- 1Department of Neurology, Union Hospital, Tongji Medical College, Huazhong University of Science and Technology, Wuhan, China
- 2Department of Pharmacology, School of Basic Medicine, Tongji Medical College, Huazhong University of Science and Technology, Wuhan, China
- 3Department of General Medicine, Binzhou Medical University Hospital, Binzhou, China
Alzheimer’s disease (AD) stands as a formidable neurodegenerative ailment and a prominent contributor to dementia. The scarcity of available therapies for AD accentuates the exigency for innovative treatment modalities. Psilocybin, a psychoactive alkaloid intrinsic to hallucinogenic mushrooms, has garnered attention within the neuropsychiatric realm due to its established safety and efficacy in treating depression. Nonetheless, its potential as a therapeutic avenue for AD remains largely uncharted. This comprehensive review endeavors to encapsulate the pharmacological effects of psilocybin while elucidating the existing evidence concerning its potential mechanisms contributing to a positive impact on AD. Specifically, the active metabolite of psilocybin, psilocin, elicits its effects through the modulation of the 5-hydroxytryptamine 2A receptor (5-HT2A receptor). This modulation causes heightened neural plasticity, diminished inflammation, and improvements in cognitive functions such as creativity, cognitive flexibility, and emotional facial recognition. Noteworthy is psilocybin’s promising role in mitigating anxiety and depression symptoms in AD patients. Acknowledging the attendant adverse reactions, we proffer strategies aimed at tempering or mitigating its hallucinogenic effects. Moreover, we broach the ethical and legal dimensions inherent in psilocybin’s exploration for AD treatment. By traversing these avenues, We propose therapeutic potential of psilocybin in the nuanced management of Alzheimer’s disease.
1 Introduction
Alzheimer’s disease (AD) is a progressive neurodegenerative disease that is the leading cause of dementia in the elderly population (Anonymous, 2021). It is characterized by the deposition of amyloid-beta (Aβ) plaques, tau neurofibrillary tangles, and neuroinflammation (Scheltens et al., 2021). The prevalence of dementia is expected to rise as the global population grows and ages, with projections estimating a significant increase in the number of cases (Anonymous, 2022b). In 2019, the total cost of healthcare, long-term care, and hospice services for individuals aged 65 years and older with dementia in the United States was estimated at $2.2billion, so AD imposes a substantial burden on individuals, families, society, and the economy (Anonymous, 2022a). The U.S. Food and Drug Administration (FDA) has approved seven drugs for the treatment of AD, including rivastigmine, donepezil, galantamine, memantine, memantine combined with donepezil, aducanumab and lecanemab. Among them, aducanumab and lecanemab are part of the first wave of Aβ-targeting drugs for AD and are solely recommended for early-stage AD (Anonymous, 2021, 2022b; van Dyck et al., 2023). It is important to highlight that these medications are linked to a significant incidence of adverse reactions, including amyloid-related imaging abnormalities, brain edema, and headaches (Salloway et al., 2022; van Dyck et al., 2023). Furthermore, they impose a considerable financial burden due to their high cost (Glymour et al., 2022; Burke et al., 2023). Given the significant burden of AD, it is crucial to continue developing new therapies to effectively treat the disease.
In recent years, research on the use of psychedelics in the field of mental and nervous systems has garnered widespread attention and enthusiasm (Roth and Gumpper, 2023; Siegel et al., 2023). Among them, psilocybin, a naturally occurring hallucinogenic compound found in certain species of mushrooms, particularly those belonging to the genus Psilocybe, has garnered increasing attention (Lenz et al., 2020). Psilocybin has shown promise as a treatment for major depressive disorder (MDD), and in 2019, psilocybin therapy was designated as a breakthrough therapy by the FDA (Donovan et al., 2021; Raison et al., 2023). In the Phase 2 double-blind trial conducted by COMPASS, administering a dosage of 25 mg of psilocybin for the treatment of treatment-resistant depression (TRD) demonstrated a significant reduction in the Montgomery-Åsberg Depression Rating Scale scores of the subjects (Goodwin et al., 2022). Furthermore, several small-scale clinical trials have indicated the potential benefits of psilocybin for a range of conditions, such as anxiety disorders (Griffiths et al., 2016; Ross et al., 2016), obsessive-compulsive disorder (Moreno et al., 2006; Ching et al., 2023), migraine (Schindler et al., 2021), cluster headache (Schindler et al., 2022), addictive behaviors (Bogenschutz et al., 2022; Slomski, 2022), body dysmorphic disorder (Schneier et al., 2023), as well as anorexia nervosa (Peck et al., 2023).
Although the use of psilocybin as a treatment for AD has not been sufficiently studied, there is still a potential for psilocybin to be explored as a new treatment option in the future. The density of 5-HT2A receptor is reduced in AD, and this reduction is associated with a decline in cognitive function (Versijpt et al., 2003). Studies on AD rats induced with streptozotocin have shown that the administration of 5-HT1A and 5-HT2A receptor agonists has significant neuroprotective effects on hippocampal neurons through anti-apoptotic and anti-inflammatory pathways (Shahidi et al., 2019). Psilocybin, known for its mechanisms in promoting neuroplasticity, anti-inflammation, and improving functional connectivity of brain networks in the treatment of depression and other diseases, may hold potential benefits for patients with AD (Nkadimeng et al., 2021; Daws et al., 2022; Du et al., 2023). Additionally, depression and anxiety are common mental symptoms of AD and can accelerate cognitive decline in AD patients (Gallagher et al., 2018; Ma, 2020; Agüera-Ortiz et al., 2021). Psilocybin may potentially delay the progression of AD by alleviating depression and anxiety symptoms.
While recent reviews have broached the potential of hallucinogens in treating neurodegenerative diseases (Vann Jones and O’Kelly, 2020; Garcia-Romeu et al., 2022; Kozlowska et al., 2022; Pilozzi et al., 2023), this review uniquely focuses on psilocybin and its application in AD. Introducing the latest research findings and clinical trials, this review provides an in-depth exploration of the pharmacology of psilocybin and the potential mechanisms underlying its therapeutic effects in AD (Figure 1). This review shines a spotlight on its thorough amalgamation of the adverse effects of psilocybin and the tactics employed to alleviate its hallucinogenic qualities, ultimately facilitating the process of clinical translation. Lastly, as the landscape of psilocybin therapy evolves, attention is drawn to the concurrent emergence of legal and ethical considerations.
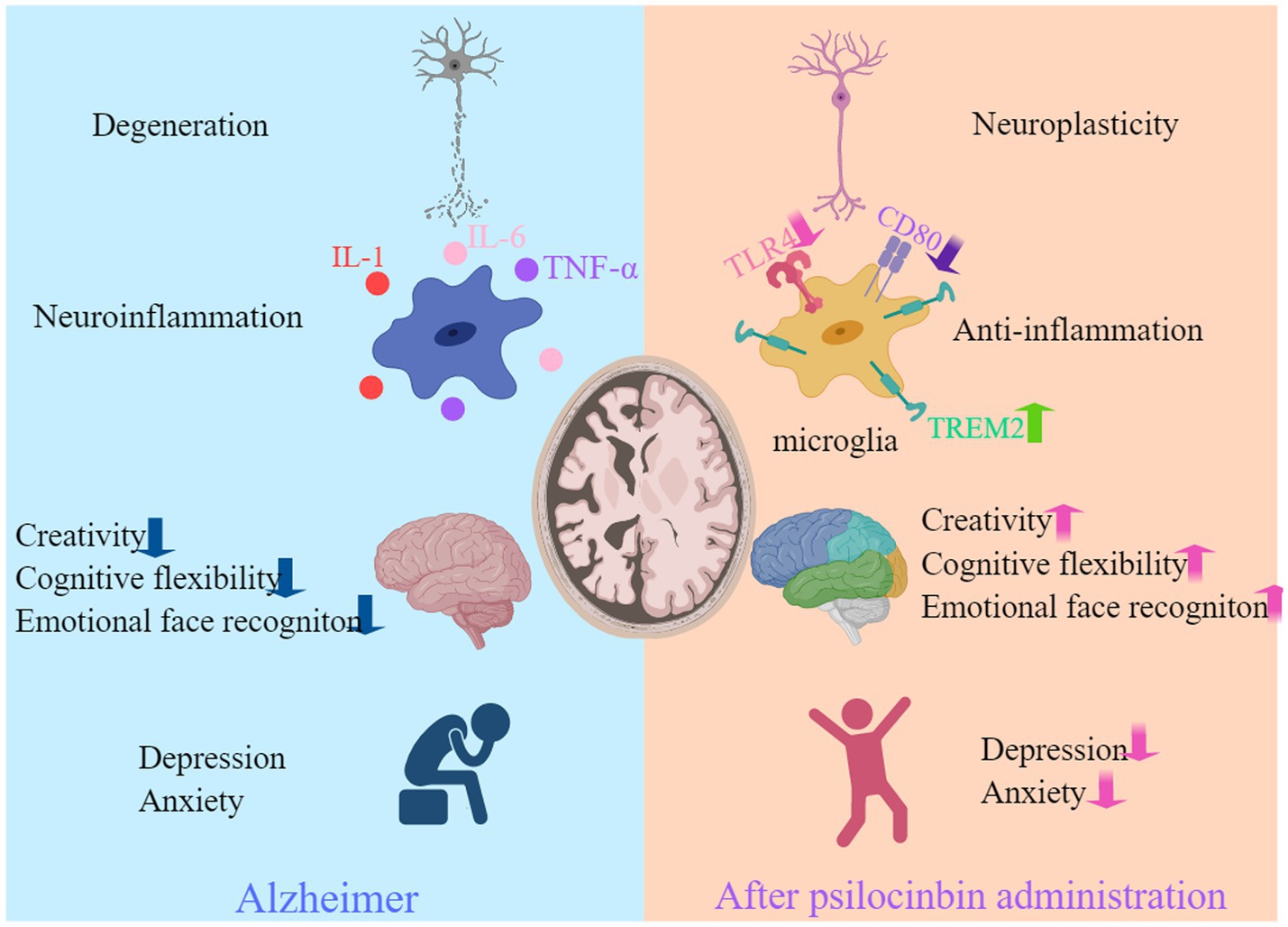
Figure 1. Psilocybin may potentially benefit individuals with AD. Psilocybin has shown benefits for AD in terms of enhancing neuroplasticity, reducing inflammation, neurocognitive improvement, and alleviating anxiety and depression. Created with MedPeer (www.medpeer.cn).
2 Pharmacological effects of psilocybin
2.1 Metabolic processes
The metabolic pathways of psilocybin have been confirmed in healthy volunteers. After oral administration, psilocybin undergoes dephosphorylation by alkaline phosphatase in the stomach and intestine to form psilocin, which can then cross the blood–brain barrier and exert its hallucinogenic effects and related pharmacological effects (Horita and Weber, 1961a,b, 1962). Psilocin has two major metabolic pathways, phase I and phase II. The phase II metabolic pathway is the primary pathway, accounting for ≥80% of the total metabolism (Manevski et al., 2010). In the phase I metabolic pathway, psilocin undergoes oxidative reactions via monoamine oxidase (MAO) in the liver, resulting in the formation of 4-hydroxyindole-3-acetaldehyde, which further undergoes oxidation to form 4-hydroxy-indole-3-acetic acid, or reduction to form 4-hydroxytryptophole (Kalberer et al., 1962; Lindenblatt et al., 1998; Kolaczynska et al., 2021). In the phase II metabolic pathway, psilocin undergoes enzymatic catalysis by UDP-glucuronosyltransferases (UGT) 1A10 in the intestine and UGT1A9 in the liver to form O-glucuronide conjugates, which are then excreted in the urine (Hasler et al., 2002; Kamata et al., 2006; Manevski et al., 2010; Figure 2). Psilocin reaches its peak plasma concentration approximately 2–3 h after administration and has a half-life of about 3 h (standard deviation 1.1) (Brown et al., 2017; Becker et al., 2022).
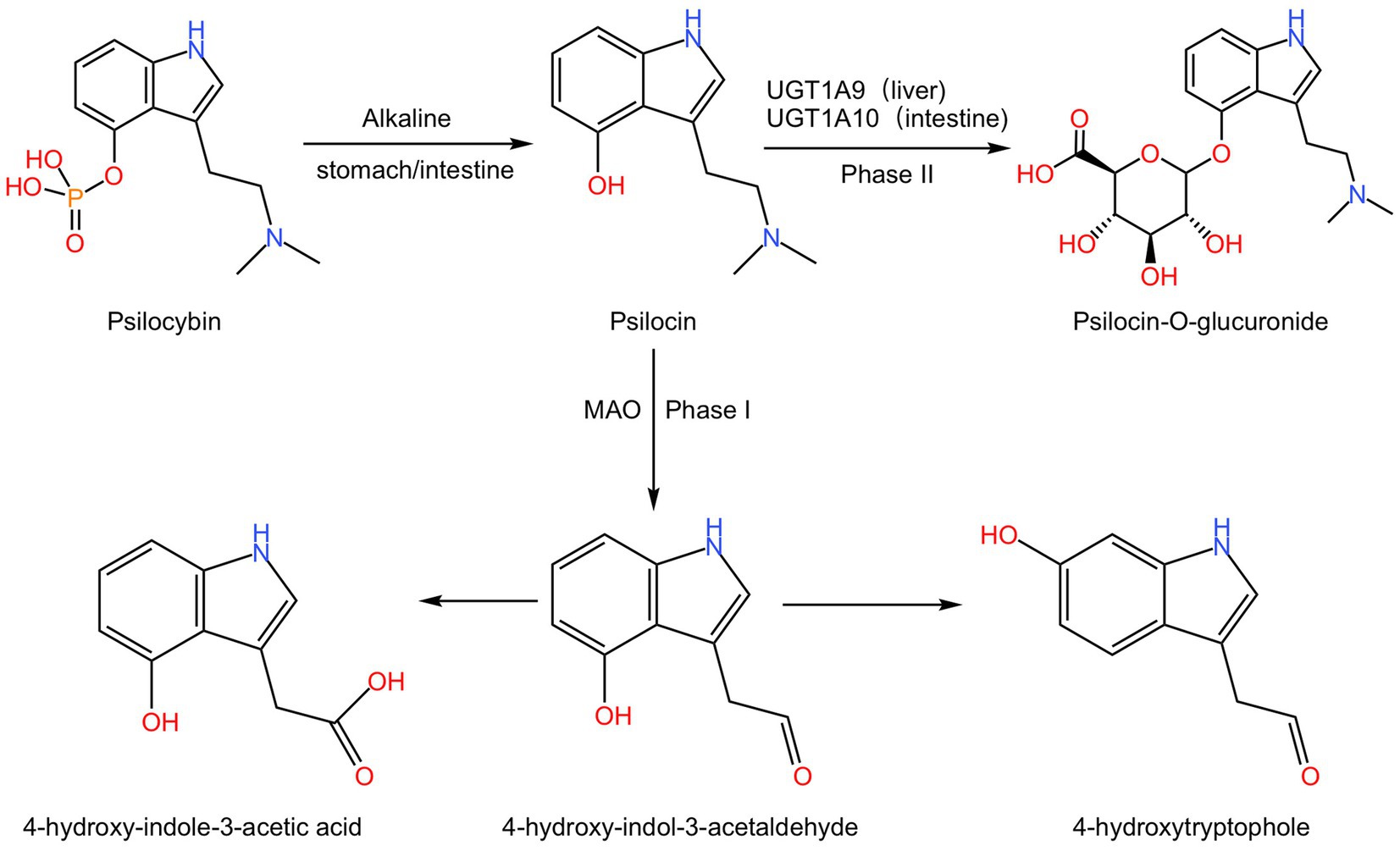
Figure 2. The metabolism process of psilocybin. Psilocybin undergoes dephosphorylation under the alkaline conditions of the stomach or intestine to form psilocin. In phase I, psilocin undergoes MAO action to generate 4-hydroxyindole-3-acetaldehyde, which further oxidizes to produce 4-hydroxy-indole-3-acetic acid or reduces to 4-hydroxytryptophole. And in phase II, psilocin is converted to psilocin-O-glucuronide by UGT1A9 in the liver or UGT1A10 in the intestine.
The majority of clinical research has utilized oral administration, resulting in limited data on intravenous metabolism. After intravenous administration of 1 mg in healthy volunteers, a mean psilocin maximum plasma concentration of 12.9 ± 5.6 ng/mL plasma was observed 1.9 ± 1.0 min after intravenous injection. 4-hydroxyindole-3-acetic acid was not detected following the intravenous psilocin administration (Hasler et al., 1997).
2.2 5-HT receptors
The mechanism of action of psilocin remains intricate and not fully elucidated. Psilocin engages with a myriad of 5-HT receptors, encompassing 5-HT1A receptor, 5-HT1B receptor, 5-HT1D receptor, 5-HT2A receptor, 5-HT2B receptor, 5-HT2C receptor, 5-HT5 receptor, 5-HT6 receptor, and 5-HT7 receptor. This information is documented in the Psychoactive Drug Screening Program database.1
Of these, the 5-HT2A receptor stands out as the principal mediator of psilocin’s hallucinogenic effects. The 5-HT2A receptor antagonist ketanserin has proven efficacy in inhibiting psilocin-induced perceptual distortions in humans and the head twitching reaction in mice, a behavioral characteristic strongly associated with hallucinations (Vollenweider et al., 1998; Madsen et al., 2019; Hesselgrave et al., 2021).
