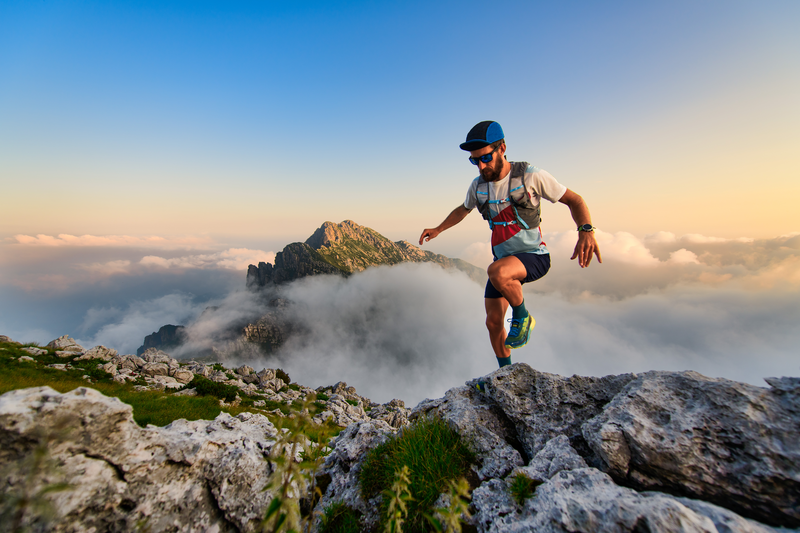
94% of researchers rate our articles as excellent or good
Learn more about the work of our research integrity team to safeguard the quality of each article we publish.
Find out more
MINI REVIEW article
Front. Neurosci. , 31 October 2024
Sec. Sleep and Circadian Rhythms
Volume 18 - 2024 | https://doi.org/10.3389/fnins.2024.1478820
This article is part of the Research Topic Sex Differences in Sleep and Circadian Rhythms View all 5 articles
There are well-documented gender differences in the risk and severity of sleep disorders and associated comorbidities. While fundamental sex differences in sleep regulatory mechanisms may contribute to gender disparities, biological responses to sleep loss and stress may underlie many of the risks for sleep disorders in women and men. Some of these sex differences appear to be dependent on sex chromosome complement (XX or XY) and the organizational effects of reproductive hormones. Reproductive development plays a critical role in the ability of sex chromosomes and reproductive hormones to produce sex differences in sleep and wakefulness. Rodent models reveal that reproductive hormones drive many but not all sex differences in sleep–wake architecture. The ability of reproductive hormones to alter sleep are often dependent on responses to sleep loss and stress. However, in the absence of reproductive hormones (in gonadectomized rodents) sex differences in sleep amount and the ability to recover from sleep loss persist. The suprachiasmatic nucleus (SCN) and the ventrolateral preoptic nucleus (VLPO) of the hypothalamus play crucial regulatory roles in mediating the effects of reproductive hormones on the sleep–wake cycle. Taken together, the work reviewed here reveals that the reproductive hormone environment and sex chromosome complement may underlie gender disparities in sleep patterns and the risk for sleep disorders.
Getting sufficient amounts of daily sleep is critical for the health and well-being of women and men. However, sleep cycles across our society exhibit a variety of gender differences which include daily sleep amount (Saelee et al., 2023), daytime sleepiness (Gonsalvez et al., 2022), and responses to sleep medications (Roehrs and Roth, 2016). In clinical and epidemiological studies, women report more difficulties sleeping, restlessness upon awakening, and sleepiness across the daytime compared to men (Armitage et al., 2000; Jung et al., 2013; Saelee et al., 2023). Moreover, there are well-documented gender differences in the risk and severity of sleep disorders such as insomnia (Zhang and Wing, 2006), hypersomnia (Deshaies-Rugama et al., 2024), and delayed sleep phase disorder (Reis and Paiva, 2019). The origins of these gender differences are complex but often arise from difficulties recovering from sleep loss and stress. While gender disparities in sleep impairments are moderate under normal environmental conditions, they are often amplified under challenging conditions such as daily insufficient sleep and chronic stress. Importantly, gender differences in sleep impairments contribute to gender disparities in the prevalence of comorbidities such as depression and anxiety disorders (Joshi et al., 2023). These gender differences likely contribute to health disparities in a variety of sleep disorders and comorbid disorders, which is why revealing potential mechanisms that may underlie them is so important.
