tobón et al., 2023) and adults with ADHD (Morellini et al., 2022). These alterations often lead to socially inappropriate responses, resulting in alterations in their adaptive behavior. Social cognition impairments in ADHD have been observed in the domains of theory of mind (ToM) (Parke et al., 2021), demonstrated by inferior performance in understanding intentional behaviors (Mary et al., 2016; Mohammadzadeh et al., 2016), empathy and emotional intelligence. Children with ADHD have shown poorer performance in tasks measuring emotional prosody (especially in perceiving emotionally angry statements) and intermodal skills (Kis et al., 2017; Uekermann et al., 2010), as well as recognizing facial emotions, with the most significant impairments observed in negative emotions such as anger and fear (Bora and Pantelis, 2016).
Schmahmann and Sherman (1998) introduced the cerebellar cognitive affective syndrome (CCAS), characterized by disturbances in executive function, visual–spatial processing, linguistic skills, and regulation of affect, linking this syndrome with cerebellar posterior lobe lesions (Schmahmann, 2019). Since then, studies evidencing cerebellar involvement in the regulation of affective reactions as well as in forming the association between sensory stimuli and their emotional values have increased to the point where cerebellar functional topography for emotional and social processing has become well-established (D’Agata and Orsi, 2022).
The cerebellar vermis, which is the principal target of limbic connections (Berntson and Torello, 1982), has been implicated in the modulation of emotions (Stoodley and Schmahmann, 2010), and lobules VI and VII (crus I–II), in the posterolateral cerebellar hemisphere, have been implicated in social cognition that involves more complex cognitive processes such as empathy (Sokolov, 2018). An increasing amount of evidence has confirmed that the cerebellum is actively involved in emotional recognition specifically processing facial expressions of negative emotion (Ferrucci et al., 2012), particularly in fear-related processes, possibly due to reciprocal cerebellar connections with the amygdala (Turner et al., 2007).
Recent studies have highlighted the function of the cerebellum in supporting social cognition and interaction, through function-specific mentalizing and somatomotor processes (Van Overwalle et al., 2015) using encoded internal models that reproduce the dynamic properties of the body involving sequencing and planning of action, to automate and fine-tune not only voluntary motor processes but also cognitive processes (Ito, 2008) in collaboration with the cortex, such as related ToM processes based on the prediction of sequential events. A previous meta-analysis by Van Overwalle et al. (2014) of fMRI studies found robust clusters of activation in the cerebellum recruited during social-cognitive processes that overlap with cerebellar non-social functions such as motor functions, emotions, executive control, and language. Therefore, in addition to balance and motor coordination functions, non-motor behaviors, including cognition and social behavior, appear to be equally affected by deficits in cerebellar networks.
One theoretical model developed to explain ADHD etiopathogenesis is the state-regulation theory introduced by Sanders (1983), which suggests that a decreased ability to regulate brain arousal may contribute to the higher-level cognitive deficits in ADHD. Arousal state regulation in the brain is related to the release of the catecholamine norepinephrine (NE) and dopamine in the PFC (Arnsten and Li, 2004). NE has marked effects on PFC function, and small changes in the autonomic arousal systems can markedly alter the connectivity of PFC networks impacting EF and cognitive performance (Arnsten and Pliszka, 2011). Autonomic dysregulated functions would directly impact brain arousal through locus coeruleus (LC) connectivity and NE pathways, coordinated by the central autonomic network (CAN), altering the overall efficiency of cognitive performance (Diamond, 2005) leading to both executive dysfunction and hyperactivity symptoms (Sergeant, 2000). Neurophysiological studies measuring activity from the autonomic sympathetic nervous system (such as heart rate, electrodermal responses, and pupillary dilatation) have evidenced dysfunction in individuals with ADHD, more often in the direction of sympathetic hypo-arousal than hyper-arousal, particularly at rest and during tasks requiring response regulation and sustained attention (Bellato et al., 2020).
Cerebellar structures were associated with autonomic cardiovascular functions by early experiments, which showed that stimulation of the cerebellar vermis interferes with autonomic reactions, by limiting blood pressure oscillations and inducing hyperventilation (Moruzzi, 1940; Nisimaru, 2004). Further studies found anatomical connections between the fastigial nucleus and the hypothalamus suggesting cerebellar vermis involvement in autonomic and emotional regulatory functions (Snider, 1950). Later studies confirmed the existence of a prominent NE pathway from the brainstem nucleus LC to all regions of the cerebellar cortex and deep cerebellar nuclei (Olson and Fuxe, 1971), recognizing the cerebellum as a key region of the CAN (Benarroch, 1993) and demonstrating neuromodulatory effects of NE on the spontaneous and evoked discharge of Purkinje neurons (Freedman et al., 1977). NE is capable of producing a relative or absolute enhancement of stimulus-driven activity in the primary output cells of the cerebellar cortex, whereby Purkinje cells exhibiting little or no response to peripheral stimuli become responsive to such inputs in the presence of NE (Moises et al., 1990). This evidence indicates that NE can produce a spectrum of effects on the spontaneous and evoked discharge of Purkinje neurons, all of which serve to regulate the responsiveness of these cells to synaptically driven inputs (Waterhouse and Navarra, 2019). The net outcome of LC-NE regulation of cerebellar circuit operations may be that voluntary and reflex motor activities, cognitive operations, and emotive responses are optimized with respect to ongoing goal-directed behaviors and unexpected environmental challenges (Predale et al., 2023) which allows for organized and adapted behavior in a stable and predictable way. Interestingly, one of the most striking clinical characteristics described by clinicians of ADHD in addition to the frequent lapses of attention is the high prevalence of intra-individual variability and inconsistency in performance (Castellanos and Tannock, 2002), which may possibly be related to cerebellar dysfunction in modulating emotional signals and behavior through extensive cerebello-cortical and cerebello-subcortical circuits.
Recent data are leading to profound changes in our understanding of cerebro-cerebellar circuits, which are now considered essential for the functional regulation of various neocortex areas, including PFC cognitive circuits, as well as of the hypothalamus and autonomic network, and the limbic system (Rudolph et al., 2023).
Considering the evidence that the cerebellum works as an integrated system with the cortex (including prefrontal and parietal areas), the basal ganglia, and the thalamus in a variety of functions—from the motor to cognitive tasks of varying complexity (Caligiore et al., 2017)—and that these areas play a critical role in attention, behavior organization, affect regulation, motor planning, and coordination (Cortese, 2012; Rudolph et al., 2023), we suggest that symptoms commonly reported in ADHD, such as executive function variability, balance and motor coordination impairments, difficulties in emotional and arousal regulation, and social adaptation problems, can largely be explained by cortico-cerebellar network deficiencies.
Furthermore, common psychiatric comorbidities associated with ADHD have also been related to cerebellar dysfunction. Anxiety disorders, being considered the most frequent and associated with worse clinical presentation of ADHD symptoms (Quenneville et al., 2022), have been linked to abnormalities in cerebellar vermis activity and vermis-amygdala connectivity (Chin and Augustine, 2023). Mood disorders and externalizing disorders, such as oppositional defiant disorder and personality disorders, are also highly prevalent comorbidities in ADHD (Katzman et al., 2017) that have been linked to cerebellar dysfunction, where studies in patients with cerebellar lesions have shown states of depression, maniac, and personality alterations (Lupo et al., 2019) suggesting that the cerebellum should be considered a key structure involved in the regulation of mood and emotion.
