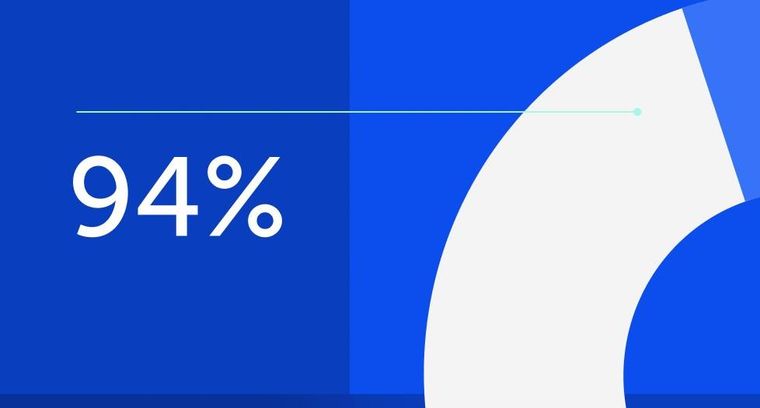
94% of researchers rate our articles as excellent or good
Learn more about the work of our research integrity team to safeguard the quality of each article we publish.
Find out more
EDITORIAL article
Front. Neurosci., 16 July 2024
Sec. Sleep and Circadian Rhythms
Volume 18 - 2024 | https://doi.org/10.3389/fnins.2024.1453328
This article is part of the Research TopicDevelopment of Circadian Clock Functions, volume IIView all 11 articles
Editorial on the Research Topic
Development of circadian clock functions, volume II
In multicellular organisms like ourselves, there exists not only a single circadian clock but rather a network of clocks throughout the body that operate in coordination. The central pacemaker of this system is the suprachiasmatic nucleus (SCN). The SCN is itself synchronized to the environment by local external light-dark cycles and it then communicates this information to the rest of the body. However, this is not a steady state relationship: As our bodies undergo changes throughout their developmental trajectory, there are corresponding adjustments in circadian organization. These adjustments necessitate consideration not only of external synchronizing cues, but also internal circadian cues that entrain and organize clocks throughout the body.
Because the proper maintenance of circadian rhythms is important in early life, addressing the developing circadian clock is critical in the neonatal intensive care unit (NICU). This is particularly pertinent for preterm infants prior to gestational week 30, who have not yet opened their eyes, making photic entrainment unreliable (Arimitsu et al.). Consequently, there is a need to consider alternative methods of entrainment, such as internal entraining signals: It is not known however, which signaling molecules mediate the internal synchronization of circadian clocks. Glucocorticoids are one potential candidate that can be especially relevant during fetal development, as their receptor response can be gated by time (Lehmann et al.). Another potential candidate are Opn4 (melanopsin) aptamers, or melapts. Given that the SCN receives light input directly from melanopsin-expressing intrinsically photosensitive retinal ganglion cells (ipRGCs), melapts can potentially intervene in light-stimulated phase shifts of circadian clocks (Nakazawa et al.). These candidate signaling molecules could have therapeutic implications for circadian entrainment in artificial environments such as the NICU.
The development of circadian clock systems appears to undergo stages of unification (from absence to presence of synchronization) and specification (organized phases through maturation) (Myung et al., 2021). Carmona-Alcocer et al. work tracing the trajectories of nascent SCN neuronal development through the patterns of neuropeptide expression reveals the delayed specification of arginine vasopressin (AVP) and vasoactive intestinal peptide (VIP) neurons. Surprisingly, this time course of specification exhibits some sexual dimorphism. In addition, the synchronization among clock neurons follows a developmental trend that deteriorates with old age. This loss of synchronization may be due to changes in the neurotransmitter GABA, one of the primary interneuronal signaling molecules in the SCN, during aging. Olde Engberink et al. find that GABA, canonically an “inhibitory” neurotransmitter, becomes less inhibitory and progressively more excitatory with age, which effectively affects the coupling strength among neurons within the SCN clock network. In aged mice, this results in changes to the spectral power of circadian rhythmicity and a longer period in the SCN oscillation. The mechanism behind this has a simple theoretical explanation: synchronization is driven by the frequencies of component oscillators, not their periods. If their period distribution of individual oscillators within the SCN is symmetric, the frequency distribution becomes skewed, making the synchronized frequency differ from the mean frequency (Myung et al.).
