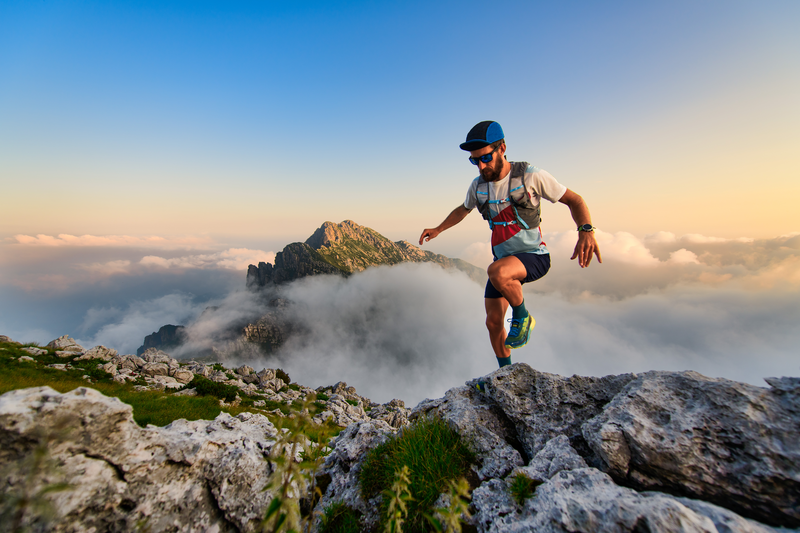
94% of researchers rate our articles as excellent or good
Learn more about the work of our research integrity team to safeguard the quality of each article we publish.
Find out more
ORIGINAL RESEARCH article
Front. Neurosci. , 10 September 2024
Sec. Neurodegeneration
Volume 18 - 2024 | https://doi.org/10.3389/fnins.2024.1435218
This article is part of the Research Topic Neuroinflammation and Neurodegenerative Diseases View all 15 articles
Background: Impaired cognitive ability is one of the most frequently reported neuropsychiatric symptoms in the post-COVID phase among patients. It is unclear whether this condition is related to structural or functional brain changes.
Purpose: In this study, we present a multimodal magnetic resonance imaging study of 36 post-COVID patients and 36 individually matched controls who had a mild form of severe acute respiratory syndrome coronavirus 2 (SARS CoV-2) infection from March 2020 to February 2022. This study aimed to investigate structural and functional brain alterations and their correlation with post-COVID symptoms and neurocognitive functions.
Materials and methods: The study protocol comprised an assessment of physical fatigue [Fatigue Severity Scale (FSS)], mental fatigue (Mental Fatigue Scale (MFS)], depression [Montgomery Asberg Depression Rating Scale (MADRS)], anxiety [Hospital Anxiety and Depression Scale (HAD)], post-COVID Symptoms Severity Score, and neurocognitive status [Repeatable Battery for the Assessment of Neuropsychological Status Update (RBANS)]. The magnetic resonance imaging protocol included morphological sequences, arterial spin labeling (ASL) and dynamic susceptibility contrast-enhanced (DSC) perfusion, diffusion tensor imaging (DTI), and resting-state functional magnetic resonance imaging (fMRI) sequences. Using these protocols, the assessments of macrostructural abnormalities, perfusion, gray matter density, white matter integrity, and brain connectivity were performed.
Results: Post-COVID patients had higher levels of physical fatigue, mental fatigue, depression, and anxiety than controls and showed cognitive impairment in all the RBANS domains except in Visuospatial/Construction. The subjective mental fatigue correlated with objective impaired cognitive ability in the RBANS test, particularly in the Attention domain. There were no differences between patients and controls regarding macrostructural abnormalities, regional volumes, regional perfusion metrics, gray matter density, or DTI parameters. We observed a significant positive correlation between RBANS Total Scale Index score and gray matter volume in the right superior/middle-temporal gyrus (p < 0.05) and a significant negative correlation between the white matter integrity and post-COVID symptoms (p < 0.05) in the same area. The connectivity differences were observed between patients and controls in a few regions, including the right middle frontal gyrus, an important area of convergence of the dorsal and ventral attention networks. We also noted a positive correlation between post-COVID symptoms and increased connectivity in the right temporoparietal junction, which is part of the ventral attention system.
Conclusion: In non-hospitalized subjects with post-COVID, we did not find any structural brain changes or changes in perfusion, compared to controls. However, we noted differences in connectivity within an important area for attention processes, which may be associated with post-COVID brain fog.
Post-COVID condition occurs in individuals with a history of probable or confirmed SARS CoV-2 infection, usually 3 months from the onset of COVID-19, with symptoms that last for at least 2 months and cannot be explained by an alternative diagnosis, according to Delphi consensus, 2021 (Soriano et al., 2022).
Impaired cognitive ability and physical fatigue are the most frequently reported neuropsychiatric symptoms in post-COVID followed by insomnia, depression, and anxiety (Badenoch et al., 2022).
Other common physical symptoms are dyspnea, postural orthostatic tachycardia syndrome (POTS), widespread muscular pain, dizziness, and headache. Physical and mental fatigue are often accompanied by exertional intolerance and post-exertional malaise. Self-reported word-finding difficulties are among the most frequent neurocognitive complaints, such as impaired memory and attention. Cognitive exhaustion can worsen after even very light physical or mental exertion and leads to social isolation and long-term sick leave. A subset of post-COVID patients also fulfills the diagnostic criteria for myalgic encephalomyelitis (ME) and chronic fatigue syndrome (CFS) (Bonilla et al., 2023).
Depression, sleep disturbance, and anxiety, which have been reported to appear at the same time as the other post-COVID symptoms and are unlikely to be a mere consequence of the impaired cognitive ability, may affect the patient's experience of fatigue and their perception of cognitive impairment (Jaltuszewska et al., 2023).
Many studies show that neuropsychiatric symptoms also occur after a mild COVID infection (Graham et al., 2021) and can persist up to 2 years (Fernández-de-Las-Peñas et al., 2022).
The SARS-CoV-2 virus affects the brain directly from the cribriform plate, which is situated close to the olfactory bulb, or follows a hematogenous route (Singh et al., 2023). At the histopathological level, the three most common brain abnormalities reported during the acute phase of infection are inflammation, hypoxia, and coagulation disorders (Lu et al., 2020). However, the mechanism underlying the pathophysiology of neurologic symptoms in the post-COVID phase, especially after a mild COVID infection, is still debated. Advanced multimodal neuroimaging techniques have been employed to identify structural and functional brain changes in post-COVID patients.
