- Institute of Neurophysiology, Charité - Universitätsmedizin Berlin, Corporate Member of Freie Universität Berlin and Humboldt Universität zu Berlin, Berlin, Germany
N-methyl-D-aspartate (NMDA) receptor antagonists are widely used to pharmacologically model schizophrenia and have been recently established in the treatment of treatment-resistant major depression demonstrating that the pharmacology of this substance class is complex. Cortical gamma oscillations, a rhythmic neuronal activity associated with cognitive processes, are increased in schizophrenia and deteriorated in depressive disorders and are increasingly used as biomarker in these neuropsychiatric diseases. The opposite use of NMDA receptor antagonists in schizophrenia and depression raises the question how their effects are in accordance with the observed disease pathophysiology and if these effects show a consequent sex-specificity. In this study in rats, we investigated the effects of subchronic (14 days) intraperitoneal injections of the NMDA receptor antagonist MK-801 at a subanesthetic daily dose of 0.2 mg/kg on the behavioral phenotype of adult female and male rats and on pharmacologically induced gamma oscillations measured ex vivo from the hippocampus. We found that MK-801 treatment leads to impaired recognition memory in the novel object recognition test, increased stereotypic behavior and reduced grooming, predominantly in female rats. MK-801 also increased the peak power of hippocampal gamma oscillations induced by kainate or acetylcholine only in female rats, without affecting the peak frequency of the oscillations. The findings indicate that blockade of NMDA receptors enhances gamma oscillations predominantly in female rats and this effect is associated with behavioral changes in females. The results are in accordance with clinical electrophysiological findings and highlight the importance of hippocampal gamma oscillations as a biomarker in schizophrenia and depression.
1 Introduction
N-methyl-D-aspartate (NMDA) receptor antagonists such as ketamine, phencyclidine (PCP) and MK-801 are widely used to pharmacologically model schizophrenia (SZ) in animals (Lee and Zhou, 2019). Beyond that, ketamine and MK-801 show antidepressant effects (Trullas and Skolnick, 1990; Adell, 2020; Johnston et al., 2024) demonstrating that the pharmacology of this substance class is complex.
SZ has a substantial and increasing global disease burden accounting for 1.5% of disability-adjusted life years in the 25–49-year age group (Vos et al., 2020). Although the pathophysiology of the disease is still not understood, recent genomic studies suggest an association with genes expressed in excitatory and inhibitory neurons in the brain (Trubetskoy et al., 2022). Fine mapping of the associated genes such as the NMDA receptor subunit GRIN2A showed a concentration of the associated genes in pre- and postsynaptic locations (Trubetskoy et al., 2022). These data are in accordance with the postulated interneuron hypothesis of schizophrenia (Lewis et al., 2005) supposing a primary disturbance of the rhythm-generating fast-spiking parvalbumin positive perisomatic inhibitory interneurons (PV INs) in the cortex. The subsequent abnormal local field potential rhythms at gamma and beta frequencies are suggested to be the central pathophysiological trait of schizophrenia, responsible for the negative, positive and cognitive symptoms of the disease (Lee et al., 2003; Lewis et al., 2005). Indeed, increased resting-state and task induced gamma oscillations have been found in patients with schizophrenia (Teale et al., 2008; Donkers et al., 2013; Hirano et al., 2015) and different disease models in animals (Jadi et al., 2016; Speers and Bilkey, 2021) such as acute application of the NMDA receptor antagonist MK-801 (Kehrer et al., 2007; Lemercier et al., 2017). Modulating these oscillatory microcircuits in combination with alternative approaches such as cognitive training and brain stimulation was suggested to represent novel and selective interventions for improving cognition in schizophrenia (Sohal, 2022).
Depressive disorders possess the sixth highest disease burden globally responsible for 3.5% of disability-adjusted life years in the 25–49-year age group (Vos et al., 2020). Although significant advances have been achieved understanding its pathophysiology, no universally accepted mechanism can explain all symptoms of the disease (Otte et al., 2016). Serotonin reuptake inhibitors, the pharmacological backbone of the treatment, are only effective in one-thirds of patients and show therapeutic effects after couple of weeks (Rush et al., 2006). Recently, the focus was shifted to therapeutics with shorter latency such as the NMDA receptor antagonist ketamine. Indeed, while initial models focused on neurochemical changes in the brain, like the monoamine hypothesis, more recent research turned toward a network mechanism to explain the key symptoms of the disease (Spellman and Liston, 2020). Preclinical and clinical data show an overstimulation of NMDA receptors within these dysfunctional neuronal networks (Adell, 2020; Johnston et al., 2024) and that antidepressants have inhibitory effects on NMDA receptors (Szasz et al., 2007). Conforming to this, several studies reported that cortical GABAergic inhibitory circuits and gamma oscillations are impaired in patients with depression or in animal models of the disease and gamma oscillations were suggested as a biomarker for major depression (Mendez et al., 2012; Fitzgerald and Watson, 2018; Liu et al., 2023).
The opposite use of the same substance family in schizophrenia and depression raises the question how the blockade of NMDA receptors changes important biomarkers of these two psychiatric diseases and if these changes are in accordance with the observed disease pathophysiology. Previous studies have investigated the effect of acutely applied NMDA receptor antagonists on cortical gamma oscillations (Kehrer et al., 2007; Lemercier et al., 2017). However, it remained unclear how a long-term administration scheme, as practiced in depressive patients, affects cortical gamma oscillations. Since women are twice as likely to be diagnosed with depression compared to men (Keers and Aitchison, 2010) it is also important to examine whether differences between females and males exist regarding the effects of NMDA receptor antagonists. To shed light onto these questions, the present study was planned to investigate the sex specific effects of chronic application of the NMDA receptor antagonist MK-801 on hippocampal gamma oscillations and its behavioral counterparts. Our results show that subchronic NMDA receptor antagonist administration increased the power of hippocampal gamma oscillations predominantly in females and this change was accompanied with decreased novel object recognition memory and increased stereotypy suggesting that female rats are more sensitive to the effects of the NMDA receptor antagonist MK-801.
2 Materials and methods
2.1 Animals
All procedures were conducted in accordance with the guidelines of the European Communities Council and the institutional guidelines approved by the Berlin Animal Ethics Committee (Landesamt für Gesundheit und Soziales Berlin, G0036/17). Wistar rats were kept in groups of two individuals of same sex and experimental group (treatment or sham group) in one cage. Animals were housed on a 12/12 h light/dark cycle with ad libitum food and water. Both sexes of animals from an age of P60 were injected daily for 14 days with an aqueous solution of MK-801 maleate (0.2 mg/kg, 4 male and 4 female rats) in the treatment group or with a sterile 0.9% NaCl solution in the sham group (4 male and 4 female rats). The last i.p. injections were done 24 h before starting the experiments (Figure 1A). While the effects of acute administration of MK-801 on hippocampal gamma oscillations were intensively investigated both in vitro (Kehrer et al., 2007; Lemercier et al., 2017) and in vivo (e.g., Sullivan et al., 2015), the hippocampal effects of a longer chronic or subchronic application was previously not tested in vitro.
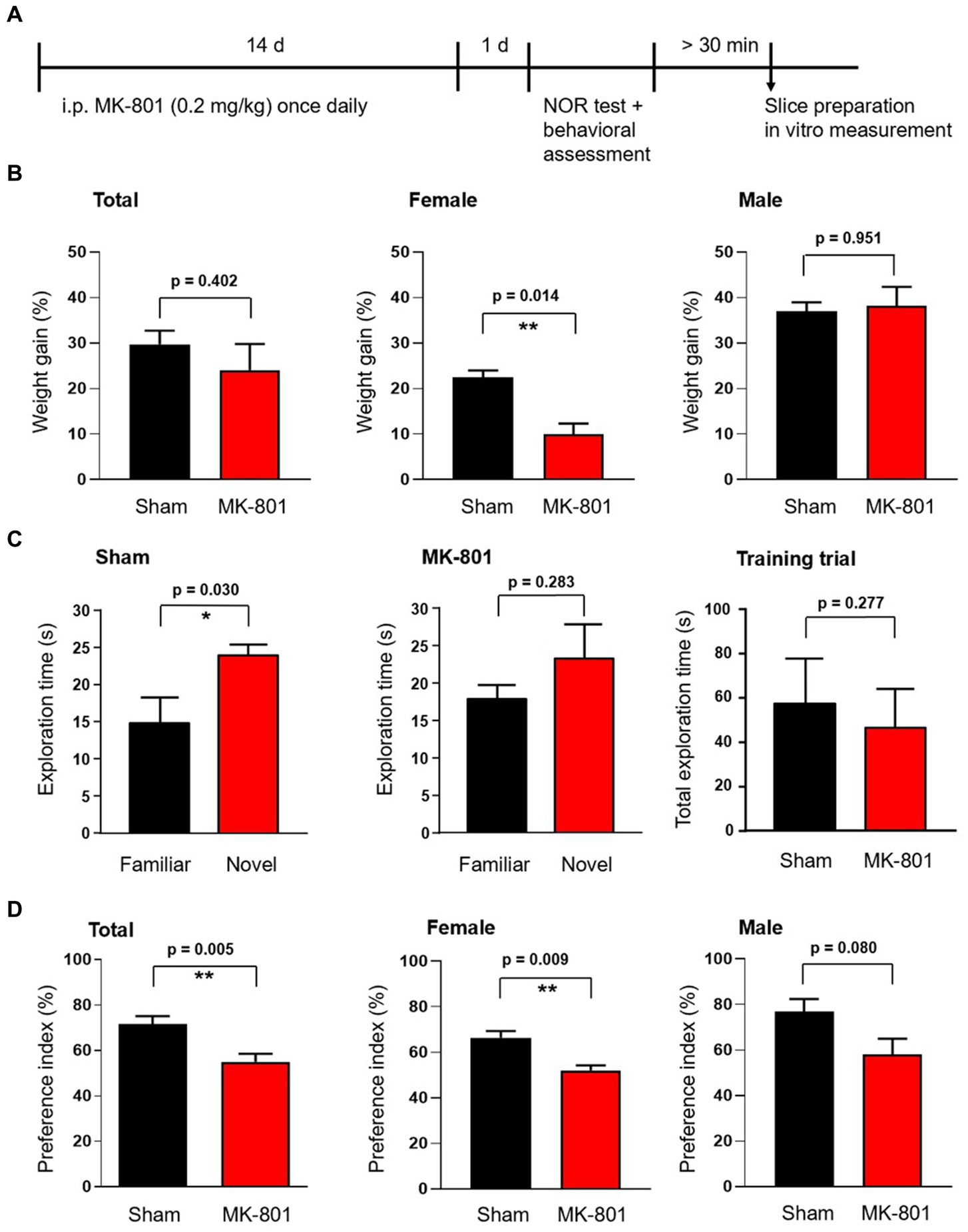
Figure 1. Effect of subchronic MK-801 treatment on the novel object recognition memory of rats. (A) Overview of the treatment scheme and time points of behavioral and ex vivo tests. (B) Bar graphs show the weight gain of animals during the 14-days long application of MK-801 (red) or saline (sham, black). Weight gain was significantly reduced in female animals but not in males. (C) Bar graphs show the exploration times spent exploring the familiar and novel objects in the novel object recognition (NOR) test. Sham treated rats explored novel objects significantly longer than the already known familiar object (left). MK-801 treatment diminished the difference in exploration time (middle). Sham and MK-801 treated animals explored both (identical) objects in the first training trial similarly (right). (D) MK-801 significantly reduced the exploration time spent with the novel object, expressed as novelty preference index (time spent by exploring of the novel object divided by the total exploration time of both objects expressed as percentage, left). The reduction of the preference index by MK-801 was significant in female rats and not in males. Data are mean ± S.E.M, *p < 0.05, **p < 0.01.