Another important aspect to consider is that the current DSM-5 classifies ADHD a neurodevelopmental condition (American Psychiatric Association, 2022), as its symptoms exhibit normative change over time (Martel et al., 2016). Studies show typical cortical development patterns in ADHD, but these are delayed by as much as 2–3 years, depending on the specific cortical region. This delay in both cortical thickness and surface area suggests a global perturbation in the mechanisms that guide cortical maturation (Shaw et al., 2007, 2012). Therefore, higher-order networks may not be directly impaired in ADHD but influenced by other circuits which may be altering the optimal development and performance of higher-order cognitive functions. The human brain does not mature in a uniform manner, and the cerebellum has been shown to be one of the first structures to start cellular differentiation. Between 20 and 40 weeks of gestation, the cerebellum undergoes an exuberant period of growth (Clouchoux et al., 2012), with a rapid development period in the third trimester of pregnancy and in the first postnatal year. This growth pattern strongly suggests the presence of a critical period for cerebellar development, making it especially vulnerable to genetic and environmental stressors disrupting development (Limperopoulos et al., 2005). Research findings suggest that the cerebellum plays a critical role in motor, cognitive, and social–behavioral development, possibly via modulatory effects on the developing cerebral cortex, guiding the maturation of remote non-motor neural circuitry and influencing cognitive learning (Gaiser et al., 2024). Cerebellar damage can cause a striking volume reduction in the opposite hemisphere of the cerebrum (Limperopoulos et al., 2005), suggesting that the cerebellum may have a direct influence on cortical maturation (Olson et al., 2023). These findings hint that early in development, the rapid growth of the cerebellum may be joined to PFC growth and activity and thus could be a key node in various neurodevelopmental disorders, as already suggested for autism spectrum disorder (Wang et al., 2014) and possibly for ADHD.
In clinical pharmaceutical treatment guidelines, methylphenidate hydrochloride (MPH) is recommended as a first-line medication for ADHD. The exact mechanism of action of MPH is not completely understood, but they are presumed to act through the dopaminergic and adrenergic pathways of the frontostriatal circuits in the brain by blocking the reuptake of dopamine into the presynaptic cleft (Nair and Moss Baylor, 2009). However, dopamine networks are not limited to frontostriatal networks. Along with the dense cerebellar connections to the frontal cortex and basal ganglia, an independent dopaminergic network exists within the cerebellum (Li et al., 2023), with high concentrations of dopamine transporters found particularly in the posterior-inferior vermis (Flace et al., 2021; Melchitzky and Lewis, 2000). Nonetheless, the effects of MPH modulating cerebellar function in ADHD have been documented (Czerniak et al., 2013; Inci Kenar et al., 2017), leading to suggest that behavioral and even motor modifications in children with ADHD taking MPH may be explained through outcomes that involve the cerebellum (Brossard-Racine et al., 2012; Bucci et al., 2016).
Research relating ADHD and cerebellar function so far has shown a high correlation between cerebellar volume reduction and ADHD symptoms, suggesting a specific contribution of the cerebellum in the etiology of this disorder (Stoodley, 2016). Goetz et al. (2017) associated impaired balance, reaction times, and cerebellar dysfunction in children with ADHD and found correlations between poor dynamic balance and reaction time consistency, concluding that impaired balance control and cognitive functioning might share a common dysfunction at the cerebellar level in ADHD. Similarly, Bledsoe et al. (2011) studied correlations between ADHD symptomatology and reductions in cerebellar vermis, finding that a large portion of the variance in reported ADHD behavior was predicted by reduced volume in posterior-inferior cerebellar vermis, suggesting the vermis of the cerebellum may be a specific structure related to symptoms of hyperactivity and impulsivity and in the modulation of attention.
As mentioned, several lines of research confirm the involvement of cerebellar structures and circuits in all areas of ADHD diagnostic outcomes. This new appreciation of cerebellar incorporation into circuits that subserve cognition and emotion mandates a deeper understanding of the cerebellum, beyond the motor domain, and its impact on neurodevelopmental disorders such as ADHD, providing potential for novel cerebellar-based approaches to diagnosis and therapy.
The ADHD is a highly complex and heterogeneous disorder in terms of its multidimensional outcomes, multi-factorial etiological risk factors, diverse neurocognitive impairments, and comorbid problems (Nigg et al., 2020). A large number of controversial results continue to exist within the study of ADHD biological and performance markers, as it is based exclusively on behavioral symptoms where the bottom-line issue of heterogeneity and variable severity has not been directly addressed.
Several lines of research confirm the involvement of the cerebellum in ADHD symptoms and behaviors, considering the cerebellum as a key factor in guiding the maturation of remote non-motor neural circuitry and influencing cognitive development. Evidence has indicated that the development of the cerebellum occurs very early in life and is more dependent upon environmental factors than most other brain regions (Giedd et al., 2007), and sensitive-period disruption of cerebellar-brain communication may have an impact on higher cognitive functions.
Future considerations should focus more specifically on the multidimensional assessment of functions associated with specific cerebellar areas or circuits through neuropsychological evaluations linked to neuroimaging studies, and assessment of functions that particularly nurture cerebellar growth such as vestibular and proprioceptive afferences, to establish correlations that might elucidate patterns of symptom expression. We suggest evaluating all areas involved in cerebellar function through motor, sensory, cognitive, and social performance, linked to neuroimaging results, to explore possible correlations that may be indicative of cerebellar-based ADHD endophenotypes and be used as indicators to monitor treatment response.
Future studies should focus on assessing the large spectrum of impaired performance areas simultaneously and search for correlated patterns of grouped symptoms that will not only complete the diagnosis but serve as a quantitative determination of endophenotypes that can lead to more targeted and efficient treatment programs.
The original contributions presented in the study are included in the article/supplementary material, further inquiries can be directed to the corresponding author.
VI: Conceptualization, Writing – original draft, Writing – review & editing. VL: Writing – review & editing. ME: Writing – review & editing.
The author(s) declare that financial support was received for the research, authorship, and/or publication of this article. This study was supported by grants from Agencia Nacional de Investigación y Desarrollo (ANID) in Chile (folio 21231682) and funds from the Research Department of Adolfo Ibañez University.
The authors declare that the research was conducted in the absence of any commercial or financial relationships that could be construed as a potential conflict of interest.
The author(s) declared that they were an editorial board member of Frontiers, at the time of submission. This had no impact on the peer review process and the final decision.
All claims expressed in this article are solely those of the authors and do not necessarily represent those of their affiliated organizations, or those of the publisher, the editors and the reviewers. Any product that may be evaluated in this article, or claim that may be made by its manufacturer, is not guaranteed or endorsed by the publisher.
Aboitiz, F., Ossandón, T., Zamorano, F., Palma, B., and Carrasco, X. (2014). Irrelevant stimulus processing in ADHD: catecholamine dynamics and attentional networks. Front. Psychol. 5:183. doi: 10.3389/fpsyg.2014.00183
Akshoomoff, N. A., and Courchesne, E. (1994). ERP evidence for a shifting attention deficit in patients with damage to the cerebellum. J. Cogn. Neurosci. 6, 388–399. doi: 10.1162/jocn.1994.6.4.388
American Psychiatric Association (2022). Diagnostic and statistical manual of mental disorders. 5th Edn. Washington, DC: American Psychiatric Association Publishing.