There are a wide range of studies that discuss the alterations in the cerebral structure and cerebral blood flow from the post-acute phase until about 1 year from SARS-CoV-2 infection (Lu et al., 2020; Thapaliya et al., 2023; Hafiz et al., 2022; Tu et al., 2021; Douaud et al., 2022; Bendella et al., 2023; Planchuelo-Gómez et al., 2023; Cecchetti et al., 2022; Paolini et al., 2023; Heine et al., 2023; Du et al., 2022; Latini et al., 2022; Ajčević et al., 2023; Churchill et al., 2023; Qin et al., 2021; Díez-Cirarda et al., 2023), with a primary focus on hospitalized patients. However, there are very few studies investigating non-hospitalized patients with persisting symptoms more than 1 year after infection.
There is a significant heterogeneity in the reported results concerning both the specific regions affected and the types of gray (GM) and white matter (WM) modifications. Studies show evidence of increased (Lu et al., 2020; Thapaliya et al., 2023; Hafiz et al., 2022; Tu et al., 2021), decreased (Douaud et al., 2022; Bendella et al., 2023; Planchuelo-Gómez et al., 2023), and unchanged (Cecchetti et al., 2022) GM volume. Similarly, changes in WM volume in different regions (Lu et al., 2020; Paolini et al., 2023; Heine et al., 2023), WM hyperintensities (Du et al., 2022; Latini et al., 2022), and decreases in perfusion in different regions (Ajčević et al., 2023) have been reported.
There is a general consensus in finding a more pronounced modification in the cortical thickness and the WM microstructure in post-COVID patients with a severe disease than in those with a mild disease (Bendella et al., 2023; Qin et al., 2021).
Many different types of alterations in functional connectivity, such as altered connectivity in the right frontal pole and in the middle-temporal gyrus (Paolini et al., 2023), have been reported in functional magnetic resonance imaging (fMRI) studies (Du et al., 2022; Churchill et al., 2023).
Only a few studies have assessed the association between brain alterations detected using magnetic resonance imaging (MRI) and cognitive impairment measured through a neuropsychological assessment (Douaud et al., 2022; Díez-Cirarda et al., 2023; Andriuta et al., 2022).
In this study, we present a multimodal neuroimaging analysis of GM, WM, brain connectivity, and perfusion-based parameters in 36 post-COVID patients and 36 individually matched controls who had mild SARS-CoV-2 infection from March 2020 to February 2022. The primary aim of this study was to investigate the structural and functional brain alterations and the secondary aim was to investigate if these alterations are associated with post-COVID symptoms, mental fatigue, and neuropsychological assessment.
We recruited 36 post-COVID patients and 36 controls individually matched for age (±5 years) and date of COVID infection (±3 months) from April 2022 to February 2023. The post-COVID patients were enrolled at the Uppsala post-COVID outpatient clinic. The patients included in the study had COVID infection from the beginning of the pandemic through February 2022. The inclusion criteria were age between 18 and 65 years, a previous acute COVID infection that did not require hospitalization, and new onset persisting symptoms for at least 3 months after COVID infection according to the WHO definition (Soriano et al., 2022). The additional inclusion criteria were mental fatigue as one of the three most disabling symptoms, along with physical examination, radiological analysis, and blood tests ruling out other causes of the symptoms. The exclusion criteria were malignancies, autoimmune diseases, chronic diseases, and neurological, psychiatric, lung, and cardiovascular diseases prior to COVID infection.
The patients were not using antidepressants or other drugs that affect the central nervous system (CNS) at the time of recruitment such as anticonvulsants, antiemetics, CNS stimulants, muscle relaxants, narcotics, anxiolytics, or sedatives. In addition, 11 patients had COVID infection during the first wave in Sweden (February 2020–August 2020), 12 patients had COVID infection in the second wave (September 2020–February 2021), six patients in the third wave (March 2021 to July 2021), and seven in the fourth wave (August 2021–April 2022). The fourth wave was primarily driven by the omicron variant.
Every patient was individually matched to a control who had contracted COVID infection during the same period (±3 months) but experienced no post-COVID symptoms. Patients and controls has similar education levels and socioeconomic statuses, with the majority having a high level of education. The matched controls were recruited at the Östhammars primary healthcare center among patients who sought care for simple disabilities not related to COVID infection.
All the participants had a polymerase chain reaction (PCR)-verified COVID infection except for five patients and three controls who had the infection at the beginning of the pandemic when PCR capacity in Sweden was not sufficient to test all the suspected cases.
The time interval between the COVID infection and recording the brain MRI was 20.7 ± 7.6 months for patients and 23.8 ± 7.5 months for controls. The participants who were infected during the fourth wave had a shorter time interval between the infection and brain imaging (8 ± 1.7 months for patients, 11 ± 2.4 months for controls) than those who acquired the infection in the first, second, and third waves (24 ± 4.9 months for patients and 27 ± 4.6 months for controls).
The study was approved by the Swedish Ethical Review Authority (2022-01626-01) and was conducted in accordance with the Declaration of Helsinki. All participants provided their written informed consent.
The study protocol comprised assessments of physical fatigue [Fatigue Severity Scale (FSS)] (Krupp et al., 1989), mental fatigue [Mental Fatigue Scale (MFS)] (Johansson et al., 2010), depression [Montgomery Asberg Depression Rating Scale (MADRS)] (Montgomery and Asberg, 1979), and anxiety [Hospital Anxiety and Depression Scale (HAD)] (Zigmond and Snaith, 1983).
The following cut-offs adjusted for the Swedish populations were used: FSS: score range 0–63, with a cut-off of ≥36. MFS: score range 0–42, with a cut-off of ≥10. MADRS: score range 0–54, categorized as 0–12 for no depression, 13–19 for mild depression, 20–34 for moderate depression, and >35 for severe depression. HAD depression: score 0–21, cut-off ≥7. HAD anxiety: score range 0–21, with a cut-off of ≥7.
Post-COVID symptom severity was assessed using a Symptom Severity Scale (SSS) based on 17 symptoms on a 10-point scale (where 0 indicates no symptom and 10 indicates the maximum severity of the symptom). The score range is from 0 to 170.