2.2 Novel object recognition (NOR) test
The NOR test (Ennaceur, 2010) was carried out according to the protocol by Mathiasen and DiCamillo (2010). Twenty-four hours after the last injection, rats were individually transferred into a separate room and left to acclimatize for 15 min in a resting cage. Before starting the tests, a rat that was not part of the experiment was placed into the test box for 15 min to saturate the box and all objects within with its odor signal. The goal of this was to eliminate exogenous olfactory stimuli in the test box and between objects that would influence the exploration of the objects and their odor-related identification. The experiment was split into two test trials. In the first training trial, two identical objects in standard distance were placed in the test box. The rat was put into the test box for 3 min and recorded on video for the whole time. After 3 min, the animal was removed and put back into the resting cage. One of the objects was exchanged for a novel object. The second test trial started after an intertrial interval of 15 min. The rat was placed for 3 min into the test box and again recorded on video for 3 min. The novel object and side of the novel object within the cage was randomly defined. Animals with no cognitive impairment explore the novel object longer compared to the familiar object. Two independent persons blinded measured the absolute times the rats spent at each object by using the recorded videos. Two parameters were calculated: the exploration time at the objects and the novelty preference index (the time spent by exploring of the novel object divided by the total exploration time of both objects expressed as percentage). Data are presented as mean ± S.E.M. Statistical comparisons of the parameters between sham and MK-801-treated animals were made using Welch t-test. Significance was set at p = 0.05.
2.3 Behavioral assessment
For the determination of psychomotor activity, we used the recorded videos of the NOR testing and analyzed them by measuring the duration of time rats spent with three different types of activity during the first trial. First, locomotor activity was defined as the fraction of time (%) during the 3-min test period spent in locomotion. Second, stereotypy was defined as time fraction (%) spent on head-weaving (>2 times side-to-side motion of the head reaching more than 30 degrees from the body axis) or circling (closed loop of locomotion) or axial turning (circling movements of the forepaws with the haunches remaining still). Third, grooming was defined as the fractional time spent with licking or preening any part of the body (Feinstein and Kritzer, 2013). The sample size was the number of animals. Group size was estimated by the program G*Power3 (Faul et al., 2007). Data are presented as mean ± S.E.M. Statistical comparisons of the parameters between sham and MK-801-treated animals were made using Student’s t-test. Significance was set at p = 0.05.
2.4 Materials
MK-801, Physostigmine (Physo) and kainate (KA) were ordered from Tocris Bioscience (Bristol, UK). Acetylcholine (ACh) was delivered from Sigma-Aldrich (Taufkirchen, Germany).
2.5 Slice preparation
After the behavioral testing, the rats were transported from the animal house to the preparation room and left for at least 30 min to acclimatize. Afterwards, they were decapitated under isoflurane anesthesia. Their brain was immediately removed and placed in an ice-cold sucrose-based and carbogenated (95% O2 / 5% CO2) solution with the following composition (in mM): sucrose, 85; NaCl, 80; NaHCO3, 25; NaH2PO4, 1.25; KCl, 2.5; glucose, 25; CaCl2, 0.5; MgCl2, 3. The hemispheres were cut into 400-μm thick horizontal slices containing the formation of the hippocampus by a vibratome (Leica VT 1200S, Leica Mikrosysteme Vertrieb GmbH, Wetzlar, Germany). The slices were immediately transferred to an interface-type recording chamber and constantly perfused with warm (35°C) and carbogenated artificial cerebrospinal fluid (ACSF) with a flow rate of 1.7 mL/min and a composition (mM) of NaCl, 129; KCl, 3; NaHCO3, 21; NaH2PO4, 1.25; MgSO4, 1.8; CaCl2, 1.6; glucose, 10. The slices were incubated for at least 30 min in the recording chamber before starting the experiment.
2.6 Recording of gamma oscillations
Gamma oscillations were induced by bath application of either KA (150 nM) or Ach and Physo (10 μM and 2 μM, respectively; Schulz et al., 2012a). Local field potentials (LFPs) were recorded by silver chloride electrodes in micropipettes (ACSF filled, resistance ~3 MΩ) placed in the stratum pyramidale of the hippocampal CA3 area as described previously (Wildner et al., 2024). Recordings were amplified by an EXT-2 (NPI electronic, Tamm, Germany) amplifier, analog low pass filtered at 1 kHz and sampled at 5 kHz by a CED 1401 interface (Cambridge Electronic Design, Milton, UK). For the analysis of pharmacologically induced hippocampal gamma oscillations, power spectra were calculated every 2 min with a 120-s window throughout the recording (Lemercier et al., 2016). From these, the power spectra, peak power, peak frequency, and the quality factor (Q-factor) of the oscillations were determined later by the usage of the Spike2 software. The Q-factor of the gamma oscillations was calculated by the equation: Q = f/B, where f is the peak frequency and B is the bandwidth at 50% of maximum peak power (Meier et al., 2020). Neuronal activity in the power spectrum was considered an oscillation if the Q factor was subcritical (above 0.5) (Lemercier et al., 2017). For the comparison between treated and non-treated groups, the above parameters between 90 and 100 min after gamma oscillation stimulation were compared between slices from MK-801 and sham-treated animals. For the analysis of hippocampal oscillations, a second-order Butterworth band-stop (49.5–50.5 Hz) filter was used to remove 50 Hz noise. Since the power of gamma oscillations is lognormally distributed, peak power (μV2) is shown as geometric mean with the geometric standard deviation factor (GeoSD) (Klemz et al., 2021) calculated by GraphPad Prism (Boston, MA, USA). Sample size n was defined as individual slices. Group size estimation was done by the program G*Power3 (Faul et al., 2007). The peak frequency (Hz) is represented as mean ± SEM. For the statistical comparisons of the parameters, we used the t-test. The lognormally distributed power values were first log transformed and then statistically analyzed by Student’s t-test (Klemz et al., 2022). Significance level was set at p = 0.05.
3 Results
3.1 Effects of subchronic MK-801 application on the behavior
To assess the action of MK-801 in female and male rats, we first measured the weight changes of the animals during the subchronic (14 days) application of MK-801 at subanesthetic doses (0.2 mg/kg). During the 2-weeks treatment, MK-801 significantly decreased the relative weight gain of female animals compared to sham-treated controls (10.0 ± 2.3% versus 22.4 ± 1.6%, respectively, p = 0.006; n = 4 in both groups; Figure 1B). We did not observe the same effect in male animals (MK-801: 38.1 ± 4.2%, n = 4; sham: 37.0 ± 1.9%, p = 0.823; n = 4; Figure 1B).
The novel object recognition (NOR) test is widely used to model recognition memory (Ennaceur, 2010). Sham (saline) treated animals spent in the test trial significantly more time exploring the novel object compared to the familiar object (24.0 ± 1.4 s and 14.7 ± 3.6 s, respectively, n = 8, p = 0.030; Figure 1C). MK-801-treated animals failed to significantly differentiate between the novel and familiar object and spent comparable time at each (23.3 ± 4.5 s and 17.8 ± 2.0 s, respectively, n = 8, p = 0.283; Figure 1C). MK-801 might have decreased the novelty preference of the animals because it lowered the object exploration duration in the training trial and herewith the encoding time. To test this possibility we calculated the total exploration times in the training trial in sham and MK-801-treated animals and did not find statistical difference (57.2 ± 7.3 s and 46.4 ± 6.2 s, respectively, n = 8, p = 0.277, Figure 1C right). Comparing the novelty preference indices (the time spent exploring the novel object divided by the total exploration time in the test trial) revealed that MK-801-treated rats spent significantly less time with exploring the novel object compared to the sham-treated animals (p = 0.005; Figure 1D). The sex specific analysis revealed a significant effect of MK-801 only in female rats (66.4 ± 2.9% and 52.0 ± 2.3% in MK-801 and sham, respectively, n = 4 in both groups, p = 0.009; Figure 1D), while male rats showed only a tendency (76.9 ± 5.5% and 57.9 ± 7.0%, respectively, n = 4 in both groups, p = 0.080; Figure 1D).