Although influenced by environmental and psychosocial factors, gender disparities in sleep are largely driven by biological sex differences in sleep regulatory mechanisms. For the remainder of this review, we will refer to sex differences instead of gender differences since we will report findings from animal models. Reproductive processes underlie several sex differences in the sleep–wake cycle. They have regulatory influences on several indices of sleep quality, such as sleep duration and frequency of daytime naps (Alzueta and Baker, 2023; Maki et al., 2024). Sex differences in sleep phenotypes are prevalent across the animal kingdom. To this end, animal models of biological sex are useful tools for identifying the roles of sex characteristics and reproductive processes on the sleep–wake cycle.
Sleep is regulated by circadian and homeostatic mechanisms, as described by the two-process model (Borbely, 1982). Process C is the circadian process in which an endogenous clock times the sleep–wake cycle and consolidates sleep to a particular time of day. In diurnal species like humans, most sleep occurs at night, while in nocturnal species like mice, most sleep occurs during the day. Process S, sleep homeostasis, is based on prior sleep and wake amount. Sleep loss is followed by a sleep rebound characterized by increased NREM and REM sleep amounts and increased NREM slow wave activity (SWA). Sex differences in the circadian regulation of sleep and sleep homeostasis have been documented in primates (Laska and Tutsch, 2000; Barger et al., 2010), rodents (Swift et al., 2020; Chiem et al., 2024), and invertebrates (Hendricks et al., 2003; Wu et al., 2018). In mice, females have more consolidated but less total sleep throughout the day (Paul et al., 2006; Wu et al., 2018). Male mice are able to recover from sleep loss more quickly than females (Paul et al., 2006). Homeostatic responses to sleep loss also exhibit sex differences in gene expression. Transcripts for brain-derived neurotropic factor (BDNF), FosB, and the adenylate cyclase Adcy7 exhibit robust sex differences in brain expression after sleep deprivation (Shi et al., 2024). These findings show that animal models have promise to help reveal the mechanisms responsible for sex differences in the sleep–wake cycle.
Mouse models of human neurological disorders also exhibit sex differences in the sleep–wake cycle. For instance, in a mouse model of Huntington’s disease, BACHD, males recapitulate sleep phenotypes that are common in Huntington’s patients, but females do not (Chiem et al., 2024). A mouse model of Alzheimer’s disease reveals that female 5xFAD mice exhibit more cognitive benefits than males when exposed to feeding times that improve sleep efficiency (Campbell et al., 2024). Also, the Shank3 mouse model of autism spectrum disorder reveals a remarkable sex difference in which early-life sleep disruption improves general sociability in males while reducing risk aversion in females (Lord et al., 2022). Studies like these exemplify the value of mouse models of human disorders to understand the importance of sex differences in sleep in the risk, severity, and treatment of neurological disorders.
Sex chromosome complement (XX or XY) and the Y-linked Sry (sex-determining region Y) gene have regulatory influences on sleep (Ehlen et al., 2013). Although some sex differences in spontaneous sleep amount are dependent on reproductive hormones, other sex differences in sleep amount during the active phase are preserved after gonadectomy (GDX) and may be driven by non-hormonal factors. The four-core genotype (FCG) mouse model offers a robust framework for investigating the persistent sex differences following GDX. This model was generated by removing the Sry gene from the Y chromosome and reinserting it onto an autosome, resulting in progeny with discordant phenotypes and genotypes—of specific interest are XX mice with testes (XXM), and XY mice with ovaries (XYF). Mice from the FCG mouse model exhibit no sex differences in rest-phase sleep amount; however, during the active-phase (nighttime), XX males have more spontaneous NREM sleep than XX females. The XY male and female mice do not exhibit sex differences in sleep amount. During rebound from forced wakefulness, there is a change in the factors regulating sleep. XY females sleep more during their mid-active phase than XX females and have higher NREM SWA (Ehlen et al., 2013). These findings suggest that processes that regulate sleep homeostasis are sex-linked, and that sleep amount and sleep propensity are regulated differently in males and females during rebound from sleep loss.