Antshel, K. M., Hier, B. O., and Barkley, R. A. (2014). “Executive functioning theory and ADHD” in Handbook of executive functioning. eds. S. Goldstein and J. A. Naglieri (New York: Springer), 107–120.
Arango-Tobón, O. E., Guevara Solórzano, A., Orejarena Serrano, S. J., and Olivera-La Rosa, A. (2023). Social cognition and prosocial behavior in children with attention deficit hyperactivity disorder: a systematic review. Healthcare 11:1366. doi: 10.3390/healthcare11101366
Arnsten, A., and Li, B. (2004). Neurobiology of executive functions: catecholamine influences on prefrontal cortical functions. Biol. Psychiatry. 57, 1377–1384. doi: 10.1016/j.bps.2004.08.019
Arnsten, A. F. T., and Pliszka, S. R. (2011). Catecholamine influences on prefrontal cortical function: relevance to treatment of attention deficit/hyperactivity disorder and related disorders. Pharmacol. Biochem. Behav. 99, 211–216. doi: 10.1016/j.pbb.2011.01.020
Baier, B., Stoeter, P., and Dieterich, M. (2009). Anatomical correlates of ocular motor deficits in cerebellar lesions. Brain 132, 2114–2124. doi: 10.1093/brain/awp165
Bellato, A., Arora, I., Hollis, C., and Groom, M. J. (2020). Is autonomic nervous system function atypical in attention deficit hyperactivity disorder (ADHD)? A systematic review of the evidence. Neurosci. Biobehav. Rev. 108, 182–206. doi: 10.1016/j.neubiorev.2019.11.001
Benarroch, E. E. (1993). The central autonomic network: functional organization, dysfunction, and perspective. Mayo Clin. Proc. 68, 988–1001. doi: 10.1016/S0025-6196(12)62272-1
Berntson, G. G., and Torello, M. W. (1982). The paleocerebellum and the integration of behavioral function. Physiol. Psychol. 10, 2–12. doi: 10.3758/BF03327003
Berquin, P. C., Giedd, J. N., Jacobsen, L. K., Hamburger, S. D., Krain, A. L., Rapoport, J. L., et al. (1998). Cerebellum in attention-deficit hyperactivity disorder: a morphometric MRI study. Neurology 50, 1087–1093. doi: 10.1212/WNL.50.4.1087
Beuriat, P. A., Cristofori, I., Gordon, B., and Grafman, J. (2022). The shifting role of the cerebellum in executive, emotional and social processing across the lifespan. Behav. Brain Funct. 18:6. doi: 10.1186/s12993-022-00193-5
Bledsoe, J. C., Semrud-Clikeman, M., and Pliszka, S. R. (2011). Neuroanatomical and neuropsychological correlates of the cerebellum in children with attention-deficit/hyperactivity disorder-combined type. J. Am. Acad. Child Adolesc. Psychiatry 50, 593–601. doi: 10.1016/j.jaac.2011.02.014
Bo, J., Block, H. J., Clark, J. E., and Bastian, A. J. (2008). A cerebellar deficit in sensorimotor prediction explains movement timing variability. J. Neurophysiol. 100, 2825–2832. doi: 10.1152/jn.90221.2008
Bora, E., and Pantelis, C. (2016). Meta-analysis of social cognition in attention-deficit/hyperactivity disorder (ADHD): comparison with healthy controls and autistic spectrum disorder. Psychol. Med. 46, 699–716. doi: 10.1017/S0033291715002573
Botez, M. I., Botez, T., Elie, R., and Attig, E. (1989). Role of the cerebellum in complex human behavior. Ital. J. Neurol. Sci. 10, 291–300. doi: 10.1007/BF02333774
Brissenden, J. A., and Somers, D. C. (2019). Cortico–cerebellar networks for visual attention and working memory. Curr. Opin. Psychol. 29, 239–247. doi: 10.1016/j.copsyc.2019.05.003
Brossard-Racine, M., Majnemer, A., Shevell, M., Snider, L., and Bélanger, S. A. (2011). Handwriting capacity in children newly diagnosed with attention deficit hyperactivity disorder. Res. Dev. Disabil. 32, 2927–2934. doi: 10.1016/j.ridd.2011.05.010
Brossard-Racine, M., Shevell, M., Snider, L., Bélanger, S. A., and Majnemer, A. (2012). Motor skills of children newly diagnosed with attention deficit hyperactivity disorder prior to and following treatment with stimulant medication. Res. Dev. Disabil. 33, 2080–2087. doi: 10.1016/j.ridd.2012.06.003
Bucci, M. P., Stordeur, C., Acquaviva, E., Peyre, H., and Delorme, R. (2016). Postural instability in children with ADHD is improved by methylphenidate. Front. Neurosci. 10:163. doi: 10.3389/fnins.2016.00163
Buckner, R. L., Krienen, F. M., Castellanos, A., Diaz, J. C., and Thomas Yeo, B. T. (2011). The organization of the human cerebellum estimated by intrinsic functional connectivity. J. Neurophysiol. 106, 2322–2345. doi: 10.1152/jn.00339.2011
Buderath, P., Gärtner, K., Frings, M., Christiansen, H., Schoch, B., Konczak, J., et al. (2009). Postural and gait performance in children with attention deficit/hyperactivity disorder. Gait Posture 29, 249–254. doi: 10.1016/j.gaitpost.2008.08.016
Caligiore, D., Pezzulo, G., Baldassarre, G., Bostan, A. C., Strick, P. L., Doya, K., et al. (2017). Consensus paper: towards a systems-level view of cerebellar function: the interplay between cerebellum, basal ganglia, and cortex. Cerebellum 16, 203–229. doi: 10.1007/s12311-016-0763-3
Castellanos, F. X. (2002). Anatomic magnetic resonance imaging studies of attention-deficit/hyperactivity disorder. Dialogues Clin. Neurosci. 4, 444–448. doi: 10.31887/DCNS.2002.4.4/fxcastellanos
Castellanos, F. X., Giedd, J. N., Marsh, W. L., Hamburger, S. D., Vaituzis, A. C., Dickstein, D. P., et al. (1996). Quantitative brain magnetic resonance imaging in attention-deficit hyperactivity disorder. Arch. Gen. Psychiatry 53:607. doi: 10.1001/archpsyc.1996.01830070053009
Castellanos, F. X., and Tannock, R. (2002). Neuroscience of attention-deficit/hyperactivity disorder: the search for endophenotypes. Nat. Rev. Neurosci. 3, 617–628. doi: 10.1038/nrn896
Chen, Y. Y., Liaw, L. J., Liang, J. M., Hung, W. T., Guo, L. Y., and Wu, W. L. (2013). Timing perception and motor coordination on rope jumping in children with attention deficit hyperactivity disorder. Phys. Ther. Sport 14, 105–109. doi: 10.1016/j.ptsp.2012.03.012
Cheng, J., and Wang, Y. F. (2007). Comparison of postural control between normal and attention deficit hyperactivity disorder boys. Beijing Da Xue Xue Bao Yi Xue Ban 39, 531–534
Chin, P. W., and Augustine, G. J. (2023). The cerebellum and anxiety. Front. Cell. Neurosci. 17:505. doi: 10.3389/fncel.2023.1130505
Clouchoux, C., Guizard, N., Evans, A. C., Du Plessis, A. J., and Limperopoulos, C. (2012). Normative fetal brain growth by quantitative in vivo magnetic resonance imaging. Am. J. Obstet. Gynecol. 206, 173.e1–173.e8. doi: 10.1016/j.ajog.2011.10.002
Cortese, S. (2012). The neurobiology and genetics of attention-deficit/hyperactivity disorder (ADHD): what every clinician should know. Eur. J. Paediatr. Neurol. 16, 422–433. doi: 10.1016/j.ejpn.2012.01.009
Czerniak, S. M., Sikoglu, E. M., King, J. A., Kennedy, D. N., Mick, E., Frazier, J., et al. (2013). Areas of the brain modulated by single-dose methylphenidate treatment in youth with ADHD during task-based fMRI: a systematic review. Harv. Rev. Psychiatry 21, 151–162. doi: 10.1097/HRP.0b013e318293749e
D’Agata, F., and Orsi, L. (2022). “Cerebellum and emotion recognition” in The emotional cerebellum: advances in experimental medicine and biology. eds. M. Adamaszek, M. Manto, and D. J. L. G. Schutter, vol. 1378 (Cham: Springer).