Beyond the 5-HT2A receptor, the 5-HT1A receptor, either directly or through functional interactions with the 5-HT2A receptor, modulates psilocin-induced hallucinations. Administration of the partial agonist buspirone, targeting the 5-HT1A receptor, effectively alleviates subjective symptoms associated with psilocybin use in humans (Pokorny et al., 2016). Activation of 5-HT1A receptor and 5-HT2A receptor manifests opposing effects, with 5-HT2A receptor activation being dominant in the presence of psilocin. Specifically, activation of the 5-HT2A receptor leads to pyramidal neuron excitation, while activation of the 5-HT1A receptor inhibits their activity (Preller et al., 2020). Moreover, changes in global brain connectivity induced by psilocin exhibit a strong positive correlation with the expression of the 5-HT2A receptor gene, but a negative correlation with the expression of the 5-HT1A receptor gene (Preller et al., 2020). Administration of the 5-HT2C receptor antagonist RS-102221 demonstrates a dose-dependent modulation of 5-hydroxytryptamine receptor activity, wherein lower doses are associated with augmentation of HTR, whereas higher doses result in a decrement in HTR (Shahar et al., 2022).
The ongoing discourse revolves around the crucial inquiry into whether the antidepressant efficacy of psilocin is contingent upon the activation of the 5-HT2A receptor. Contrasting findings exist; certain investigations propose that ketanserin’s incapacity to impede the antidepressant effects and dendritic spine formation in chronically stressed mice induced by psilocybin implies a potential dissociation from 5-HT2A receptor blockade (Hesselgrave et al., 2021; Shao et al., 2021). The prospect that the 5-HT2A receptor mediates these effects warrants careful consideration. This discordance may arise from various factors: a potential inadequacy in ketanserin dosage to effectively obstruct the 5-HT2A receptor, direct initiation of the brain-derived neurotrophic factor-tropomyosin receptor kinase B (BDNF–TRKB) signaling pathway downstream of 5-HT2A receptor by psilocin, or the plausible involvement of other 5-HT receptors in facilitating the antidepressant effects. The augmentation of neuroplasticity and enhancement of neural connectivity through the activation of intracellular 5-HT2A receptor by psilocin substantiate the critical role of 5-HT2A receptor activation in achieving sustained antidepressant outcomes (Vargas et al., 2023). The proposition to generate 5-HT2A receptor knockout mice could potentially elucidate the necessity of 5-HT2A receptor activation in the context of psilocybin’s effects (Table 1).
In further elucidating the pharmacological actions of psilocybin upon additional 5-HT receptors, it becomes paramount to expand our investigative purview to encompass the comprehensive spectrum of psilocybin’s effects, transcending the confines of 5-HT receptors.
2.3 Dosage
The optimal dosage of psilocybin continues to pose an unresolved challenge in current research. Conventional investigations rely on a fixed dose ranging from 10 to 30 mg, incorporating micro-dosing or weight-adjusted dosing strategies (Griffiths et al., 2016; Goodwin et al., 2022; Marschall et al., 2022). Nevertheless, recent discoveries indicate that body weight exerts minimal influence on psilocin plasma concentration, and body mass index fails as a predictor of responses to psilocybin, challenging the necessity of weight-adjusted dosing (Holze et al., 2023; Spriggs et al., 2023).
The therapeutic viability of micro-dosing psilocybin for anxiety and depression remains a subject of debate. Some investigations propose that recurrent micro-dosing significantly diminishes negative emotions, amplifies cognitive capabilities (Lea et al., 2020; Rootman et al., 2021, 2022), and offers relief from chronic pain in humans (Lyes et al., 2023). Supporting this notion, there is empirical support indicating that micro-dosing can enhance cognitive functions in rodent models. Noteworthy experiments illustrate that low doses of psilocybin enhance motivation and attention in rats exhibiting suboptimal performance (Higgins et al., 2021). Additionally, micro-dosing with Psilocybin has been demonstrated to ameliorate cognitive deficits observed in a rat model of Fragile X syndrome (Buzzelli et al., 2023). Conversely, alternate findings suggest that while micro-dosing may induce perceptible subjective effects and modify brain electroencephalogram rhythms, no noteworthy distinctions in anxiety, depression, and stress symptoms emerge when compared to a placebo cohort (Cavanna et al., 2022; Marschall et al., 2022). Moreover, at present, there exists a dearth of evidence supporting improvements in happiness, creativity, and cognitive function through micro-dosing in humans (Cavanna et al., 2022; Marschall et al., 2022).
The restricted sample sizes in extant studies contribute to the ambiguity surrounding the optimal dosage of psilocybin and the efficacy of micro-dosing. Consequently, Comprehensive comparative studies encompassing diverse dosage levels are imperative to clarify the optimum psilocybin dose and appraise the effectiveness of micro-dosing.
2.4 Gender and/or sex
Earlier investigations hint at the potential for gender variations in the therapeutic outcomes of psilocybin. Notably, the application of psilocybin prompts gender-specific alterations in the reactivity of the central amygdala (Effinger et al., 2023). Moreover, compelling evidence indicates that a singular dose of psilocybin leads to a reduction in ethanol consumption among male C57BL/6 J mice, with no corresponding effect observed in their female counterparts (Alper et al., 2022). These observations underscore the significance of incorporating gender as a crucial biological variable in the context of psilocybin research.
3 Potential mechanisms of psilocybin treatment for AD
3.1 Neuronal plasticity
3.1.1 Impaired neuroplasticity in AD patients
Neuronal plasticity is an intricate response of neurons to both internal and external stimuli, encompassing a range of mechanisms. These mechanisms include neurogenesis, dendritogenesis, and synaptogenesis (Castrén and Hen, 2013; Von Bernhardi et al., 2017). In the context of AD, autopsy findings have revealed a significant reduction in brain synapses compared to healthy individuals (Chang et al., 2013; Scheff et al., 2016). Positron emission tomography imaging targeting synaptic vesicle glycoprotein 2A (SV2A) has demonstrated a decline in synaptic density in the early stages of AD, which correlates with cognitive function (Mecca et al., 2022). Furthermore, aside from synaptic damage, decreased neurogenesis in the dentate gyrus has been observed, as evidenced by a reduction in the number of doublecortin (DCX)- and bromodeoxyuridine (BrdU)-positive cells in APPswe/PSEN1ΔE9 mice (Arredondo et al., 2021). Importantly, impaired neurogenesis occurs prior to the formation of Aβ plaques and neurofibrillary tangles (Moreno-Jiménez et al., 2019). Overall, these findings shed light on the intricate relationship between neuronal plasticity and the pathological progression of AD.
3.1.2 BDNF/TrkB and mTOR signaling pathways
Neuronal plasticity involves the BDNF/TrkB and mammalian target of rapamycin (mTOR) signaling pathways (Ly et al., 2018). BDNF, a crucial neurotrophic factor, plays important roles in promoting neuronal growth and maturation during development, as well as regulating synaptic transmission and plasticity in adulthood. BDNF and its receptor TrkB are localized at glutamatergic synapses, regulating synaptogenesis, neuronal plasticity, memory formation, and learning (Wang et al., 2022). Deficient BDNF/TrkB activity is associated with neurodegeneration in AD (Wang et al., 2019). As the disease progresses, levels of BDNF decrease in the brains (Aarons et al., 2019), blood (Piancatelli et al., 2022) and cerebrospinal fluid (CSF) of AD patients (Bharani et al., 2020). Deprivation of BDNF/TrkB leads to increased inflammatory cytokines and activates the Janus Kinase 2/Signal Transducer and Activator of Transcription 3 (JAK2/STAT3) pathway. This upregulates the transcription factor CCAAT-enhancer binding protein β (C/EBPβ), resulting in increased expression of δ-secretase. Consequently, both amyloid precursor protein (APP) and Tau are fragmented by δ-secretase, leading to neuronal loss (Wang et al., 2019; Xiang et al., 2019; Wu et al., 2021). Intracerebroventricular injection of adeno-associated viruses encoding human BDNF gene alleviates behavioral deficits, prevents neuronal loss, mitigates synaptic degeneration, and reduces neuronal abnormalities in the brains of P301L mice (Jiao et al., 2016). Furthermore, the mTOR signaling pathway also plays a role in neurogenesis, dendritogenesis, and LTP (Jaworski and Sheng, 2006). The relationship between mTOR signaling and AD is not yet fully understood. Some studies suggest that excessive activation of mTOR signaling in AD patients impairs autophagy, leading to increased Aβ deposition and tau phosphorylation (Rapaka et al., 2022). However, other studies suggest that mTOR activation in microglial cells enhances Aβ plaque clearance in AD animal models.
3.1.3 Psilocybin enhances neuroplasticity
Psilocybin has demonstrated potential in enhancing neuronal plasticity at both the cellular and molecular levels, suggesting promise for Alzheimer’s disease (AD) treatment (Figure 3). However, it is crucial to acknowledge that much of this remains theoretical or speculative in the absence of robust experimental evidence. Further investigation using AD animal models is necessary to validate these findings.
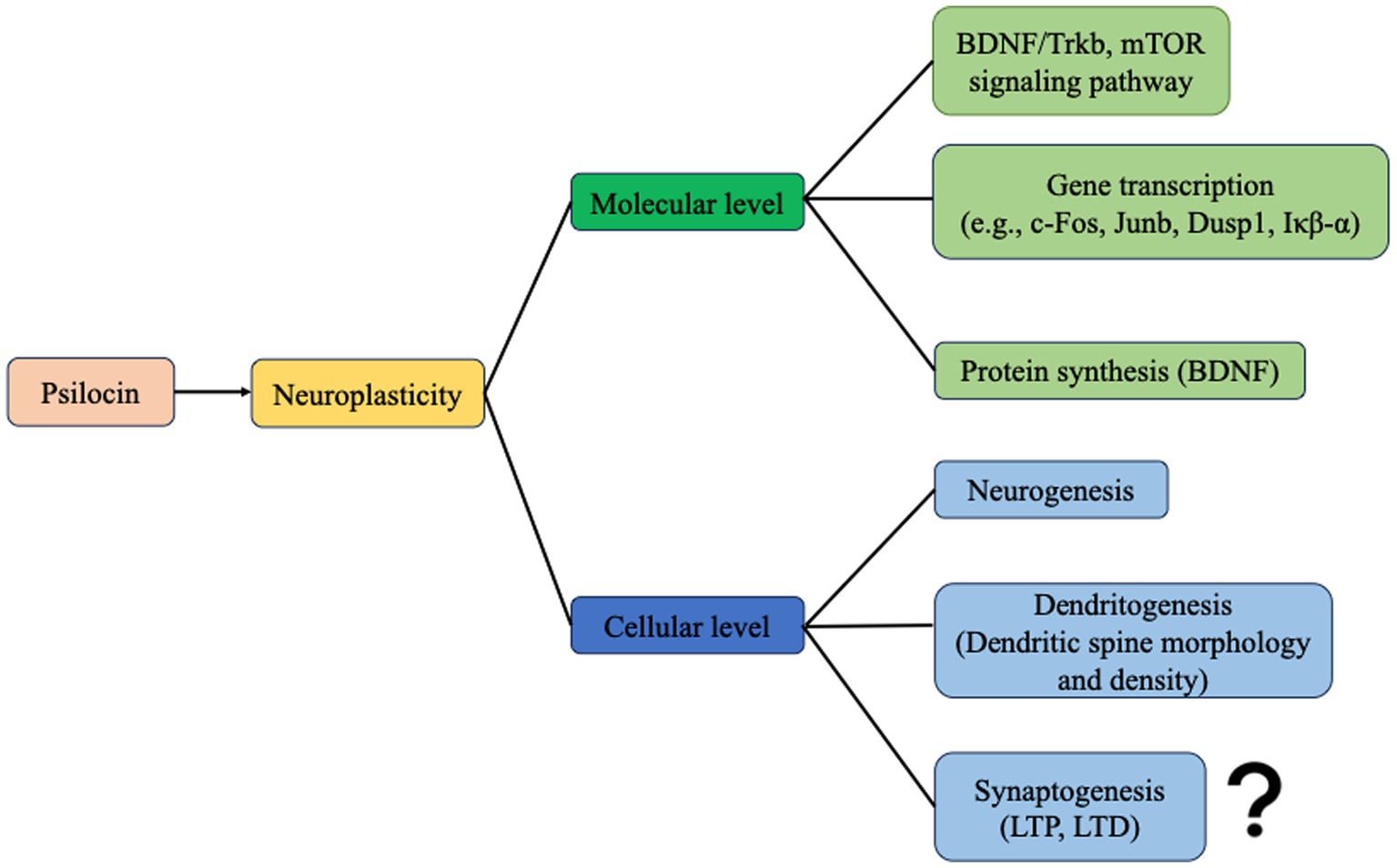
Figure 3. Psilocin promotes neuroplasticity at the molecular and cellular levels. At the molecular level, psilocin enhances neuroplasticity-related signaling pathways, gene transcription, and protein synthesis. And at the cellular level, psilocin promotes neurogenesis and dendritogenesis. However, synaptogenesis has not been evaluated yet.
3.1.3.1 Cellular level
At the cellular level, psilocybin promotes neurogenesis by rescuing the reduction in DCX and BrdU positive cells caused by fear conditioning-induced stress in the hippocampus (Du et al., 2023; Zhao et al., 2024). Moreover, mice administered low doses of psilocybin have shown a swifter extinction of cued fear conditioning compared to those given higher doses. Lower doses of psilocybin are generally correlated with increased neurogenesis (Catlow et al., 2013).
In addition to its neurogenesis-promoting effects, psilocybin also enhances dendritogenesis. For example, it increases the size and density of apical dendritic spines on layer V pyramidal neurons in the medial prefrontal cortex (PFC) by 10%, leading to rapid structural remodeling within 24 h that persists for 1 month (Shao et al., 2021). Similarly, psilocybin rescues the decline in dendritic complexity and spine density induced by conditioned fear in the hippocampus (Du et al., 2023). Additionally, it has been observed to increase the density of SV2A in the hippocampus and PFC of pig brains, indicating greater presynaptic density (Raval et al., 2021). Psilocybin has been shown to mitigate the inhibition of neuroplasticity caused by chronic corticosterone exposure in the PFC and hippocampus. This includes increasing neuroplasticity, as evidenced by a higher total number of dendritic branches and dendritic spine density, as well as elevated levels of synaptic proteins such as phosphorylated GluA1, PSD95, and synapsin-1 (Zhao et al., 2024). Psychedelic mushroom extract and chemically synthesized psilocybin may have different effects on neuroplasticity. Psychedelic mushroom extract significantly increased GAP43, PSD95, synaptophysin, and SV2A across all brain areas, while psilocybin effects were limited to PSD95 and SV2A in the hippocampus and amygdala (Shahar et al., 2024).
LTP and LTD are considered to be fundamental mechanisms underlying synaptic plasticity, which is crucial for learning, memory, and adaptive behavior (Diering and Huganir, 2018). While the effects of psilocybin on the initiation, maintenance, and decline of LTP and LTD have been sparsely explored. Therefore, further research is warranted to enhance our comprehension in this area.
3.1.3.2 Molecular level
At the molecular level, psilocin primarily exerts its influence on neuronal plasticity by activating the 5-HT2A receptor on glutamatergic pyramidal cells in the deep cortical layers (Figure 4; Vollenweider and Kometer, 2010; Shao et al., 2021). Psilocybin administration leads to a notable increase in extracellular glutamate levels in the prefrontal cortex (Vollenweider and Kometer, 2010). This glutamate release activates α-amino-3-hydroxy-5-methyl-4-isoxazole propionic acid receptor (AMPAR) and N-methyl-D-aspartate receptor (NMDAR) on cortical pyramidal neurons (Vollenweider and Kometer, 2010). However, recent studies have shown that psilocybin administration leads to a significant increase in AMPA current amplitude, while NMDA current amplitude remained unchanged, indicating that psilocybin-induced synaptic enhancement may be regulated by postsynaptic AMPAR activity (Hesselgrave et al., 2021). The binding of glutamate to the AMPAR and NMDAR triggers the release of BDNF, leading to the activation of the BDNF/TrkB pathway (Vollenweider and Kometer, 2010; Zhao et al., 2024).