The symptoms included in the SSS are shown in Table 1.
Neurocognitive status was assessed using the Repeatable Battery for the Assessment of Neuropsychological Status (RBANS) (Randolph et al., 1998; Birberg Thornberg et al., 2022). RBANS generates index scores for five neurocognitive domains, including immediate memory, visuospatial/constructional, language, attention, and delayed memory, as well as a Total Scale Index score.
All patients except two, who were not possible to reach, were followed up with a new assessment of their post-COVID Symptom Severity Score 1 year after the first test.
Continuous data are represented as mean and standard deviation. Categorical data are represented as frequency and percentage.
Clinical symptoms and assessments between patients and controls were compared using the Mann–Whitney U-test. The correlation between MFS and RBANS was assessed using the Spearman's correlation coefficient and p-values were adjusted using the Bonferroni correction. The symptom scores from the first assessment and 1-year follow-up were compared using the Wilcoxon signed-rank test. Clinical symptoms and assessments between the first three waves and the fourth wave were compared using the Mann–Whitney U-test, and p-values were adjusted using the Bonferroni correction. p-values < 0.05 were considered statistically significant. Statistical analyses were performed using R (version 4.2.2).
MRI scans was performed at the Uppsala University Hospital. All examinations were performed using a 3T system (Achieva d-Stream, Philips Healthcare, Amsterdam, The Netherlands). All subjects were scanned in a supine position using a 32-channel head coil. The images obtained through three-dimensional (3D) T1-weighted (3D-T1w), axial two-dimensional (2D) T2-weighted (2D-T2w), 3D fluid-attenuated inversion recovery (FLAIR), 2D axial diffusion-weighted imaging (DWI), and susceptibility-weighted imaging (SWI) were acquired for morphological evaluation and volumetric analysis. Perfusion-weighted imaging included a 3D pseudo-continuous arterial spin labeling (pCASL) sequence with gradient spin-echo readout and dynamic susceptibility contrast (DSC) acquisition. Additionally, resting-state fMRI (rs-fMRI) and diffusion tensor imaging (DTI) were acquired as part of the imaging protocol. A full description of the imaging protocol is provided in Supplementary Table 1.
Two certified neuroradiologists (JW and DF) performed independent evaluations regarding WM changes, cortical infarcts, lacunar infarcts, microbleeds, and global atrophy. WM changes were assessed using the FLAIR images and categorized according to the Fazekas scale (0–III) (Fazekas et al., 1987). The number of cortical infarcts, lacunar infarcts, and microbleeds were noted; the infarcts were assessed using mainly FLAIR and 2D-T2-weighted sequences, and microbleeds were assessed in the SWI images. When microbleeds were present, they were assessed using a slightly modified Medication Adherence Rating Scale (MARS) (Gregoire et al., 2009) (using the SWIp sequence instead of GRE T2* and not recording laterality).
The width of the cerebral sulci was assessed using the global cerebral atrophy (GCA) scale (Pasquier et al., 1996).
The differences in routine morphological measures were compared between patients and controls using chi-squared or Fisher tests as appropriate, utilizing SPSS (version 28.0.1.0, IBM SPSS, Armonk, NY). A p-value of < 0.05 was regarded as statistically significant.
The 3D-T1w images of all the subjects were processed with FreeSurfer software (http://surfer.nmr.mgh.harvard.edu/, version 7.4.0) using the recon-all pipeline. GM volumes and cortical thickness were extracted for the following cortical and subcortical regions: the frontal, parietal, occipital, and temporal lobes (see Supplementary Table 2 for components), cerebellum, hippocampus, putamen, pallidum, and thalamus. In addition, total cerebral gray matter, total cerebral white matter, and total cerebellar white matter were extracted.
The pCASL-based cerebral blood flow (CBF) maps (CBFpCASL) were automatically calculated by the scanner according to the model recommended by Alsop et al. (2015). Based on the DSC acquisition, parametric maps of CBF, cerebral blood volume (CBV), mean transit time (MTT), time to peak (TTP), leakage, coefficient of variation (CoV), capillary transit time heterogeneity (CTH), cerebral metabolic rate of oxygen (CMRO2), and oxygen extraction fraction (OEF) were calculated using Cercare Medical Neurosuite (Cercare Medical, Aarhus, Denmark). Parametric maps were registered to the corresponding 3D-T1w image. The perfusion-based measures as listed above were extracted from cortical and subcortical regions derived from FreeSurfer as described above.
Hypothesis testing was focused on the derived regional parameters, that is, perfusion-based metrics, cortical volumes, and thicknesses, comparing the measures between controls and patients. First, the Shapiro–Wilk test was performed to test for normality. To test whether any differences were present between controls and patients, we performed a Kruskal–Wallis test for non-normal distributed data with Dunn's correction for multiple comparisons. The post-hoc analysis was performed for all the cortical regions for each parameter.
The voxel-based morphometry (VBM) analysis of the gray matter was performed in FSL (the FMRIB Software Library) toolbox (https://fsl.fmrib.ox.ac.uk) version 6.0.6.1 using the standard processing pipeline FSL_VBM as described in detail (Jenkinson et al., 2012).
(i) A group comparison was performed for patients vs. controls using age and gender as non-explanatory co-regressors.
(ii) The analysis of patients vs. controls was conducted using age and gender as non-explanatory co-regressors, adding “time between COVID infection and MRI” as an additional non-explanatory co-regressor.
(iii) A group comparison was performed for patients vs. controls using only the first, second, and third waves again using age and gender as non-explanatory co-regressors.
(iv) A correlation analysis was performed using the three most relevant test scores, notably RBANS total scale index score, MFS, and SSS, again using age and gender as non-explanatory co-regressors.
All variables were transformed into the range of −1 to +1 with a mean of 0. A statistical threshold was defined as p < 0.05 corrected using threshold-free cluster enhancement (TFCE) (Smith and Nichols, 2009).
The track-based spatial statistics (TBSS) analysis of the white matter was performed in FSL version 6.0.6.1 using the standard processing pipeline FSL_TBSS as described in detail (Jenkinson et al., 2012). The studied parameters related to the white matter integrity included fractional anisotropy (FA), mean diffusivity, and axial and radial diffusivity. Four equivalent analyses were performed similar to the VBM analysis described above. Again, a statistical threshold of TFCE-corrected p < 0.05 was applied.