We investigated next the effect of MK-801 on the behavior of the rats and found that MK-801 treatment did not change the locomotor activity of both female and male animals (Figure 2A). In contrast, we observed an increase of the duration of stereotypic behavior from 16.7 ± 1.3% to 24.3 ± 2.0% (p = 0.006; n = 8 in both groups, Figure 2B). This increase was only observed in female rats (from 17.8 ± 0.5% to 29.0 ± 1.5%, p = 0.0004) and not in males (15.5 ± 2.7% vs. 19.5 ± 1.1%, p = 0.217; Figure 2B). Measuring the time rats spent with grooming revealed a significant decrease from 10.5 ± 2.9% to 1.9 ± 1.1% (p = 0.009, n = 8) without an observed sex difference (Figure 2C).
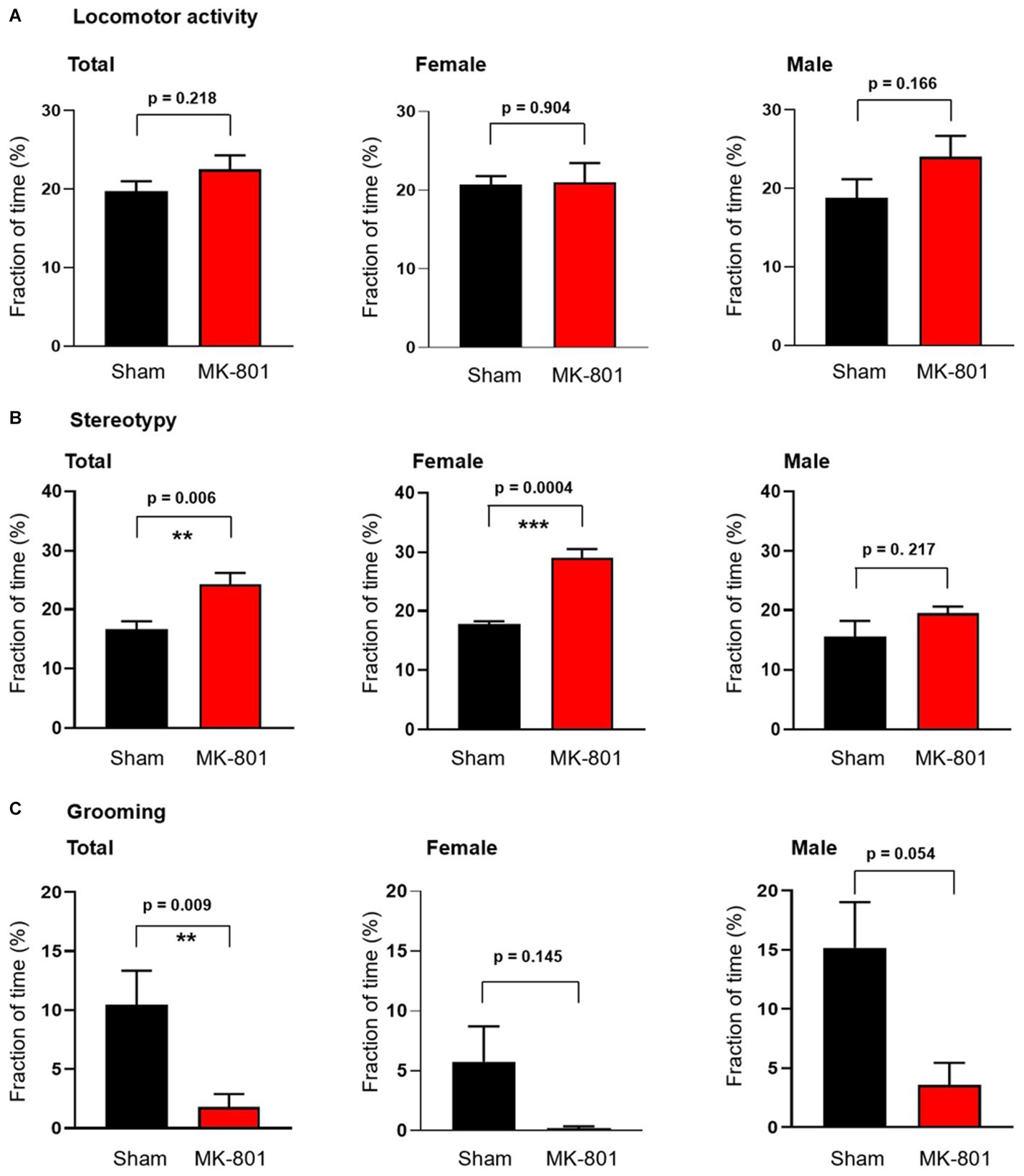
Figure 2. Sex-specific behavioral effects of subchronic MK-801 treatment. (A) MK-801 had no effect on the locomotor activity. (B) 14-day long treatment with MK-801 increased stereotypy in female animals but not in males. (C) MK-801 decreased grooming behavior. Data are shown as mean ± S.E.M, **p < 0.01, ***p < 0.001.
In summary, subchronic low and subanesthetic application of MK-801 had a significant behavioral effect characterized by a reduced weight gain during the 14 days of treatment, an impaired novel object recognition, increased stereotypic behavior and decreased grooming, predominantly in female animals.
3.2 Effects of subchronic MK-801 on in vitro hippocampal gamma oscillations
Local in vitro gamma oscillations can be induced pharmacologically by activation of KA and muscarinic receptors (Fisahn et al., 1998, 2004). Because these gamma oscillations are probably generated by different networks (Bartos et al., 2007) and can be modulated differentially (Schulz et al., 2012b) we tested the effects of MK-801 administration on both variants. The peak power of KA-induced gamma oscillations in hippocampal slices from female and male sham-treated rats did not differ (geometric mean, geometric SD factor: 18.7 μV2, 31.4 μV2, n = 22 and 26.5 μV2, 35.8 μV2, n = 15, respectively, p = 0.891; Figure 3B). MK-801 treatment significantly increased the peak power (geometric mean, geometric SD factor: 21.5 μV2, 31.7 μV2, n = 37 and 109.8 μV2, 8.3 μV2, n = 32, respectively, p = 0.019; Figures 3A,Ca). When analyzed for sex-specific effect, we observed an increase of power only in female rats (sham: geometric mean, geometric SD factor: 18.7 μV2, 31.4 μV2, n = 17; MK-801: 197.7 μV2, 4.5 μV2, n = 22; p = 0.007; Figure 3Cb) while in male animals MK-801 did not have an effect (sham: geometric mean, geometric SD factor: 26.54 μV2, 35.84 μV2, n = 15; MK-801: 56.39 μV2, 12.82 μV2, n = 15, respectively, p = 0.555; Figure 3Cc). We observed neither difference or sex-specific effects in the peak frequency of the induced gamma oscillations from sham and MK-801 treated animals (33.7 ± 0.8 Hz and 34.3 ± 0.9 Hz, respectively, p = 0.614; Figure 3D).
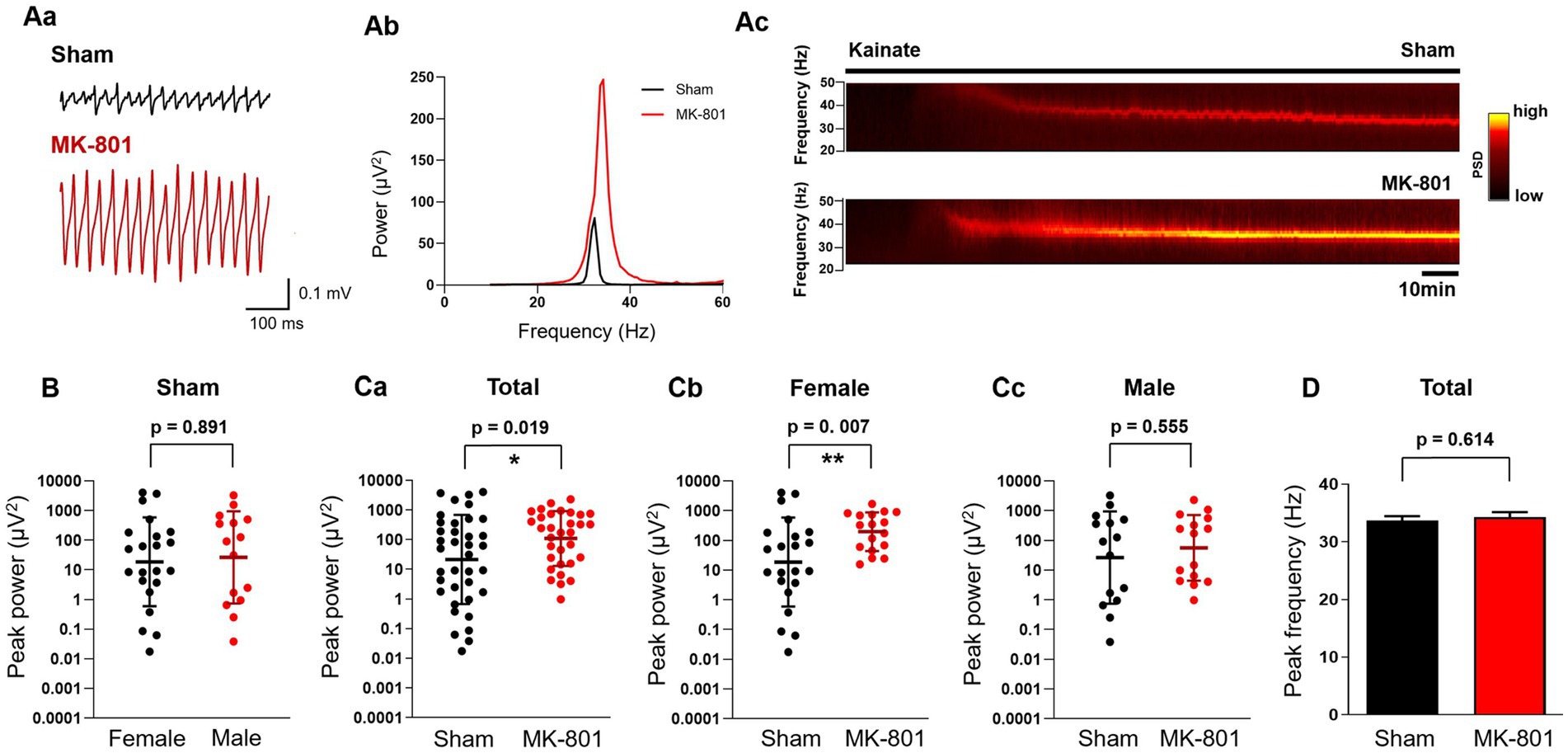
Figure 3. Subchronic MK-801 treatment increased the power of ex vivo gamma oscillations induced by bath application of kainate (KA). (Aa) Representative local field potential traces in hippocampal CA3 slices of sham and MK-801 treated rats after the application of KA (150 nM). (Ab) Representative power spectra of KA-induced hippocampal gamma oscillations from sham (black) and MK-801 (red) treated animals. (Ac) Representative spectrograms from sham (black) and MK-801 (red) treated animals. Horizontal bar indicates the duration of KA application. (B) Scatter plots of the peak power of KA-induced gamma oscillations in female (black) and male (red) sham treated animals. Bars indicate geometric mean and geometric standard deviation factor. (Ca): MK-801 (red) increased the peak gamma power compared to sham (black) treated animals. Bars indicate geometric mean and geometric standard deviation factor. MK-801 (red) increased the peak power of gamma oscillations compared to sham (black) treatment in female rats (Cb) but not in males (Cc). Bars indicate geometric mean and geometric standard deviation factor. (D) MK-801 did not affect the peak frequency of gamma oscillations. PSD, Power spectral density; *p < 0.05, **p < 0.01.
