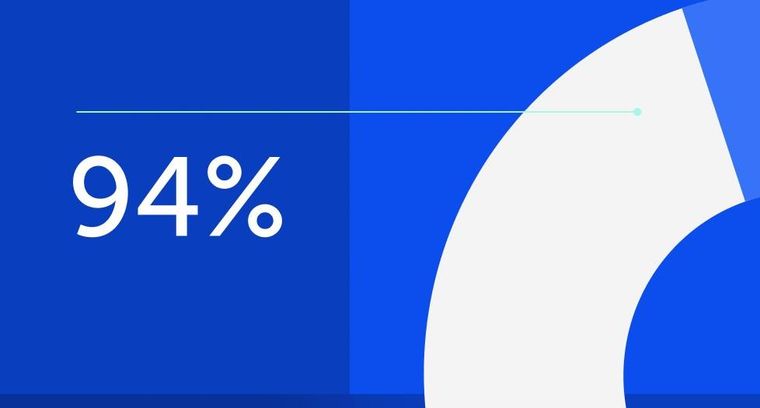
94% of researchers rate our articles as excellent or good
Learn more about the work of our research integrity team to safeguard the quality of each article we publish.
Find out more
ORIGINAL RESEARCH article
Front. Neurosci., 27 March 2024
Sec. Sleep and Circadian Rhythms
Volume 18 - 2024 | https://doi.org/10.3389/fnins.2024.1306070
This article is part of the Research TopicWomen in Sleep and Circadian RhythmsView all 4 articles
Introduction: Night-shift workers often face various health issues stemming from circadian rhythm shift and the consequent poor sleep quality. We aimed to study nurses working night shifts, evaluate the electroencephalogram (EEG) pattern of daytime sleep, and explore possible pattern changes due to ambient light exposure (30 lux) compared to dim conditions (<5 lux) during daytime sleep.
Moethods: The study involved 31 participants who worked night shifts and 24 healthy adults who had never worked night shifts. The sleep macro and microstructures were analyzed, and electrophysiological activity was compared (1) between nighttime sleep and daytime sleep with dim light and (2) between daytime sleep with dim and 30 lux light conditions.
Results: The daytime sleep group showed lower slow or delta wave power during non-rapid eye movement (NREM) sleep than the nighttime sleep group. During daytime sleep, lower sigma wave power in N2 sleep was observed under light exposure compared to no light exposure. Moreover, during daytime sleep, lower slow wave power in N3 sleep in the last cycle was observed under light exposure compared to no light exposure.
Discussion: Our study demonstrated that night shift work and subsequent circadian misalignment strongly affect sleep quality and decrease slow and delta wave activities in NREM sleep. We also observed that light exposure during daytime sleep could additionally decrease N2 sleep spindle activity and N3 waves in the last sleep cycle.
Night-shift workers, defined as individuals who generally work between 21:00 and 08:00 (Wright et al., 2013), comprise 3.3% of the total working population (May, 2011). They often face various health issues stemming from poor sleep quality, which is affected by reduced time in bed, outside noise, and family interruptions (May, 2011). The reduced sleep quality associated with night-shift work leads to excessive sleepiness and impaired alertness and may negatively impact patient care and safety (Kecklund and Axelsson, 2016; Alfonsi et al., 2021).
Circadian rhythms, primarily concurring with the 24-h light/dark cycle, are regulated by the suprachiasmatic nuclei (SCN) located in the hypothalamus (Wright et al., 2013; Alshahrani et al., 2017). This system typically encourages wakefulness during the day and sleep at night (Wright et al., 2013; Alshahrani et al., 2017). However, owing to circadian rhythm misalignment, night-shift workers may face difficulties in maintaining quality daytime sleep. In particular, exposure to strong lights during nighttime work has been associated with a disrupted circadian system (Yoon et al., 2002; Knufinke et al., 2021), lowered sleep efficiency (Martin et al., 2015), decreased time in bed (Choi and Joo, 2016), and attenuated alertness (or wakefulness) after waking (Yoon et al., 2002; Chang et al., 2015; Esaki et al., 2019). Furthermore, light exposure during sleep can impair glucose homeostasis, potentially via increased sympathetic nervous system activation (Mason et al., 2022). Thus, two questions arise: (1) Whether daytime sleep for night shift workers is different from nighttime sleep for daytime workers? (2) If this is true, is the difference due to circadian rhythm shift or light exposure during sleep or both?