Diamond, A. (2000). Close interrelation of motor development and cognitive development and of the cerebellum and prefrontal cortex. Child Dev. 71, 44–56. doi: 10.1111/1467-8624.00117
Diamond, D. M. (2005). Cognitive, endocrine and mechanistic perspectives on non-linear relationships between arousal and brain function. Nonlinearity Biol Toxicol Med. 3, 1–7. doi: 10.2201/nonlin.003.01.001
Dijkstra, B. W., Bekkers, E. M. J., Gilat, M., de Rond, V., Hardwick, R. M., and Nieuwboer, A. (2020). Functional neuroimaging of human postural control: a systematic review with meta-analysis. In. Neurosci. Biobehav. Rev. 115, 351–362. doi: 10.1016/j.neubiorev.2020.04.028
Feng, Y., Zhu, Y., Guo, X., Luo, X., Dang, C., Liu, Q., et al. (2023). Exploring the potential “brain-cognition-behavior” relationship in children with ADHD based on resting-state brain local activation and functional connectivity. J. Atten. Disord. 27, 1638–1649. doi: 10.1177/10870547231197206
Ferrin, M., and Vance, A. (2012). Examination of neurological subtle signs in ADHD as a clinical tool for the diagnosis and their relationship to spatial working memory. J. Child Psychol. Psychiatry Allied Discip. 53, 390–400. doi: 10.1111/j.1469-7610.2011.02496.x
Ferrucci, R., Giannicola, G., Rosa, M., Fumagalli, M., Boggio, P. S., Hallett, M., et al. (2012). Cerebellum and processing of negative facial emotions: cerebellar transcranial DC stimulation specifically enhances the emotional recognition of facial anger and sadness. Cognit. Emot. 26, 786–799. doi: 10.1080/02699931.2011.619520
Flace, P., Livrea, P., Basile, G. A., Galletta, D., Bizzoca, A., Gennarini, G., et al. (2021). The cerebellar dopaminergic system. Front. Syst. Neurosci. 15:650614. doi: 10.3389/fnsys.2021.650614
Fliers, E., Rommelse, N., Vermeulen, S. H. H. M., Altink, M., Buschgens, C. J. M., Faraone, S. V., et al. (2008). Motor coordination problems in children and adolescents with ADHD rated by parents and teachers: effects of age and gender. J. Neural Transm. 115, 211–220. doi: 10.1007/s00702-007-0827-0
Frazier, T. W., Demaree, H. A., and Youngstrom, E. A. (2004). Meta-analysis of intellectual and neuropsychological test performance in attention-deficit/hyperactivity disorder. Neuropsychology 18, 543–555. doi: 10.1037/0894-4105.18.3.543
Freedman, R., Hoffer, B. J., Woodward, D. J., and Puro, D. (1977). Interaction of norepinephrine with cerebellar activity evoked by mossy and climbing fibers. Exp. Neurol. 55, 269–288. doi: 10.1016/0014-4886(77)90175-3
Fried, M., Tsitsiashvili, E., Bonneh, Y. S., Sterkin, A., Wygnanski-Jaffe, T., Epstein, T., et al. (2014). ADHD subjects fail to suppress eye blinks and microsaccades while anticipating visual stimuli but recover with medication. Vis. Res. 101, 62–72. doi: 10.1016/j.visres.2014.05.004
Gaiser, C., van der Vliet, R., de Boer, A. A. A., Donchin, O., Berthet, P., Devenyi, G. A., et al. (2024). Population-wide cerebellar growth models of children and adolescents. Nat. Commun. 15:2351. doi: 10.1038/s41467-024-46398-2
Ghanizadeh, A. (2010). Predictors of different types of developmental coordination problems in ADHD: the effect of age, gender, ADHD symptom severity and comorbidities. Neuropediatrics 41, 176–181. doi: 10.1055/s-0030-1267962
Giedd, J. N., Schmitt, J. E., and Neale, M. C. (2007). Structural brain magnetic resonance imaging of pediatric twins. Hum. Brain Mapp. 28, 474–481. doi: 10.1002/hbm.20403
Gill, K. K., Lang, D., and Zwicker, J. G. (2022). Cerebellar and brainstem differences in children with developmental coordination disorder: a voxel-based morphometry study. Front. Hum. Neurosci. 16:921505. doi: 10.3389/fnhum.2022.921505
Goetz, M., Schwabova, J. P., Hlavka, Z., Ptacek, R., and Surman, C. B. H. (2017). Dynamic balance in children with attention-deficit hyperactivity disorder and its relationship with cognitive functions and cerebellum. Neuropsychiatr. Dis. Treat. 13, 873–880. doi: 10.2147/NDT.S125169
Goto, Y., Hatakeyama, K., Kitama, T., Sato, Y., Kanemura, H., Aoyagi, K., et al. (2010). Saccade eye movements as a quantitative measure of frontostriatal network in children with ADHD. Brain Dev. 32, 347–355. doi: 10.1016/j.braindev.2009.04.017
Goulardins, J. B., Marques, J. C. B., Casella, E. B., Nascimento, R. O., and Oliveira, J. A. (2013). Motor profile of children with attention deficit hyperactivity disorder, combined type. Res. Dev. Disabil. 34, 40–45. doi: 10.1016/j.ridd.2012.07.014
Habas, C. (2021). Functional connectivity of the cognitive cerebellum. Front. Syst. Neurosci. 15:642225. doi: 10.3389/fnsys.2021.642225
Horak, F. B., and Macpherson, J. M. (1996). Postural Orientation and Equilibrium. In L. B. Rowell, and J. T. Sheperd (Eds.), Handbook of Physiology, Section 12. Exercise: Regulation and Integration of Multiple Systems (pp. 255–292). New York: Oxford University Press. doi: 10.1002/cphy.cp120107
Hove, M. J., Zeffiro, T. A., Biederman, J., Li, Z., Schmahmann, J., and Valera, E. M. (2015). Postural sway and regional cerebellar volume in adults with attention-deficit/hyperactivity disorder. NeuroImage Clinical 8, 422–428. doi: 10.1016/j.nicl.2015.05.005
Inci Kenar, A. N., Unal, G. A., Kiroglu, Y., and Herken, H. (2017). Effects of methylphenidate treatment on the cerebellum in adult attention-deficit hyperactivity disorder: a magnetic resonance spectroscopy study. Eur. Rev. Med. Pharmacol. Sci. 21, 383–388
Isaac, V., Olmedo, D., Aboitiz, F., and Delano, P. H. (2017). Altered cervical vestibular-evoked myogenic potential in children with attention deficit and hyperactivity disorder. Front. Neurol. 8:90. doi: 10.3389/fneur.2017.00090
Ito, M. (2008). Control of mental activities by internal models in the cerebellum. Nat. Rev. Neurosci. 9, 304–313. doi: 10.1038/nrn2332