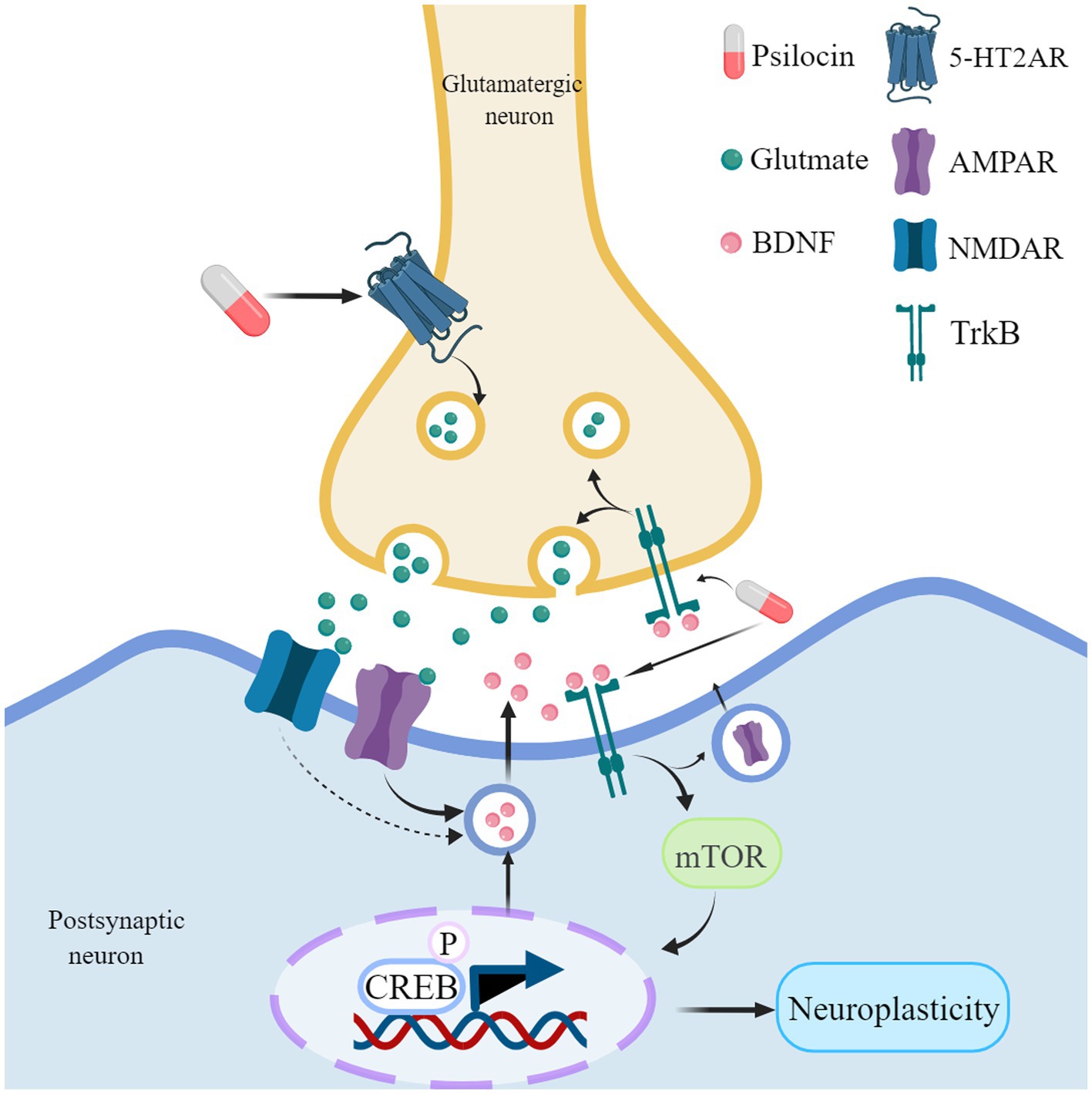
Figure 4. Psilocin promotes neuroplasticity mechanisms. Psilocin interacts with 5-HT2A receptors located on glutamatergic neurons, which in turn promotes the release of glutamate. The release of glutamate can potentially activate both AMPA and NMDA receptors, leading to the release of BDNF. When BDNF binds to its receptor Trkb, it activates the mTOR signaling pathway, resulting in the phosphorylation of CREB. This phosphorylation ultimately leads to an upregulation of genes and proteins associated with neuroplasticity. Furthermore, it facilitates the release of presynaptic glutamate and promotes the transmission of AMPA receptors to the synapse, sustaining the activation of AMPA receptors. In addition, psilocybin may directly bind to TrkB receptors and enhance endogenous BDNF signaling. Created with MedPeer (www.medpeer.cn).
mTOR regulates the phosphorylation of the cyclic AMP-responsive element-binding protein (CREB), resulting in an upregulation of neuroplasticity-related proteins (including BDNF) and gene expression (e.g., c-Fos, Junb, Dusp1, Iκβ-α) (Takei et al., 2004; Jefsen et al., 2021; Davoudian et al., 2023). Psilocybin increased activity in brain regions associated with stress, reward, and motivation in rats. Specifically, it boosted a protein called Fos in areas like the frontal cortex, nucleus accumbens, amygdala, and locus coeruleus. This effect was stronger with higher doses of psilocybin (Funk et al., 2024). In addition, BDNF can induce the release of glutamate in cortical neurons and enhance the transmission of AMPA receptors to synapses (Takei et al., 1998; Matsumoto et al., 2001; Numakawa et al., 2002; Caldeira et al., 2007). This may explain why AMPAR activation continues even after blocking 5-HT2A receptors with ketaserin. Excessive activation of mTOR can have a dual impact, potentially both promoting synaptic plasticity and impairing autophagy (Jaworski and Sheng, 2006; Rapaka et al., 2022). Therefore, it is crucial to demonstrate that psilocybin does not exacerbate the phenotype in rodent models of AD.
3.2 Anti-inflammation
3.2.1 Neuroinflammation and its role in AD progression
Neuroinflammation is of utmost importance in the pathogenesis and advancement of AD. The deposition of Aβ peptides triggers the activation of microglial cells, leading to brain neuroinflammation (Heneka et al., 2013). Activation of the NLRP3 (NOD-, LRR- and pyrin domain-containing 3) inflammasome results in the cleavage and increased activity of caspase-1 within microglial cells, ultimately releasing interleukin (IL)-1β. The tau protein can activate the NLRP3 inflammasome, and the intracerebral injection of brain homogenate containing fibrillar Aβ induces NLRP3-dependent tau pathology changes (Ising et al., 2019). Pro-inflammatory cytokines such as IL-1, IL-6, and tumor necrosis factor alpha (TNF-α) enhance inflammation by stimulating microglial cells and increasing Aβ, thereby contributing to the inflammatory progression of AD. Inflammatory mediators activate cyclooxygenase (COX) -2 in neuroinflammation, which, through the arachidonic acid pathway, synthesizes and releases prostaglandins, further stimulating microglial cells (Dhapola et al., 2021). Triggering receptor expressed on myeloid cells 2 (TREM2), expressed on the surface of microglial cells, has been found to increase the risk of AD when mutated. Genetic ablation of TREM2 resulted in less severe plaque pathology in the early stages of plaque deposition but more severe plaque pathology in the later stages (Carmona et al., 2018). Elevating the amount of TREM2 through the introduction of a human TREM2 transgene reduced pathology in Aβ-bearing mice (Zhong et al., 2019; Wang et al., 2020).
3.2.2 Anti-inflammatory effects of psilocybin
The current research suggests some potential benefits of Psilocybin in the treatment of neuroinflammatory diseases. Activation of the 5-HT2A receptor which is found on lymphocytes, and macrophages, has been proven to regulate immune function and cytokine production (Pellegrino and Bayer, 2002; Xiao et al., 2016). In the context of peripheral immunity, extracts from psilocybin-containing mushrooms have been shown to suppress the production of inflammatory mediators COX-2, nitric oxide, and prostaglandin E2 in Lipopolysaccharide (LPS)-induced macrophages, while also reducing pro-inflammatory cytokines IL-1β, IL-6, and TNF-α, and increasing anti-inflammatory cytokine IL-10 (Nkadimeng et al., 2020a, 2021). Psilocin, but not psilocybin, exerts anti-inflammatory effects on classically activated macrophages (Laabi et al., 2024). Additionally, psilocybin mushrooms have the potential to protect the heart by mitigating TNF-α-induced cardiomyocyte injury and death (Nkadimeng et al., 2020b). Moreover, in an inflammatory response induced by TNF-α and interferon (IFN)-γ in human small intestinal epithelial cells, psilocybin reduces the levels of COX-2 and IL-6 without affecting cell viability. Animal models have also yielded similar results. Psilocybin was shown to reduce inflammation in a 3D model of human intestinal tissue. This anti-inflammatory effect was evidenced by a decrease in TNF-α, IFN-γ, IL-6, and IL-8 levels (Robinson et al., 2023). There is still debate regarding whether psilocybin can alter inflammatory markers in healthy subjects. One study has demonstrated that psilocybin can reduce the concentrations of TNF-α, IL-6, and C-reactive protein (CRP) in healthy volunteers (Mason et al., 2023). However, another research has found no significant changes in the levels of CRP, TNF, or soluble urokinase plasminogen activator receptor (suPAR) (Burmester et al., 2023). It’s possible that the conflicting results could be attributed to the small sample size and the low baseline levels of inflammatory markers in healthy subjects.
Regarding central immunity, psilocybin exhibits significant anti-inflammatory effects in an immortalized microglial cell line, as evidenced by decreased TNF-α secretion in response to LPS challenge (Smedfors et al., 2022). Additionally, psilocin can influence microglial functions by reducing the level of toll-like receptor 4 (TLR4), NF-κB p65 (p65), and cluster of differentiation 80 (CD80) proteins, which are markers of the immune response, and upregulate the TREM2 neuroprotective receptor (Kozłowska et al., 2021). In an LPS-induced mouse brain inflammation model, eugenol, psilocybin, and their combination treatment reduce the expression of various markers such as COX-2, TNF-α, IL-1β, IL-6, and IL-8 (Zanikov et al., 2023). In the 5xFAD mouse model, the activation of mTOR in microglial cells can upregulate TREM2 and promote the clearance of Aβ (Shi et al., 2022). Therefore, it can be speculated that psilocybin may also clear Aβ through this mechanism. Previous research has often focused primarily on peripheral markers when selecting inflammatory markers. Thus, in future studies, it is recommended to enhance the sample size and assess the direct influence of psilocybin on neuroinflammation, for instance, metabolites of the kynurenine-tryptophan, nitric oxide, and neopterin pathways have been suggested as suitable CSF biomarkers for neuroinflammation (Burmester et al., 2023).
The precise anti-inflammatory mechanisms of psilocybin remain to be fully elucidated. While Laabi et al. have proposed potential pathways, further experimental validation is necessary to confirm these hypotheses and gain a comprehensive understanding of psilocybin’s anti-inflammatory actions (Laabi et al., 2024).
Besides, there is also evidence suggesting a connection between autoimmune disorders and AD (Lim et al., 2021). Case studies have indicated that weekly administration of low-dose psilocybin, 3–4 times per week, is effective in treating neuro-Lyme disease. The pathogenesis of neuro-psychiatric Lyme disease is associated with autoimmune-induced neuroinflammation, suggesting that the anti-inflammatory effects of psilocybin may be valuable in autoimmune neuroinflammation (Kinderlehrer, 2023).
3.3 Neuropsychology
Neuropsychology constitutes an interdisciplinary field dedicated to elucidating the intricate interplay between the brain and psychology, with a primary focus on the cognitive, emotional, and behavioral functionalities of the cerebral organ. Emerging research suggests that psilocybin may exert effects within the realm of neuropsychology, an area continuously evolving in scientific inquiry and exploration.
3.3.1 Cognitive flexibility
Cognitive flexibility, characterized by the adaptive capacity to transition between diverse cognitive operations in response to changing environmental demands, constitutes a pivotal facet of cognitive function (Uddin, 2021). Evaluation typically involves tasks related to set-shifting or rule-switching, gauging the ability to transition from previously acquired rules (Koch et al., 2018). In rodents, acute administration of psilocybin has been observed to enhance cognitive flexibility, with specificity noted in the augmentation of switching between previously learned behavioral strategies rather than influencing Pavlovian reversal learning. The 5HT 2AR antagonist ketanserin was found to attenuate psilocybin-induced effects on set-shifting, while a 5HT2C-selective antagonist exhibited no such influence (Torrado Pacheco et al., 2023). For healthy adults and individuals with Major Depressive Disorder (MDD), psilocybin treatment has shown promise in augmenting cognitive and neural flexibility (Doss et al., 2021; Nayak et al., 2023).
This enhancement is postulated to stem from increased functional connectivity dynamics between the anterior cingulate cortex and posterior cingulate cortex following psilocybin administration, coupled with the neuroplasticity promoted by the substance (Doss et al., 2021). Additionally, metabotropic glutamate receptor (mGluR) 2 has been implicated in cognitive flexibility. Knocking out neuron-specific frontal mGluR2 in rats resulted in reduced cognitive flexibility and heightened alcohol-seeking behavior, a deficit that psilocybin was able to ameliorate by restoring mGluR2 expression (Meinhardt et al., 2021). Interestingly, mGluR2 is considered a potential target for Alzheimer’s disease (AD) drug treatment. Studies on transgenic mouse models of tauopathy have demonstrated that the selective agonist LY379268, targeting both mGluR2/3, effectively inhibits tau release in synaptosomes and prevents tau propagation between neurons. Conversely, BCI-838, an orally active pro-drug acting as an mGluR2/3 receptor antagonist, has exhibited efficacy in improving learning behavior deficits (Mazzo et al., 2022; Perez-Garcia et al., 2023). Consequently, additional experimental evidence is requisite to elucidate whether the impact of psilocin on mGluR2 could translate into cognitive flexibility improvements.
3.3.2 Emotional face recognition
Mild cognitive impairment (MCI) and AD patients often experience deficits in emotion recognition (Yang et al., 2015; Mazzi et al., 2020). Remarkably, when administered alongside psychological support, psilocybin has shown potential in improving the processing of emotional faces in TRD (Stroud et al., 2018). Furthermore, in studies involving healthy individuals, psilocybin has been observed to act on the 5-HT2A receptor, influencing facial recognition, goal-directed behavior, and emotional states by promoting positive emotions over negative ones (Kometer et al., 2012; Kraehenmann et al., 2015). Patients with TRD or healthy volunteers who receive psilocybin treatment may experience a decrease in amygdala response to negative emotions, likely due to heightened functional connectivity between the amygdala and the ventromedial prefrontal cortex and posterior cingulate cortex. Unlike selective serotonin reuptake inhibitors (SSRIs), which alleviate negative emotions, psilocybin enables patients to confront and address them (Roseman et al., 2018; Barrett et al., 2020; Mertens et al., 2020; Zeifman et al., 2023).
3.3.3 Creativity
Creativity, a fundamental cognitive faculty intricately woven into various facets of daily functioning (Ross et al., 2023), manifests differently in individuals with Alzheimer’s disease (AD), particularly in artistic and literary pursuits. Artists with AD tend to gradually produce paintings characterized by simplicity and abstraction, while writers create works featuring less intricate plots and a constrained vocabulary (Acosta, 2014; Pelowski et al., 2022). A comprehensive examination of psilocybin macrodosing effects on cognition and creativity, elucidated through a scoping review, revealed a time-dependent variation. Initial impairment post-intake was noted, receding over time, with subsequent emergence of positive effects (Bonnieux et al., 2023). However, the controversy surrounding micro-dosing of psilocybin persists. Studies exploring this avenue propose acute enhancements in creativity (Anderson et al., 2019), yet a specific investigation posits that low doses of psilocybe cubensis may not significantly impact creativity (Cavanna et al., 2022). This discrepancy could be attributed to the possibility that the effective dose of psilocybin within psilocybe cubensis might be insufficient to exert a noteworthy influence on creativity.
Psilocybin acutely reduces convergent thinking, increases spontaneous divergent thinking and goal-oriented divergent thinking. These effects are associated with a decrease in the integrity of the default mode network (DMN) and an increase in functional connectivity between resting-state networks induced by psilocybin (Mason et al., 2019, 2021).
3.4 AD comorbid with depression and anxiety
3.4.1 The acceleration of AD progression by depression and anxiety
Depression and anxiety are prevalent neuropsychiatric manifestations in individuals with AD. Intriguingly, a discernible genetic correlation between depression and AD has been identified, suggesting a shared genetic foundation (Martín-Sánchez et al., 2021; Monereo-Sánchez et al., 2021; Harerimana et al., 2022). Noteworthy is the association of late-onset depression and anxiety with a significantly elevated risk of dementia development, with the severity of depression being linked to an increased susceptibility to AD. Moreover, anxiety has been implicated in the deposition of Aβ protein and cognitive decline (Becker et al., 2018; Chan et al., 2020; Johansson et al., 2020; Kuring et al., 2020; Kim D et al., 2021; Kim H et al., 2021). It is crucial to emphasize that depression and anxiety serve not only as risk factors for AD but also actively contribute to the progression of the disease (Gallagher et al., 2018; Lee et al., 2019; Ruthirakuhan et al., 2019; Ma, 2020; Agüera-Ortiz et al., 2021).
3.4.2 Psilocybin therapy for depression and anxiety in AD
There is currently no consensus regarding the preferred choice of treatment for depression in patients with dementia, with selective serotonin reuptake inhibitors (SSRIs) appearing to be one commonly considered option. Initial findings from a longitudinal study suggested that the use of antidepressants may result in a slower decline in cognition and daily functioning (Dutcher et al., 2014). However, the Health Technology Assessment Study of the Use of Antidepressants for Depression in Dementia (HTA-SADD) trial contradicted this, revealing no therapeutic efficacy of sertraline or mirtazapine and an increased risk of adverse events (Banerjee et al., 2013; Zuidersma et al., 2019). A recent systematic review and meta-analysis of randomized controlled trials failed to demonstrate any beneficial effects of second-generation antidepressants on cognition and depression, including various informative subgroups (Qin et al., 2022). Another systematic review and network meta-analysis indicated psychoeducation was the sole effective intervention against anxiety (Sun et al., 2022). Consequently, the management of depression in individuals with Alzheimer’s disease (AD) and comorbid anxiety necessitates innovative therapeutic approaches.