Resting fMRI analysis was performed in FSL version 6.0.6.1 using the standard processing pipeline MELODIC as described in detail (Jenkinson et al., 2012). First, a tensorial independent component analysis (TICA) was performed using 20 independent components. Then, a dual regression analysis was performed using the same setup as above: first, a group comparison between patients and controls was carried out and then a correlation analysis was performed with the same three clinical scores, notably RBANS Total Scale Index score, MFS, and SSS, again using age and gender as non-explanatory co-regressors and a statistical threshold of TFCE-corrected p < 0.05.
The detailed clinical information regarding the date of COVID infection and responses to rating scales and neurocognitive tests are reported in Supplementary Table 3. The participants were aged between 24 and 64 years. The mean age of COVID patients was 44.2 ± 10.2 years and that of controls was 44.6 ± 10.5 years. The majority (66.6%) of the patients in both groups were women (Table 1).
The post-COVID Symptom Severity Score (values ranging between 0 and 170) was 62.8 ± 24.9 for patients and 8.6 ± 12.8 for controls (p < 0.001).
The patient-reported complaints with the highest mean value were cognitive fatigue (7.7 ± 1.9), physical fatigue (6.8 ± 2.2), and dyspnea (5.1 ± 3.2; Table 1).
The patients fulfilled the criteria for mild depression (MADRS 15.7 ± 8.0, HAD depression 7.4 ± 4.5) and were just below the cut-off score for anxiety (HAD anxiety 6.5 ± 4.1). The levels of depressive symptoms and anxiety were anyway higher (p < 0.001) compared to healthy controls (Table 1).
They reported higher levels of both physical fatigue (FSS 55.4 ± 9.8) and mental fatigue (MFS 22.8 ± 6.0) compared to healthy controls (p < 0.001).
Neurocognitive testing showed a lower RBANS Total Scale Index score in patients (p < 0.001). Differences were found between patients and controls in attention and language (p < 0.001), immediate memory (p = 0.027), and delayed memory domains (p < 0.002), whereas no difference was observed in visuospatial/construction domain (p < 0.152).
Subjective mental fatigue assessed with the MFS test correlated with objective cognitive impairment measured with the RBANS score (r = −0.46, p < 0.05, Table 2). Particularly, patients with higher levels of subjective mental fatigue had lower performance in the attention domain (r = −0.56, p = 0.002; Table 2). The other four RBANS domains did not correlate with the subjective mental fatigue.
When we compared patients who contracted the omicron variant (fourth wave) with patients with earlier COVID variants, there was no significant difference between the groups in symptoms, neurocognitive ability, and responses to rating scales (Supplementary Table 4).
One year after the first assessment, 34 patients were re-evaluated with the post-COVID Symptoms Assessment Scale (SAS). Post-COVID Symptom Severity Score decreased from 62.7 ± 25.6 at the first medical visit to 47.3 ± 26.7 after 1 year (p < 0.001). Self-reported cognitive fatigue was also found to be improved (from 7.7 ± 1.9 at the first visit to 6.2 ± 2.5 after 1 year, p < 0.001). However, when asked to estimate their health in relation to post-COVID symptoms, only two patients reported feeling completely recovered (Supplementary Table 3).
Both reviewers observed that there were no significant differences in the number of cortical infarcts, lacunar infarcts, white matter hyperintensities, global cerebral atrophy, or microbleeds between patients and controls (Table 3).
There were no significant differences in volume or cortical thickness in any of the anatomical regions between patients and controls (Figures 1, 2).
Figure 1. Volume analysis. Volumes of the cerebral lobes, cerebral central gray matter structures and cerebellum. No significant differences were observed between patients and controls. GM, total cerebral gray matter. WM, total cerebral white matter.
Figure 2. Cortical thickness of cerebral lobes. No significant differences were observed between patients and controls.
There were no significant differences in CBFpCASL, CBF, CBV, MTT, TTP, leakage, CV, CTH, CMRO2, or OEF in any of the anatomical regions between patients and controls (Supplementary Figures 1–10).
(i) There was no significant group difference between patients and controls in gray matter VBM.
(ii) Adding “time between COVID infection and MRI” as an additional co-regressor did not change those results.
(iii) The comparison between patients and controls exclusively in the first, second, and third waves (early variants) resulted still in no significant differences.
(iv) In the whole group, we observed a significant positive correlation with RBANS total index score (p < 0.05 TFCE-corrected) for gray matter volume in the right superior/middle-temporal gyrus (Figure 3).
Figure 3. Correlation analysis shows a positive correlation between gray matter volume and RBANS Total Index score in the posterior part of the right superior-/middle-temporal gyrus (yellow).
(i) There was no significant group difference between patients and controls in the white matter TBSS analysis.
(ii) Adding “time between COVID infection and MRI” as an additional co-regressor did not change the results.
(iii) Again, analysis of patients and controls in only the first, second, and third waves (early variants) resulted in no significant differences.
(iv) In the whole group, we observed a significant negative correlation between FA in the right superior-/middle-temporal gyrus and the SSS (TFCE-corrected p < 0.05; Figure 4).
Figure 4. Track-based spatial statistics shows a significant negative correlation between Symptom Severity Score (SSS) and FA in the right superior-/middle-temporal gyrus (blue).
Based on dual regression, patients had a significantly stronger connectivity in the right middle frontal gyrus (MFG) compared to controls, while they exhibited a significantly weaker connectivity in the right inferior parietal lobule and the left fronto-parietal junction compared to controls (see Figure 5).
Figure 5. Dual regression resting state fMRI analysis shows stronger connectivity in the right middle frontal gyrus (orange) in patients compared to controls and weaker connectivity in the right inferior parietal lobule (blue) and left fronto-parietal junction (green).
Additionally, we observed a significantly positive correlation in the whole group using the SSS, notably in the right posterior temporoparietal junction (TPJ) and bilateral temporo-occipital junction, and weaker correlations in the left frontobasal and left superior parietal areas were observed. There was a minor negative correlation in the left parietal region (Figure 6).
Figure 6. Correlation between SSS and connectivity. Positive correlation in the right posterior temporoparietal junction (TPJ; blue) and bilateral temporo-occipital junction (pink), and weaker correlations in the left frontobasal (green) and left superior parietal (red) areas. There was a minor negative correlation in the left parietal region (yellow).