Next, we induced gamma oscillations in hippocampal slices with ACh (10 μM) and Physo (2 μM). The peak power values did not differ in sham treated female and male rats (geometric mean, geometric SD factor: 7.7 μV2, 8.8 μV2, n = 19 and 22.2 μV2, 13.0 μV2, n = 15, respectively, p = 0.238; Figure 4B), similar to findings in mice (Guneykaya et al., 2023). Subchronic treatment with MK-801 increased the power of cholinergically induced gamma oscillations (geometric mean, geometric SD factor: 44.0 μV2, 5.6 μV2, n = 29) compared to sham treatment (13.9 μV2, 11.6 μV2, n = 35; p = 0.031; Figures 4A,Ca). The effect of MK-801 was stronger in female animals compared to males, although it did not reach the significance level (sham: geometric mean, geometric SD factor: 9.8 μV2, 10.7 μV2, n = 20; MK-801: 34.1 μV2, 6.1 μV2, n = 14; p = 0.091; Figure 4Cb). In male rats, MK-801 did not increase the power (sham: geometric mean, geometric SD factor: 22.2 μV2, 13.0 μV2, n = 15; MK-801: 56.0 μV2, 5.3 μV2, n = 15, respectively, p = 0.253; Figure 4Cc). MK-801 treatment did not affect the peak frequency of the oscillations (sham: 37.0 ± 0.8 Hz, n = 35 and MK-801 36.7 ± 0.8 Hz, n = 29, respectively, p = 0.765; Figure 4D) and we did not observe a sex-specific effect of MK-801 on the peak frequency (not shown).
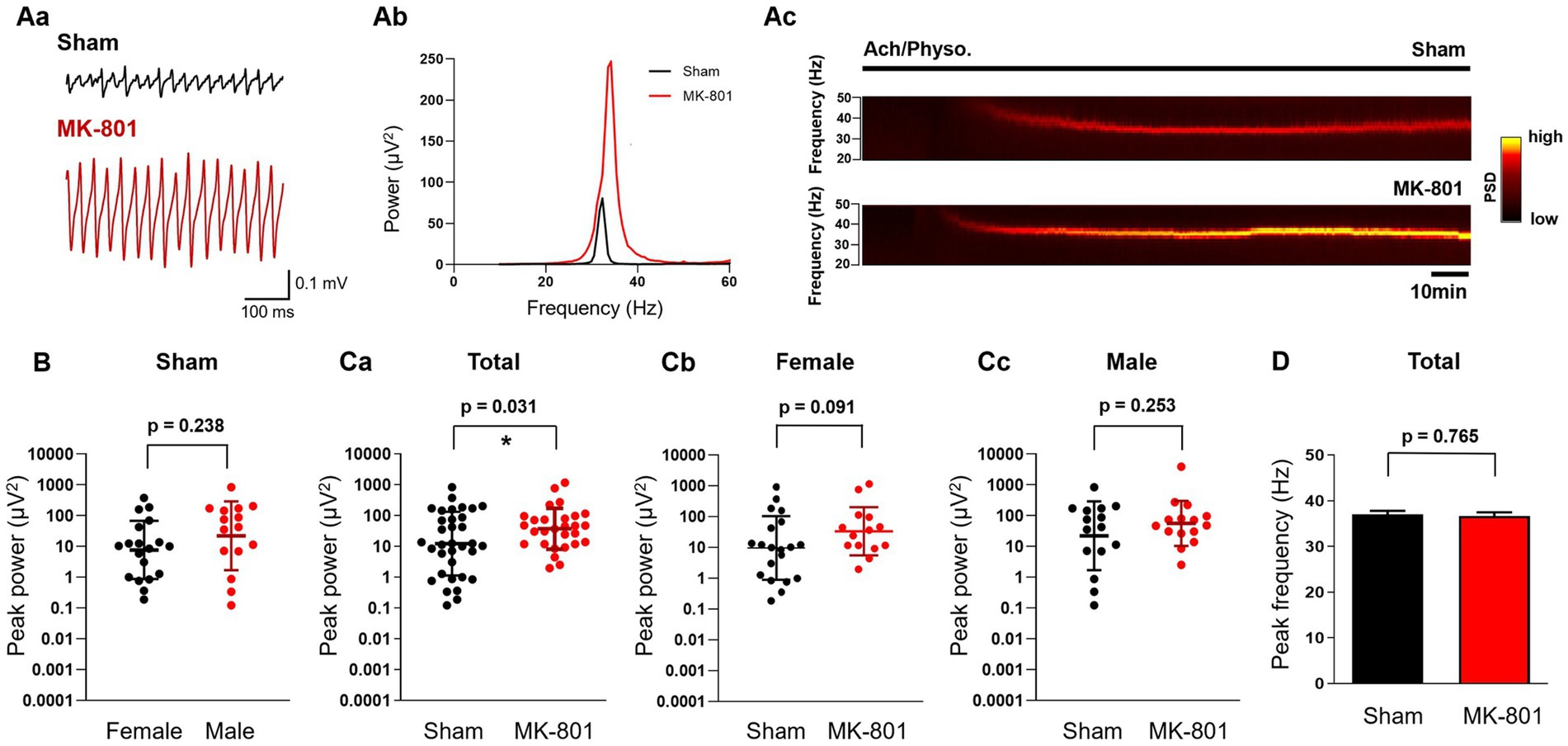
Figure 4. Subchronic MK-801 treatment increased the power of ex vivo gamma oscillations induced by bath application of ACh and Physo. (Aa) Representative local field potential traces in hippocampal CA3 slices of sham and MK-801 treated rats after the application of ACh (10 μM) & Physo (2 μM). (Ab) Representative power spectra of cholinergically induced hippocampal gamma oscillations from sham (black) and MK-801 (red) treated animals. (Ac) Representative spectrograms from sham (black) and MK-801 (red) treated animals. Horizontal bar indicates the duration of Ach/Physo application. (B) Scatter plots of the peak power of cholinergically induced gamma oscillations in female (black) and male (red) sham treated animals. Bars indicate geometric mean and geometric standard deviation factor. (Ca) MK-801 (red) increased the peak gamma power compared to sham (black) treated animals. Bars indicate geometric mean and geometric standard deviation factor. (Cb) Peak gamma oscillation power in sham (black) and MK-801 (red) treated female rats. Bars indicate geometric mean and geometric standard deviation factor. (Cc) Peak power of gamma oscillations in sham (black) and MK-801 (red) treated male rats. Bars indicate geometric mean and geometric standard deviation factor. (D) MK-801 did not affect the peak frequency of gamma oscillations. PSD, Power spectral density; *p < 0.05.
Thus, subchronic MK-801 treatment increased the power of both KA- and acetylcholine induced gamma oscillations in the hippocampus. KA-induced gamma oscillations were clearly sex-specific and significantly increased only in females rats. Cholinergic gamma oscillations showed only a tendency for sex-specificity.
4 Discussion
The aim of this study was to evaluate the effects of subchronic application of the NMDA antagonist MK-801 on the behavior and hippocampal local network activity in rats. We found that a 14-day subanesthetic treatment with MK-801 enhanced the amplitude of hippocampal gamma oscillations. This effect was seen predominantly in female animals and was associated with increased stereotypy, decreased grooming and impaired recognition memory.
Gamma oscillations reflect rhythmic neuronal network activity that is synchronized across neurons and brain areas (Buzsáki and Wang, 2012). They can be registered by scalp electroencephalography (EEG), electrocorticography, magnetoencephalography or by measuring local field potentials (LFPs) intracranially in the brain tissue. In addition, since gamma oscillations are generated locally by an interplay between pyramidal cells and parvalbumin positive interneurons (Hájos and Paulsen, 2009) it is possible to induce and measure them ex vivo in acutely isolated brain slices by means of LFP measurements (Fisahn et al., 1998; Schulz et al., 2012b). Pharmacologically induced ex vivo gamma oscillations in the CA3 were shown to correlate with in vivo neuronal activity and space reference memory (Lu et al., 2011) confirming that they are a useful measure of in vivo network activity and associated behavior in brain slices offering the advantage of investigating real local processes.