Notably, an open-label pilot study initiated by Johns Hopkins University in 2019 aims to assess the safety and efficacy of administering psilocybin under supportive conditions for depression in patients with mild cognitive impairment (MCI) or early-stage AD. Preliminary results from this study are expected to be disclosed in 2024 (ClinicalTrials.gov NCT04123314).
Addressing the symptoms of depression and anxiety accompanying Alzheimer’s disease (AD) becomes crucial for slowing down disease progression. Several clinical studies have presented evidence supporting the effectiveness of psilocybin in treating MDD (Carhart-Harris et al., 2016, 2021; Davis et al., 2021), TRD (Goodwin et al., 2022, 2023a), and depression and anxiety symptoms in advanced cancer patients (Griffiths et al., 2016).
The DMN refers to a group of brain regions that show decreased activation during goal-directed or attention-demanding tasks. It primarily includes the medial PFC, posterior cingulate/retrosplenial cortex, and left and right inferior parietal lobules (Whitfield-Gabrieli and Ford, 2012). Individuals with anxiety and depression share similar alterations in the DMN, characterized by increased functional connectivity within the DMN and reduced connectivity between the DMN and other higher-order networks like the salience network and central executive network (Whitfield-Gabrieli and Ford, 2012; Li et al., 2023). In comparison to escitalopram, psilocybin exhibits a faster onset, better tolerance, and longer duration of effect, potentially leading to improved outcomes. Psilocybin may contribute to a decrease in functional connectivity within the DMN and an increase in connectivity between the DMN and other functional networks rich in 5-HT2A receptors (Carhart-Harris et al., 2021; Daws et al., 2022; Gukasyan et al., 2022; Zeifman et al., 2023). Combining psilocybin with mindfulness meditation and other psychological therapy techniques could be beneficial, as both mindfulness and psilocybin therapy seem to modulate the DMN in similar ways and may have a synergistic effect (Smigielski et al., 2019). Additionally, psilocybin treatment has been associated with reduced amygdala cerebral blood flow, correlating with the alleviation of depressive symptoms (Carhart-Harris et al., 2017). The decrease in amygdala blood flow caused by psilocybin may reflect the neuroregulatory effects of 5-HT1A receptors (Lewis et al., 2017). Furthermore, transient elevation of plasma glucocorticoids supports psilocybin-induced anxiolysis in mice, suggesting that acute, resolvable psilocybin-induced glucocorticoid release drives the post-acute anxiolytic-like effects of psilocybin in mice (Jones et al., 2023).
4 Adverse effect
Clinical investigations spanning from 2018 to the present, emphasizing the utilization of psilocybin in the treatment of Major Depressive Disorder (MDD), Treatment-Resistant Depression (TRD), migraine, and other maladies, alongside trials involving subjects without pre-existing conditions, reveal a low incidence of severe adverse events. Predominantly reported adverse reactions encompass transient and self-limiting manifestations such as headache, nausea, vomiting, fatigue, among others (Table 2). These reactions are often dose-dependent and typically do not necessitate specific interventions (Davis et al., 2021; Schindler et al., 2021; Perez et al., 2023). Symptomatic medications like paracetamol or ibuprofen can be employed, if required, to mitigate headaches (Goodwin et al., 2023b). Notably, a clinical trial reported serious adverse events including suicidal ideation, codeine withdrawal syndrome, and self-injury depression (Goodwin et al., 2022).
In the current examination of psilocybin’s adverse reactions, several limitations merit acknowledgment. Firstly, the modest sample size in some studies may lead to the omission of infrequent adverse events, thus constraining the generalizability of the results. Secondly, the studied patient population often excludes individuals with a history of mental illness and severe suicide attempts. Administering psilocybin to such patients without rigorous supervision could result in severe consequences, exemplified by a case report detailing a manic episode following psilocybin use in an individual with bipolar II disorder (Daws et al., 2022; Gukasyan, 2023; Halim et al., 2023). Thirdly, the scarcity of placebo-controlled trials hinders the establishment of a causal relationship between psilocybin and adverse events. Numerous variables, encompassing drug dosage, age, gender, education, and experimental conditions, may influence adverse reactions in patients. Fourthly, as psilocybin trials broaden to include a larger participant pool, it becomes imperative to investigate whether the increase in blood pressure induced by psilocybin has implications for hypertension prevalence in the general population. Additionally, uncertainties persist regarding potential significant adverse reactions arising from the combination of psilocybin with other psychotropic medications, necessitating exploration into the advisability of discontinuing these medications before undergoing psilocybin treatment.
The administration of psilocybin requires rigorous supervision, given the potential serious consequences of self-administration. Several case reports underscore the risks associated with self-administration of psilocybin mushrooms, including takotsubo cardiomyopathy (Nef et al., 2009; Kotts et al., 2022), severe rhabdomyolysis (Bickel et al., 2005; Suleiman et al., 2022), bipolar disorder (Hendin and Penn, 2021), distressing flashback phenomena hallucinogen (Müller et al., 2022), and even persisting perception disorder (Espiard et al., 2005).
5 Removing the hallucinogenic effects of psilocybin
5.1 Hallucinogenic effects constrain the development of psilocybin
Profound investigations into the mechanisms of psilocybin’s action are imperative for addressing its hallucinogenic effects. The distinctive psychedelic properties of psilocybin raise concerns about its prospective clinical application. Effective mitigation of its hallucinogenic effects is paramount for potential clinical utilization, even upon prospective market approval. Importantly, it should be noted that hallucination is not classified as a side effect.
A pivotal inquiry emerges: Are the hallucinogenic effects of psilocybin inherently therapeutic, or does their separation result in a decline in therapeutic efficacy? The current absence of non-hallucinogenic 5-HT2A agonists with demonstrated clinical effectiveness in humans underscores the imperative for further investigation. Additionally, scrutinizing the limitations of existing non-hallucinogenic antidepressants is essential, particularly considering the reliance on rodent models and tests that may lack direct applicability to humans.
5.2 Exploring methods to reduce the hallucinogenic effects of psilocybin
Several approaches are explored to reduce psilocybin’s hallucinogenic effects (Figure 5).
5.2.1 Medication combination
The head twitch response mediated by the 5-HT2A receptor, triggered by psilocybin, is observed to be reduced by the agonist activity of the 5-HT1A receptor, exemplified by buspirone, especially at elevated doses in mice (Glatfelter et al., 2023; Singh et al., 2023). Despite the potential inhibitory effect of 5-HT2A receptor antagonists on the head twitching reaction, caution is warranted, as blocking the 5-HT2A receptor may significantly impact therapeutic efficacy. Given the plausible involvement of the 5-HT2A receptor in mediating neural plasticity, blocking 5-HT2A receptor may not be an optimal choice.
5.2.2 Mechanistic insights
In-depth research into the mechanisms of psilocybin’s action contributes to discerning its hallucinogenic effects. Although both psilocin and serotonin interact with the 5-HT2A receptor, the specific neuroplasticity-promoting effects observed with psilocybin are not exhibited by serotonin. This distinction is primarily attributed to a location bias, as the intracellular 5-HT2A receptor mediates the neuronal plasticity induced by psilocin, rather than the receptor on the cell membrane (Vargas et al., 2023). In addition to the classical binding mode of psilocin to 5-HT2A receptor, there is an alternative lipid-regulated binding mode that plays a crucial role in activating β-arrestin signaling downstream of 5-HT2A receptor through agonist bias. With this understanding, researchers have designed and synthesized compounds like IHCH-7086 and IHCH-7079, demonstrating rapid antidepressant effects without hallucinogenic side effects in animal experiments (Cao et al., 2022). Research has also indicated the pursuit of structure-based design methodologies for developing high-affinity TrkB-selective ligands characterized by swift and enduring antidepressant effects, while concurrently exhibiting a potential absence of hallucinogenic-like activity (Moliner et al., 2023). Although some studies speculate that the activation of Gs proteins plays a key role in distinguishing between hallucinogenic and non-hallucinogenic 5-HT2A receptor agonists (Liu et al., 2022), this viewpoint may be intricate, considering that the interaction between 5-HT2A receptor and Gs proteins might be just one of many factors influencing hallucinogenic properties.
5.2.3 Virtual drug development
Recent advancements in computer drug development technology have transformed the concept of “virtual drugs” into reality (Gorgulla et al., 2020). The utilization of psychLight, a gene-encoded fluorescence sensor based on the structure of the 5-HT2A receptor, enables precise prediction of the psychedelic behavioral effects of structurally similar ligands that target the 5-HT2A receptor. Moreover, it also facilitates the identification of non-psychedelic analogs that exhibit antidepressant properties (Dong et al., 2021). By exploring the virtual molecular library and discovering a molecule that shares similar characteristics to psilocybin, followed by a comparison of its 3D structure with that of 5-HT2A receptor, it becomes feasible to synthesize a candidate compound that possesses antidepressant activity without any hallucinogenic effects (Kaplan et al., 2022). In addition, transcriptome fingerprints have proven successful in distinguishing between the hallucinogenic and nonhallucinogenic effects of 5-HT2A receptor agonists in mice (González-Maeso et al., 2003). These findings contribute to a rational design approach for the development of psilocybin analogs targeting specific signal pathways.
5.2.4 Chemical engineering
Through precise chemical engineering, researchers are actively working to modify psilocybin, aiming to produce safer variants retaining therapeutic efficacy while eliminating hallucinogenic properties. In recent studies, researchers have introduced a diverse range of cleavable groups at the 4-hydroxy position of the core indole moiety. This strategic modification aims to modulate metabolic processing, resulting in the creation of a novel prodrug of psilocin. Among these active prodrugs, two distinct molecules have demonstrated lasting anxiolytic benefits in chronically stressed mice, as evaluated in the marble-burying psychiatric model (Raithatha et al., 2023).
In general, these methods require in-depth research and clinical trials to ensure that the modified drugs meet the expected standards in terms of safety and therapeutic efficacy.
6 Legal and ethical issues
Psilocybin remains a substance of significant controversy, eliciting varied societal perspectives on its utilization (Doss et al., 2022). In light of this, ensuring the legal and ethical recognition of psilocybin treatment for AD is paramount.
6.1 Legality
Psilocybin encountered pervasive regulatory constraints within the realm of clinical psychiatry commencing in 1967, concomitant with its legal reclassification as a Schedule I controlled substance. This classification limited its usage and research potential. This categorization precipitated limitations on both its clinical utilization and research prospects. In the year 2015, a treatise featured in the British Medical Journal articulated the imperative for a legal reclassification of psychedelic substances, positing a compelling argument for researchers to delve into their therapeutic potential (Rucker, 2015).
Evolving regulatory landscapes have witnessed a gradual relaxation of restrictions pertaining to the medical applications of psilocybin in several nations. In the United States, Psilocybin presently occupies the classification of a Schedule I controlled substance according to federal mandates. Despite this federal designation, select states have commenced authorizing its medical deployment. A watershed moment occurred in May 2019, as Denver, Colorado emerged as the inaugural U.S. city to decriminalize psilocybin, while 2020 saw Oregon becoming the pioneering U.S. state to legalize its supervised medical application for the amelioration of mental disorders (Basky, 2021; Siegel et al., 2023). Projections posit a trajectory wherein the majority of U.S. states will have legislatively embraced psychedelics by the temporal juncture spanning 2034–2037 (Siegel et al., 2023). Besides, Canada has been progressively expanding avenues for therapeutic access to psychedelic substances. In the year 2020, historic exemptions were granted, permitting legal possession and personal use of psilocybin mushrooms—signifying a landmark development nearly five decades subsequent to their criminalization (Mocanu et al., 2022). Recent developments in Jamaica reveal a paradigm shift, where adult patients within psychiatric practice settings have been presented with the option of incorporating psilocybin products into their treatment regimens for conditions encompassing major depressive disorder, post-traumatic stress disorder, substance use disorders, and existential distress (De La Haye et al., 2023). On the antipodean front, noteworthy endeavors have been undertaken in Australia to pave the way for the prescription of MDMA and psilocybin in the treatment of post-traumatic stress disorder and depression—an epochal stride within the global landscape (Haridy, 2023).
However, a prevailing disposition persists in which the majority of countries or regions continue to regard psilocybin as an illicit substance. Divergent perspectives on legalization manifest across societies and nations, underscored by historical, cultural, and legal differentials. It is incumbent upon researchers and practitioners to exercise due diligence within the bounds of legal and regulatory frameworks, ensuring strict adherence to pertinent legislations.
6.2 Informed consent
In the context of conducting psilocybin research on AD patients, the informed consent process demands particular attention and meticulous consideration. Given the cognitive decline faced by AD patients, ensuring the effectiveness of the informed consent process is paramount for safeguarding patient rights (Gauthier et al., 2013). Prior to the commencement of the study, a rigorous individual assessment, specifically focusing on cognitive capabilities, is imperative. If significant impairment in cognitive capacity is identified among AD patients, researchers must ascertain the necessity of legal guardians’ involvement and ensure their legal eligibility for providing informed consent (Lawrence et al., 2014; Ortiz et al., 2022).
The materials provided to patients for informed consent should be lucid, succinct, and tailored to the patient’s level of comprehension, presented through both oral and written modalities. Given the novel therapeutic process, mechanism, and potential unexpected side effects of psilocybin, including shifts in values and personality, rare mental health side effects, and the potential use of therapeutic touch during therapy, special attention is warranted in the context of enhanced consent (Smith and Sisti, 2020).
Considering the potential memory issues faced by AD patients, the informed consent process may necessitate multiple iterations (High, 1992). Repeatedly confirming consent at different time points ensures that patients maintain a clear understanding and possess the ability to withdraw consent at any given moment during their participation in the study. Despite the fluctuating cognitive states of AD patients, their right to decline participation in research should be consistently respected.
6.3 Patient safety and well-being
During the administration of Psilocybin, ensuring a systematic safety monitoring protocol is paramount, encompassing the surveillance of physiological metrics and mental states. Similarly, the formulation of contingency plans is imperative to address potential adverse reactions or unforeseen incidents (Belouin et al., 2022). Given the absence of prior Psilocybin utilization in AD patients, it is crucial, during Psilocybin administration, to ensure the presence of a mental health therapist or a qualified psychological professional for support. These professionals should be equipped to navigate and address potential emotional or cognitive responses that may arise during Psilocybin use.
6.4 Appropriate experimental design
Given the potential variability in individual responses to Psilocybin, particularly among AD patients, a precise calculation of personalized doses is imperative. Furthermore, the duration of Psilocybin administration and the overall research protocol should be individualized to cater to the unique needs of each patient.
The cognitive state of AD patients may influence their perception of time and processing abilities (Honma et al., 2015). Therefore, the study design should contemplate the reduction of experimental duration to accommodate potential shorter attention spans and tolerance levels in patients (Ortiz et al., 2022).
Given that the psychedelic properties of Psilocybin may induce fatigue or emotional fluctuations in patients (Griffiths et al., 2011), the research design should allow for adequate resting intervals. This approach aids in alleviating the psychological burden on patients and facilitates adaptive periods.
In the context of AD patients, consideration should be given to involving family members or caregivers. Their participation can provide supplementary support, assist in monitoring patient responses, and offer additional safety and emotional backing when necessary.
7 Conclusions and perspectives
Recent strides in utilizing psilocybin for the treatment of neuropsychiatric disorders exhibit considerable promise. Psilocybin holds potential for conferring distinctive advantages in terms of neuroprotection and cognitive enhancement for individuals with Alzheimer’s disease (AD) through mechanisms such as neuroplasticity, inflammation regulation, and enhanced neuropsychology. Furthermore, the antidepressant and anti-anxiety effects of psilocybin may signify substantial advancements in addressing the mental well-being of patients with neuropsychiatric conditions associated with AD. Nonetheless, it is imperative to recognize that a substantial journey lies ahead before psilocybin can be judiciously and efficaciously employed in AD patient care. Stringent scientific inquiry is indispensable to comprehensively discern its efficacy, safety profile, and optimal utilization. Further exploration is warranted to elucidate the precise mechanisms of action of psilocybin. Moreover, the viability of alternative strategies, including combination therapies involving psilocybin, the development of high-affinity TrkB positive allosteric modulators without 5-HT2A activity, and modification of psilocybin’s chemical composition to obviate hallucinogenic effects, necessitates investigation. Additionally, regulatory approvals and ethical considerations demand meticulous attention prior to embarking on clinical trials. Prudent development of psilocybin-based treatments for AD mandates adherence to rigorous scientific standards and ethical guidelines.
Author contributions
SZ: Writing – original draft, Writing – review & editing. RM: Conceptualization, Supervision, Writing – original draft. YY: Conceptualization, Supervision, Writing – original draft. GL: Conceptualization, Supervision, Writing – review & editing.