The aim of the present study was to use a multimodal imaging approach to evaluate brain alterations in post-COVID patients after a mild COVID infection and their correlation with post-COVID symptoms (SSS), mental fatigue (MFS), and neuropsychological assessments (RBANS test). We found no significant morphological, microstructural, or perfusion brain changes in non-hospitalized post-COVID patients with brain fog who were treated at an outpatient post-COVID clinic in comparison with age-matched healthy controls who had acquired COVID infection in the same period. However, some differences were observed between the two groups regarding functional brain connectivity, particularly in the right middle frontal gyrus, which may suggest a disturbed regulation mechanism between ventral and dorsal attention networks.
We found no differences in macrostructural abnormalities, such as infarcts and atrophy, microbleeds, number, and localization of WM hyperintensities and general brain volume. The absence of differences in focal lesions is in agreement with a recent review of routine MR imaging studies, which found minimal abnormalities in post-COVID subjects and no conclusive evidence of a correlation between MRI findings and symptoms (Vasilev et al., 2023). As for brain volumes, previous studies have shown divergent results (Lu et al., 2020; Bendella et al., 2023).
Regional perfusion measures were not different in our study between post-COVID subjects and controls. Apart from CBF measurements, we also used novel perfusion-based measures such as CTH, OEF, and CMRO2 (Mouridsen et al., 2014) for an assessment of microvascular derangements. We found no evidence of alterations in post-COVID patients.
Regional GM density and regional WM fractional anisotropy were also similar between the patient groups. The absence of microstructural white matter changes in the whole brain is mostly in line with the findings of Heine et al. (2023) who compared 47 subjects with post-COVID symptoms with subjects without COVID infection although they found aberrant FA of the thalamus. Paolini et al. (2023) also failed to identify any changes in fractional anisotropy but, on the other hand, they showed increases in diffusivity measures in several areas in patients previously hospitalized because of COVID infection, which can be interpreted as an increase in water content. The differences in results may be explained by both differences in infection severity (hospitalized vs. non-hospitalized) and choice of the comparison group (without a medical history of COVID infection vs. with a medical history of COVID infection but without remaining symptoms).
The only positive findings were differences in resting-state functional connectivity, where post-COVID patients showed higher connectivity in the right middle frontal gyrus. Altered connectivity in a similar frontal region in post-COVID patients was also detected by Paolini et al. (2023). The right middle frontal gyrus has been proposed to be the node that links the ventral and dorsal attention networks by acting as a “circuit-breaker” between exogenous and endogenous attention control (Corbetta et al., 2008). Fox et al. (2006) have shown that the right middle frontal gyrus interrupts ongoing processes in the dorsal network and reorients attention to a novel task-relevant external stimulus. Impaired connectivity in the right MFG and the resulting loss of the flexible modulation between endogenous and exogenous attention may eventually be responsible for certain post-COVID symptoms such as difficulties with focus and concentration in a specific task, as well as oversensitivity to minimal external stimuli, which are perceived as distracting and increasing the mental exhaustion.
This hypothesis is supported by the fact that we additionally found a positive correlation between post-COVID symptoms and increased connectivity in the right TPJ, which is the main part of the ventral attentional system. The increased connectivity in the ventral network in participants with post-COVID symptoms may reflect their inability to filter distracting signals.
Post-COVID patients showed cognitive impairment in all RBANS domains except visuospatial/construction. These results are in accord with another study in which a neurocognitive test was performed using Montreal Cognitive Assessment (MoCA) and where no differences in orientation and visuoconstructive functions were observed (Birberg Thornberg et al., 2022).
The patients who reported a higher value of subjective mental fatigue at the MFS also had a more pronounced objective impaired cognitive ability at the RBANS test, especially in the Attention domain. The fact that impaired attention ability is the neurocognitive domain that correlates with the subjective feeling of mental exhaustion in our patients reinforces the hypothesis that deficit in attention mechanisms can be the basis of the subjective mental fatigue in post-COVID patients and it is in line with our results in connectivity.
We found a positive correlation between neurocognitive test and GM thickness in the right superior-/middle-temporal gyrus. Interestingly, there was also a correlation between the intensity of post-COVID symptoms (SSS) and WM anisotropy in the same area. These findings are difficult to interpret but imply that this region is involved in both cognitive processes and post-COVID symptoms. Neurocognitive impairment did not correlate with WM anisotropy, similar to a previous study in 86 post-COVID patients (Díez-Cirarda et al., 2023).
Another point of interest to consider is the time of infection. Patients in our study acquired SARS-CoV-2 infection from the beginning of the pandemic in 2020 to February 2022. This period includes four waves of the pandemic in Sweden, where the fourth wave was dominated by the omicron variant. The participants who had the infection in the first three waves were not vaccinated, while those who contracted omicron (the fourth wave) were vaccinated with at least two shots. To assess whether individuals who contracted the virus before receiving the vaccination—during a period when the virus was more aggressive—could have more pronounced structural brain changes, we separately analyzed patients and controls in the first three waves but found no structural differences in brain changes.
There was also no difference in post-COVID symptoms, neurocognitive ability, depression, anxiety, and physical and mental fatigue between the patients who had contracted the infection in the first three waves and those who were infected by the omicron variant. However, the small number of patients in the omicron group may not be sufficient to detect a difference between the two groups (omicron and “pre-omicron”). Therefore, these data must be interpreted with caution.
We did not find any correlation between brain changes and the time passed between infection and MRI.
Except for the participants who were infected with the omicron variant (a minority), our patients had experienced post-COVID symptoms for a long time before undergoing MRI (24 ± 5 months), which is longer compared to most other studies.
After 2 years of illness, one patient in our study was diagnosed with myalgic encephalomyelitis/chronic fatigue syndrome (ME/CFS), and others were under investigation since most of the symptoms reported by individuals with ME/CFS and post-COVID are similar. According to evidence from a prospective study, it appears that the subset of post-COVID patients who also fulfill the diagnostic criteria for ME/CFS presents more persistent, high-severity symptoms at a 20-month follow-up, while patients affected by COVID show an overall health improvement in the post-COVID phase (Legler et al., 2023).
In our study, when we reassessed the patients using the post-COVID Symptoms Assessment Scale (SAS) after 1 year, all of them had a lower intensity of symptoms, although only two felt fully recovered; there was thus a trend toward slow improvement.