It is unclear if the effects of Sry or sex chromosomes are due to the constitutive expression of sex-linked genes or the organizational influences of reproductive hormones during neonatal or pubertal development. Reproductive hormones have two primary effects on brain structures and secondary sex characteristics: organizational and activational (Arnold and Gorski, 1984). In most mammals, the reproductive hormone environment around the time of birth plays a major role in “organizing” neural circuitry (Han and De Vries, 2003; Morris et al., 2004). The activation of sex-related behaviors by the reproductive hormone environment in adulthood depends on the organizational effects of reproductive hormones during the perinatal environment and pubertal development. The synthesis and secretion of androgens and estrogens in mammals has a variable longitudinal profile during neonatal and juvenile development. Though the profile of reproductive hormone secretion exhibits several prolonged periods of stability, abrupt and dramatic adjustments in the magnitude and timing of their secretions are hallmarks of ontogeny.
In the FCG mouse line, mice that express the Sry gene exhibit more NREM sleep and total sleep during the active phase than mice that do not express Sry. Sry drives active phase “siesta” sleep amount on both XX and XY chromosomal backgrounds (Ehlen et al., 2013). However, all mice that express Sry develop into phenotypic males, regardless of the sex chromosome complement. This is the result of the organizational effect of androgens during perinatal development and the activational effects of androgens during pubertal development and adulthood. Early work has shown that the organizational effects of reproductive hormones in neonatal male and female rats are responsible for some of the activational effects of reproductive hormones on sleep in adulthood (Branchey et al., 1971). A seminal animal study reported that neonatal castration alters sleep responses to estradiol and progesterone injections in adult male rats (Branchey et al., 1973). In this study, perinatally feminized (castrated) male mice exhibited decreased REM and NREM sleep amounts in response to exogenous female reproductive hormones. These data demonstrate that reproductive hormones have organizational influences on sleep–wake architecture.
In adult GDX FCG mice, sex differences in spontaneous sleep phenotypes are coincident with the presence or absence of Sry. This association with Sry may be attributable to the activity of reproductive hormones prior to GDX. Following sleep deprivation, sex differences in recovery sleep in GDX wild-type mice are well established (Paul et al., 2006; Paul et al., 2009a) and differences between XX female and XY male mice recapitulate this male–female difference. In both spontaneous and recovery sleep, there is a positive effect of Sry on NREM, REM and total sleep in XX mice. However, in XY mice, the positive effect of Sry appears to be specific to spontaneous sleep but not recovery from sleep loss. These findings reveal that the association of Sry with sleep state is dependent on the underlying sex chromosome complement. More importantly, the specific effect of sex chromosomes appears to be restricted to homeostatic regulation of NREM sleep. The positive effect of Sry on REM sleep is conserved between baseline and sleep deprivation conditions; however, sleep deprivation reveals an interaction of Sry with sex chromosomes during REM sleep, with Sry having a positive effect on REM in XX mice, and no effect within XY mice (Ehlen et al., 2013). Thus, increasing homeostatic sleep pressure changes the relationship between sex chromosome complement and the Sry gene. These findings provide evidence that sex chromosomes, and sex-linkage, may play regulatory roles on the ability to recover from sleep loss.