Kaiser, M. L., Schoemaker, M. M., Albaret, J. M., and Geuze, R. H. (2015). What is the evidence of impaired motor skills and motor control among children with attention deficit hyperactivity disorder (ADHD)? Systematic review of the literature. Res. Dev. Disabil. 36, 338–357. doi: 10.1016/j.ridd.2014.09.023
Karaokur, R., Cak Esen, H. T., and Uysal, S. A. (2017). An examination of gross and fine motor skills and Their correlates in school age children with attention-deficit/hyperactivity disorder: preliminary results. Psychiat. Clin. Psychopharmacol. 27:11. doi: 10.1080/24750573.2017.1308706
Katzman, M. A., Bilkey, T. S., Chokka, P. R., Fallu, A., and Klassen, L. J. (2017). Adult ADHD and comorbid disorders: clinical implications of a dimensional approach. BMC Psychiatry 17:302. doi: 10.1186/s12888-017-1463-3
Kessler, R. C., Adler, L., Berkley, R., Biederman, J., Conners, C. K., Demler, O., et al. (2006). The prevalence and correlates of adult ADHD in the United States: results from the National Comorbidity Survey Replication. Am. J. Psychiatry 163, 716–723. doi: 10.1176/ajp.2006.163.4.716
Kim, S. M., Hyun, G. J., Jung, T. W., Son, Y. D., Cho, I. H., Kee, B. S., et al. (2017). Balance deficit and brain connectivity in children with attention-deficit/hyperactivity disorder. Psychiatry Investig. 14, 452–457. doi: 10.4306/pi.2017.14.4.452
Kis, B., Guberina, N., Kraemer, M., Niklewski, F., Dziobek, I., Wiltfang, J., et al. (2017). Perception of emotional prosody in adults with attention deficit hyperactivity disorder. Acta Psychiatr. Scand. 135, 506–514. doi: 10.1111/acps.12719
Klupp, S., Möhring, W., Lemola, S., and Grob, A. (2021). Relations between fine motor skills and intelligence in typically developing children and children with attention deficit hyperactivity disorder. Res. Dev. Disabil. 110:103855. doi: 10.1016/j.ridd.2021.103855
Konicarova, J., Bob, P., and Raboch, J. (2014). Balance deficits and ADHD symptoms in medication-naïve school-aged boys. Neuropsychiatr. Dis. Treat. 10, 85–88. doi: 10.2147/NDT.S56017
Koziol, L. F., Budding, D., Andreasen, N., D’Arrigo, S., Bulgheroni, S., Imamizu, H., et al. (2014). Consensus paper: the cerebellum’s role in movement and cognition. Cerebellum 13, 151–177. doi: 10.1007/s12311-013-0511-x
Kühn, S., Romanowski, A., Schilling, C., Banaschewski, T., Barbot, A., Barker, G. J., et al. (2012). Manual dexterity correlating with right lobule VI volume in right-handed 14-year-olds. NeuroImage 59, 1615–1621. doi: 10.1016/j.neuroimage.2011.08.100
Leitner, Y., Barak, R., Giladi, N., Peretz, C., Eshel, R., Gruendlinger, L., et al. (2007). Gait in attention deficit hyperactivity disorder: effects of methylphenidate and dual tasking. J. Neurol. 254, 1330–1338. doi: 10.1007/s00415-006-0522-3
Li, C., Saliba, N. B., Martin, H., Losurdo, N. A., Kolahdouzan, K., Siddiqui, R., et al. (2023). Purkinje cell dopaminergic inputs to astrocytes regulate cerebellar-dependent behavior. Nat. Commun. 14:1613. doi: 10.1038/s41467-023-37319-w
Limperopoulos, C., Soul, J. S., Gauvreau, K., Huppi, P. S., Warfield, S. K., Bassan, H., et al. (2005). Late gestation cerebellar growth is rapid and impeded by premature birth. Pediatrics 115, 688–695. doi: 10.1542/peds.2004-1169
Lupo, M., Siciliano, L., and Leggio, M. (2019). From cerebellar alterations to mood disorders: a systematic review. Neurosci. Biobehav. Rev. 103, 21–28. doi: 10.1016/j.neubiorev.2019.06.008
Manicolo, O., Grob, A., Lemola, S., and Arx, P. H.Von. (2016). Age-related decline of gait variability in children with attention-deficit/hyperactivity disorder: support for the maturational delay hypothesis in gait. Gait Posture, 44. doi: 10.1016/j.gaitpost.2015.12.012, 245–249
Mannarelli, D., Pauletti, C., Currà, A., Marinelli, L., Corrado, A., Delle Chiaie, R., et al. (2019). The cerebellum modulates attention network functioning: evidence from a cerebellar transcranial direct current stimulation and attention network test study. Cerebellum 18, 457–468. doi: 10.1007/s12311-019-01014-8
Mao, H. Y., Kuo, L. C., Yang, A. L., and Su, C. T. (2014). Balance in children with attention deficit hyperactivity disorder-combined type. Res. Dev. Disabil. 35, 1252–1258. doi: 10.1016/j.ridd.2014.03.020
Mariën, P., Wackenier, P., De Surgeloose, D., De Deyn, P. P., and Verhoeven, J. (2010). Developmental coordination disorder: disruption of the cerebello-cerebral network evidenced by SPECT. Cerebellum 9, 405–410. doi: 10.1007/s12311-010-0177-6
Maron, D. N., Bowe, S. J., Spencer-Smith, M., Mellahn, O. J., Perrykkad, K., Bellgrove, M. A., et al. (2021). Oculomotor deficits in attention deficit hyperactivity disorder (ADHD): a systematic review and comprehensive meta-analysis. Neurosci. Biobehav. Rev. 131, 1198–1213. doi: 10.1016/j.neubiorev.2021.10.012
Martel, M. M., Levinson, C. A., Langer, J. K., and Nigg, J. T. (2016). A network analysis of developmental change in ADHD symptom structure from preschool to adulthood. Clin. Psychol. Sci. 4, 988–1001. doi: 10.1177/2167702615618664
Martinussen, R., Hayden, J., Hogg-Johnson, S., and Tannock, R. (2005). A meta-analysis of working memory impairments in children with attention-deficit/hyperactivity disorder. J. Am. Acad. Child Adolesc. Psychiatry 44, 377–384. doi: 10.1097/01.chi.0000153228.72591.73
Mary, A., Slama, H., Mousty, P., Massat, I., Capiau, T., Drabs, V., et al. (2016). Executive and attentional contributions to theory of mind deficit in attention deficit/hyperactivity disorder (ADHD). Child Neuropsychol. 22, 345–365. doi: 10.1080/09297049.2015.1012491
Mauk, M. D., and Buonomano, D. V. (2004). The neural basis of temporal processing. Annu. Rev. Neurosci. 27, 307–340. doi: 10.1146/annurev.neuro.27.070203.144247