Interestingly, the localization of the impaired brain connectivity seems different between patients with ME/CFS and post-COVID patients. In ME/CFS, connectivity seems impaired within the brainstem and from the brainstem to key subcortical structures. Moreover, hippocampal connections to the midbrain cuneiform nucleus and the medulla are enhanced, suggesting that the hippocampus has a compensatory role for the impaired connections (Barnden et al., 2019; Inderyas et al., 2024). In our post-COVID study, we could not find any impairment in the brainstem connectivity, suggesting that different brain areas are possibly affected.
A strength of the study is the stringent inclusion criteria for controls. The higher proportion of women (66.6%) in our study reflects the higher prevalence of post-COVID symptoms in women in the general population.
Another strength is the comprehensive MR protocol, including macrostructural, microstructural, perfusion, and cortical activation outcomes. The perfusion protocol included an assessment of capillary transit time heterogeneity, which is a recently introduced metric with the potential to provide significant insights into microvascular function. However, a potential weakness is that the statistical power of the present study may not have been sufficient to detect small structural differences between the study groups. In particular, the results of the subgroup analysis (omicron variant vs. “pre-omicron” variant) can be affected by the low number of patients in the fourth wave.
Our study presents a hypothesis (post-COVID brain fog is related to attention problem, which in turn is related to impaired connectivity in the right MFG and increased connectivity in the ventral network) that has potential high relevance in clinical practice and future research. This hypothesis needs to be confirmed in larger studies. Additionally, the negative finding concerning the absence of morphological, microstructural, or perfusion brain changes is relevant in clinical practice to avoid unnecessary brain MRI examinations in post-COVID patients, since connectivity studies are not included in the routine MRI examination.
This study found no macrostructural or microstructural brain changes, nor alterations in perfusion, in patients who exhibited persistent subjective and objective impaired cognitive abilities long after a mild COVID infection. However, differences in functional connectivity were observed in a few regions, notably the right medial frontal gyrus, which is an important area for attention processes. These changes could play a role in post-COVID brain fog. However, the results of our exploratory analysis in this region require confirmation through larger studies.
The raw data supporting the conclusions of this article will be made available by the authors, without undue reservation.
The studies involving humans were approved by Swedish Ethical Review Authority (2022-01626-01). The studies were conducted in accordance with the local legislation and institutional requirements. The participants provided their written informed consent to participate in this study.
SF: Conceptualization, Writing – original draft, Writing – review & editing, Data curation, Formal analysis, Funding acquisition, Investigation, Methodology, Resources, Supervision. MF: Formal analysis, Investigation, Methodology, Software, Writing – original draft, Writing – review & editing. DF: Data curation, Formal analysis, Investigation, Methodology, Writing – review & editing. SH: Formal analysis, Investigation, Methodology, Software, Writing – review & editing. JW: Conceptualization, Data curation, Formal analysis, Funding acquisition, Investigation, Methodology, Resources, Supervision, Writing – original draft, Writing – review & editing.
The author(s) declare financial support was received for the research, authorship, and/or publication of this article. The study was funded by grants from the Healthcare Board, Region of Uppsala, Sweden (FoU grant to SF). David Fällmar was supported by the Swedish Society for Medical Research (SSMF, PD21-0136) and by Hjärnfonden (PS2021-0026).
The authors thank the statistician Katja Gabrysch at the Uppsala Clinical Research Center for assisting the statistical analysis of clinical tests.
The authors declare that the research was conducted in the absence of any commercial or financial relationships that could be construed as a potential conflict of interest.
All claims expressed in this article are solely those of the authors and do not necessarily represent those of their affiliated organizations, or those of the publisher, the editors and the reviewers. Any product that may be evaluated in this article, or claim that may be made by its manufacturer, is not guaranteed or endorsed by the publisher.
The Supplementary Material for this article can be found online at: https://www.frontiersin.org/articles/10.3389/fnins.2024.1435218/full#supplementary-material
Ajčević, M., Iscra, K., Furlanis, G., Michelutti, M., Miladinović, A., Buoite Stella, A., et al. (2023). Cerebral hypoperfusion in post-COVID-19 cognitively impaired subjects revealed by arterial spin labeling MRI. Sci. Rep. 13:5808. doi: 10.1038/s41598-023-32275-3
Alsop, D. C., Detre, J. A., Golay, X., Günther, M., Hendrikse, J., Hernandez-Garcia, L., et al. (2015). Recommended implementation of arterial spin-labeled perfusion MRI for clinical applications: a consensus of the ISMRM perfusion study group and the European consortium for ASL in dementia. Magn. Reson. Med. 73, 102–116. doi: 10.1002/mrm.25197
Andriuta, D., Si-Ahmed, C., Roussel, M., Constans, J. M., Makki, M., Aarabi, A., et al. (2022). Clinical and imaging determinants of neurocognitive disorders in post-acute COVID-19 patients with cognitive complaints. J. Alzheimers Dis. 87, 1239–1250. doi: 10.3233/JAD-215506