Funding
The author(s) declare financial support was received for the research, authorship, and/or publication of this article. This study was funded by grants from National Natural Science Foundation of China (grant no. 81974161).
Conflict of interest
The authors declare that the research was conducted in the absence of any commercial or financial relationships that could be construed as a potential conflict of interest.
Publisher’s note
All claims expressed in this article are solely those of the authors and do not necessarily represent those of their affiliated organizations, or those of the publisher, the editors and the reviewers. Any product that may be evaluated in this article, or claim that may be made by its manufacturer, is not guaranteed or endorsed by the publisher.
Footnotes
References
Aarons, T., Bradburn, S., Robinson, A., Payton, A., Pendleton, N., and Murgatroyd, C. (2019). Dysregulation of BDNF in prefrontal cortex in Alzheimer's disease. J. Alzheimers Disease 69, 1089–1097. doi: 10.3233/JAD-190049
Aaronson, S. T., Van Der Vaart, A., Miller, T., Lapratt, J., Swartz, K., Shoultz, A., et al. (2024). Single-dose synthetic psilocybin with psychotherapy for treatment-resistant bipolar type II major depressive episodes: a nonrandomized open-label trial. JAMA Psychiatry 81, 555–562. doi: 10.1001/jamapsychiatry.2023.4685
Acosta, L. M. Y. (2014). Creativity and neurological disease. Curr. Neurol. Neurosci. Rep. 14:464. doi: 10.1007/s11910-014-0464-6
Agrawal, M., Richards, W., Beaussant, Y., Shnayder, S., Ameli, R., Roddy, K., et al. (2024). Psilocybin-assisted group therapy in patients with cancer diagnosed with a major depressive disorder. Cancer 130, 1137–1146. doi: 10.1002/cncr.35010
Agüera-Ortiz, L., García-Ramos, R., Grandas Pérez, F. J., López-Álvarez, J., Montes Rodríguez, J. M., Olazarán Rodríguez, F. J., et al. (2021). Depression in Alzheimer's disease: a Delphi consensus on etiology, risk factors, and clinical management. Front. Psych. 12:638651. doi: 10.3389/fpsyt.2021.638651
Alper, K., Cange, J., Sah, R., Schreiber-Gregory, D., Sershen, H., and Vinod, K. Y. (2022). Psilocybin sex-dependently reduces alcohol consumption in C57BL/6J mice. Front. Pharmacol. 13:1074633. doi: 10.3389/fphar.2022.1074633
Anderson, B. T., Danforth, A., Daroff, P. R., Stauffer, C., Ekman, E., Agin-Liebes, G., et al. (2020). Psilocybin-assisted group therapy for demoralized older long-term AIDS survivor men: an open-label safety and feasibility pilot study. EClinicalMedicine 27:100538. doi: 10.1016/j.eclinm.2020.100538
Anderson, T., Petranker, R., Rosenbaum, D., Weissman, C. R., Dinh-Williams, L. A., Hui, K., et al. (2019). Microdosing psychedelics: personality, mental health, and creativity differences in microdosers. Psychopharmacol. 236, 731–740. doi: 10.1007/s00213-018-5106-2
Anonymous (2021). Alzheimer's disease facts and figures. Alzheimers Dement. 17, 327–406. doi: 10.1002/alz.12328
Anonymous (2022a). Alzheimer's disease facts and figures. Alzheimers Dement. 18, 700–789. doi: 10.1002/alz.12638
Anonymous (2022b). Estimation of the global prevalence of dementia in 2019 and forecasted prevalence in 2050: an analysis for the global burden of disease study 2019. Lancet Public Health 7, e105–e125. doi: 10.1016/S2468-2667(21)00249-8
Arredondo, S. B., Reyes, D. T., Herrera-Soto, A., Mardones, M. D., Inestrosa, N. C., and Varela-Nallar, L. (2021). Andrographolide promotes hippocampal neurogenesis and spatial memory in the APPswe/PS1ΔE9 mouse model of Alzheimer's disease. Sci. Rep. 11:22904. doi: 10.1038/s41598-021-01977-x
Banerjee, S., Hellier, J., Romeo, R., Dewey, M., Knapp, M., Ballard, C., et al. (2013). Study of the use of antidepressants for depression in dementia: the HTA-SADD trial--a multicentre, randomised, double-blind, placebo-controlled trial of the clinical effectiveness and cost-effectiveness of sertraline and mirtazapine. Health Technol. Asses. 17:7070. doi: 10.3310/hta17070
Barrett, F. S., Doss, M. K., Sepeda, N. D., Pekar, J. J., and Griffiths, R. R. (2020). Emotions and brain function are altered up to one month after a single high dose of psilocybin. Sci. Rep. 10:2214. doi: 10.1038/s41598-020-59282-y
Basky, G. (2021). Policy in focus: Is psilocybin the next cannabis? CMAJ: Canadian Medical Association Journal = Journal de L’Association Medicale Canadienne 193, E1741–E1742. doi: 10.1503/cmaj.1095974
Becker, A. M., Holze, F., Grandinetti, T., Klaiber, A., Toedtli, V. E., Kolaczynska, K. E., et al. (2022). Acute effects of psilocybin after escitalopram or placebo pretreatment in a randomized, double-blind, placebo-controlled, crossover study in healthy subjects. Clin. Pharmacol. Ther. 111, 886–895. doi: 10.1002/cpt.2487
Becker, E., Orellana Rios, C. L., Lahmann, C., Rücker, G., Bauer, J., and Boeker, M. (2018). Anxiety as a risk factor of Alzheimer's disease and vascular dementia. Br. J. Psychiatry J. Ment. Sci. 213, 654–660. doi: 10.1192/bjp.2018.173
Belouin, S. J., Averill, L. A., Henningfield, J. E., Xenakis, S. N., Donato, I., Grob, C. S., et al. (2022). Policy considerations that support equitable access to responsible, accountable, safe, and ethical uses of psychedelic medicines. Neuropharmacol. 219:109214. doi: 10.1016/j.neuropharm.2022.109214
Bharani, K. L., Ledreux, A., Gilmore, A., Carroll, S. L., and Granholm, A.-C. (2020). Serum pro-BDNF levels correlate with phospho-tau staining in Alzheimer's disease. Neurobiol. Aging 87, 49–59. doi: 10.1016/j.neurobiolaging.2019.11.010
Bickel, M., Ditting, T., Watz, H., Roesler, A., Weidauer, S., Jacobi, V., et al. (2005). Severe rhabdomyolysis, acute renal failure and posterior encephalopathy after 'magic mushroom' abuse. Eur. J. Emerg. Med. 12, 306–308. doi: 10.1097/00063110-200512000-00011
Bogenschutz, M. P., Ross, S., Bhatt, S., Baron, T., Forcehimes, A. A., Laska, E., et al. (2022). Percentage of heavy drinking days following psilocybin-assisted psychotherapy vs placebo in the treatment of adult patients with alcohol use disorder: a randomized clinical trial. JAMA Psychiatry 79, 953–962. doi: 10.1001/jamapsychiatry.2022.2096
Bonnieux, J. N., Vanderzwaag, B., Premji, Z., Garcia-Romeu, A., and Garcia-Barrera, M. A. (2023). Psilocybin's effects on cognition and creativity: a scoping review. J. Psychopharmacol. 37, 635–648. doi: 10.1177/02698811231179801
Brown, R. T., Nicholas, C. R., Cozzi, N. V., Gassman, M. C., Cooper, K. M., Muller, D., et al. (2017). Pharmacokinetics of escalating doses of Oral psilocybin in healthy adults. Clin. Pharmacokinet. 56, 1543–1554. doi: 10.1007/s40262-017-0540-6
Burke, J. F., Kerber, K. A., Langa, K. M., Albin, R. L., and Kotagal, V. (2023). Lecanemab: Looking before we leap. Neurology 101, 661–665. doi: 10.1212/WNL.0000000000207505
Burmester, D. R., Madsen, M. K., Szabo, A., Aripaka, S. S., Stenbæk, D. S., Frokjaer, V. G., et al. (2023). Subacute effects of a single dose of psilocybin on biomarkers of inflammation in healthy humans: an open-label preliminary investigation. Compr. Psychoneuroen. 13:100163. doi: 10.1016/j.cpnec.2022.100163
Buzzelli, V., Carbone, E., Manduca, A., Schiavi, S., Feo, A., Perederiy, J. V., et al. (2023). Psilocybin mitigates the cognitive deficits observed in a rat model of fragile X syndrome. Psychopharmacol. 240, 137–147. doi: 10.1007/s00213-022-06286-3
Caldeira, M. V., Melo, C. V., Pereira, D. B., Carvalho, R., Correia, S. S., Backos, D. S., et al. (2007). Brain-derived neurotrophic factor regulates the expression and synaptic delivery of alpha-amino-3-hydroxy-5-methyl-4-isoxazole propionic acid receptor subunits in hippocampal neurons. J. Biol. Chem. 282, 12619–12628. doi: 10.1074/jbc.M700607200
Cao, D., Yu, J., Wang, H., Luo, Z., Liu, X., He, L., et al. (2022). Structure-based discovery of nonhallucinogenic psychedelic analogs. Science 375, 403–411. doi: 10.1126/science.abl8615
Carhart-Harris, R. L., Bolstridge, M., Rucker, J., Day, C. M. J., Erritzoe, D., Kaelen, M., et al. (2016). Psilocybin with psychological support for treatment-resistant depression: an open-label feasibility study. Lancet Psychiatry 3, 619–627. doi: 10.1016/S2215-0366(16)30065-7
Carhart-Harris, R., Giribaldi, B., Watts, R., Baker-Jones, M., Murphy-Beiner, A., Murphy, R., et al. (2021). Trial of psilocybin versus escitalopram for depression. N. Engl. J. Med. 384, 1402–1411. doi: 10.1056/NEJMoa2032994
Carhart-Harris, R. L., Roseman, L., Bolstridge, M., Demetriou, L., Pannekoek, J. N., Wall, M. B., et al. (2017). Psilocybin for treatment-resistant depression: fMRI-measured brain mechanisms. Sci. Rep. 7:13187. doi: 10.1038/s41598-017-13282-7
Carmona, S., Zahs, K., Wu, E., Dakin, K., Bras, J., and Guerreiro, R. (2018). The role of TREM2 in Alzheimer's disease and other neurodegenerative disorders. Lancet Neurol. 17, 721–730. doi: 10.1016/S1474-4422(18)30232-1
Castrén, E., and Hen, R. (2013). Neuronal plasticity and antidepressant actions. Trends Neurosci. 36, 259–267. doi: 10.1016/j.tins.2012.12.010
Catlow, B. J., Song, S., Paredes, D. A., Kirstein, C. L., and Sanchez-Ramos, J. (2013). Effects of psilocybin on hippocampal neurogenesis and extinction of trace fear conditioning. Exp. Brain Res. 228, 481–491. doi: 10.1007/s00221-013-3579-0
Cavanna, F., Muller, S., De La Fuente, L. A., Zamberlan, F., Palmucci, M., Janeckova, L., et al. (2022). Microdosing with psilocybin mushrooms: a double-blind placebo-controlled study. Transl. Psychiatry 12:307. doi: 10.1038/s41398-022-02039-0
Chan, Y.-L. E., Chen, M.-H., Tsai, S.-J., Bai, Y.-M., Tsai, C.-F., Cheng, C.-M., et al. (2020). Treatment-resistant depression enhances risks of dementia and alzheimer's disease: a nationwide longitudinal study. J. Affect. Disord. 274, 806–812. doi: 10.1016/j.jad.2020.05.150
Chang, R. Y. K., Nouwens, A. S., Dodd, P. R., and Etheridge, N. (2013). The synaptic proteome in Alzheimer's disease. Alzheimers Dement. 9, 499–511. doi: 10.1016/j.jalz.2012.04.009
Ching, T. H. W., Grazioplene, R., Bohner, C., Kichuk, S. A., Depalmer, G., D'amico, E., et al. (2023). Safety, tolerability, and clinical and neural effects of single-dose psilocybin in obsessive-compulsive disorder: protocol for a randomized, double-blind, placebo-controlled, non-crossover trial. Front. Psych. 14:1178529. doi: 10.3389/fpsyt.2023.1178529
Davis, A. K., Barrett, F. S., May, D. G., Cosimano, M. P., Sepeda, N. D., Johnson, M. W., et al. (2021). Effects of psilocybin-assisted therapy on major depressive disorder: a randomized clinical trial. JAMA Psychiatry 78, 481–489. doi: 10.1001/jamapsychiatry.2020.3285
Davoudian, P. A., Shao, L.-X., and Kwan, A. C. (2023). Shared and distinct brain regions targeted for immediate early gene expression by ketamine and psilocybin. ACS Chem. Neurosci. 14, 468–480. doi: 10.1021/acschemneuro.2c00637
Daws, R. E., Timmermann, C., Giribaldi, B., Sexton, J. D., Wall, M. B., Erritzoe, D., et al. (2022). Increased global integration in the brain after psilocybin therapy for depression. Nat. Med. 28, 844–851. doi: 10.1038/s41591-022-01744-z
De La Haye, W., Walcott, G., Eaton, J., Beckford, J., and Greene, J. (2023). Psychedelic Assisted Psychotherapy preparing your target using psychohistoriography: a Jamaican perspective. Front. Psyc. 14:1136990. doi: 10.3389/fpsyt.2023.1136990
Dhapola, R., Hota, S. S., Sarma, P., Bhattacharyya, A., Medhi, B., and Reddy, D. H. (2021). Recent advances in molecular pathways and therapeutic implications targeting neuroinflammation for Alzheimer's disease. Inflammopharmacol. 29, 1669–1681. doi: 10.1007/s10787-021-00889-6
Diering, G. H., and Huganir, R. L. (2018). The AMPA receptor code of synaptic plasticity. Neuron 100, 314–329. doi: 10.1016/j.neuron.2018.10.018
Dong, C., Ly, C., Dunlap, L. E., Vargas, M. V., Sun, J., Hwang, I.-W., et al. (2021). Psychedelic-inspired drug discovery using an engineered biosensor. Cell 184, 2779–2792.e18. doi: 10.1016/j.cell.2021.03.043
Donovan, L. L., Johansen, J. V., Ros, N. F., Jaberi, E., Linnet, K., Johansen, S. S., et al. (2021). Effects of a single dose of psilocybin on behaviour, brain 5-HT2A receptor occupancy and gene expression in the pig. Eur. Neuropsychopharmacol. 42, 1–11. doi: 10.1016/j.euroneuro.2020.11.013
Doss, M. K., Barrett, F. S., and Corlett, P. R. (2022). Skepticism about recent evidence that psilocybin "liberates" depressed minds. ACS Chem. Neurosci. 13, 2540–2543. doi: 10.1021/acschemneuro.2c00461
Doss, M. K., Považan, M., Rosenberg, M. D., Sepeda, N. D., Davis, A. K., Finan, P. H., et al. (2021). Psilocybin therapy increases cognitive and neural flexibility in patients with major depressive disorder. Transl. Psychiatry 11:574. doi: 10.1038/s41398-021-01706-y
Du, Y., Li, Y., Zhao, X., Yao, Y., Wang, B., Zhang, L., et al. (2023). Psilocybin facilitates fear extinction in mice by promoting hippocampal neuroplasticity. Chin. Med. J. 136, 2983–2992. doi: 10.1097/CM9.0000000000002647
Dutcher, S. K., Rattinger, G. B., Langenberg, P., Chhabra, P. T., Liu, X., Rosenberg, P. B., et al. (2014). Effect of medications on physical function and cognition in nursing home residents with dementia. J. Am. Geriatr. Soc. 62, 1046–1055. doi: 10.1111/jgs.12838
Effinger, D. P., Quadir, S. G., Ramage, M. C., Cone, M. G., and Herman, M. A. (2023). Sex-specific effects of psychedelic drug exposure on central amygdala reactivity and behavioral responding. Transl. Psychiatry 13:119. doi: 10.1038/s41398-023-02414-5
Espiard, M.-L., Lecardeur, L., Abadie, P., Halbecq, I., and Dollfus, S. (2005). Hallucinogen persisting perception disorder after psilocybin consumption: a case study. Eur. Psychiatry 20, 458–460. doi: 10.1016/j.eurpsy.2005.04.008
Funk, D., Araujo, J., Slassi, M., Lanthier, J., Atkinson, J., Feng, D., et al. (2024). Effect of a single psilocybin treatment on Fos protein expression in male rat brain. Neurosci. 539, 1–11. doi: 10.1016/j.neuroscience.2024.01.001
Gallagher, D., Kiss, A., Lanctot, K., and Herrmann, N. (2018). Depression and risk of Alzheimer dementia: a longitudinal analysis to determine predictors of increased risk among older adults with depression. Am. J. Geriatr. Psychiatry 26, 819–827. doi: 10.1016/j.jagp.2018.05.002
Garcia-Romeu, A., Darcy, S., Jackson, H., White, T., and Rosenberg, P. (2022). Psychedelics as Novel Therapeutics in Alzheimer’s Disease: Rationale and Potential Mechanisms. Curr. Topics Behav. Neurosci. 56, 287–317. doi: 10.1007/7854_2021_267