Meachon, E. J., Klupp, S., and Grob, A. (2023). Gait in children with and without ADHD: a systematic literature review. Gait Posture 104, 31–42. doi: 10.1016/j.gaitpost.2023.06.003
Melchitzky, D. S., and Lewis, D. A. (2000). Tyrosine hydroxylase-and dopamine transporter-Immunoreactive axons in the primate cerebellum evidence for a lobular-and laminar-specific dopamine innervation. Neuropsychopharmacology 22, 466–472. doi: 10.1016/S0893-133X(99)00139-6
Mohammadzadeh, A., Tehrani-Doost, M., Khorrami, A., and Noorian, N. (2016). Understanding intentionality in children with attention-deficit/hyperactivity disorder. Atten Defic Hyperact Disord 8, 73–78. doi: 10.1007/s12402-015-0187-9
Moises, H. C., Burne, R. A., and Woodward, D. J. (1990). Modification of the visual response properties of cerebellar neurons by norepinephrine. Brain Res. 514, 259–275. doi: 10.1016/0006-8993(90)91421-C
Mokobane, M., Pillay, B. J., and Meyer, A. (2019). Fine motor deficits and attention deficit hyperactivity disorder in primary school children. S. Afr. J. Psychiatry 25:a1232. doi: 10.4102/sajpsychiatry.v25i0.1232
Montes-Montes, R., Delgado-Lobete, L., and Rodríguez-Seoane, S. (2021). Developmental coordination disorder, motor performance, and daily participation in children with attention deficit and hyperactivity disorder. Children 8:187. doi: 10.3390/children8030187
Morellini, L., Ceroni, M., Rossi, S., Zerboni, G., Rege-Colet, L., Biglia, E., et al. (2022). Social cognition in adult ADHD: a systematic review. Front. Psychol. 13:940445. doi: 10.3389/fpsyg.2022.940445
Moruzzi, G. (1940). Paleocerebellar inhibition of vasomotor and respiratory carotid sinus reflexes. J. Neurophysiol. 3, 20–32. doi: 10.1152/jn.1940.3.1.20
Mostofsky, S. H., Reiss, A. L., Lockhart, P., and Bridge Denckla, M. (1998). Evaluation of cerebellar size in attention-deficit hyperactivity disorder. J. Child Neurol. 13, 434–439. doi: 10.1177/088307389801300904
Munoz, D. P., Armstrong, I. T., Hampton, K. A., and Moore, K. D. (2003). Altered control of visual fixation and saccadic eye movements in attention-deficit hyperactivity disorder. J. Neurophysiol. 90, 503–514. doi: 10.1152/jn.00192.2003
Nair, R., and Moss Baylor, S. B. (2009). Neuropsychiatric disease and treatment management of attention-deficit hyperactivity disorder in adults: focus on methylphenidate hydrochloride. Neuropsychiatr. Dis. Treat. 5, 421–432. doi: 10.2147/NDT.S4101
Nguyen-Vu, T. D. B., Kimpo, R. R., Rinaldi, J. M., Kohli, A., Zeng, H., Deisseroth, K., et al. (2013). Cerebellar Purkinje cell activity drives motor learning. Nat. Neurosci. 16, 1734–1736. doi: 10.1038/nn.3576
Nigg, J. T., Karalunas, S. L., Feczko, E., and Fair, D. A. (2020). Toward a revised nosology for attention-deficit/hyperactivity disorder heterogeneity. Biol. Psychiatry Cogn. Neurosci. Neuroimaging 5, 726–737. doi: 10.1016/j.bpsc.2020.02.005
Nisimaru, N. (2004). Cardiovascular modules in the cerebellum. Jpn. J. Physiol. 54, 431–448. doi: 10.2170/jjphysiol.54.431
Noreika, V., Falter, C. M., and Rubia, K. (2013). Timing deficits in attention-deficit/hyperactivity disorder (ADHD): evidence from neurocognitive and neuroimaging studies. Neuropsychologia 51, 235–266. doi: 10.1016/j.neuropsychologia.2012.09.036
Olson, L., and Fuxe, K. (1971). On the projections from the locus coeruleus noradrenaline neurons: the cerebellar innervation. Brain Res. 28, 165–171. doi: 10.1016/0006-8993(71)90533-6
Olson, I. R., Hoffman, L. J., Jobson, K. R., Popal, H. S., and Wang, Y. (2023). Little brain, little minds: the big role of the cerebellum in social development. Dev. Cogn. Neurosci. 60:101238. doi: 10.1016/j.dcn.2023.101238
Palmis, S., Velay, J. L., Habib, M., Anton, J. L., Nazarian, B., Sein, J., et al. (2021). The handwriting brain in middle childhood. Dev. Sci. 24:e13046. doi: 10.1111/desc.13046
Parke, E. M., Becker, M. L., Graves, S. J., Baily, A. R., Paul, M. G., Freeman, A. J., et al. (2021). Social cognition in children with ADHD. J. Atten. Disord. 25, 519–529. doi: 10.1177/1087054718816157
Pereira-Sanchez, V., and Castellanos, F. X. (2021). Neuroimaging in attention-deficit/hyperactivity disorder. Curr. Opin. Psychiatry 34, 105–111. doi: 10.1097/YCO.0000000000000669
Piek, J. P., Dyck, M. J., Nieman, A., Anderson, M., Hay, D., Smith, L. M., et al. (2004). The relationship between motor coordination, executive functioning and attention in school aged children. Arch. Clin. Neuropsychol. 19, 1063–1076. doi: 10.1016/j.acn.2003.12.007
Piek, J. P., Pitcher, T. M., and Hay, D. A. (1999). Motor coordination and kinaesthesis in boys with attention deficit–hyperactivity disorder. Dev. Med. Child Neurol. 41, 159–165. doi: 10.1111/j.1469-8749.1999.tb00575.x
Pitcher, T. M., Piek, J. P., and Hay, D. A. (2003). Fine and gross motor ability in males with ADHD. Dev. Med. Child Neurol. 45, 525–535. doi: 10.1111/j.1469-8749.2003.tb00952.x
Poblano, A., Luna, B., and Reynoso, C. (2014). Differential motor alterations in children with three types of attention deficit hyperactivity disorder. Arq. Neuropsiquiatr. 72, 856–861. doi: 10.1590/0004-282X20140147
Polanczyk, G., Silva de Lima, M., Lessa Horta, B., Biederman, J., and Augusto Rohde, L. (2007). The worldwide prevalence of ADHD: a systematic review and metaregression analysis. Am. J. Psychiatry 164, 942–948. doi: 10.1176/ajp.2007.164.6.942
Popa, L. S., Streng, M. L., Hewitt, A. L., and Ebner, T. J. (2016). The errors of our ways: understanding error representations in cerebellar-dependent motor learning. Cerebellum 15, 93–103. doi: 10.1007/s12311-015-0685-5
Predale, H. K., Chandler, D. J., and Waterhouse, B. D. (2023). “Norepinephrine in the cerebellum” in Essentials of cerebellum and cerebellar disorders: a primer for graduate students. eds. D. L. Gruol, N. Koibuchi, M. Manto, M. Molinari, J. D. Schmahmann, and Y. Shen. 2nd ed (Cham: Springer), 201–208.