Gamma oscillations are proposed to coordinate fundamental neuronal processes underlying cognition (Fries, 2009) and have been associated with higher cognitive functions such as perception, attention, learning and memory (Bosman et al., 2014). In the hippocampus, they play a role in working memory (Yamamoto et al., 2014), in memory replay (Carr et al., 2012) as well as in novel object recognition (Trimper et al., 2014, 2017). The novel object recognition test is widely used to model recognition memory (Ennaceur, 2010). The hippocampus operates as a novelty detector comparing incoming and stored information which might be a key function of exploratory behavior during discovering the environment (Honey et al., 1998; Johnson et al., 2012). The hippocampus is involved in the novel object recognition memory when a delay longer than 10 min is entailed between the first and the second trials of NOR (Cohen and Stackman, 2015). Our results, using 15 min delay between the trials, showed that MK-801 affected both hippocampal gamma oscillations and NOR, including the predominant effect in female animals. This suggests that the increased power of hippocampal gamma oscillations is associated with the impaired recognition memory.
While gamma oscillations play a key role in cognition, their malfunction was observed both in schizophrenia and depressive disorders. In schizophrenia patients, baseline resting-state and task-induced gamma oscillations are increased similar to gamma oscillations in animal models (Teale et al., 2008; Donkers et al., 2013; Hirano et al., 2015; Lemercier et al., 2017; Hasam-Henderson et al., 2018). On the contrary, steady-state gamma responses evoked by repetitive sensory, in most cases auditory stimuli are decreased in both patients and animals studies (Sivarao et al., 2013; Thuné et al., 2016; Choi et al., 2023). While the power of induced gamma oscillations correlated with cognitive performance (Johannesen et al., 2016; Perrottelli et al., 2022) most studies reported no significant associations between the steady state gamma response and cognition in schizophrenia patients (Light et al., 2006; Kirihara et al., 2012; Perrottelli et al., 2022). An explanation for the opposing change of gamma oscillation power could be that pathologically increased baseline gamma power preceding the trial might limit the ability of bottom-up sensory transmission evoked gamma activity to further increase. This might impede the encoding of new information leading to reduced trial accuracy (Johannesen et al., 2016). We modeled the disease with a 14-day long i.p. application of 0.2 mg/kg MK-801 to adult rats. In contrast to single dose NMDA antagonist applications modeling acute first episode schizophrenia (Kehrer et al., 2007; Sullivan et al., 2015; Lemercier et al., 2017) the longer application is supposed to simulate a chronic disease state in animals (McNally et al., 2013). To test the effect of MK-801 on the behavior we analyzed the locomotor activity, stereotypy and grooming of the of the animals. While hyperlocomotion and stereotypy are considered as surrogates for the positive symptoms of schizophrenia, reduced grooming models the negative signs (Feinstein and Kritzer, 2013). In our experimental setup, subchronic treatment with MK-801 increased stereotypy only on female rats, in line with previous studies showing more pronounced effects in this sex (Hönack and Löscher, 1993; Andiné et al., 1999; Devaud, 2003). Subchronic MK-801 treatment did not significantly suppressed grooming in females which is in agreement with earlier findings after acute application (Feinstein and Kritzer, 2013). Although a profound hyperlocomotor activity was observed after acute application of MK-801 in females (Feinstein and Kritzer, 2013), we did not observe a treatment effect after subchronic MK-801 application presumably because the induction of stereotyped behavior counteracted ambulatory activity (van den Buuse, 2010). The results suggest that subchronic application of MK-801 daily induced a behavioral phenotype in the treated rats resembling both positive and negative symptoms of schizophrenia.
Present study investigated for the first time the effect of subchronic MK-801 on ex vivo pharmacologically induced hippocampal gamma oscillations. While the effects of acute systemic application of MK-801 on hippocampal gamma oscillations was intensively investigated in animal studies both in vitro (Kehrer et al., 2007; Lemercier et al., 2017) and in vivo (e.g., Sullivan et al., 2015; Cui et al., 2022), a longer application was previously not tested in vitro in the hippocampus. In prefrontal cortex slices, five-day-long MK-801 application decreased the power of gamma oscillations (McNally et al., 2013). In vivo measurements from the hippocampus of rats showed no effects of a 21-day-long MK-801 treatment on the hippocampal gamma (Sullivan et al., 2015). We found that MK-801 increased the gamma power in hippocampal slices indicating that ex vivo induced gamma oscillations response to systemic MK-801 application similar to baseline resting-state and task-induced gamma oscillations measured in schizophrenia patients. Our results suggest that modeling schizophrenia with systemic application of an NMDA receptor antagonist and the subsequent registration of pharmacologically induced ex vivo gamma oscillations in the hippocampus reproduces electrophysiological traits found in patients and can used as a biomarker for further research and pharmacological testing.
NMDA receptor antagonists, on the other hand, are used in the treatment of depressive disorders. Recent studies described reduced resting-state pre-task gamma oscillations in patients (Pizzagalli et al., 2006; Wang et al., 2023), and emotional task induced and steady-state evoked gamma oscillations were also found to be impaired (Lee et al., 2010; Liu et al., 2014, 2023). Animal models of depression induced with chronic unpredictable mild stress showed decreased auditory steady-state gamma responses (He et al., 2023). In last years, gamma oscillations have been increasingly suggested as a novel biomarker for major depression (Fitzgerald and Watson, 2018; He et al., 2023; Liu et al., 2023). Our results indicate that a longer application of the NMDA receptor antagonist MK-801 significantly increases the power of gamma oscillations suggesting that pharmacological treatment with an NMDA antagonist might normalize the reduction of the power observed in both patients and animal models. These findings are in line with experiments showing that memantine, an NMDA receptor antagonist used in the treatment of Alzheimer’s disease, also increased the power of hippocampal and neocortical gamma oscillations (Guadagna et al., 2012; Ahnaou et al., 2014; Ma et al., 2015).
The mechanism behind the increased gamma power after NMDA receptor antagonism currently remains unknown, however, two main hypotheses are considered to play a role: the theory of direct inhibition of cortical pyramidal cells and the disinhibition theory (Miller et al., 2016). The direct inhibition hypothesis postulates that an antagonism of extrasynaptic NMDARs on pyramidal cells, normally activated by low-level ambient glutamate, induces activity-independent homeostatic plasticity leading to increased excitatory synaptic inputs onto these neurons and increased gamma oscillations (Miller et al., 2016; Petzi et al., 2023). The disinhibition theory suggests that antagonism or dysfunction of the NMDA receptors on INs decreases the inhibitory tone in the circuit resulting in a higher neuronal activity within the network (Molina et al., 2014; Miller et al., 2016; Johnston et al., 2024). This theory assumes that NMDA receptors are either predominantly expressed on cortical INs compared to pyramidal cells (Lisman et al., 2008; Povysheva and Johnson, 2016) or are functionally more conductive, because the most abundant IN type in the cortex, the PV INs, are more depolarized and their receptors are thus less blocked by Mg2+ (Grunze et al., 1996; Homayoun and Moghaddam, 2007). This would mean that antagonists might influence this cell type more effectively. Because of their reduced excitability by NMDA receptor antagonism, PV INs require more synchronized inputs resulting in more synchronous perisomatic inhibition and enhanced gamma oscillation power (Spencer, 2009; Jadi et al., 2016).
An important finding of our study is the sex specificity of the MK-801 effect on hippocampal gamma oscillations. Previous studies tested only male animals (Sullivan et al., 2015; Cui et al., 2022) or did not investigate sex effects (Kehrer et al., 2007; Lemercier et al., 2017). Our study is the first that investigated the effect of NMDA receptor antagonists in female and male animals on hippocampal gamma oscillations and shows that MK-801 had a significantly stronger network effect in female rats compared to males. These observations are in accordance with the sex-specific behavioral effects observed in this and previous studies (Hönack and Löscher, 1993; Andiné et al., 1999; Devaud, 2003; Feinstein and Kritzer, 2013). We have two possible explanations for the sex difference. First, the ovarian hormone estrogen in female animals inhibits the expression of cytochrome P450 2D6 (CYP2D6) (Wang et al., 2014; Konstandi et al., 2020), which is the predicted primary metabolizing enzyme of MK-801 (Banerjee et al., 2020). It can be proposed that the applied MK-801 reached higher levels in female animals. Second, estrogen increases the expression of NMDA receptors in the membrane of neurons (Weiland, 1992; El-Bakri et al., 2004; Ikeda et al., 2010) and enhances the NMDA receptor dependent synaptic currents and calcium transients on hippocampal neurons (Foy et al., 1999; Pozzo-Miller et al., 1999). Increased expression of NMDA receptors in the membranes might cause more intense effects of NMDA receptor antagonists as seen in our experiments. A recent study has shown that besides pyramidal cells also PV INs express aromatase (Hernández-Vivanco et al., 2022), the enzyme to produce estradiol (Do Rego et al., 2009). Under these circumstances, NMDA receptor antagonists might have a stronger direct inhibitory or disinhibition effect on the network as discussed above. These considerations might also explain why KA-induced gamma oscillations had a stronger sex-specificity. Since KA receptors seem to preferentially activate interneurons compared to muscarinic ACh receptors (believed to be predominantly expressed on principal cells), KA-induced gamma oscillations are postulated to be generated by a primary stimulation of interneurons (Bartos et al., 2007). The disinhibition theory postulates that interneurons express more functional NMDA receptors, which might be further increased by estrogen in female animals. Thus, NMDA receptor antagonists may disinhibit the interneuron-based KA-induced gamma oscillations in female animals more effectively. Preclinical studies have shown that female animals are more sensitive to treatment with ketamine compared to males and develop more severe side effects (Ponton et al., 2021). These results are not consistent with the existing few human data, where the treatment response after systemic acute low dose ketamine application seems to be similar in women and men (Coyle and Laws, 2015; Freeman et al., 2019; Ponton et al., 2021). The sex effect of ketamine, however, might depend on the dose applicated because higher (1 mg/kg) dose reduced the Hamilton Depression Rating Scale scores more in women than in men (Freeman et al., 2019). In contrast to the treatment response, the side effects show clear sex-specific differences (Liebe et al., 2017; Ponton et al., 2021) indicating that ketamine acts sex-specifically also in human. Our results suggest that the higher sensitivity in females is not specific to ketamine but seems to be a generic characteristic trait of NMDA receptor antagonists.