Reproductive hormones have constitutive effects on sleep–wake architecture, sleep fragmentation, and sleep homeostasis (the ability to recover from sleep loss). In rats, the hormonal estrous cycle produces fluctuations in sleep–wake amount that include decreases in sleep amount during proestrus and a concomitant increase in wakefulness (Swift et al., 2020). NREM slow wave activity (SWA), a measurement of sleep pressure was also enhanced during proestrus. Sex differences in total sleep amount and sleep–wake fragmentation during spontaneous (sleep-replete) states are largely driven by 17β-estradiol and testosterone (Paul et al., 2006; Paul et al., 2009b; Schwartz and Mong, 2013; Cusmano et al., 2014; Choi et al., 2021). GDX in male and female mice and rats eliminates many sex differences in sleep–wake architecture (Paul et al., 2006; Cusmano et al., 2014). However, several sex differences in the homeostatic mechanism that regulates the ability to recover from sleep loss persist following GDX.
Sex differences in the ability of stress to increase REM sleep amount are also largely driven by reproductive hormones (Jefferson et al., 2014). These findings suggest that sex differences in responses to sleep loss differ from sex differences in responses to stress modalities that activate physiological stress response mechanisms. Stated differently, homeostatic sleep responses to stress are modulated by the reproductive hormone environment, whereas the effects of sleep loss remain largely unaffected by these hormonal influences. Sex differences in sleep responses to stress are partially encoded by nitric oxide synthase pathways in the basal forebrain (Kalinchuk et al., 2010; Chiem et al., 2021). The combined results from these studies demonstrate (1) the regulatory roles of sex chromosomes and reproductive hormones on sleep regulatory mechanisms, (2) that sex differences in sleep responses to stress are driven by different mechanisms than those that drive sleep homeostasis, and (3) that sex-linked factors constitutively drive sex differences in homeostatic sleep responses.
In rats, reproductive hormones organize neonatal sleep regulatory pathways in a way that primes adult sleep responsivity to the reproductive hormone environment (Cusmano et al., 2014). This study revealed that neonatal exposure to androgens reorganizes the sleep regulatory pathways of female rats. This work also revealed that most of the influences of androgens on sleep are driven by the aromatization of testosterone into estradiol. Work in this area could be pivotal in understanding the etiology of sex differences in the prevalence of sleep disorders and could provide important clues about the potential for sleep impairments to be co-morbid in other diseases and disorders that exhibit gender disparities.
Several systems that regulate circadian rhythms and sleep homeostasis have sensitivity to the reproductive hormone environment. The suprachiasmatic nucleus (SCN), located in the anterior hypothalamus, is a primary circadian regulatory region of the brain. The SCN is responsible for the daily timing and coordination of sleep and wake onsets and the diurnal consolidation of sleep and wake states across the 24-h day (Ono et al., 2024). The SCN exhibits several sexual dimorphisms, many of which are sensitive to the reproductive environment. For instance, female mice have a larger number of estrogen receptor (ER)-expressing neurons in the SCN than male mice (Vida et al., 2008; Zuloaga et al., 2014), but male mice have a larger number of androgen receptor (AR)-expressing neurons in the SCN than females (Iwahana et al., 2008). These dimorphisms largely drive sex differences in circadian locomotor activity (Vida et al., 2008). The VLPO is an important component of the system responsible for sleep homeostasis (Alam et al., 2014). VLPO neurons are responsible for inhibiting arousal-promoting centers of the brainstem and hypothalamus (Saper et al., 2005). The VLPO has been revealed to be a critical region for the effects of reproductive hormones on sleep. Neurons in the VLPO exhibit sexual dimorphisms that are sensitive to the reproductive hormone environment (Peterfi et al., 2004). The wake-promoting effects of estradiol in mice are driven by inhibition of sleep-active neurons in the VLPO (Deurveilher et al., 2008). The ability of estradiol to suppress REM sleep is related to the inhibitory effects of estrogen receptor activation in the VLPO (Hadjimarkou et al., 2008). In these studies, animal models have revealed potential brain-mediated mechanisms through which reproductive processes contribute to sex differences in the sleep–wake cycle. Still, more work needs to be done to determine the degree to which they underlie health disparities in sleep disorders.