Gauthier, S., Leuzy, A., Racine, E., and Rosa-Neto, P. (2013). Diagnosis and management of Alzheimer's disease: past, present and future ethical issues. Prog. Neurobiol. 110, 102–113. doi: 10.1016/j.pneurobio.2013.01.003
Glatfelter, G. C., Naeem, M., Pham, D. N. K., Golen, J. A., Chadeayne, A. R., Manke, D. R., et al. (2023). Receptor binding profiles for tryptamine psychedelics and effects of 4-Propionoxy-N, N-dimethyltryptamine in mice. ACS Pharmacol. Trans. Sci. 6, 567–577. doi: 10.1021/acsptsci.2c00222
Glymour, M. M., Weuve, J., Dufouil, C., and Mayeda, E. R. (2022). Aduhelm, the newly approved medication for Alzheimer disease: what epidemiologists Can learn and what epidemiology Can offer. Am. J. Epidemiol. 191, 1347–1351. doi: 10.1093/aje/kwac063
González-Maeso, J., Yuen, T., Ebersole, B. J., Wurmbach, E., Lira, A., Zhou, M., et al. (2003). Transcriptome fingerprints distinguish hallucinogenic and nonhallucinogenic 5-hydroxytryptamine 2A receptor agonist effects in mouse somatosensory cortex. J. Neurosci. 23, 8836–8843. doi: 10.1523/JNEUROSCI.23-26-08836.2003
Goodwin, G. M., Aaronson, S. T., Alvarez, O., Arden, P. C., Baker, A., Bennett, J. C., et al. (2022). Single-dose psilocybin for a treatment-resistant episode of major depression. N. Engl. J. Med. 387, 1637–1648. doi: 10.1056/NEJMoa2206443
Goodwin, G. M., Aaronson, S. T., Alvarez, O., Atli, M., Bennett, J. C., Croal, M., et al. (2023a). Single-dose psilocybin for a treatment-resistant episode of major depression: impact on patient-reported depression severity, anxiety, function, and quality of life. J. Affect. Disord. 327, 120–127. doi: 10.1016/j.jad.2023.01.108
Goodwin, G. M., Croal, M., Feifel, D., Kelly, J. R., Marwood, L., Mistry, S., et al. (2023b). Psilocybin for treatment resistant depression in patients taking a concomitant SSRI medication. Neuropsychopharmacology: official publication of the American college of. Neuropsychopharmacology 48, 1492–1499. doi: 10.1038/s41386-023-01648-7
Gorgulla, C., Boeszoermenyi, A., Wang, Z.-F., Fischer, P. D., Coote, P. W., Padmanabha Das, K. M., et al. (2020). An open-source drug discovery platform enables ultra-large virtual screens. Nature 580, 663–668. doi: 10.1038/s41586-020-2117-z
Griffiths, R. R., Johnson, M. W., Richards, W. A., Richards, B. D., Mccann, U., and Jesse, R. (2011). Psilocybin occasioned mystical-type experiences: immediate and persisting dose-related effects. Psychopharmacology 218, 649–665. doi: 10.1007/s00213-011-2358-5
Griffiths, R. R., Johnson, M. W., Carducci, M. A., Umbricht, A., Richards, W. A., Richards, B. D., et al. (2016). Psilocybin produces substantial and sustained decreases in depression and anxiety in patients with life-threatening cancer: a randomized double-blind trial. J. Psychopharmacol. 30, 1181–1197. doi: 10.1177/0269881116675513
Gukasyan, N. (2023). On blinding and suicide risk in a recent trial of psilocybin-assisted therapy for treatment-resistant depression. Med 4, 8–9. doi: 10.1016/j.medj.2022.12.003
Gukasyan, N., Davis, A. K., Barrett, F. S., Cosimano, M. P., Sepeda, N. D., Johnson, M. W., et al. (2022). Efficacy and safety of psilocybin-assisted treatment for major depressive disorder: prospective 12-month follow-up. J. Psychopharmacol. 36, 151–158. doi: 10.1177/02698811211073759
Halim, H. J., Burk, B. G., Fargason, R. E., and Birur, B. (2023). Manic episode following psilocybin use in a man with bipolar II disorder: a case report. Front. Psych. 14:1221131. doi: 10.3389/fpsyt.2023.1221131
Haridy, R. (2023). Australia to prescribe MDMA and psilocybin for PTSD and depression in world first. Nature 619, 227–228. doi: 10.1038/d41586-023-02093-8
Harerimana, N. V., Liu, Y., Gerasimov, E. S., Duong, D., Beach, T. G., Reiman, E. M., et al. (2022). Genetic evidence supporting a causal role of depression in Alzheimer's disease. Biol. Psych. 92, 25–33. doi: 10.1016/j.biopsych.2021.11.025
Hasler, F., Bourquin, D., Brenneisen, R., Bär, T., and Vollenweider, F. X. (1997). Determination of psilocin and 4-hydroxyindole-3-acetic acid in plasma by HPLC-ECD and pharmacokinetic profiles of oral and intravenous psilocybin in man. Pharm. Acta Helv. 72, 175–184. doi: 10.1016/S0031-6865(97)00014-9
Hasler, F., Bourquin, D., Brenneisen, R., and Vollenweider, F. X. (2002). Renal excretion profiles of psilocin following oral administration of psilocybin: a controlled study in man. J. Pharm. Biomed. Anal. 30, 331–339. doi: 10.1016/S0731-7085(02)00278-9
Hendin, H. M., and Penn, A. D. (2021). An episode of mania following self-reported ingestion of psilocybin mushrooms in a woman previously not diagnosed with bipolar disorder: a case report. Bipolar Disord. 23, 733–735. doi: 10.1111/bdi.13095
Heneka, M. T., Kummer, M. P., Stutz, A., Delekate, A., Schwartz, S., Vieira-Saecker, A., et al. (2013). NLRP3 is activated in Alzheimer's disease and contributes to pathology in APP/PS1 mice. Nat. 493, 674–678. doi: 10.1038/nature11729
Hesselgrave, N., Troppoli, T. A., Wulff, A. B., Cole, A. B., and Thompson, S. M. (2021). Harnessing psilocybin: antidepressant-like behavioral and synaptic actions of psilocybin are independent of 5-HT2R activation in mice. Proc. Natl. Acad. Sci. USA 118:9118. doi: 10.1073/pnas.2022489118
Higgins, G. A., Carroll, N. K., Brown, M., Macmillan, C., Silenieks, L. B., Thevarkunnel, S., et al. (2021). Low doses of psilocybin and ketamine enhance motivation and attention in poor performing rats: evidence for an antidepressant property. Front. Pharmacol. 12:640241. doi: 10.3389/fphar.2021.640241
High, D. M. (1992). Research with Alzheimer’s disease subjects: informed consent and proxy decision making. J. American Geriatrics Soc. 40, 950–957.
Holze, F., Becker, A. M., Kolaczynska, K. E., Duthaler, U., and Liechti, M. E. (2023). Pharmacokinetics and pharmacodynamics of Oral psilocybin Administration in Healthy Participants. Clin. Pharmacol. Ther. 113, 822–831. doi: 10.1002/cpt.2821
Holze, F., Ley, L., Müller, F., Becker, A. M., Straumann, I., Vizeli, P., et al. (2022). Direct comparison of the acute effects of lysergic acid diethylamide and psilocybin in a double-blind placebo-controlled study in healthy subjects. Neuropsychopharmacology: official publication of the American college of. Neuropsychopharmacol. 47, 1180–1187. doi: 10.1038/s41386-022-01297-2
Honma, M., Kuroda, T., Futamura, A., Sugimoto, A., and Kawamura, M. (2015). Mental time dysfunction in Parkinson’s and Alzheimer’s diseases. Brain and Nerve = Shinkei Kenkyu No Shinpo 67, 297–302. doi: 10.11477/mf.1416200135
Horita, A., and Weber, L. J. (1961a). Dephosphorylation of psilocybin to psilocin by alkaline phosphatase. Proc. Soc. Exp. Biol. Med. 106, 32–34. doi: 10.3181/00379727-106-26228
Horita, A., and Weber, L. J. (1961b). The enzymic dephosphorylation and oxidation of psilocybin and psilocin by mammalian tissue homogenates. Biochem. Pharmacol. 7, 47–54. doi: 10.1016/0006-2952(61)90124-1
Horita, A., and Weber, L. J. (1962). Dephosphorylation of psilocybin in the intact mouse. Toxicol. Appl. Pharmacol. 4, 730–737. doi: 10.1016/0041-008X(62)90102-3
Ising, C., Venegas, C., Zhang, S., Scheiblich, H., Schmidt, S. V., Vieira-Saecker, A., et al. (2019). NLRP3 inflammasome activation drives tau pathology. Nat. 575, 669–673. doi: 10.1038/s41586-019-1769-z
Jaworski, J., and Sheng, M. (2006). The growing role of mTOR in neuronal development and plasticity. Mol. Neurobiol. 34, 205–220. doi: 10.1385/MN:34:3:205
Jefsen, O. H., Elfving, B., Wegener, G., and Müller, H. K. (2021). Transcriptional regulation in the rat prefrontal cortex and hippocampus after a single administration of psilocybin. J. Psychopharm. 35, 483–493. doi: 10.1177/0269881120959614
Jiao, S. S., Shen, L. L., Zhu, C., Bu, X. L., Liu, Y. H., Liu, C. H., et al. (2016). Brain-derived neurotrophic factor protects against tau-related neurodegeneration of Alzheimer's disease. Transl. Psych. 6:e907. doi: 10.1038/tp.2016.186
Johansson, M., Stomrud, E., Lindberg, O., Westman, E., Johansson, P. M., Van Westen, D., et al. (2020). Apathy and anxiety are early markers of Alzheimer's disease. Neurobiol. Aging 85, 74–82. doi: 10.1016/j.neurobiolaging.2019.10.008
Jones, N. T., Zahid, Z., Grady, S. M., Sultan, Z. W., Zheng, Z., Razidlo, J., et al. (2023). Transient elevation of plasma glucocorticoids supports psilocybin-induced Anxiolysis in mice. ACS Pharmacol. Trans. Sci. 6, 1221–1231. doi: 10.1021/acsptsci.3c00123
Kalberer, F., Kreis, W., and Rutschmann, J. (1962). The fate of psilocin in the rat. Biochem. Pharmacol. 11, 261–269. doi: 10.1016/0006-2952(62)90050-3
Kamata, T., Katagi, M., Kamata, H. T., Miki, A., Shima, N., Zaitsu, K., et al. (2006). Metabolism of the psychotomimetic tryptamine derivative 5-methoxy-NN-diisopropyltryptamine in humans: identification and quantification of its urinary metabolites. Drug Metab. Dispos. 34, 281–287. doi: 10.1124/dmd.105.005835
Kaplan, A. L., Confair, D. N., Kim, K., Barros-Álvarez, X., Rodriguiz, R. M., Yang, Y., et al. (2022). Bespoke library docking for 5-HT2A receptor agonists with antidepressant activity. Nature 610, 582–591. doi: 10.1038/s41586-022-05258-z
Kim, H., Jeong, W., Kwon, J., Kim, Y., Park, E.-C., and Jang, S.-I. (2021). Association between depression and the risk of Alzheimer's disease using the Korean National Health Insurance Service-elderly cohort. Sci. Rep. 11:22591. doi: 10.1038/s41598-021-02201-6
Kim, D., Wang, R., Kiss, A., Bronskill, S. E., Lanctot, K. L., Herrmann, N., et al. (2021). Depression and increased risk of Alzheimer's dementia: longitudinal analyses of modifiable risk and sex-related factors. Am. J. Geriatr. Psychiatry 29, 917–926. doi: 10.1016/j.jagp.2020.12.031
Kinderlehrer, D. A. (2023). The effectiveness of microdosed psilocybin in the treatment of neuropsychiatric Lyme disease: a case study. Int. Med. Case Rep. J. 16, 109–115. doi: 10.2147/IMCRJ.S395342
Koch, I., Poljac, E., Müller, H., and Kiesel, A. (2018). Cognitive structure, flexibility, and plasticity in human multitasking – an integrative review of dual-task and task-switching research. Psychol. Bull. 144, 557–583. doi: 10.1037/bul0000144
Kolaczynska, K. E., Liechti, M. E., and Duthaler, U. (2021). Development and validation of an LC-MS/MS method for the bioanalysis of psilocybin's main metabolites, psilocin and 4-hydroxyindole-3-acetic acid, in human plasma. J. Chromatogr. B Analyt. Technol. Biomed. Life Sci. 1164:122486. doi: 10.1016/j.jchromb.2020.122486
Kometer, M., Schmidt, A., Bachmann, R., Studerus, E., Seifritz, E., and Vollenweider, F. X. (2012). Psilocybin biases facial recognition, goal-directed behavior, and mood state toward positive relative to negative emotions through different serotonergic subreceptors. Biol. Psych. 72, 898–906. doi: 10.1016/j.biopsych.2012.04.005
Kotts, W. J., Gamble, D. T., Dawson, D. K., and Connor, D. (2022). Psilocybin-induced takotsubo cardiomyopathy. BMJ Case Rep. 15:e245863. doi: 10.1136/bcr-2021-245863
Kozłowska, U., Klimczak, A., Wiatr, K., and Figiel, M. (2021). The DMT and psilocin treatment changes CD11b+ activated microglia immunological phenotype. BioRXiv 2021:4103. doi: 10.1101/2021.03.07.434103
Kozlowska, U., Nichols, C., Wiatr, K., and Figiel, M. (2022). From psychiatry to neurology: Psychedelics as prospective therapeutics for neurodegenerative disorders. J. Neurochem. 162. doi: 10.1111/jnc.15509
Kraehenmann, R., Preller, K. H., Scheidegger, M., Pokorny, T., Bosch, O. G., Seifritz, E., et al. (2015). Psilocybin-induced decrease in amygdala reactivity correlates with enhanced positive mood in healthy volunteers. Biol. Psych. 78, 572–581. doi: 10.1016/j.biopsych.2014.04.010
Kuring, J. K., Mathias, J. L., and Ward, L. (2020). Risk of dementia in persons who have previously experienced clinically-significant depression, anxiety, or PTSD: a systematic review and meta-analysis. J. Affect. Disord. 274, 247–261. doi: 10.1016/j.jad.2020.05.020
Laabi, S., Lemmon, C., Vogel, C., Chacon, M., and Jimenez, V. M. (2024). Deciphering psilocybin: cytotoxicity, anti-inflammatory effects, and mechanistic insights. Int. Immunopharmacol. 130:111753. doi: 10.1016/j.intimp.2024.111753
Lawrence, V., Pickett, J., Ballard, C., and Murray, J. (2014). Patient and carer views on participating in clinical trials for prodromal Alzheimer's disease and mild cognitive impairment. Int. J. Geriatr. Psych. 29, 22–31. doi: 10.1002/gps.3958
Lea, T., Amada, N., Jungaberle, H., Schecke, H., and Klein, M. (2020). Microdosing psychedelics: motivations, subjective effects and harm reduction. Int. J. Drug Policy 75:102600. doi: 10.1016/j.drugpo.2019.11.008
Lee, C. H., Kim, D. H., and Moon, Y. S. (2019). Differential associations between depression and cognitive function in MCI and AD: a cross-sectional study. Int. Psychogeriatr. 31, 1151–1158. doi: 10.1017/S1041610218001527
Lenz, C., Wick, J., Braga, D., García-Altares, M., Lackner, G., Hertweck, C., et al. (2020). Injury-triggered blueing reactions of psilocybe "magic" mushrooms. Angew. Chem. Int. Ed Engl. 59, 1450–1454. doi: 10.1002/anie.201910175
Lewis, B. R., Garland, E. L., Byrne, K., Durns, T., Hendrick, J., Beck, A., et al. (2023). HOPE: a pilot study of psilocybin enhanced group psychotherapy in patients with Cancer. J. Pain Symptom Manag. 66, 258–269. doi: 10.1016/j.jpainsymman.2023.06.006
Lewis, C. R., Preller, K. H., Kraehenmann, R., Michels, L., Staempfli, P., and Vollenweider, F. X. (2017). Two dose investigation of the 5-HT-agonist psilocybin on relative and global cerebral blood flow. NeuroImage 159, 70–78. doi: 10.1016/j.neuroimage.2017.07.020
Ley, L., Holze, F., Arikci, D., Becker, A. M., Straumann, I., Klaiber, A., et al. (2023). Comparative acute effects of mescaline, lysergic acid diethylamide, and psilocybin in a randomized, double-blind, placebo-controlled cross-over study in healthy participants. Neuropsychopharm. 48, 1659–1667. doi: 10.1038/s41386-023-01607-2
Li, R., Shen, F., Sun, X., Zou, T., Li, L., Wang, X., et al. (2023). Dissociable salience and default mode network modulation in generalized anxiety disorder: a connectome-wide association study. Cerebral Cortex 33, 6354–6365. doi: 10.1093/cercor/bhac509
Lim, B., Prassas, I., and Diamandis, E. P. (2021). Alzheimer disease pathogenesis: the role of autoimmunity. J. Appl. Lab. Med. 6, 756–764. doi: 10.1093/jalm/jfaa171