In conclusion, presented data demonstrate that longer systemic application of the highly selective, non-competitive, use-dependent NMDA receptor antagonist MK-801 increases the amplitude of hippocampal gamma oscillations. The effects are associated with behavioral alterations such as impaired recognition memory, increased stereotypy and decreased grooming, surrogate symptoms of the cognitive, positive and negative symptoms of schizophrenia, respectively. Both the electrophysiological and behavioral changes were predominantly observed in female animals suggesting a sex-specific effect of NMDA receptor antagonists. The results reproduce electrophysiological and behavioral traits found in patients and in other schizophrenia animal models and suggest that hippocampal gamma oscillations can be used as a biomarker for further research and pharmacological testing.
Data availability statement
The raw data supporting the conclusions of this article will be made available by the authors, without undue reservation.
Ethics statement
The animal study was approved by Landesamt für Gesundheit und Soziales, Berlin, Germany. The study was conducted in accordance with the local legislation and institutional requirements.
Author contributions
TN: Formal analysis, Investigation, Project administration, Visualization, Writing – original draft, Writing – review & editing. ZG: Conceptualization, Formal analysis, Methodology, Resources, Supervision, Visualization, Writing – original draft, Writing – review & editing.
Funding
The author(s) declare that no financial support was received for the research, authorship, and/or publication of this article.
Acknowledgments
The authors thank Sophia Wollschläger, Andrea Wilke, and Katrin Schulze for technical assistance.
Conflict of interest
The authors declare that the research was conducted in the absence of any commercial or financial relationships that could be construed as a potential conflict of interest.
Publisher’s note
All claims expressed in this article are solely those of the authors and do not necessarily represent those of their affiliated organizations, or those of the publisher, the editors and the reviewers. Any product that may be evaluated in this article, or claim that may be made by its manufacturer, is not guaranteed or endorsed by the publisher.
References
Adell, A. (2020). Brain NMDA receptors in schizophrenia and depression. Biomol. Ther. 10:947. doi: 10.3390/biom10060947
Ahnaou, A., Huysmans, H., Jacobs, T., and Drinkenburg, W. H. I. M. (2014). Cortical EEG oscillations and network connectivity as efficacy indices for assessing drugs with cognition enhancing potential. Neuropharmacology 86, 362–377. doi: 10.1016/j.neuropharm.2014.08.015
Andiné, P., Widermark, N., Axelsson, R., Nyberg, G., Olofsson, U., Mårtensson, E., et al. (1999). Characterization of MK-801-induced behavior as a putative rat model of psychosis. J. Pharmacol. Exp. Ther. 290, 1393–1408
Banerjee, P., Dunkel, M., Kemmler, E., and Preissner, R. (2020). SuperCYPsPred-a web server for the prediction of cytochrome activity. Nucleic Acids Res. 48, W580–W585. doi: 10.1093/nar/gkaa166
Bartos, M., Vida, I., and Jonas, P. (2007). Synaptic mechanisms of synchronized gamma oscillations in inhibitory interneuron networks. Nat. Rev. Neurosci. 8, 45–56. doi: 10.1038/nrn2044
Bosman, C. A., Lansink, C. S., and Pennartz, C. M. A. (2014). Functions of gamma-band synchronization in cognition: from single circuits to functional diversity across cortical and subcortical systems. Eur. J. Neurosci. 39, 1982–1999. doi: 10.1111/ejn.12606
Buzsáki, G., and Wang, X.-J. (2012). Mechanisms of gamma oscillations. Annu. Rev. Neurosci. 35, 203–225. doi: 10.1146/annurev-neuro-062111-150444
Carr, M. F., Karlsson, M. P., and Frank, L. M. (2012). Transient slow gamma synchrony underlies hippocampal memory replay. Neuron 75, 700–713. doi: 10.1016/j.neuron.2012.06.014
Choi, K.-M., Im, C.-H., Yang, C., Lee, H. S., Kim, S., and Lee, S.-H. (2023). Influence of inter-stimulus interval on 40-Hz auditory steady-state response in patients with schizophrenia. Schizophrenia 9:46. doi: 10.1038/s41537-023-00377-6
Cohen, S. J., and Stackman, R. W. Jr. (2015). Assessing rodent hippocampal involvement in the novel object recognition task. A review. Behav. Brain Res. 285, 105–117. doi: 10.1016/j.bbr.2014.08.002
Coyle, C. M., and Laws, K. R. (2015). The use of ketamine as an antidepressant: a systematic review and meta-analysis. Hum. Psychopharmacol. 30, 152–163. doi: 10.1002/hup.2475
Cui, K., Yu, Z., Xu, L., Jiang, W., Wang, L., Wang, X., et al. (2022). Behavioral features and disorganization of oscillatory activity in C57BL/6J mice after acute low dose MK-801 administration. Front. Neurosci. 16:1001869. doi: 10.3389/fnins.2022.1001869
Devaud, L. L. (2003). Effects of ethanol or rimcazole on dizocilpine maleate-induced behaviors in male and female rats. Alcohol 29, 69–81. doi: 10.1016/s0741-8329(02)00326-9
Do Rego, J. L., Seong, J. Y., Burel, D., Leprince, J., Luu-The, V., Tsutsui, K., et al. (2009). Neurosteroid biosynthesis: enzymatic pathways and neuroendocrine regulation by neurotransmitters and neuropeptides. Front. Neuroendocrinol. 30, 259–301. doi: 10.1016/j.yfrne.2009.05.006
Donkers, F. C. L., Englander, Z. A., Tiesinga, P. H. E., Cleary, K. M., Gu, H., and Belger, A. (2013). Reduced delta power and synchrony and increased gamma power during the P3 time window in schizophrenia. Schizophr. Res. 150, 266–268. doi: 10.1016/j.schres.2013.07.050
El-Bakri, N. K., Islam, A., Zhu, S., Elhassan, A., Mohammed, A., Winblad, B., et al. (2004). Effects of estrogen and progesterone treatment on rat hippocampal NMDA receptors: relationship to Morris water maze performance. J. Cell. Mol. Med. 8, 537–544. doi: 10.1111/j.1582-4934.2004.tb00478.x
Ennaceur, A. (2010). One-trial object recognition in rats and mice: methodological and theoretical issues. Behav. Brain Res. 215, 244–254. doi: 10.1016/j.bbr.2009.12.036
Faul, F., Erdfelder, E., Lang, A.-G., and Buchner, A. (2007). G*power 3: a flexible statistical power analysis program for the social, behavioral, and biomedical sciences. Behav. Res. Methods 39, 175–191. doi: 10.3758/bf03193146
Feinstein, I., and Kritzer, M. F. (2013). Acute NMDA receptor hypofunction induced by MK801 evokes sex-specific changes in behaviors observed in open field testing in adult male and proestrus female rats. Neuroscience 228, 200–214. doi: 10.1016/j.neuroscience.2012.10.026
Fisahn, A., Contractor, A., Traub, R. D., Buhl, E. H., Heinemann, S. F., and McBain, C. J. (2004). Distinct roles for the Kainate receptor subunits GluR5 and GluR6 in Kainate-induced hippocampal gamma oscillations. J. Neurosci. 24, 9658–9668. doi: 10.1523/JNEUROSCI.2973-04.2004
Fisahn, A., Pike, F. G., Buhl, E. H., and Paulsen, O. (1998). Cholinergic induction of network oscillations at 40 Hz in the hippocampus in vitro. Nature 394, 186–189. doi: 10.1038/28179
Fitzgerald, P. J., and Watson, B. O. (2018). Gamma oscillations as a biomarker for major depression: an emerging topic. Transl. Psychiatry 8:177. doi: 10.1038/s41398-018-0239-y
Foy, M. R., Xu, J., Xie, X., Brinton, R. D., Thompson, R. F., and Berger, T. W. (1999). 17beta-estradiol enhances NMDA receptor-mediated EPSPs and long-term potentiation. J. Neurophysiol. 81, 925–929. doi: 10.1152/jn.1999.81.2.925
Freeman, M. P., Papakostas, G. I., Hoeppner, B., Mazzone, E., Judge, H., Cusin, C., et al. (2019). Sex differences in response to ketamine as a rapidly acting intervention for treatment resistant depression. J. Psychiatr. Res. 110, 166–171. doi: 10.1016/j.jpsychires.2019.01.010
Fries, P. (2009). Neuronal gamma-band synchronization as a fundamental process in cortical computation. Annu. Rev. Neurosci. 32, 209–224. doi: 10.1146/annurev.neuro.051508.135603
Grunze, H. C., Rainnie, D. G., Hasselmo, M. E., Barkai, E., Hearn, E. F., McCarley, R. W., et al. (1996). NMDA-dependent modulation of CA1 local circuit inhibition. J. Neurosci. 16, 2034–2043. doi: 10.1523/JNEUROSCI.16-06-02034.1996
Guadagna, S., Bundgaard, C., Hovelsø, N., Volbracht, C., Francis, P. T., Egebjerg, J., et al. (2012). Memantine potentiates hippocampal θ oscillations at a therapeutic dose in anesthetized mice: a mechanistic link to its cognitive-enhancing properties. Neuropharmacology 62, 2208–2218. doi: 10.1016/j.neuropharm.2012.01.014
Guneykaya, D., Ugursu, B., Logiacco, F., Popp, O., Feiks, M. A., Meyer, N., et al. (2023). Sex-specific microglia state in the Neuroligin-4 knock-out mouse model of autism spectrum disorder. Brain Behav. Immun. 111, 61–75. doi: 10.1016/j.bbi.2023.03.023