Pretegiani, E., Piu, P., Rosini, F., Federighi, P., Serchi, V., Tumminelli, G., et al. (2018). Anti-saccades in cerebellar ataxias reveal a contribution of the cerebellum in executive functions. Front. Neurol. 9:274. doi: 10.3389/fneur.2018.00274
Prsa, M., and Their, P. (2022). Cerebellum: Eye Movements. In: Pfaff, D.W., Volkow, N.D., Rubenstein, J.L. (eds) Neuroscience in the 21st Century: From basic to clinica. 3rd edition. Cham: Springer. doi: 10.1007/978-3-030-88832-9_39
Quenneville, A. F., Kalogeropoulou, E., Nicastro, R., Weibel, S., Chanut, F., and Perroud, N. (2022). Anxiety disorders in adult ADHD: a frequent comorbidity and a risk factor for externalizing problems. Psychiatry Res. 310:114423. doi: 10.1016/j.psychres.2022.114423
Ramnani, N. (2012). Frontal lobe and posterior parietal contributions to the cortico-cerebellar system. Cerebellum 11, 366–383. doi: 10.1007/s12311-011-0272-3
Rau, A., Hosp, J. A., Rijntjes, M., Weiller, C., Kellner, E., Berberovic, E., et al. (2024). Cerebellar, not nigrostriatal degeneration impairs dexterity in multiple system atrophy. Mov. Disord. 39, 130–140. doi: 10.1002/mds.29661
Rudolph, S., Badura, A., Lutzu, S., Pathak, S. S., Thieme, A., Verpeut, J. L., et al. (2023). Cognitive-affective functions of the cerebellum. J. Neurosci. 43, 7554–7564. doi: 10.1523/JNEUROSCI.1451-23.2023
Salman, M. S., and Tsai, P. (2016). The role of the pediatric cerebellum in motor functions, cognition, and behavior: a clinical perspective. Neuroimaging Clin. N. Am. 26, 317–329. doi: 10.1016/j.nic.2016.03.003
Sanders, A. F. (1983). Towards a model of stress and human performance. Acta Psychol. 53, 61–97. doi: 10.1016/0001-6918(83)90016-1
Sayal, K., Prasad, V., Daley, D., Ford, T., and Coghill, D. (2018). ADHD in children and young people: prevalence, care pathways, and service provision. Lancet Psychiatry 5, 175–186. doi: 10.1016/S2215-0366(17)30167-0
Scharoun, S. M., Bryden, P. J., Otipkova, Z., Musalek, M., and Lejcarova, A. (2013). Motor skills in Czech children with attention-deficit/hyperactivity disorder and their neurotypical counterparts. Res. Dev. Disabil. 34, 4142–4153. doi: 10.1016/j.ridd.2013.08.011
Schmahmann, J. D. (2019). The cerebellum and cognition. Neurosci. Lett. 688, 62–75. doi: 10.1016/j.neulet.2018.07.005
Schmahmann, J. D., and Sherman, J. C. (1998). The cerebellar cognitive affective syndrome. Brain 121, 561–579. doi: 10.1093/brain/121.4.561
Sergeant, J. (2000). The cognitive-energetic model: an empirical approach to attention-deficit hyperactivity disorder. Neurosci. Biobehav. Rev. 24, 7–12. doi: 10.1016/S0149-7634(99)00060-3
Shaw, P., Eckstrand, K., Sharp, W., Blumenthal, J., Lerch, J. P., Greenstein, D., et al. (2007). Attention-deficit/hyperactivity disorder is characterized by a delay in cortical maturation. Proc. Natl. Acad. Sci. USA 104, 19649–19654. doi: 10.1073/pnas.0707741104
Shaw, P., Malek, M., Watson, B., Sharp, W., Evans, A., and Greenstein, D. (2012). Development of cortical surface area and gyrification in attention-deficit/hyperactivity disorder. Biol. Psychiatry 72, 191–197. doi: 10.1016/j.biopsych.2012.01.031
Shen, I. H., Lee, T. Y., and Chen, C. L. (2012). Handwriting performance and underlying factors in children with attention deficit hyperactivity disorder. Res. Dev. Disabil. 33, 1301–1309. doi: 10.1016/j.ridd.2012.02.010
Sherigar, S. S., Gamsa, A. H., and Srinivasan, K. (2023). Oculomotor deficits in attention deficit hyperactivity disorder: a systematic review and meta-analysis. Eye 37, 1975–1981. doi: 10.1038/s41433-022-02284-z
Shiels Rosch, K., Dirlikov, B., and Mostofsky, S. H. (2013). Increased intrasubject variability in boys with ADHD across tests of motor and cognitive control. J. Abnorm. Child Psychol. 41, 485–495. doi: 10.1007/s10802-012-9690-z
Shum, S. B. M., and Pang, M. Y. C. (2009). Children with attention deficit hyperactivity disorder have impaired balance function: involvement of somatosensory, visual, and vestibular systems. J. Pediatr. 155, 245–249. doi: 10.1016/j.jpeds.2009.02.032
Simmons, R. W., Taggart, T. C., Thomas, J. D., Mattson, S. N., and Riley, E. P. (2020). Gait control in children with attention-deficit/hyperactivity disorder. Hum. Mov. Sci. 70:102584. doi: 10.1016/j.humov.2020.102584
Slaats-Willemse, D., De Sonneville, L., Swaab-Barneveld, H., and Buitelaar, J. (2005). Motor flexibility problems as a marker for genetic susceptibility to attention-deficit/hyperactivity disorder. Biol. Psychiatry 58, 233–238. doi: 10.1016/j.biopsych.2005.03.046
Slater, J. L., and Tate, M. C. (2018). Timing deficits in ADHD: insights from the neuroscience of musical rhythm. Front. Comput. Neurosci. 12:51. doi: 10.3389/fncom.2018.00051
Snider, R. S. (1950). Recent contributions to the anatomy and physiology of the cerebellum. Arch. Neurol. Psychiatr. 64, 196–219. doi: 10.1001/archneurpsyc.1950.02310260034002
Sobanski, E. (2006). Psychiatric comorbidity in adults with attention-deficit/hyperactivity disorder (ADHD). Eur. Arch. Psychiatry Clin. Neurosci. 256, i26–i31. doi: 10.1007/s00406-006-1004-4
Sokolov, A. A. (2018). The cerebellum in social cognition. Front. Cell. Neurosci. 12:145. doi: 10.3389/fncel.2018.00145
Soteropoulos, D. S., and Baker, S. N. (2008). Bilateral representation in the deep cerebellar nuclei. J. Physiol. 586, 1117–1136. doi: 10.1113/jphysiol.2007.144220
Stein, H. (2021). Why does the neocortex need the cerebellum for working memory? J. Neurosci. 41, 6368–6370. doi: 10.1523/JNEUROSCI.0701-21.2021
Stoodley, C. J. (2016). The cerebellum and neurodevelopmental disorders. Cerebellum 15, 34–37. doi: 10.1007/s12311-015-0715-3
Stoodley, C. J., and Schmahmann, J. D. (2010). Evidence for topographic organization in the cerebellum of motor control versus cognitive and affective processing. Cortex 46, 831–844. doi: 10.1016/j.cortex.2009.11.008