The effects of circadian rhythm shifts on daytime sleep in night-shift workers have been investigated using objective sleep parameters based on polysomnography (PSG) and subjective measures using self-report questionnaires or sleep diary. These studies showed that the main clinical characteristics of people with sleep disturbance from night-shift work are shorter sleep duration and sleepiness (Wright et al., 2013). In the analysis of the effect of light exposure during daytime sleep, PSG did not reveal significant changes in those working 12-h night shifts (Choi et al., 2019). This suggests that analysis of the standard PSG parameters may not fully explain the effects of ambient light on daytime sleep. Therefore, more sensitive quantitative markers are required to elucidate the effects of light exposure.
In sleep electroencephalography (EEG), several types of characteristic field potential oscillations act as important biomarkers for assessing physiological brain activity in relation to different sleep stages. Slow wave sleep (SWS), with a peak frequency of ~0.8 Hz in humans, occurs primarily in non-rapid eye movement (NREM) N3 sleep (Carlson, 2013; Gage and Baars, 2018). Sleep spindles, brief and powerful bursts originating from specific oscillations generated in thalamic circuits, occur mainly in N2 sleep, with a synchronous 12–15 Hz neuronal firing rate (Manoach and Shinn, 2013; Siclari and Tononi, 2016). Such EEG features may further characterize brain activities that alter during sleep exposed to ambient light. For example, nighttime sleep with lights on was associated with decreased theta power during rapid eye movement (REM) sleep and reductions in slow, delta, and spindle power during NREM sleep (Cho et al., 2013). This suggests that light exposure may lead to less deep nighttime sleep. However, it remains unclear whether light exposure adversely affects daytime sleep in night shift workers. It is hypothesized that the effects of light exposure may be different and more complicated during daytime sleep because of the known adverse effects of circadian rhythm shifts on sleep (James et al., 2020; Mentink et al., 2020).
Thus, we aimed to study the pattern of relative power changes of EEG in each cycle and stage of daytime sleep in nurses, after the first night shift in their weekly work schedule, and explore possible pattern changes due to ambient light exposure (30 lux) compared to dim conditions (< 5 lux). We further investigated the temporal dynamics of the relative power of sleep EEG in various brain regions.
Participants were recruited from two cohorts at the Samsung Medical Center (SMC) in Seoul, South Korea: (a) night shift nurses with daytime sleep and (b) day workers with regular nighttime sleep. We advertised in the hospital cafeteria to recruit participants. All participants were female Koreans and were older than 24 years of age. In case of night shift nurse, they worked in rotating shifts work for more than 1 year at a metropolitan hospital (≥2,000 beds) in Seoul, Republic of Korea. Participants were excluded if they were currently using hypnotics or central nervous system stimulants, had a history of any psychiatric illness or major systemic disease, smoked cigarettes, drank more than 2 glasses per day, or were pregnant or lactating during the study period. Participants who experienced sleep disorders, other than insomnia, such as obstructive sleep apnea with Apnea-hypopnea index equal to or greater than 5 events per hour in PSG, narcolepsy, rapid eye movement sleep behavior disorder, restless legs syndrome, or periodic limb movement disorder confirmed by PSG with a total periodic limb movement index of 15 events per hour or higher and a movement arousal index equal to or greater than 5 events per hour in PSG were also excluded. All participants completed questionnaires on sleep, including the Epworth Sleepiness Scale (ESS) (Johns, 1991), and Insomnia Severity Index (ISI) at their first laboratory visit (Bastien et al., 2001). All participants voluntarily enrolled in this study, and provided written informed consent. All procedures were approved by the Institutional Review Board of Samsung Medical Center (IRB No:2018–05-120 for daytime sleep in shift workers and 2018–10-037 for nighttime sleep in healthy adults) and conducted in accordance with the Declaration of Helsinki.
Thirty-three healthy female nurses who worked two 12-h night shifts were enrolled in this study. Eligible participants had rotating shift work careers, including night shifts, for at least 1 year, and worked a minimum of 24 h per week. The nurses had a two-shift work schedule of D12-D12-N12-N12, followed by four or five rest days (D12 shift from 7:00 a.m. to 7:30 p.m. and N12 shift from 7:00 p.m. to 7:30 a.m., including a 30-min mealtime in each shift). Data collection was conducted between 22 July 2018 and 25 January 2019. A total of 33 shift nurses participated, two of whom participants were excluded due to unmet work schedule requirements. In total, 31 nurses (mean 26.7 ± 3.06 years) were included in the study (Supplementary Figure S1).