Hájos, N., and Paulsen, O. (2009). Network mechanisms of gamma oscillations in the CA3 region of the hippocampus. Neural Netw. 22, 1113–1119. doi: 10.1016/j.neunet.2009.07.024
Hasam-Henderson, L. A., Gotti, G. C., Mishto, M., Klisch, C., Gerevich, Z., Geiger, J. R. P., et al. (2018). NMDA-receptor inhibition and oxidative stress during hippocampal maturation differentially alter parvalbumin expression and gamma-band activity. Sci. Rep. 8:9545. doi: 10.1038/s41598-018-27830-2
He, Y., Guo, W., Ren, Z., Liang, J., Liu, S., and Ming, D. (2023). Gamma auditory steady-state response as a promising electrophysiological biomarker for depression: an in vivo study with chronic unpredictable mild stress (CUS)-induced rats. Cereb. Cortex 33, 7741–7753. doi: 10.1093/cercor/bhad076
Hernández-Vivanco, A., Cano-Adamuz, N., Sánchez-Aguilera, A., González-Alonso, A., Rodríguez-Fernández, A., Azcoitia, Í., et al. (2022). Sex-specific regulation of inhibition and network activity by local aromatase in the mouse hippocampus. Nat. Commun. 13:3913. doi: 10.1038/s41467-022-31635-3
Hirano, Y., Oribe, N., Kanba, S., Onitsuka, T., Nestor, P. G., and Spencer, K. M. (2015). Spontaneous gamma activity in schizophrenia. JAMA Psychiatry 72, 813–821. doi: 10.1001/jamapsychiatry.2014.2642
Homayoun, H., and Moghaddam, B. (2007). NMDA receptor hypofunction produces opposite effects on prefrontal cortex interneurons and pyramidal neurons. J. Neurosci. 27, 11496–11500. doi: 10.1523/JNEUROSCI.2213-07.2007
Hönack, D., and Löscher, W. (1993). Sex differences in NMDA receptor mediated responses in rats. Brain Res. 620, 167–170. doi: 10.1016/0006-8993(93)90287-w
Honey, R. C., Watt, A., and Good, M. (1998). Hippocampal lesions disrupt an associative mismatch process. J. Neurosci. 18, 2226–2230. doi: 10.1523/JNEUROSCI.18-06-02226.1998
Ikeda, K., Fukushima, T., Ogura, H., Tsukui, T., Mishina, M., Muramatsu, M., et al. (2010). Estrogen regulates the expression of N-methyl-D-aspartate (NMDA) receptor subunit epsilon 4 (Grin2d), that is essential for the normal sexual behavior in female mice. FEBS Lett. 584, 806–810. doi: 10.1016/j.febslet.2009.12.054
Jadi, M. P., Behrens, M. M., and Sejnowski, T. J. (2016). Abnormal gamma oscillations in N-methyl-D-aspartate receptor hypofunction models of schizophrenia. Biol. Psychiatry 79, 716–726. doi: 10.1016/j.biopsych.2015.07.005
Johannesen, J. K., Bi, J., Jiang, R., Kenney, J. G., and Chen, C.-M. A. (2016). Machine learning identification of EEG features predicting working memory performance in schizophrenia and healthy adults. Neuropsychiatr. Electrophysiol. 2:3. doi: 10.1186/s40810-016-0017-0
Johnson, A., Varberg, Z., Benhardus, J., Maahs, A., and Schrater, P. (2012). The hippocampus and exploration: dynamically evolving behavior and neural representations. Front. Hum. Neurosci. 6:216. doi: 10.3389/fnhum.2012.00216
Johnston, J. N., Kadriu, B., Kraus, C., Henter, I. D., and Zarate, C. A. (2024). Ketamine in neuropsychiatric disorders: an update. Neuropsychopharmacology 49, 23–40. doi: 10.1038/s41386-023-01632-1
Keers, R., and Aitchison, K. J. (2010). Gender differences in antidepressant drug response. Int. Rev. Psychiatry 22, 485–500. doi: 10.3109/09540261.2010.496448
Kehrer, C., Dugladze, T., Maziashvili, N., Wójtowicz, A., Schmitz, D., Heinemann, U., et al. (2007). Increased inhibitory input to CA1 pyramidal cells alters hippocampal gamma frequency oscillations in the MK-801 model of acute psychosis. Neurobiol. Dis. 25, 545–552. doi: 10.1016/j.nbd.2006.10.015
Kirihara, K., Rissling, A. J., Swerdlow, N. R., Braff, D. L., and Light, G. A. (2012). Hierarchical organization of gamma and theta oscillatory dynamics in schizophrenia. Biol. Psychiatry 71, 873–880. doi: 10.1016/j.biopsych.2012.01.016
Klemz, A., Kreis, P., Eickholt, B. J., and Gerevich, Z. (2021). The actin binding protein drebrin helps to protect against the development of seizure-like events in the entorhinal cortex. Sci. Rep. 11:8662. doi: 10.1038/s41598-021-87967-5
Klemz, A., Wildner, F., Tütüncü, E., and Gerevich, Z. (2022). Regulation of hippocampal gamma oscillations by modulation of intrinsic neuronal excitability. Front. Neural Circ. 15:778022. doi: 10.3389/fncir.2021.778022
Konstandi, M., Andriopoulou, C. E., Cheng, J., and Gonzalez, F. J. (2020). Sex steroid hormones differentially regulate CYP2D in female wild-type and CYP2D6-humanized mice. J. Endocrinol. 245, 301–314. doi: 10.1530/JOE-19-0561
Lee, P.-S., Chen, Y.-S., Hsieh, J.-C., Su, T.-P., and Chen, L.-F. (2010). Distinct neuronal oscillatory responses between patients with bipolar and unipolar disorders: a magnetoencephalographic study. J. Affect. Disord. 123, 270–275. doi: 10.1016/j.jad.2009.08.020
Lee, K.-H., Williams, L. M., Breakspear, M., and Gordon, E. (2003). Synchronous gamma activity: a review and contribution to an integrative neuroscience model of schizophrenia. Brain Res. Rev. 41, 57–78. doi: 10.1016/S0165-0173(02)00220-5
Lee, G., and Zhou, Y. (2019). NMDAR hypofunction animal models of schizophrenia. Front. Mol. Neurosci. 12:185. doi: 10.3389/fnmol.2019.00185
Lemercier, C. E., Holman, C., and Gerevich, Z. (2017). Aberrant alpha and gamma oscillations ex vivo after single application of the NMDA receptor antagonist MK-801. Schizophr. Res. 188, 118–124. doi: 10.1016/j.schres.2017.01.017
Lemercier, C. E., Schulz, S. B., Heidmann, K. E., Kovács, R., and Gerevich, Z. (2016). Dopamine D3 receptors inhibit hippocampal gamma oscillations by disturbing CA3 pyramidal cell firing synchrony. Front. Pharmacol. 6:297. doi: 10.3389/fphar.2015.00297
Lewis, D. A., Hashimoto, T., and Volk, D. W. (2005). Cortical inhibitory neurons and schizophrenia. Nat. Rev. Neurosci. 6, 312–324. doi: 10.1038/nrn1648
Liebe, T., Li, S., Lord, A., Colic, L., Krause, A. L., Batra, A., et al. (2017). Factors influencing the cardiovascular response to subanesthetic ketamine: a randomized, placebo-controlled trial. Int. J. Neuropsychopharmacol. 20, 909–918. doi: 10.1093/ijnp/pyx055
Light, G. A., Hsu, J. L., Hsieh, M. H., Meyer-Gomes, K., Sprock, J., Swerdlow, N. R., et al. (2006). Gamma band oscillations reveal neural network cortical coherence dysfunction in schizophrenia patients. Biol. Psychiatry 60, 1231–1240. doi: 10.1016/j.biopsych.2006.03.055
Lisman, J. E., Coyle, J. T., Green, R. W., Javitt, D. C., Benes, F. M., Heckers, S., et al. (2008). Circuit-based framework for understanding neurotransmitter and risk gene interactions in schizophrenia. Trends Neurosci. 31, 234–242. doi: 10.1016/j.tins.2008.02.005
Liu, T. Y., Chen, Y. S., Su, T. P., Hsieh, J. C., and Chen, L. F. (2014). Abnormal early gamma responses to emotional faces differentiate unipolar from bipolar disorder patients. Biomed. Res. Int. 2014:e906104. doi: 10.1155/2014/906104
Liu, S., Liu, X., Chen, S., Su, F., Zhang, B., Ke, Y., et al. (2023). Neurophysiological markers of depression detection and severity prediction in first-episode major depressive disorder. J. Affect. Disord. 331, 8–16. doi: 10.1016/j.jad.2023.03.038
Lu, C. B., Jefferys, J. G. R., Toescu, E. C., and Vreugdenhil, M. (2011). In vitro hippocampal gamma oscillation power as an index of in vivo CA3 gamma oscillation strength and spatial reference memory. Neurobiol. Learn. Mem. 95, 221–230. doi: 10.1016/j.nlm.2010.11.008
Ma, J., Mufti, A., and Stan Leung, L. (2015). Effects of memantine on hippocampal long-term potentiation, gamma activity, and sensorimotor gating in freely moving rats. Neurobiol. Aging 36, 2544–2554. doi: 10.1016/j.neurobiolaging.2015.05.017
Mathiasen, J. R., and DiCamillo, A. (2010). Novel object recognition in the rat: a facile assay for cognitive function. Curr. Protoc. Pharmacol. Chapter 5:Unit 5.59. doi: 10.1002/0471141755.ph0559s49
McNally, J. M., McCarley, R. W., and Brown, R. E. (2013). Chronic ketamine reduces the peak frequency of gamma oscillations in mouse prefrontal cortex ex vivo. Front. Psych. 4:106. doi: 10.3389/fpsyt.2013.00106
Meier, M. A., Lemercier, C. E., Kulisch, C., Kiss, B., Lendvai, B., Adham, N., et al. (2020). The novel antipsychotic cariprazine stabilizes gamma oscillations in rat hippocampal slices. Br. J. Pharmacol. 177, 1622–1634. doi: 10.1111/bph.14923
Mendez, P., Pazienti, A., Szabo, G., and Bacci, A. (2012). Direct alteration of a specific inhibitory circuit of the Hippocampus by antidepressants. J. Neurosci. 32, 16616–16628. doi: 10.1523/JNEUROSCI.1720-12.2012