Badenoch, J. B., Rengasamy, E. R., Watson, C., Jansen, K., Chakraborty, S., Sundaram, R. D., et al. (2022). Persistent neuropsychiatric symptoms after COVID-19: a systematic review and meta-analysis. Brain Commun. 4:fcab297. doi: 10.1093/braincomms/fcab297
Barnden, L. R., Shan, Z. Y., Staines, D. R., Marshall-Gradisnik, S., Finegan, K., Ireland, T., et al. (2019). Intra brainstem connectivity is impaired in chronic fatigue syndrome. Neuroimage Clin. 24:102045. doi: 10.1016/j.nicl.2019.102045
Bendella, Z., Widmann, C. N., Layer, J. P., Layer, Y. L., Haase, R., Sauer, M., et al. (2023). Brain volume changes after COVID-19 compared to healthy controls by artificial intelligence-based MRI volumetry. Diagnostics 13:1716. doi: 10.3390/diagnostics13101716
Birberg Thornberg, U., Andersson, A., Lindh, M., Hellgren, L., Divanoglou, A., Levi, R., et al. (2022). Neurocognitive deficits in COVID-19 patients five months after discharge from hospital. Neuropsychol. Rehabil. 14, 1–25. doi: 10.1080/09602011.2022.2125020
Bonilla, H., Quach, T. C., Tiwari, A., Bonilla, A. E., Miglis, M., Yang, P. C., et al. (2023). Myalgic encephalomyelitis/chronic fatigue syndrome is common in post-acute sequelae of SARS-CoV-2 infection (PASC): results from a post-COVID-19 multidisciplinary clinic. Front. Neurol. 14:1090747. doi: 10.3389/fneur.2023.1090747
Cecchetti, G., Agosta, F., Canu, E., Basaia, S., Barbieri, A., Cardamone, R., et al. (2022). Cognitive, EEG, and MRI features of COVID-19 survivors: a 10-month study. J. Neurol. 269, 3400–3412. doi: 10.1007/s00415-022-11047-5
Churchill, N. W., Roudaia, E., Chen, J. J., Gilboa, A., Sekuler, A., Ji, X., et al. (2023). Effects of post-acute COVID-19 syndrome on the functional brain networks of non-hospitalized individuals. Front. Neurol. 14:1136408. doi: 10.3389/fneur.2023.1136408
Corbetta, M., Patel, G., and Shulman, G. L. (2008). The reorienting system of the human brain: from environment to theory of mind. Neuron 58, 306–24. doi: 10.1016/j.neuron.2008.04.017
Díez-Cirarda, M., Yus, M., Gómez-Ruiz, N., Polidura, C., Gil-Martínez, L., Delgado-Alonso, C., et al. (2023). Multimodal neuroimaging in post-COVID syndrome and correlation with cognition. Brain 146, 2142–2152. doi: 10.1093/brain/awac384
Douaud, G., Lee, S., Alfaro-Almagro, F., Arthofer, C., Wang, C., McCarthy, P., et al. (2022). SARS-CoV-2 is associated with changes in brain structure in UK Biobank. Nature 604, 697–707. doi: 10.1038/s41586-022-04569-5
Du, Y. Y., Zhao, W., Zhou, X. L., Zeng, M., Yang, D. H., Xie, X. Z., et al. (2022). Survivors of COVID-19 exhibit altered amplitudes of low frequency fluctuation in the brain: a resting-state functional magnetic resonance imaging study at 1-year follow-up. Neural Regen Res. 17, 1576–1581. doi: 10.4103/1673-5374.327361
Fazekas, F., Chawluk, J. B., Alavi, A., Hurtig, H. I., and Zimmerman, RA. (1987). MR signal abnormalities at 1.5 T in Alzheimer's dementia and normal aging. AJR Am. J. Roentgenol. 149, 351–356. doi: 10.2214/ajr.149.2.351
Fernández-de-Las-Peñas, C., Rodríguez-Jiménez, J., Cancela-Cilleruelo, I., Guerrero-Peral, A., Martín-Guerrero, J. D., García-Azorín, D., et al. (2022). Post-COVID-19 symptoms 2 years after SARS-CoV-2 Infection among hospitalized vs. nonhospitalized patients. JAMA Netw. Open 5:e2242106. doi: 10.1001/jamanetworkopen.2022.42106
Fox, M. D., Corbetta, M., Snyder, A. Z., Vincent, J. L., and Raichle, M. E. (2006). Spontaneous neuronal activity distinguishes human dorsal and ventral attention systems. Proc. Natl. Acad. Sci. USA. 103, 10046–10051. doi: 10.1073/pnas.0604187103
Graham, E. L., Clark, J. R., Orban, Z. S., Lim, P. H., Szymanski, A. L., Taylor, C., et al. (2021). Persistent neurologic symptoms and cognitive dysfunction in non-hospitalized Covid-19 “long haulers”. Ann. Clin. Transl. Neurol. 8, 1073–1085. doi: 10.1002/acn3.51350
Gregoire, S. M., Chaudhary, U. J., Brown, M. M., Yousry, T. A., Kallis, C., Jäger, H. R., et al. (2009). The Microbleed Anatomical Rating Scale (MARS): reliability of a tool to map brain microbleeds. Neurology 73, 1759–1766. doi: 10.1212/WNL.0b013e3181c34a7d
Hafiz, R., Gandhi, T. K., Mishra, S., Prasad, A., Mahajan, V., Di, X., et al. (2022). Higher limbic and basal ganglia volumes in surviving COVID-negative patients and the relations to fatigue. Neuroimage Rep. 2:100095. doi: 10.1016/j.ynirp.2022.100095
Heine, J., Schwichtenberg, K., Hartung, T. J., Rekers, S., Chien, C., Boesl, F., et al. (2023). Structural brain changes in patients with post-COVID fatigue: a prospective observational study. EClinicalMedicine 58:101874. doi: 10.1016/j.eclinm.2023.101874
Inderyas, M., Thapaliya, K., Marshall-Gradisnik, S., Barth, M., and Barnden, L. (2024). Subcortical and default mode network connectivity is impaired in myalgic encephalomyelitis/chronic fatigue syndrome. Front. Neurosci. 17:1318094. doi: 10.3389/fnins.2023.1318094
Jaltuszewska, S., Chojnacka-Szawlowska, G., Majkowicz, M., Zdonczyk, S., Homenda, W., Hebel, K., et al. (2023). Illness perception and the severity of depression and anxiety symptoms in patients with multimorbidity: observational cohort studies. J. Clin. Med. 13:69. doi: 10.3390/jcm13010069
Jenkinson, M., Beckmann, C. F., Behrens, T. E., Woolrich, M. W., and Smith, S. M. (2012). FSL. Neuroimage 62, 782–790. doi: 10.1016/j.neuroimage.2011.09.015
Johansson, B., Starmark, A., Berglund, P., Rödholm, M., and Rönnbäck, L. (2010). A self-assessment questionnaire for mental fatigue and related symptoms after neurological disorders and injuries. Brain Inj. 24, 2–12. doi: 10.3109/02699050903452961
Krupp, L. B., LaRocca, N. G., Muir-Nash, J., and Steinberg, A. D. (1989). The fatigue severity scale. Application to patients with multiple sclerosis and systemic lupus erythematosus. Arch. Neurol. 46, 1121–1123. doi: 10.1001/archneur.1989.00520460115022