Twenty-four healthy female adults (mean 26.9 ± 3.55 years) were identified as the intermediate chronotype as defined by a Morningness-eveningness questionnaire (MEQ) (Horne and Ostberg, 1976) and 7-days sleep diary. The participants slept between 11:00 p.m. and 8:00 a.m. This cohort was recruited and analyzed in our previous study (Park et al., 2020; Jo et al., 2021). Data collection was conducted between 04 December 2018 and 24 August 2019.
For daytime sleep in the night shift nurse group, a one-sample crossover design was used and included 2-day PSG studies: one PSG study was performed under dim light environmental exposure (<5 lux), and another was performed under 30 lux light exposure (at the level of the participants’ eyes) using a bright-controllable light-emitting diode (LED) lamp on the ceiling. Light was maintained throughout the session. The order of light exposure during PSG was determined by 6-block randomization using the R-program. All experiments were performed in a sleep laboratory.
• Sleep with light exposure condition: EEG from PSG during daytime sleep with the light on (30 lux).
• Sleep under dim light conditions: EEG from PSG acquired from the same subjects as above but during another instance of daytime sleep with dim light (<5 lux).
To compare the daytime sleep group described above with the nighttime sleep group, we used our previously published nighttime sleep cohort (described in Section 2.1.2) that was studied in the same laboratory settings (Park et al., 2020; Jo et al., 2021).
For daytime sleep, night shift nurses visited the sleep laboratory after completing their first night shift and eating breakfast. Sleep PSG was performed using the Embla N7000 system (Embla, Reykjavik, Iceland). Six-channel EEG signals (F3/F4/C3/C4/O1/O2) were collected with an electrooculogram (EOG), electromyogram, and electrocardiogram to assess sleep stages, recorded at a 200 Hz sampling rate. The usual bedtime of the participants was relatively constant from 9:00 a.m. to 10:00 a.m. and habitual wake-up time varied from 12:00 p.m. to 5:30 p.m. The lights off and start of the sleep study were adjusted to the habitual sleep hours taken after the 12 h night shift. The PSG study was considered completed when the participants woke up of their own will. If the participants failed to wake up at 5:00 p.m., they were woken at 5:30 p.m. for the next night shift and completed the PSG study. Then, the participants were requested to fill out their estimated sleep (subjective total sleep time [TST] and sleep latency). The 2nd PSG study was conducted for each participant during the next shift cycle at the same time. Sleep architecture was scored in 30-s epochs according to the standard criteria by an experienced technician who was blinded to the light conditions.
At night, participants were admitted to the sleep laboratory at approximately 4:00 p.m. They consumed the provided meal and then applied the PSG electrodes. The participants sat on a chair and remained awake with 3,000 or 4,000 K LED light exposure from 6:00 p.m. to 12:00 a.m. The participants went to bed with their usual sleeping arrangements and woke up in the morning at the time they normally wake up for work.
We analyzed the relative spectral power of EEG in the sleep stages for nighttime and daytime sleep under dim and 30 lux environmental light conditions. The EEG signals were referenced to the contralateral mastoid. We performed 0.5–100 Hz band-pass filter using a Chebyshev digital filter. We removed ECG and EOG signal components using the EEGLAB toolbox from six-channel sleep EEG. If the power at a time point was higher than 5 standard deviations (SD), we considered it an artifact and removed it. When the proportion of artifacts in the given channel was more than 30% of the EEG data, we flagged it as a “bad” channel and replaced it with its opposite hemispherical channel data (for example, F3 could be replaced with F4, C3 with C4, and O1 with O2).