Miller, O. H., Moran, J. T., and Hall, B. J. (2016). Two cellular hypotheses explaining the initiation of ketamine’s antidepressant actions: direct inhibition and disinhibition. Neuropharmacology 100, 17–26. doi: 10.1016/j.neuropharm.2015.07.028
Molina, L. A., Skelin, I., and Gruber, A. J. (2014). Acute NMDA receptor antagonism disrupts synchronization of action potential firing in rat prefrontal cortex. PLoS One 9:e85842. doi: 10.1371/journal.pone.0085842
Otte, C., Gold, S. M., Penninx, B. W., Pariante, C. M., Etkin, A., Fava, M., et al. (2016). Major depressive disorder. Nat. Rev. Dis. Prim. 2:16065. doi: 10.1038/nrdp.2016.65
Perrottelli, A., Giordano, G. M., Brando, F., Giuliani, L., Pezzella, P., Mucci, A., et al. (2022). Unveiling the associations between EEG indices and cognitive deficits in schizophrenia-Spectrum disorders: a systematic review. Diagnostics 12:2193. doi: 10.3390/diagnostics12092193
Petzi, M., Singh, S., Trappenberg, T., and Nunes, A. (2023). Mechanisms of sustained increases in γ power post-ketamine in a computational model of the hippocampal CA3: implications for Ketamine’s antidepressant mechanism of action. Brain Sci. 13:1562. doi: 10.3390/brainsci13111562
Pizzagalli, D. A., Peccoralo, L. A., Davidson, R. J., and Cohen, J. D. (2006). Resting anterior cingulate activity and abnormal responses to errors in subjects with elevated depressive symptoms: a 128-channel EEG study. Hum. Brain Mapp. 27, 185–201. doi: 10.1002/hbm.20172
Ponton, E., Turecki, G., and Nagy, C. (2021). Sex differences in the behavioral, molecular, and structural effects of ketamine treatment in depression. Int. J. Neuropsychopharmacol. 25, 75–84. doi: 10.1093/ijnp/pyab082
Povysheva, N. V., and Johnson, J. W. (2016). Effects of memantine on the excitation-inhibition balance in prefrontal cortex. Neurobiol. Dis. 96, 75–83. doi: 10.1016/j.nbd.2016.08.006
Pozzo-Miller, L. D., Inoue, T., and Murphy, D. D. (1999). Estradiol increases spine density and NMDA-dependent Ca2+ transients in spines of CA1 pyramidal neurons from hippocampal slices. J. Neurophysiol. 81, 1404–1411. doi: 10.1152/jn.1999.81.3.1404
Rush, A. J., Trivedi, M. H., Wisniewski, S. R., Nierenberg, A. A., Stewart, J. W., Warden, D., et al. (2006). Acute and longer-term outcomes in depressed outpatients requiring one or several treatment steps: a STAR*D report. Am. J. Psychiatry 163, 1905–1917. doi: 10.1176/ajp.2006.163.11.1905
Schulz, S. B., Heidmann, K. E., Mike, A., Klaft, Z.-J., Heinemann, U., and Gerevich, Z. (2012a). First and second generation antipsychotics influence hippocampal gamma oscillations by interactions with 5-HT 3 and D 3 receptors: antipsychotics alter gamma oscillations. Br. J. Pharmacol. 167, 1480–1491. doi: 10.1111/j.1476-5381.2012.02107.x
Schulz, S. B., Klaft, Z.-J., Rösler, A. R., Heinemann, U., and Gerevich, Z. (2012b). Purinergic P2X, P2Y and adenosine receptors differentially modulate hippocampal gamma oscillations. Neuropharmacology 62, 914–924. doi: 10.1016/j.neuropharm.2011.09.024
Sivarao, D. V., Frenkel, M., Chen, P., Healy, F. L., Lodge, N. J., and Zaczek, R. (2013). MK-801 disrupts and nicotine augments 40 Hz auditory steady state responses in the auditory cortex of the urethane-anesthetized rat. Neuropharmacology 73, 1–9. doi: 10.1016/j.neuropharm.2013.05.006
Sohal, V. S. (2022). Transforming discoveries about cortical microcircuits and gamma oscillations into new treatments for cognitive deficits in schizophrenia. AJP 179, 267–276. doi: 10.1176/appi.ajp.20220147
Speers, L. J., and Bilkey, D. K. (2021). Disorganization of oscillatory activity in animal models of schizophrenia. Front. Neural Circ. 15:741767. doi: 10.3389/fncir.2021.741767
Spellman, T., and Liston, C. (2020). Toward circuit mechanisms of pathophysiology in depression. AJP 177, 381–390. doi: 10.1176/appi.ajp.2020.20030280
Spencer, K. (2009). The functional consequences of cortical circuit abnormalities on gamma oscillations in schizophrenia: insights from computational modeling. Front. Hum. Neurosci. 3:33. doi: 10.3389/neuro.09.033.2009
Sullivan, E. M., Timi, P., Hong, L. E., and O’Donnell, P. (2015). Reverse translation of clinical electrophysiological biomarkers in behaving rodents under acute and chronic NMDA receptor antagonism. Neuropsychopharmacology 40, 719–727. doi: 10.1038/npp.2014.228
Szasz, B. K., Mike, A., Karoly, R., Gerevich, Z., Illes, P., Vizi, E. S., et al. (2007). Direct inhibitory effect of fluoxetine on N-methyl-D-aspartate receptors in the central nervous system. Biol. Psychiatry 62, 1303–1309. doi: 10.1016/j.biopsych.2007.04.014
Teale, P., Collins, D., Maharajh, K., Rojas, D. C., Kronberg, E., and Reite, M. (2008). Cortical source estimates of gamma band amplitude and phase are different in schizophrenia. Neuroimage 42, 1481–1489. doi: 10.1016/j.neuroimage.2008.06.020
Thuné, H., Recasens, M., and Uhlhaas, P. J. (2016). The 40-Hz auditory steady-state response in patients with schizophrenia: a meta-analysis. JAMA Psychiatry 73:1145. doi: 10.1001/jamapsychiatry.2016.2619
Trimper, J. B., Galloway, C. R., Jones, A. C., Mandi, K., and Manns, J. R. (2017). Gamma oscillations in rat hippocampal subregions dentate gyrus, CA3, CA1, and subiculum underlie associative memory encoding. Cell Rep. 21, 2419–2432. doi: 10.1016/j.celrep.2017.10.123
Trimper, J. B., Stefanescu, R. A., and Manns, J. R. (2014). Recognition memory and theta–gamma interactions in the hippocampus. Hippocampus 24, 341–353. doi: 10.1002/hipo.22228
Trubetskoy, V., Pardiñas, A. F., Qi, T., Panagiotaropoulou, G., Awasthi, S., Bigdeli, T. B., et al. (2022). Mapping genomic loci implicates genes and synaptic biology in schizophrenia. Nature 604, 502–508. doi: 10.1038/s41586-022-04434-5
Trullas, R., and Skolnick, P. (1990). Functional antagonists at the NMDA receptor complex exhibit antidepressant actions. Eur. J. Pharmacol. 185, 1–10. doi: 10.1016/0014-2999(90)90204-J
van den Buuse, M. (2010). Modeling the positive symptoms of schizophrenia in genetically modified mice: pharmacology and methodology aspects. Schizophr. Bull. 36, 246–270. doi: 10.1093/schbul/sbp132
Vos, T., Lim, S. S., Abbafati, C., Abbas, K. M., Abbasi, M., Abbasifard, M., et al. (2020). Global burden of 369 diseases and injuries in 204 countries and territories, 1990–2019: a systematic analysis for the global burden of disease study 2019. Lancet 396, 1204–1222. doi: 10.1016/S0140-6736(20)30925-9
Wang, X., Li, J., Dong, G., and Yue, J. (2014). The endogenous substrates of brain CYP2D. Eur. J. Pharmacol. 724, 211–218. doi: 10.1016/j.ejphar.2013.12.025
Wang, H., Tian, S., Yan, R., Tang, H., Shi, J., Zhu, R., et al. (2023). Convergent and divergent cognitive impairment of unipolar and bipolar depression: a magnetoencephalography resting-state study. J. Affect. Disord. 321, 8–15. doi: 10.1016/j.jad.2022.09.126
Weiland, N. G. (1992). Estradiol selectively regulates agonist binding sites on the N-methyl-D-aspartate receptor complex in the CA1 region of the hippocampus. Endocrinology 131, 662–668. doi: 10.1210/endo.131.2.1353442
Wildner, F., Neuhäusel, T. S., Klemz, A., Kovács, R., Ulmann, L., Geiger, J. R. P., et al. (2024). Extracellular ATP inhibits excitatory synaptic input on parvalbumin positive interneurons and attenuates gamma oscillations via P2X4 receptors. Br. J. Pharm. 181, 1635–1653. doi: 10.1111/bph.16298
Keywords: electroencephalography (EEG), gamma oscillations, sex-specific, cognition, electrophysiology, ketamine
Citation: Neuhäusel TS and Gerevich Z (2024) Sex-specific effects of subchronic NMDA receptor antagonist MK-801 treatment on hippocampal gamma oscillations. Front. Neurosci. 18:1425323. doi: 10.3389/fnins.2024.1425323
Edited by:
Chuanliang Han, The Chinese University of Hong Kong, ChinaReviewed by:
Nagaraja Sethuraman Balakathiresan, National Institute on Alcohol Abuse and Alcoholism (NIH), United StatesMengyu Tu, Johns Hopkins University, United States
Dennis Kätzel, University of Ulm, Germany
Copyright © 2024 Neuhäusel and Gerevich. This is an open-access article distributed under the terms of the Creative Commons Attribution License (CC BY). The use, distribution or reproduction in other forums is permitted, provided the original author(s) and the copyright owner(s) are credited and that the original publication in this journal is cited, in accordance with accepted academic practice. No use, distribution or reproduction is permitted which does not comply with these terms.
*Correspondence: Zoltan Gerevich, zoltan.gerevich@charite.de