Lindenblatt, H., Krämer, E., Holzmann-Erens, P., Gouzoulis-Mayfrank, E., and Kovar, K. A. (1998). Quantitation of psilocin in human plasma by high-performance liquid chromatography and electrochemical detection: comparison of liquid-liquid extraction with automated on-line solid-phase extraction. J. Chromatogr. B Biomed. Sci. Appl. 709, 255–263. doi: 10.1016/S0378-4347(98)00067-X
Liu, X., Zhu, H., Gao, H., Tian, X., Tan, B., and Su, R. (2022). GS signaling pathway distinguishes hallucinogenic and nonhallucinogenic 5-HT2A receptor agonists induced head twitch response in mice. Biochem. Biophys. Res. Commun. 598, 20–25. doi: 10.1016/j.bbrc.2022.01.113
Ly, C., Greb, A. C., Cameron, L. P., Wong, J. M., Barragan, E. V., Wilson, P. C., et al. (2018). Psychedelics promote structural and functional neural plasticity. Cell Rep. 23, 3170–3182. doi: 10.1016/j.celrep.2018.05.022
Lyes, M., Yang, K. H., Castellanos, J., and Furnish, T. (2023). Microdosing psilocybin for chronic pain: a case series. Pain 164, 698–702. doi: 10.1097/j.pain.0000000000002778
Ma, L. (2020). Depression, anxiety, and apathy in mild cognitive impairment: current perspectives. Front. Aging Neurosci. 12:9. doi: 10.3389/fnagi.2020.00009
Madsen, M. K., Fisher, P. M., Burmester, D., Dyssegaard, A., Stenbæk, D. S., Kristiansen, S., et al. (2019). Psychedelic effects of psilocybin correlate with serotonin 2A receptor occupancy and plasma psilocin levels. Neuropsychopharmacol. 44, 1328–1334. doi: 10.1038/s41386-019-0324-9
Madsen, M. K., Petersen, A. S., Stenbaek, D. S., Sørensen, I. M., Schiønning, H., Fjeld, T., et al. (2024). CCH attack frequency reduction after psilocybin correlates with hypothalamic functional connectivity. Headache 64, 55–67. doi: 10.1111/head.14656
Manevski, N., Kurkela, M., Höglund, C., Mauriala, T., Court, M. H., Yli-Kauhaluoma, J., et al. (2010). Glucuronidation of psilocin and 4-hydroxyindole by the human UDP-glucuronosyltransferases. Drug Metab. Dispos. 38, 386–395. doi: 10.1124/dmd.109.031138
Marschall, J., Fejer, G., Lempe, P., Prochazkova, L., Kuchar, M., Hajkova, K., et al. (2022). Psilocybin microdosing does not affect emotion-related symptoms and processing: a preregistered field and lab-based study. J. Psychopharmacol. 36, 97–113. doi: 10.1177/02698811211050556
Martín-Sánchez, A., Piñero, J., Nonell, L., Arnal, M., Ribe, E. M., Nevado-Holgado, A., et al. (2021). Comorbidity between Alzheimer's disease and major depression: a behavioural and transcriptomic characterization study in mice. Alzheimers Res. Ther. 13:73. doi: 10.1186/s13195-021-00810-x
Mason, N. L., Kuypers, K. P. C., Reckweg, J. T., Müller, F., Tse, D. H. Y., Da Rios, B., et al. (2021). Spontaneous and deliberate creative cognition during and after psilocybin exposure. Transl. Psychiatry 11:209. doi: 10.1038/s41398-021-01335-5
Mason, N. L., Mischler, E., Uthaug, M. V., and Kuypers, K. P. C. (2019). Sub-acute effects of psilocybin on empathy, creative thinking, and subjective well-being. J. Psychoactive Drugs 51, 123–134. doi: 10.1080/02791072.2019.1580804
Mason, N. L., Szabo, A., Kuypers, K. P. C., Mallaroni, P. A., De La Torre, F. R., Reckweg, J. T., et al. (2023). Psilocybin induces acute and persisting alterations in immune status in healthy volunteers: an experimental, placebo-controlled study. Brain Behav. Immun. 114, 299–310. doi: 10.1016/j.bbi.2023.09.004
Matsumoto, T., Numakawa, T., Adachi, N., Yokomaku, D., Yamagishi, S., Takei, N., et al. (2001). Brain-derived neurotrophic factor enhances depolarization-evoked glutamate release in cultured cortical neurons. J. Neurochem. 79, 522–530. doi: 10.1046/j.1471-4159.2001.00591.x
Mazzi, C., Massironi, G., Sanchez-Lopez, J., De Togni, L., and Savazzi, S. (2020). Face recognition deficits in a patient with Alzheimer's disease: amnesia or agnosia? The importance of electrophysiological markers for differential diagnosis. Front. Aging Neurosci. 12:580609. doi: 10.3389/fnagi.2020.580609
Mazzo, F., Butnaru, I., Grubisha, O., Ficulle, E., Sanger, H., Fitzgerald, G., et al. (2022). Metabotropic glutamate receptors modulate Exocytotic tau release and propagation. J. Pharmacol. Exp. Ther. 383, 117–128. doi: 10.1124/jpet.122.001307
Mecca, A. P., O'dell, R. S., Sharp, E. S., Banks, E. R., Bartlett, H. H., Zhao, W., et al. (2022). Synaptic density and cognitive performance in Alzheimer's disease: a PET imaging study with [11 C]UCB-J. Alzheimers Dement. 18, 2527–2536. doi: 10.1002/alz.12582
Meinhardt, M. W., Pfarr, S., Fouquet, G., Rohleder, C., Meinhardt, M. L., Barroso-Flores, J., et al. (2021). Psilocybin targets a common molecular mechanism for cognitive impairment and increased craving in alcoholism. Science. Advances 7:eabh2399. doi: 10.1126/sciadv.abh2399
Mertens, L. J., Wall, M. B., Roseman, L., Demetriou, L., Nutt, D. J., and Carhart-Harris, R. L. (2020). Therapeutic mechanisms of psilocybin: changes in amygdala and prefrontal functional connectivity during emotional processing after psilocybin for treatment-resistant depression. J. Psychopharmacol. 34, 167–180. doi: 10.1177/0269881119895520
Mocanu, V., Mackay, L., Christie, D., and Argento, E. (2022). Safety considerations in the evolving legal landscape of psychedelic-assisted psychotherapy. Substance Abuse Treatment, Prevention, and Policy 17:37. doi: 10.1186/s13011-022-00468-0
Moliner, R., Girych, M., Brunello, C. A., Kovaleva, V., Biojone, C., Enkavi, G., et al. (2023). Psychedelics promote plasticity by directly binding to BDNF receptor TrkB. Nat. Neurosci. 26, 1032–1041. doi: 10.1038/s41593-023-01316-5
Monereo-Sánchez, J., Schram, M. T., Frei, O., O'connell, K., Shadrin, A. A., Smeland, O. B., et al. (2021). Genetic overlap between Alzheimer's disease and depression mapped onto the brain. Front. Neurosci. 15:653130. doi: 10.3389/fnins.2021.653130
Moreno, F. A., Wiegand, C. B., Taitano, E. K., and Delgado, P. L. (2006). Safety, tolerability, and efficacy of psilocybin in 9 patients with obsessive-compulsive disorder. J. Clin. Psychiatry 67, 1735–1740. doi: 10.4088/JCP.v67n1110
Moreno-Jiménez, E. P., Flor-García, M., Terreros-Roncal, J., Rábano, A., Cafini, F., Pallas-Bazarra, N., et al. (2019). Adult hippocampal neurogenesis is abundant in neurologically healthy subjects and drops sharply in patients with Alzheimer's disease. Nat. Med. 25, 554–560. doi: 10.1038/s41591-019-0375-9
Müller, F., Kraus, E., Holze, F., Becker, A., Ley, L., Schmid, Y., et al. (2022). Flashback phenomena after administration of LSD and psilocybin in controlled studies with healthy participants. Psychopharmacol. 239, 1933–1943. doi: 10.1007/s00213-022-06066-z
Nayak, S. M., Jackson, H., Sepeda, N. D., Mathai, D. S., So, S., Yaffe, A., et al. (2023). Naturalistic psilocybin use is associated with persisting improvements in mental health and wellbeing: results from a prospective, longitudinal survey. Front. Psych. 14:1199642. doi: 10.3389/fpsyt.2023.1199642
Nef, H. M., Möllmann, H., Hilpert, P., Krause, N., Troidl, C., Weber, M., et al. (2009). Apical regional wall motion abnormalities reminiscent to Tako-Tsubo cardiomyopathy following consumption of psychoactive fungi. Int. J. Cardiol. 134, e39–e41. doi: 10.1016/j.ijcard.2007.12.064
Nkadimeng, S. M., Nabatanzi, A., Steinmann, C. M. L., and Eloff, J. N. (2020a). Phytochemical, cytotoxicity, antioxidant and anti-inflammatory effects of psilocybe natalensis magic mushroom. Plants 9:1127. doi: 10.3390/plants9091127
Nkadimeng, S. M., Steinmann, C. M. L., and Eloff, J. N. (2020b). Effects and safety of Psilocybe cubensis and Panaeolus cyanescens magic mushroom extracts on endothelin-1-induced hypertrophy and cell injury in cardiomyocytes. Sci. Rep. 10:22314. doi: 10.1038/s41598-020-79328-5
Nkadimeng, S. M., Steinmann, C. M. L., and Eloff, J. N. (2021). Anti-inflammatory effects of four psilocybin-containing magic mushroom water extracts in vitro on 15-lipoxygenase activity and on lipopolysaccharide-induced Cyclooxygenase-2 and inflammatory cytokines in human U937 macrophage cells. J. Inflamm. Res. 14, 3729–3738. doi: 10.2147/JIR.S317182
Numakawa, T., Yamagishi, S., Adachi, N., Matsumoto, T., Yokomaku, D., Yamada, M., et al. (2002). Brain-derived neurotrophic factor-induced potentiation of ca(2+) oscillations in developing cortical neurons. J. Biol. Chem. 277, 6520–6529. doi: 10.1074/jbc.M109139200
Ortiz, C. E., Dourron, H. M., Sweat, N. W., Garcia-Romeu, A., Maccarthy, S., Anderson, B. T., et al. (2022). Special considerations for evaluating psilocybin-facilitated psychotherapy in vulnerable populations. Neuropharmacol. 214:109127. doi: 10.1016/j.neuropharm.2022.109127
Pagni, B. A., Petridis, P. D., Podrebarac, S. K., Grinband, J., Claus, E. D., and Bogenschutz, M. P. (2024). Psilocybin-induced changes in neural reactivity to alcohol and emotional cues in patients with alcohol use disorder: an fMRI pilot study. Sci. Rep. 14:3159. doi: 10.1038/s41598-024-52967-8
Peck, S. K., Shao, S., Gruen, T., Yang, K., Babakanian, A., Trim, J., et al. (2023). Psilocybin therapy for females with anorexia nervosa: a phase 1, open-label feasibility study. Nat. Med. 29, 1947–1953. doi: 10.1038/s41591-023-02455-9
Pellegrino, T. C., and Bayer, B. M. (2002). Role of central 5-HT(2) receptors in fluoxetine-induced decreases in T lymphocyte activity. Brain Behav. Immun. 16, 87–103. doi: 10.1006/brbi.2001.0625
Pelowski, M., Spee, B. T. M., Arato, J., Dörflinger, F., Ishizu, T., and Richard, A. (2022). Can we really 'read' art to see the changing brain? A review and empirical assessment of clinical case reports and published artworks for systematic evidence of quality and style changes linked to damage or neurodegenerative disease. Phys Life Rev 43, 32–95. doi: 10.1016/j.plrev.2022.07.005
Perez, N., Langlest, F., Mallet, L., De Pieri, M., Sentissi, O., Thorens, G., et al. (2023). Psilocybin-assisted therapy for depression: a systematic review and dose-response meta-analysis of human studies. Eur. Neuropsychopharmacol. 76, 61–76. doi: 10.1016/j.euroneuro.2023.07.011
Perez-Garcia, G., Bicak, M., Haure-Mirande, J.-V., Perez, G. M., Otero-Pagan, A., Gama Sosa, M. A., et al. (2023). BCI-838, an orally active mGluR2/3 receptor antagonist pro-drug, rescues learning behavior deficits in the PS19 MAPTP301S mouse model of tauopathy. Neurosci. Lett. 797:137080. doi: 10.1016/j.neulet.2023.137080
Piancatelli, D., Aureli, A., Sebastiani, P., Colanardi, A., Del Beato, T., Del Cane, L., et al. (2022). Gene- and gender-related decrease in serum BDNF levels in Alzheimer's disease. Int. J. Mol. Sci. 23:4599. doi: 10.3390/ijms232314599
Pilozzi, A., Foster, S., Mischoulon, D., Fava, M., and Huang, X. (2023). A Brief Review on the Potential of Psychedelics for Treating Alzheimer’s Disease and Related Depression. Intern. J. Molec. Sci. 24. doi: 10.3390/ijms241512513
Pokorny, T., Preller, K. H., Kraehenmann, R., and Vollenweider, F. X. (2016). Modulatory effect of the 5-HT1A agonist buspirone and the mixed non-hallucinogenic 5-HT1A/2A agonist ergotamine on psilocybin-induced psychedelic experience. Eur. Neuropsychopharmacol. 26, 756–766. doi: 10.1016/j.euroneuro.2016.01.005
Preller, K. H., Duerler, P., Burt, J. B., Ji, J. L., Adkinson, B., Stämpfli, P., et al. (2020). Psilocybin induces time-dependent changes in global functional connectivity. Biol. Psychiatry 88, 197–207. doi: 10.1016/j.biopsych.2019.12.027
Qin, M, Wu, J, Zhou, Q, and Liang, Z, Su Y (2022). Corrigendum to “Global cognitive effects of secondgeneration antidepressants in patients with Alzheimer’s disease: A systematic review and metaanalysisof randomized controlled trials” [J. Psychiatr. Res. 155 (2022) 371-379]. J. Psychi. Res. 156:44 doi: 10.1016/j.jpsychires.2022.10.030
Raison, C. L., Sanacora, G., Woolley, J., Heinzerling, K., Dunlop, B. W., Brown, R. T., et al. (2023). Single-dose psilocybin treatment for major depressive disorder: a randomized clinical trial. JAMA 330, 843–853. doi: 10.1001/jama.2023.14530
Raithatha, S. A., Hagel, J. M., Matinkhoo, K., Yu, L., Press, D., Cook, S. G., et al. (2023). Novel psilocin prodrugs with altered pharmacological properties as candidate therapies for treatment-resistant anxiety disorders. J. Med. Chem. 67, 1024–1043. doi: 10.1021/acs.jmedchem.3c01225
Rapaka, D., Bitra, V. R., Challa, S. R., and Adiukwu, P. C. (2022). mTOR signaling as a molecular target for the alleviation of Alzheimer's disease pathogenesis. Neurochem. Int. 155:105311. doi: 10.1016/j.neuint.2022.105311
Raval, N. R., Johansen, A., Donovan, L. L., Ros, N. F., Ozenne, B., Hansen, H. D., et al. (2021). A single dose of psilocybin increases synaptic density and decreases 5-HT2A receptor density in the pig brain. Int. J. Mol. Sci. 22:835. doi: 10.3390/ijms22020835
Robinson, G. I., Li, D., Wang, B., Rahman, T., Gerasymchuk, M., Hudson, D., et al. (2023). Psilocybin and eugenol reduce inflammation in human 3D EpiIntestinal tissue. Life 13:2345. doi: 10.3390/life13122345
Rootman, J. M., Kiraga, M., Kryskow, P., Harvey, K., Stamets, P., Santos-Brault, E., et al. (2022). Psilocybin microdosers demonstrate greater observed improvements in mood and mental health at one month relative to non-microdosing controls. Sci. Rep. 12:11091. doi: 10.1038/s41598-022-14512-3
Rootman, J. M., Kryskow, P., Harvey, K., Stamets, P., Santos-Brault, E., Kuypers, K. P. C., et al. (2021). Adults who microdose psychedelics report health related motivations and lower levels of anxiety and depression compared to non-microdosers. Sci. Rep. 11:22479. doi: 10.1038/s41598-021-01811-4
Roseman, L., Demetriou, L., Wall, M. B., Nutt, D. J., and Carhart-Harris, R. L. (2018). Increased amygdala responses to emotional faces after psilocybin for treatment-resistant depression. Neuropharmacology 142, 263–269. doi: 10.1016/j.neuropharm.2017.12.041
Rosenblat, J. D., Meshkat, S., Doyle, Z., Kaczmarek, E., Brudner, R. M., Kratiuk, K., et al. (2024). Psilocybin-assisted psychotherapy for treatment resistant depression: a randomized clinical trial evaluating repeated doses of psilocybin. Med 5:5. doi: 10.1016/j.medj.2024.01.005
Ross, S., Bossis, A., Guss, J., Agin-Liebes, G., Malone, T., Cohen, B., et al. (2016). Rapid and sustained symptom reduction following psilocybin treatment for anxiety and depression in patients with life-threatening cancer: a randomized controlled trial. J. Psychopharmacol. 30, 1165–1180. doi: 10.1177/0269881116675512