While the origins of gender disparities in the risk and severity of sleep disorders are elusive, sleep research in model systems reveals that sex chromosome complement and the reproductive hormone environment play critical roles in the organization of the sleep–wake cycle. Gonadectomies in male and female mice reduce many sex differences in sleep architecture, yet many sex differences in the ability to recover from sleep loss remain. Moreover, the ability of reproductive hormones to influence sleep is largely determined by early exposure. The studies described in this review reveal that while the hormone environment causes some sex differences in sleep, others are ingrained at a more fundamental, perhaps genetic, level. By gaining a greater understanding of the systems that underlie these sex differences, we are likely to improve our ability to treat sleep disorders and associated co-morbidities.
Understanding the mechanism of interaction between sex chromosome complement and reproductive hormones is crucial, not only for advancing knowledge in this field but also for the potential impact on public health. Due to a predominant focus on male subjects in animal research, this area has been historically underexplored. The neglect of sex as a biological variable in past research has led to a significant knowledge gap regarding such mechanisms in females, and thus the contribution to observed differences in sleep regulation and related disorders is unclear. The notion that certain sex differences in sleep may be “hard-wired” underscores the importance of early developmental processes, including the differentiation of sex chromosomes and the organizational effects of reproductive hormones. Future research should aim to dissect the relative contributions of sex chromosomes versus reproductive hormones in shaping these differences. Ongoing studies investigating rodent models throughout development, along with explorations of stress and sleep loss under varying hormonal environments, could reveal new pathways that are susceptible to intervention. These inquiries will likely yield critical insights into the etiology of sex-specific sleep disorders and allow us to move toward more inclusive and effective health strategies.
KP: Conceptualization, Investigation, Writing – original draft, Writing – review & editing. MR: Conceptualization, Investigation, Writing – original draft, Writing – review & editing. JE: Conceptualization, Investigation, Writing – original draft, Writing – review & editing.
The author(s) declare that no financial support was received for the research, authorship, and/or publication of this article.
The authors declare that the research was conducted in the absence of any commercial or financial relationships that could be construed as a potential conflict of interest.
All claims expressed in this article are solely those of the authors and do not necessarily represent those of their affiliated organizations, or those of the publisher, the editors and the reviewers. Any product that may be evaluated in this article, or claim that may be made by its manufacturer, is not guaranteed or endorsed by the publisher.
Alam, M. A., Kumar, S., McGinty, D., Alam, M. N., and Szymusiak, R. (2014). Neuronal activity in the preoptic hypothalamus during sleep deprivation and recovery sleep. J. Neurophysiol. 111, 287–299. doi: 10.1152/jn.00504.2013
Alzueta, E., and Baker, F. C. (2023). The menstrual cycle and sleep. Sleep Med. Clin. 18, 399–413. doi: 10.1016/j.jsmc.2023.06.003