Tanaka, M., Kunimatsu, J., Suzuki, T. W., Kameda, M., Ohmae, S., Uematsu, A., et al. (2021). Roles of the cerebellum in motor preparation and prediction of timing. Neuroscience 462, 220–234. doi: 10.1016/j.neuroscience.2020.04.039
Thomas, R., Sanders, S., Doust, J., Beller, E., and Glasziou, P. (2015). Prevalence of attention-deficit/hyperactivity disorder: a systematic review and meta-analysis. Pediatrics 135, e994–e1001. doi: 10.1542/peds.2014-3482
Tomasi, D., and Volkow, N. D. (2012). Abnormal functional connectivity in children with attention-deficit/hyperactivity disorder. Biol. Psychiatry 71, 443–450. doi: 10.1016/j.biopsych.2011.11.003
Toplak, M. E., Dockstader, C., and Tannock, R. (2006). Temporal information processing in ADHD: findings to date and new methods. J. Neurosci. Methods 151, 15–29. doi: 10.1016/j.jneumeth.2005.09.018
Tseng, M. H., Henderson, A., Chow, S. M. K., and Yao, G. (2004). Relationship between motor proficiency, attention, impulse, and activity in children with ADHD. Dev. Med. Child Neurol. 46, 381–388. doi: 10.1017/S0012162204000623
Tucha, O., and Lange, K. W. (2001). Effects of methylphenidate on kinematic aspects of handwriting in hyperactive boys. J. Abnorm. Child Psychol. 29, 351–356. doi: 10.1023/A:1010366014095
Turner, B. M., Paradiso, S., Marvel, C. L., Pierson, R., Boles Ponto, L. L., Hichwa, R. D., et al. (2007). The cerebellum and emotional experience. Neuropsychologia 45, 1331–1341. doi: 10.1016/j.neuropsychologia.2006.09.023
Uekermann, J., Kraemer, M., Abdel-Hamid, M., Schimmelmann, B. G., Hebebrand, J., Daum, I., et al. (2010). Social cognition in attention-deficit hyperactivity disorder (ADHD). Neurosci. Biobehav. Rev. 34, 734–743. doi: 10.1016/j.neubiorev.2009.10.009
Valera, E. M., Faraone, S. V., Murray, K. E., and Seidman, L. J. (2007). Meta-analysis of structural imaging findings in attention-deficit/hyperactivity disorder. Biol. Psychiatry 61, 1361–1369. doi: 10.1016/j.biopsych.2006.06.011
Van Overwalle, F., Baetens, K., Mariën, P., and Vandekerckhove, M. (2014). Social cognition and the cerebellum: a meta-analysis of over 350 fMRI studies. NeuroImage 86, 554–572. doi: 10.1016/j.neuroimage.2013.09.033
Van Overwalle, F., D’aes, T., and Mariën, P. (2015). Social cognition and the cerebellum: a meta-analytic connectivity analysis. Hum. Brain Mapp. 36, 5137–5154. doi: 10.1002/hbm.23002
Van, J. H., and Thomas, J. R. (2002). Arm movement control: differences between children with and without attention deficit hyperactivity disorder. Res. Q. Exerc. Sport 73, 10–18. doi: 10.1080/02701367.2002.10608987
Wang, S. S. H., Kloth, A. D., and Badura, A. (2014). The cerebellum, sensitive periods, and autism. Neuron 83, 518–532. doi: 10.1016/j.neuron.2014.07.016
Wang, P., Wang, J., Jiang, Y., Wang, Z., Meng, C., Castellanos, F. X., et al. (2022). Cerebro-cerebellar dysconnectivity in children and adolescents with attention-deficit/hyperactivity disorder. J. Am. Acad. Child Adolesc. Psychiatry 61, 1372–1384. doi: 10.1016/j.jaac.2022.03.035
Waterhouse, B. D., and Navarra, R. L. (2019). The locus coeruleus-norepinephrine system and sensory signal processing: a historical review and current perspectives. Brain Res. 1709, 1–15. doi: 10.1016/j.brainres.2018.08.032
Welniarz, Q., Worbe, Y., and Gallea, C. (2021). The forward model: a unifying theory for the role of the cerebellum in motor control and sense of agency. Front. Syst. Neurosci. 15:644059. doi: 10.3389/fnsys.2021.644059
Willcutt, E. G., Doyle, A. E., Nigg, J. T., Faraone, S. V., and Pennington, B. F. (2005). Validity of the executive function theory of attention-deficit/hyperactivity disorder: a meta-analytic review. Biol. Psychiatry 57, 1336–1346. doi: 10.1016/j.biopsych.2005.02.006
Zelaznik, H. N., Vaughn, A. J., Green, J. T., Smith, A. L., Hoza, B., and Linnea, K. (2012). Motor timing deficits in children with attention-deficit/hyperactivity disorder. Hum. Mov. Sci. 31, 255–265. doi: 10.1016/j.humov.2011.05.003
Zhang, J., Kang, L., Li, J., Li, Y., Bi, H., and Yang, Y. (2022). Brain correlates of Chinese handwriting and Their relation to Reading development in children: an fMRI study. Brain Sci. 12:1724. doi: 10.3390/brainsci12121724
Keywords: attention-deficit/hyperactivity disorder, development, motor impairment, cerebellar dysfunction, cortico-cerebellar network
Citation: Isaac V, Lopez V and Escobar MJ (2025) Can attention-deficit/hyperactivity disorder be considered a form of cerebellar dysfunction? Front. Neurosci. 19:1453025. doi: 10.3389/fnins.2025.1453025
Received: 24 June 2024; Accepted: 02 January 2025;
Published: 22 January 2025.
Edited by:
Masaya Tachibana, Osaka University, JapanCopyright © 2025 Isaac, Lopez and Escobar. This is an open-access article distributed under the terms of the Creative Commons Attribution License (CC BY). The use, distribution or reproduction in other forums is permitted, provided the original author(s) and the copyright owner(s) are credited and that the original publication in this journal is cited, in accordance with accepted academic practice. No use, distribution or reproduction is permitted which does not comply with these terms.
*Correspondence: Valeria Isaac, bXZpc2FhY0B1Zy51Y2hpbGUuY2w=
Disclaimer: All claims expressed in this article are solely those of the authors and do not necessarily represent those of their affiliated organizations, or those of the publisher, the editors and the reviewers. Any product that may be evaluated in this article or claim that may be made by its manufacturer is not guaranteed or endorsed by the publisher.
Research integrity at Frontiers
Learn more about the work of our research integrity team to safeguard the quality of each article we publish.