In our study, we utilized MNELAB, a Python-based processing toolbox, to analyze the spectral patterns of six-channel sleep EEG data (Gramfort et al., 2014) by applied a 3rd order Chebyshev bandpass filter to split the data into five frequency bands: slow (0.5–1 Hz), delta (1–4 Hz), theta (4–8 Hz), alpha (8–12 Hz), and sigma (12–15 Hz). The power in each frequency band was then computed. The sleep stages (N1–N3, REM) were manually scored according to the guidelines outlined by the American Academy of Sleep Medicine (AASM) standards (Berry et al., 2017). Subsequently, we calculated the spectral pattern of the sleep EEG data separately for each sleep stage. To enhance the spectral characteristics, we averaged the power values of the six-channel EEGs to obtain a single value. Finally, we determined the relative EEG power for each sleep stage by calculating the power ratio within a specific frequency band to the total power (0.5–15 Hz) of each participant. This approach, chosen over absolute power measurements, accounts for individual differences in factors such as skull thickness and scalp conductivity. Furthermore, relative power provides a more nuanced view of how specific frequency bands (e.g., delta or sigma waves) change in proportion to the overall EEG activity, amplifying alterations in specific types of brain activity.
We segmented the sleep cycle, which initiates with NREM sleep and transitions into REM sleep as sleep becomes more profound (Carskadon and Rechtschaffen, 2011). Subsequently, we analyzed the changes in EEG spectral patterns corresponding to the progression of sleep. These comparisons were performed between nighttime and daytime sleep under dim light conditions, and between daytime sleep with dim light and nighttime sleep with 30 lux light exposure conditions. We developed a MATLAB toolbox for sleep cycle partitioning1 to visualize the sleep stages of the subjects and perform single clicks on the hypnogram to record the split points of different cycles.
The nighttime sleep group exhibited an average of 4.52 ± 0.79 cycles, ranging from 3 to 6 cycles. In the daytime sleep group exposed to 30 lux light, the average number of sleep cycles was 4.41 ± 0.77, also ranging from 3 to 6 cycles. Meanwhile, under the dim light setting, the daytime sleep group had an average of 4.57 ± 0.85 sleep cycles, also within a 3 to 6 cycles range. Because the minimum number of sleep cycles in the data was three and the number of cycles varied across individuals, to allow between-individual analysis, we extracted the first, second, and final sleep cycles from all individuals and analyzed them for EEG temporal pattern changes.
To explore how circadian misalignment affects sleep quality, we compared nighttime and daytime sleep under dim light conditions. First, we analyzed demographic and PSG variables using the Student’s independent sample t-test. We then conducted separate linear regression analyses to compare the relative power of electrophysiological activity for the entire night between nighttime and daytime sleep. This comparison was performed for five frequency bands and three NREM sleep stages. Additionally, we examined the relative EEG power between the two groups representing different sleep conditions for the first, second, and final sleep cycles using linear regression, again considering all five frequency bands and the three NREM sleep stages. Because the insomnia severity index (ISI) was significantly different between the two groups (Table 1; participants’ detailed characteristics are further reported in the Results section 3.1), we included it as a covariate in the EEG power comparison to correct for its possible effect. All statistical results were corrected for multiple comparisons by controlling for false discovery rate (FDR).
To examine the influence of light exposure on daytime sleep quality, we followed procedures similar to those described in section 2.3.1. However, paired t-tests were used instead of linear regression because the two conditions were tested within the same cohort. We compared the relative power of EEG for the entire night, and first, second, and final sleep cycles between dim light and 30 lux light conditions, considering the five frequency bands and three NREM sleep stages. As in the previous analysis, all statistical results were adjusted for multiple comparisons using the FDR control method.
The average time of employment as shift nurses was 3.8 ± 2.74 years (range 1.2–14.3 years). The mean ISI score of the daytime sleep group was higher than that of the nighttime sleep group (15.8 ± 3.74 h vs. 5.7 ± 2.97 h, p < 0.001). The mean ESS scores did not differ significantly between the daytime and nighttime sleep groups (Table 1).
The daytime sleep group showed shorter sleep latency, higher percentage of wakefulness after sleep onset (WASO), lower sleep efficiency, reduced percentage of N2 sleep, and higher percentage of N3 sleep than the nighttime sleep group. The total arousal index was similar between the groups; however, the spontaneous arousal index was higher in the daytime sleep group. The subjectively estimated TST was longer in the daytime sleep group than in the nighttime sleep group. However, the TST for the nighttime sleep group, at 5.5 ± 1.41 h, was shorter than the time of 6.5 h suggested by previous studies (Patel et al., 2009; Åkerstedt et al., 2022). This discrepancy can be attributed to our nighttime sleep group being primarily hospital employees, as they were recruited from hospitals. The average TST for hospital workers has been reported to be 5.75 ± 0.74 h, which is consistent with our findings (Table 2; Choi et al., 2021).