Armitage, R., Hoffmann, R., Trivedi, M., and Rush, A. J. (2000). Slow-wave activity in NREM sleep: sex and age effects in depressed outpatients and healthy controls. Psychiatry Res. 95, 201–213. doi: 10.1016/S0165-1781(00)00178-5
Arnold, A. P., and Gorski, R. A. (1984). Gonadal steroid induction of structural sex differences in the central nervous system. Annu. Rev. Neurosci. 7, 413–442. doi: 10.1146/annurev.ne.07.030184.002213
Barger, L. K., Hoban-Higgins, T. M., and Fuller, C. A. (2010). Gender differences in the circadian rhythms of rhesus monkeys. Physiol. Behav. 101, 595–600. doi: 10.1016/j.physbeh.2010.06.002
Branchey, M., Branchey, L., and Nadler, R. D. (1971). Effects of estrogen and progesterone on sleep patterns of female rats. Physiol. Behav. 6, 743–746. doi: 10.1016/0031-9384(71)90267-8
Branchey, L., Branchey, M., and Nadler, R. D. (1973). Effects of sex hormones on sleep patterns of male rats gonadectomized in adulthood and in the neonatal period. Physiol. Behav. 11, 609–611. doi: 10.1016/0031-9384(73)90244-8
Campbell, K. J., Jiang, P., Olker, C., Lin, X., Kim, S. Y., Lee, C. J., et al. (2024). The impacts of sex and the 5xFAD model of Alzheimer's disease on the sleep and spatial learning responses to feeding time. Front. Neurol. 15:1430989. doi: 10.3389/fneur.2024.1430989
Chiem, E., Nichols, I., Van, C., Kori, S., and Paul, K. (2021). Sleep loss mediates the effect of stress on nitrergic signaling in female mice. Neurosci. Lett. 740:135362. doi: 10.1016/j.neulet.2020.135362
Chiem, E., Zhao, K., Stark, G., Ghiani, C. A., Colwell, C. S., and Paul, K. N. (2024). Sex differences in sleep architecture in a mouse model of Huntington's disease. J. Neurosci. Res. 102:e25290. doi: 10.1002/jnr.25290
Choi, J., Kim, S. J., Fujiyama, T., Miyoshi, C., Park, M., Suzuki-Abe, H., et al. (2021). The role of reproductive hormones in sex differences in sleep homeostasis and arousal response in mice. Front. Neurosci. 15:739236. doi: 10.3389/fnins.2021.739236
Cusmano, D. M., Hadjimarkou, M. M., and Mong, J. A. (2014). Gonadal steroid modulation of sleep and wakefulness in male and female rats is sexually differentiated and neonatally organized by steroid exposure. Endocrinology 155, 204–214. doi: 10.1210/en.2013-1624
Deshaies-Rugama, A. S., Mombelli, S., Blais, H., Sekerovic, Z., Massicotte, M., Thompson, C., et al. (2024). Sleep architecture in idiopathic hypersomnia: the influence of age, sex, and body mass index. Sci. Rep. 14:16407. doi: 10.1038/s41598-024-67203-6
Deurveilher, S., Cumyn, E. M., Peers, T., Rusak, B., and Semba, K. (2008). Estradiol replacement enhances sleep deprivation-induced c-Fos immunoreactivity in forebrain arousal regions of ovariectomized rats. Am. J. Physiol. Regul. Integr. Comp. Physiol. 295, R1328–R1340. doi: 10.1152/ajpregu.90576.2008
Ehlen, J. C., Hesse, S., Pinckney, L., and Paul, K. N. (2013). Sex chromosomes regulate nighttime sleep propensity during recovery from sleep loss in mice. PLoS One 8:e62205. doi: 10.1371/journal.pone.0062205
Gonsalvez, I., Li, J. J., Stevens, C., Chen, J. A., and Liu, C. H. (2022). Preexisting depression and daytime sleepiness in women and men. Behav. Sleep Med. 20, 380–392. doi: 10.1080/15402002.2021.1924720
Hadjimarkou, M. M., Benham, R., Schwarz, J. M., Holder, M. K., and Mong, J. A. (2008). Estradiol suppresses rapid eye movement sleep and activation of sleep-active neurons in the ventrolateral preoptic area. Eur. J. Neurosci. 27, 1780–1792. doi: 10.1111/j.1460-9568.2008.06142.x