Latini, F., Fahlström, M., Fällmar, D., Marklund, N., Cunningham, J. L., Feresiadou, A., et al. (2022). Can diffusion tensor imaging (DTI) outperform standard magnetic resonance imaging (MRI) investigations in post-COVID-19 autoimmune encephalitis? Ups J. Med. Sci. 127. doi: 10.48101/ujms.v127.8562
Legler, F., Meyer-Arndt, L., Mödl, L., Kedor, C., Freitag, H., Stein, E., et al. (2023). Long-term symptom severity and clinical biomarkers in post-COVID-19/chronic fatigue syndrome: results from a prospective observational cohort. EClinicalMedicine 63:102146. doi: 10.1016/j.eclinm.2023.102146
Lu, Y., Li, X., Geng, D., Mei, N., Wu, P. Y., Huang, C. C., et al. (2020). Cerebral micro-structural changes in COVID-19 patients - an MRI-based 3-month Follow-up Study. EClinicalMedicine 25:100484. doi: 10.1016/j.eclinm.2020.100484
Montgomery, S. A., and Asberg, M. (1979). A new depression scale designed to be sensitive to change. Br J Psychiatry. 134, 382–389. doi: 10.1192/bjp.134.4.382
Mouridsen, K., Hansen, M. B., Østergaard, L., and Jespersen, S. N. (2014). Reliable estimation of capillary transit time distributions using DSC-MRI. J. Cereb. Blood Flow. Metab. 34, 1511–1521. doi: 10.1038/jcbfm.2014.111
Paolini, M., Palladini, M., Mazza, M. G., Colombo, F., Vai, B., Rovere-Querini, P., et al. (2023). Brain correlates of subjective cognitive complaints in COVID-19 survivors: a multimodal magnetic resonance imaging study. Eur. Neuropsychopharmacol. 68, 1–10. doi: 10.1016/j.euroneuro.2022.12.002
Pasquier, F., Leys, D., Weerts, J. G., Mounier-Vehier, F., Barkhof, F., Scheltens, P., et al. (1996). Inter- and intraobserver reproducibility of cerebral atrophy assessment on MRI scans with hemispheric infarcts. Eur. Neurol. 36, 268–72. doi: 10.1159/000117270
Planchuelo-Gómez, Á., García-Azorín, D., Guerrero, Á. L., Rodríguez, M., Aja-Fernández, S., and de Luis-García, R. (2023). Structural brain changes in patients with persistent headache after COVID-19 resolution. J. Neurol. 270, 13–31. doi: 10.1007/s00415-022-11398-z
Qin, Y., Wu, J., Chen, T., Li, J., Zhang, G., Wu, D., et al. (2021). Long-term microstructure and cerebral blood flow changes in patients recovered from COVID-19 without neurological manifestations. J. Clin. Invest. 131:e147329. doi: 10.1172/JCI147329
Randolph, C., Tierney, M. C., Mohr, E., and Chase, T. N. (1998). The Repeatable Battery for the Assessment of Neuropsychological Status (RBANS): preliminary clinical validity. J. Clin. Exp. Neuropsychol. 20, 310–3109. doi: 10.1076/jcen.20.3.310.823
Singh, S., Meher, N., Mohammed, A., Razab, M. K. A. A., Bhaskar, L. V. K. S., Nawi, N. M., et al. (2023). Neurological infection and complications of SARS-CoV-2: a review. Medicine 102:e30284. doi: 10.1097/MD.0000000000030284
Smith, S. M., and Nichols, T. E. (2009). Threshold-free cluster enhancement: addressing problems of smoothing, threshold dependence and localisation in cluster inference. Neuroimage 44, 83–98. doi: 10.1016/j.neuroimage.2008.03.061
Soriano, J. B., Murthy, S., Marshall, J. C., Relan, P., Diaz, J. V., and WHO Clinical Case Definition Working Group on Post-COVID-19 Condition (2022). A clinical case definition of post-COVID-19 condition by a Delphi consensus. Lancet Infect. Dis. 22, e102–e107. doi: 10.1016/S1473-3099(21)00703-9
Thapaliya, K., Marshall-Gradisnik, S., Barth, M., Eaton-Fitch, N., and Barnden, L. (2023). Brainstem volume changes in myalgic encephalomyelitis/chronic fatigue syndrome and long COVID patients. Front Neurosci. 17:1125208. doi: 10.3389/fnins.2023.1125208
Tu, Y., Zhang, Y., Li, Y., Zhao, Q., Bi, Y., Lu, X., et al. (2021). Post-traumatic stress symptoms in COVID-19 survivors: a self-report and brain imaging follow-up study. Mol Psychiatry. 26, 7475–7480. doi: 10.1038/s41380-021-01223-w
Vasilev, Y., Blokhin, I., Khoruzhaya, A., Kodenko, M., Kolyshenkov, V., Nanova, O., et al. (2023). Routine brain MRI findings on the long-term effects of COVID-19: a scoping review. Diagnostics 13:2533. doi: 10.3390/diagnostics13152533
Keywords: post-COVID, MRI, attention network, cognitive impairment, resting state fMRI, right middle frontal gyrus, right temporoparietal junction
Citation: Fineschi S, Fahlström M, Fällmar D, Haller S and Wikström J (2024) Comprehensive MRI assessment reveals subtle brain findings in non-hospitalized post-COVID patients with cognitive impairment. Front. Neurosci. 18:1435218. doi: 10.3389/fnins.2024.1435218
Received: 19 May 2024; Accepted: 07 August 2024;
Published: 10 September 2024.
Edited by:
Pradeep Kumar, All India Institute of Medical Sciences, IndiaReviewed by:
Miren Altuna, Fundacion CITA Alzheimer, SpainCopyright © 2024 Fineschi, Fahlström, Fällmar, Haller and Wikström. This is an open-access article distributed under the terms of the Creative Commons Attribution License (CC BY). The use, distribution or reproduction in other forums is permitted, provided the original author(s) and the copyright owner(s) are credited and that the original publication in this journal is cited, in accordance with accepted academic practice. No use, distribution or reproduction is permitted which does not comply with these terms.
*Correspondence: Serena Fineschi, c2VyZW5hLmZpbmVzY2hpQHB1YmNhcmUudXUuc2U=
Disclaimer: All claims expressed in this article are solely those of the authors and do not necessarily represent those of their affiliated organizations, or those of the publisher, the editors and the reviewers. Any product that may be evaluated in this article or claim that may be made by its manufacturer is not guaranteed or endorsed by the publisher.
Research integrity at Frontiers
Learn more about the work of our research integrity team to safeguard the quality of each article we publish.