The molecular clock itself—the transcriptional-translational feedback loop intrinsic to single cells—also changes during development. Indeed, the core clock component Cryptochrome (Cry) has previously been found to have developmental criticality (Ono et al., 2013). The Cry1 isoform specifically influences circadian period in behavior across development, summarized as: “when Cry1 expression was higher for longer [in early development], the free-running period [of locomotor activity] was longer” (Schirmer et al.).
Finally, the development of the circadian clock has effects on physiology in both model organisms and importantly, in humans. A human's intrinsic circadian period is reflected in their chronotype (morningness-eveningness). In a two-sample Mendelian randomization analysis, Yang et al. find a causal relationship between a morning chronotype in humans and the structural features of the cerebral cortex. Intriguingly, in mice, electroencephalography (EEG) recordings of cortex reflect these structural changes and show age-dependent circadian changes with circadian power weakened with age (Masuda et al.). Developing circadian clocks also have significant relevance to neurovascular function (Mitchell and Gillette).
In conclusion, circadian clocks are present in all developing systems and they appear with different strengths and specifications, enabling coordination of multiple subsystems in the brain and the body. The precise mechanisms that determine how such coordination is achieved through various interactions will continue to provoke ongoing investigation.
JM: Writing – original draft, Writing – review & editing. RS: Writing – review & editing. JJ: Writing – review & editing. TN: Writing – review & editing. DO: Writing – review & editing.
The author(s) declare financial support was received for the research, authorship, and/or publication of this article. This work was financially supported by the Higher Education Sprout Project by the Ministry of Education (MOE) in Taiwan, and the National Science and Technology Council (NSTC), Taiwan (112-2314-B-038-063) (to JM), National Science Foundation (1749500) and National Institute of Health (1R01NS102962) (to RS), National Institute of Health (R35GM151020) (to JJ), and JSPS KAKENHI (19K06360, 24K10029) (to TN), (18H02477, 20KK0177, 21H00422, 21H00307, 21H02526) (to DO).
The authors declare that the research was conducted in the absence of any commercial or financial relationships that could be construed as a potential conflict of interest.
The author(s) declared that they were an editorial board member of Frontiers, at the time of submission. This had no impact on the peer review process and the final decision.
All claims expressed in this article are solely those of the authors and do not necessarily represent those of their affiliated organizations, or those of the publisher, the editors and the reviewers. Any product that may be evaluated in this article, or claim that may be made by its manufacturer, is not guaranteed or endorsed by the publisher.
Myung, J., Nakamura, T. J., Jones, J. R., Silver, R., and Ono, D. (2021). Development of circadian clock functions. Front. Neurosci. 15:735007. doi: 10.3389/fnins.2021.735007
Keywords: circadian clock, development, aging, entrainment, synchronization, suprachiasmatic nucleus (SCN)
Citation: Myung J, Silver R, Jones JR, Nakamura TJ and Ono D (2024) Editorial: Development of circadian clock functions, volume II. Front. Neurosci. 18:1453328. doi: 10.3389/fnins.2024.1453328
Received: 22 June 2024; Accepted: 01 July 2024;
Published: 16 July 2024.
Edited and reviewed by: Radhika Basheer, United States Department of Veterans Affairs, United States
Copyright © 2024 Myung, Silver, Jones, Nakamura and Ono. This is an open-access article distributed under the terms of the Creative Commons Attribution License (CC BY). The use, distribution or reproduction in other forums is permitted, provided the original author(s) and the copyright owner(s) are credited and that the original publication in this journal is cited, in accordance with accepted academic practice. No use, distribution or reproduction is permitted which does not comply with these terms.
*Correspondence: Jihwan Myung, amlod2FuQHRtdS5lZHUudHc=
Disclaimer: All claims expressed in this article are solely those of the authors and do not necessarily represent those of their affiliated organizations, or those of the publisher, the editors and the reviewers. Any product that may be evaluated in this article or claim that may be made by its manufacturer is not guaranteed or endorsed by the publisher.
Research integrity at Frontiers
Learn more about the work of our research integrity team to safeguard the quality of each article we publish.