Han, T. M., and De Vries, G. J. (2003). Organizational effects of testosterone, estradiol, and dihydrotestosterone on vasopressin mRNA expression in the bed nucleus of the stria terminalis. J. Neurobiol. 54, 502–510. doi: 10.1002/neu.10157
Hendricks, J. C., Lu, S., Kume, K., Yin, J. C., Yang, Z., and Sehgal, A. (2003). Gender dimorphism in the role of cycle (BMAL1) in rest, rest regulation, and longevity in Drosophila melanogaster. J. Biol. Rhythm. 18, 12–25. doi: 10.1177/0748730402239673
Iwahana, E., Karatsoreos, I., Shibata, S., and Silver, R. (2008). Gonadectomy reveals sex differences in circadian rhythms and suprachiasmatic nucleus androgen receptors in mice. Horm. Behav. 53, 422–430. doi: 10.1016/j.yhbeh.2007.11.014
Jefferson, F., Ehlen, J. C., Williams, N. S., Montemarano, J. J., and Paul, K. N. (2014). A dopamine receptor D2-type agonist attenuates the ability of stress to Alter sleep in mice. Endocrinology 155, 4411–4421. doi: 10.1210/en.2014-1134
Joshi, K., Cambron-Mellott, M. J., Costantino, H., Pfau, A., and Jha, M. K. (2023). The real-world burden of adults with major depressive disorder with moderate or severe insomnia symptoms in the United States. J. Affect. Disord. 323, 698–706. doi: 10.1016/j.jad.2022.12.005
Jung, K. I., Song, C. H., Ancoli-Israel, S., and Barrett-Connor, E. (2013). Gender differences in nighttime sleep and daytime napping as predictors of mortality in older adults: the rancho Bernardo study. Sleep Med. 14, 12–19. doi: 10.1016/j.sleep.2012.06.004
Kalinchuk, A. V., McCarley, R. W., Porkka-Heiskanen, T., and Basheer, R. (2010). Sleep deprivation triggers inducible nitric oxide-dependent nitric oxide production in wake-active basal forebrain neurons. J. Neurosci. 30, 13254–13264. doi: 10.1523/JNEUROSCI.0014-10.2010
Laska, M., and Tutsch, M. (2000). Laterality of tail resting posture in three species of New World primates. Neuropsychologia 38, 1040–1046. doi: 10.1016/S0028-3932(99)00147-5
Lord, J. S., Gay, S. M., Harper, K. M., Nikolova, V. D., Smith, K. M., Moy, S. S., et al. (2022). Early life sleep disruption potentiates lasting sex-specific changes in behavior in genetically vulnerable Shank3 heterozygous autism model mice. Mol. Autism. 13:35. doi: 10.1186/s13229-022-00514-5
Maki, P. M., Panay, N., and Simon, J. A. (2024). Sleep disturbance associated with the menopause. Menopause 31, 724–733. doi: 10.1097/GME.0000000000002386
Morris, J. A., Jordan, C. L., and Breedlove, S. M. (2004). Sexual differentiation of the vertebrate nervous system. Nat. Neurosci. 7, 1034–1039. doi: 10.1038/nn1325
Ono, D., Weaver, D. R., Hastings, M. H., Honma, K. I., Honma, S., and Silver, R. (2024). The Suprachiasmatic nucleus at 50: looking Back, then looking forward. J. Biol. Rhythm. 39, 135–165. doi: 10.1177/07487304231225706
Paul, K. N., Dugovic, C., Turek, F. W., and Laposky, A. D. (2006). Diurnal sex differences in the sleep-wake cycle of mice are dependent on gonadal function. Sleep 29, 1211–1223. doi: 10.1093/sleep/29.9.1211
Paul, K. N., Laposky, A. D., and Turek, F. W. (2009a). Reproductive hormone replacement alters sleep in mice. Neurosci. Lett. 463, 239–243. doi: 10.1016/j.neulet.2009.07.081
Paul, K. N., Losee-Olson, S., Pinckney, L., and Turek, F. W. (2009b). The ability of stress to alter sleep in mice is sensitive to reproductive hormones. Brain Res. 1305, 74–85. doi: 10.1016/j.brainres.2009.09.055
Peterfi, Z., Churchill, L., Hajdu, I., Obal, F. Jr., Krueger, J. M., and Parducz, A. (2004). Fos-immunoreactivity in the hypothalamus: dependency on the diurnal rhythm, sleep, gender, and estrogen. Neuroscience 124, 695–707. doi: 10.1016/j.neuroscience.2003.10.047