Ross, S. D., Lachmann, T., Jaarsveld, S., Riedel-Heller, S. G., and Rodriguez, F. S. (2023). Creativity across the lifespan: changes with age and with dementia. BMC Geriatr. 23:160. doi: 10.1186/s12877-023-03825-1
Roth, B. L., and Gumpper, R. H. (2023). Psychedelics as transformative therapeutics. Am. J. Psychiatry 180, 340–347. doi: 10.1176/appi.ajp.20230172
Rucker, J. J. H. (2015). Psychedelic drugs should be legally reclassified so that researchers can investigate their therapeutic potential. BMJ (Clinical Research ed.) 350:h2902. doi: 10.1136/bmj.h2902
Rucker, J. J., Marwood, L., Ajantaival, R.-L. J., Bird, C., Eriksson, H., Harrison, J., et al. (2022). The effects of psilocybin on cognitive and emotional functions in healthy participants: results from a phase 1, randomised, placebo-controlled trial involving simultaneous psilocybin administration and preparation. J. Psychopharmacol. 36, 114–125. doi: 10.1177/02698811211064720
Ruthirakuhan, M., Herrmann, N., Vieira, D., Gallagher, D., and Lanctôt, K. L. (2019). The roles of apathy and depression in predicting Alzheimer disease: a longitudinal analysis in older adults with mild cognitive impairment. Am. J. Geriatr. Psychiatry 27, 873–882. doi: 10.1016/j.jagp.2019.02.003
Salloway, S., Chalkias, S., Barkhof, F., Burkett, P., Barakos, J., Purcell, D., et al. (2022). Amyloid-related imaging abnormalities in 2 phase 3 studies evaluating Aducanumab in patients with early Alzheimer disease. JAMA Neurol. 79, 13–21. doi: 10.1001/jamaneurol.2021.4161
Scheff, S. W., Ansari, M. A., and Mufson, E. J. (2016). Oxidative stress and hippocampal synaptic protein levels in elderly cognitively intact individuals with Alzheimer's disease pathology. Neurobiol. Aging 42, 1–12. doi: 10.1016/j.neurobiolaging.2016.02.030
Scheltens, P., De Strooper, B., Kivipelto, M., Holstege, H., Chételat, G., Teunissen, C. E., et al. (2021). Alzheimer's disease. Lancet 397, 1577–1590. doi: 10.1016/S0140-6736(20)32205-4
Schindler, E. A. D., Sewell, R. A., Gottschalk, C. H., Flynn, L. T., Zhu, Y., Pittman, B. P., et al. (2024). Psilocybin pulse regimen reduces cluster headache attack frequency in the blinded extension phase of a randomized controlled trial. J. Neurol. Sci. 460:122993. doi: 10.1016/j.jns.2024.122993
Schindler, E. A. D., Sewell, R. A., Gottschalk, C. H., Luddy, C., Flynn, L. T., Lindsey, H., et al. (2021). Exploratory controlled study of the migraine-suppressing effects of psilocybin. Neurotherapeutics 18, 534–543. doi: 10.1007/s13311-020-00962-y
Schindler, E. A. D., Sewell, R. A., Gottschalk, C. H., Luddy, C., Flynn, L. T., Zhu, Y., et al. (2022). Exploratory investigation of a patient-informed low-dose psilocybin pulse regimen in the suppression of cluster headache: results from a randomized, double-blind, placebo-controlled trial. Headache 62, 1383–1394. doi: 10.1111/head.14420
Schneier, F. R., Feusner, J., Wheaton, M. G., Gomez, G. J., Cornejo, G., Naraindas, A. M., et al. (2023). Pilot study of single-dose psilocybin for serotonin reuptake inhibitor-resistant body dysmorphic disorder. J. Psychiatr. Res. 161, 364–370. doi: 10.1016/j.jpsychires.2023.03.031
Shahar, O., Botvinnik, A., Esh-Zuntz, N., Brownstien, M., Wolf, R., Lotan, A., et al. (2022). Role of 5-HT2A, 5-HT2C, 5-HT1A and TAAR1 receptors in the head twitch response induced by 5-Hydroxytryptophan and psilocybin: translational implications. Int. J. Mol. Sci. 23:4148. doi: 10.3390/ijms232214148
Shahar, O., Botvinnik, A., Shwartz, A., Lerer, E., Golding, P., Buko, A., et al. (2024). Effect of chemically synthesized psilocybin and psychedelic mushroom extract on molecular and metabolic profiles in mouse brain. Mol. Psychiatry. doi: 10.1038/s41380-024-02477-w
Shahidi, S., Hashemi-Firouzi, N., Afshar, S., Asl, S. S., and Komaki, A. (2019). Protective effects of 5-HT1A receptor inhibition and 5-HT2A receptor stimulation against Streptozotocin-induced apoptosis in the Hippocampus. Malaysian J. Med. Sci. 26, 40–51. doi: 10.21315/mjms2019.26.2.5
Shao, L.-X., Liao, C., Gregg, I., Davoudian, P. A., Savalia, N. K., Delagarza, K., et al. (2021). Psilocybin induces rapid and persistent growth of dendritic spines in frontal cortex in vivo. Neuron 109, 2535–2544.e4. doi: 10.1016/j.neuron.2021.06.008
Shi, Q., Chang, C., Saliba, A., and Bhat, M. A. (2022). Microglial mTOR activation upregulates Trem2 and enhances β-amyloid plaque clearance in the 5XFAD Alzheimer's disease model. J. Neurosci. Off. J. Soc. Neurosci. 42, 5294–5313. doi: 10.1523/JNEUROSCI.2427-21.2022
Siegel, J. S., Daily, J. E., Perry, D. A., and Nicol, G. E. (2023). Psychedelic drug legislative reform and legalization in the US. JAMA Psychiatry 80, 77–83. doi: 10.1001/jamapsychiatry.2022.4101
Singh, S., Botvinnik, A., Shahar, O., Wolf, G., Yakobi, C., Saban, M., et al. (2023). Effect of psilocybin on marble burying in ICR mice: role of 5-HT1A receptors and implications for the treatment of obsessive-compulsive disorder. Transl. Psychiatry 13:164. doi: 10.1038/s41398-023-02456-9
Slomski, A. (2022). Psilocybin for treatment of alcohol use disorder. JAMA 328:1288. doi: 10.1001/jama.2022.15436
Smedfors, G., Glotfelty, E., Kalani, N., Hjelle, C. P., Horntvedt, O., Wellfelt, K., et al. (2022). Psilocybin combines rapid synaptogenic and anti-inflammatory effects in vitro. Res. Sq. doi: 10.21203/rs.3.rs-1321542/v1
Smigielski, L., Scheidegger, M., Kometer, M., and Vollenweider, F. X. (2019). Psilocybin-assisted mindfulness training modulates self-consciousness and brain default mode network connectivity with lasting effects. NeuroImage 196, 207–215. doi: 10.1016/j.neuroimage.2019.04.009
Smith, W. R., and Sisti, D. (2020). Ethics and ego dissolution: the case of psilocybin. J. Med. Ethics 47, 807–814. doi: 10.1136/medethics-2020-106070
Spriggs, M. J., Giribaldi, B., Lyons, T., Rosas, F. E., Kärtner, L. S., Buchborn, T., et al. (2023). Body mass index (BMI) does not predict responses to psilocybin. J. Psychopharmacol. 37, 107–116. doi: 10.1177/02698811221131994
Stroud, J. B., Freeman, T. P., Leech, R., Hindocha, C., Lawn, W., Nutt, D. J., et al. (2018). Psilocybin with psychological support improves emotional face recognition in treatment-resistant depression. Psychopharmacology 235, 459–466. doi: 10.1007/s00213-017-4754-y
Suleiman, M., Basu, A., Belal, S., and Jacob, T. (2022). From mushrooms to Myolysis: a case of Rhabdo in psilocybin-induced mood and psychotic disorder. J. Nerv. Ment. Dis. 210, 638–639. doi: 10.1097/NMD.0000000000001489
Sun, Y., Ji, M., Leng, M., Li, X., Zhang, X., and Wang, Z. (2022). Comparative efficacy of 11 non-pharmacological interventions on depression, anxiety, quality of life, and caregiver burden for informal caregivers of people with dementia: a systematic review and network meta-analysis. Int. J. Nurs. Stud. 129:104204. doi: 10.1016/j.ijnurstu.2022.104204
Takei, N., Inamura, N., Kawamura, M., Namba, H., Hara, K., Yonezawa, K., et al. (2004). Brain-derived neurotrophic factor induces mammalian target of rapamycin-dependent local activation of translation machinery and protein synthesis in neuronal dendrites. J. Neurosci. 24, 9760–9769. doi: 10.1523/JNEUROSCI.1427-04.2004
Takei, N., Numakawa, T., Kozaki, S., Sakai, N., Endo, Y., Takahashi, M., et al. (1998). Brain-derived neurotrophic factor induces rapid and transient release of glutamate through the non-exocytotic pathway from cortical neurons. J. Biol. Chem. 273, 27620–27624. doi: 10.1074/jbc.273.42.27620
Torrado Pacheco, A., Olson, R. J., Garza, G., and Moghaddam, B. (2023). Acute psilocybin enhances cognitive flexibility in rats. Neuropsychopharmacology: official publication of the American college of. Neuropsychopharmacology 48, 1011–1020. doi: 10.1038/s41386-023-01545-z
Uddin, L. Q. (2021). Cognitive and behavioural flexibility: neural mechanisms and clinical considerations. Nat. Rev. Neurosci. 22, 167–179. doi: 10.1038/s41583-021-00428-w
Vann Jones, S A , O’kelly A (2020). Psychedelics as a Treatment for Alzheimer’s Disease Dementia. Frontiers In Synaptic Neuroscience, 12:34 doi: 10.3389/fnsyn.2020.00034
Van Dyck, C. H., Swanson, C. J., Aisen, P., Bateman, R. J., Chen, C., Gee, M., et al. (2023). Lecanemab in early Alzheimer's disease. N. Engl. J. Med. 388, 9–21. doi: 10.1056/NEJMoa2212948
Vargas, M. V., Dunlap, L. E., Dong, C., Carter, S. J., Tombari, R. J., Jami, S. A., et al. (2023). Psychedelics promote neuroplasticity through the activation of intracellular 5-HT2A receptors. Science 379, 700–706. doi: 10.1126/science.adf0435
Versijpt, J., Van Laere, K. J., Dumont, F., Decoo, D., Vandecapelle, M., Santens, P., et al. (2003). Imaging of the 5-HT2A system: age-, gender-, and Alzheimer's disease-related findings. Neurobiol. Aging 24, 553–561. doi: 10.1016/S0197-4580(02)00137-9
Vollenweider, F. X., and Kometer, M. (2010). The neurobiology of psychedelic drugs: implications for the treatment of mood disorders. Nat. Rev. Neurosci. 11, 642–651. doi: 10.1038/nrn2884
Vollenweider, F. X., Vollenweider-Scherpenhuyzen, M. F., Bäbler, A., Vogel, H., and Hell, D. (1998). Psilocybin induces schizophrenia-like psychosis in humans via a serotonin-2 agonist action. Neuroreport 9, 3897–3902. doi: 10.1097/00001756-199812010-00024
Von Bernhardi, R., Bernhardi, L. E.-V., and Eugenín, J. (2017). What is neural plasticity? Adv. Exp. Med. Biol. 1015, 1–15. doi: 10.1007/978-3-319-62817-2_1
Von Rotz, R., Schindowski, E. M., Jungwirth, J., Schuldt, A., Rieser, N. M., Zahoranszky, K., et al. (2023). Single-dose psilocybin-assisted therapy in major depressive disorder: a placebo-controlled, double-blind, randomised clinical trial. EClinicalMedicine 56:101809. doi: 10.1016/j.eclinm.2022.101809
Wang, C. S., Kavalali, E. T., and Monteggia, L. M. (2022). BDNF signaling in context: from synaptic regulation to psychiatric disorders. Cell 185, 62–76. doi: 10.1016/j.cell.2021.12.003
Wang, S., Mustafa, M., Yuede, C. M., Salazar, S. V., Kong, P., Long, H., et al. (2020). Anti-human TREM2 induces microglia proliferation and reduces pathology in an Alzheimer's disease model. J. Exp. Med. 217:785. doi: 10.1084/jem.20200785
Wang, Z.-H., Xiang, J., Liu, X., Yu, S. P., Manfredsson, F. P., Sandoval, I. M., et al. (2019). Deficiency in BDNF/TrkB neurotrophic activity stimulates δ-secretase by upregulating C/EBPβ in Alzheimer's disease. Cell Rep. 28, 655–669.e5. doi: 10.1016/j.celrep.2019.06.054
Weiss, B., Ginige, I., Shannon, L., Giribaldi, B., Murphy-Beiner, A., Murphy, R., et al. (2024). Personality change in a trial of psilocybin therapy v. escitalopram treatment for depression. Psychol. Med. 54, 1–15. doi: 10.1017/S0033291723001514
Whitfield-Gabrieli, S., and Ford, J. M. (2012). Default mode network activity and connectivity in psychopathology. Annu. Rev. Clin. Psychol. 8, 49–76. doi: 10.1146/annurev-clinpsy-032511-143049
Wu, Z., Chen, C., Kang, S. S., Liu, X., Gu, X., Yu, S. P., et al. (2021). Neurotrophic signaling deficiency exacerbates environmental risks for Alzheimer's disease pathogenesis. Proc. Natl. Acad. Sci. USA 118:6118. doi: 10.1073/pnas.2100986118
Xiang, J., Wang, Z.-H., Ahn, E. H., Liu, X., Yu, S.-P., Manfredsson, F. P., et al. (2019). Delta-secretase-cleaved tau antagonizes TrkB neurotrophic signalings, mediating Alzheimer's disease pathologies. Proc. Natl. Acad. Sci. USA 116, 9094–9102. doi: 10.1073/pnas.1901348116
Xiao, J., Shao, L., Shen, J., Jiang, W., Feng, Y., Zheng, P., et al. (2016). Effects of ketanserin on experimental colitis in mice and macrophage function. Int. J. Mol. Med. 37, 659–668. doi: 10.3892/ijmm.2016.2486
Yang, L., Zhao, X., Wang, L., Yu, L., Song, M., and Wang, X. (2015). Emotional face recognition deficit in amnestic patients with mild cognitive impairment: behavioral and electrophysiological evidence. Neuropsychiatr. Dis. Treat. 11, 1973–1987. doi: 10.2147/NDT.S85169
Zanikov, T., Gerasymchuk, M., Ghasemi Gojani, E., Robinson, G. I., Asghari, S., Groves, A., et al. (2023). The effect of combined treatment of psilocybin and eugenol on lipopolysaccharide-induced brain inflammation in mice. Molecules 28:2624. doi: 10.3390/molecules28062624
Zeifman, R. J., Wagner, A. C., Monson, C. M., and Carhart-Harris, R. L. (2023). How does psilocybin therapy work? An exploration of experiential avoidance as a putative mechanism of change. J. Affect. Disord. 334, 100–112. doi: 10.1016/j.jad.2023.04.105
Zhao, X., Du, Y., Yao, Y., Dai, W., Yin, Y., Wang, G., et al. (2024). Psilocybin promotes neuroplasticity and induces rapid and sustained antidepressant-like effects in mice. J. Psychopharmacol. 38, 489–499. doi: 10.1177/02698811241249436
Zhong, L., Xu, Y., Zhuo, R., Wang, T., Wang, K., Huang, R., et al. (2019). Soluble TREM2 ameliorates pathological phenotypes by modulating microglial functions in an Alzheimer's disease model. Nat. Commun. 10:1365. doi: 10.1038/s41467-019-09118-9
Zuidersma, M., Chua, K.-C., Hellier, J., Voshaar, R. O., and Banerjee, S. (2019). Sertraline and mirtazapine versus placebo in subgroups of depression in dementia: findings from the HTA-SADD randomized controlled trial. Am. J. Geriatr. Psychiatry 27, 920–931. doi: 10.1016/j.jagp.2019.03.021
Glossary
Keywords: psilocybin, Alzheimer’s disease, 5-HT2A receptor, neuroplasticity, anti-inflammation, pharmacology
Citation: Zheng S, Ma R, Yang Y and Li G (2024) Psilocybin for the treatment of Alzheimer’s disease. Front. Neurosci. 18:1420601. doi: 10.3389/fnins.2024.1420601
Edited by:
Katherine Conant, Georgetown University Medical Center, United StatesReviewed by:
Nakul Ravi Raval, Yale University, United StatesMohd Moin Khan, Brigham and Women’s Hospital and Harvard Medical School, United States
Dinesh Pal, University of Michigan, United States
Copyright © 2024 Zheng, Ma, Yang and Li. This is an open-access article distributed under the terms of the Creative Commons Attribution License (CC BY). The use, distribution or reproduction in other forums is permitted, provided the original author(s) and the copyright owner(s) are credited and that the original publication in this journal is cited, in accordance with accepted academic practice. No use, distribution or reproduction is permitted which does not comply with these terms.
*Correspondence: Gang Li, Z2FuZ2xpMjAwOEBodXN0LmVkdS5jbg==