Reis, C., and Paiva, T. (2019). Delayed sleep-wake phase disorder in a clinical population: gender and sub-population diferences. Sleep Sci 12, 203–213. doi: 10.5935/1984-0063.20190086
Roehrs, T. A., and Roth, T. (2016). Gender differences in the efficacy and safety of chronic nightly zolpidem. J. Clin. Sleep Med. 12, 319–325. doi: 10.5664/jcsm.5574
Saelee, R., Haardörfer, R., Johnson, D. A., Gazmararian, J. A., and Suglia, S. F. (2023). Racial/ethnic and sex/gender differences in sleep duration trajectories from adolescence to adulthood in a US National Sample. Am. J. Epidemiol. 192, 51–61. doi: 10.1093/aje/kwac156
Saper, C. B., Cano, G., and Scammell, T. E. (2005). Homeostatic, circadian, and emotional regulation of sleep. J. Comp. Neurol. 493, 92–98. doi: 10.1002/cne.20770
Schwartz, M. D., and Mong, J. A. (2013). Estradiol modulates recovery of REM sleep in a time-of-day-dependent manner. Am. J. Physiol. Regul. Integr. Comp. Physiol. 305, R271–R280. doi: 10.1152/ajpregu.00474.2012
Shi, T., Shah, I., Dang, Q., Taylor, L., and Jagannath, A. (2024). Sex-specific regulation of the cortical transcriptome in response to sleep deprivation. Front. Neurosci. 17:1303727. doi: 10.3389/fnins.2023.1303727
Swift, K. M., Keus, K., Echeverria, C. G., Cabrera, Y., Jimenez, J., Holloway, J., et al. (2020). Sex differences within sleep in gonadally intact rats. Sleep 43:zsz289. doi: 10.1093/sleep/zsz289
Vida, B., Hrabovszky, E., Kalamatianos, T., Coen, C. W., Liposits, Z., and Kallo, I. (2008). Oestrogen receptor alpha and beta immunoreactive cells in the suprachiasmatic nucleus of mice: distribution, sex differences and regulation by gonadal hormones. J. Neuroendocrinol. 20, 1270–1277. doi: 10.1111/j.1365-2826.2008.01787.x
Wu, B., Ma, L., Zhang, E., Du, J., Liu, S., Price, J., et al. (2018). Sexual dimorphism of sleep regulated by juvenile hormone signaling in Drosophila. PLoS Genet. 14:e1007318. doi: 10.1371/journal.pgen.1007318
Zhang, B., and Wing, Y. K. (2006). Sex differences in insomnia: a meta-analysis. Sleep 29, 85–93. doi: 10.1093/sleep/29.1.85
Keywords: sex chromosome, gender differences, estrogen, androgen, NREM, REM
Citation: Ralston M, Ehlen JC and Paul K (2024) Reproductive hormones and sex chromosomes drive sex differences in the sleep–wake cycle. Front. Neurosci. 18:1478820. doi: 10.3389/fnins.2024.1478820
Received: 11 August 2024; Accepted: 11 October 2024;
Published: 31 October 2024.
Edited by:
Melissa A. St. Hilaire, Merrimack College, United StatesReviewed by:
Marilyn J. Duncan, University of Kentucky, United StatesCopyright © 2024 Ralston, Ehlen and Paul. This is an open-access article distributed under the terms of the Creative Commons Attribution License (CC BY). The use, distribution or reproduction in other forums is permitted, provided the original author(s) and the copyright owner(s) are credited and that the original publication in this journal is cited, in accordance with accepted academic practice. No use, distribution or reproduction is permitted which does not comply with these terms.
*Correspondence: Ketema Paul, a2V0ZW1hLnBhdWxAdWNsYS5lZHU=
Disclaimer: All claims expressed in this article are solely those of the authors and do not necessarily represent those of their affiliated organizations, or those of the publisher, the editors and the reviewers. Any product that may be evaluated in this article or claim that may be made by its manufacturer is not guaranteed or endorsed by the publisher.
Research integrity at Frontiers
Learn more about the work of our research integrity team to safeguard the quality of each article we publish.