Table 2. Polysomnography results during daytime sleep of shift workers and nighttime sleep of healthy adults.
There were no significant differences in PSG parameters between the two light conditions except for time in bed and REM arousal index: Time in bed and REM arousal index in the dim light condition was longer than that in the 30 lux light exposure condition (Table 2).
The relative power characteristics of EEG in the N1-N3 and REM sleep stages between nighttime and daytime sleep under dim light conditions are shown in Figure 1. In the N1 sleep stage, the relative power of the slow wave was significantly lower during daytime sleep with dim light than during nighttime sleep (p < 0.05). Other frequency bands did not differ between the two groups (Figure 1A). In the N2 sleep stage, the relative power of the slow wave was significantly lower, and the relative power of the theta band was higher during daytime than during nighttime sleep (p < 0.05, Figure 1B). In the N3 sleep stage, the relative powers of the slow and delta waves were significantly lower during daytime sleep than during nighttime sleep (slow: p < 0.001; delta: p < 0.05), and the relative power of the theta waves was significantly higher during daytime than during nighttime sleep (p < 0.05). In-depth visualization of the relative power in the narrow band from 0 to 8 Hz (the bands of slow, delta, and theta waves) showed that the nighttime sleep group exhibited a peak relative power at approximately 1 Hz between the slow and delta bands, whereas the daytime sleep group showed a peak at approximately 4 Hz between the delta and theta bands (Figure 1C). This suggests that the poorer N3 sleep for daytime sleepers is due to a lack of slow-wave activities and more promoted theta wave activities compared to nighttime sleepers.
Figure 1. Comparison of the relative power of sleep EEG between nighttime sleep and daytime sleep [both under dim light conditions (<5 lux)]. We analyzed separately five frequency bands (slow, delta, theta, alpha, and sigma) and four sleep stages (A). In N1 sleep, slow wave was higher during nighttime sleep (B). In N2 sleep, slow wave was higher and theta wave was lower during nighttime sleep (C). In N3 sleep, slow and delta waves were higher, while theta wave was lower during nighttime sleep (D). There were no significant differences in REM sleep (*p < 0.05, **p < 0.001).
During REM sleep, there were no differences in the relative powers of all frequency bands between nighttime and daytime sleep (Figure 1D).
The relative power of EEG differences in the N1-N3 and REM sleep stages under different ambient light conditions is shown in Figure 2. In the N2 sleep stage, the relative power of the sigma wave, which is the main band frequency of sleep spindles, was significantly higher under dim light than under 30 lux light condition (p < 0.05) (Figure 2B). Other frequency band waves were not significantly different between the dim light and 30 lux light conditions. There were no differences in the relative power of all frequency bands between the dim light and 30 lux light conditions in the N1, N3, and REM sleep stages (Figures 2A,C,D).
Figure 2. Comparison of the relative power of sleep EEG between 30 lux and dim (<5 lux) light conditions during daytime sleep. We separately analyzed five frequency bands (slow, delta, theta, alpha, and sigma) and four sleep stages (A). In N1 sleep, there were no significant differences between 30 lux and dim light conditions (B). In N2 sleep, sigma wave activity was lower in the 30 lux light condition (C). In N3 sleep, there were no significant differences (D). There were no significant differences in REM sleep either (*p < 0.05).
When the time group was used as a covariate in the linear regression analysis, there was no change in the results. Absolute power was measured across all EEG frequency bands, and comparisons between groups were conducted. These results are presented in Supplementary Table S1.
The pattern of relative EEG power of slow waves that varied with the sleep cycle in the different groups is shown in Figure 3. The relative EEG power of N3 sleep during daytime sleep with dim light was significantly lower than that during nighttime sleep with dim light in the first, second, and last cycles (p < 0.05; Figure 3A). Frequency bands in sleep stages other than N3 did not show significant differences between daytime and nighttime sleep with dim light conditions.
Figure 3. Comparison of temporal patterns of N3 slow wave power between different sleep groups and between different light conditions. We separately analyzed the N3 slow wave in each of the three sleep cycles (first, second, and last) (*p < 0.05) (A). Relative power differences between nighttime sleep and daytime sleep under the same dim light conditions (B). Relative power differences between 30 lux and dim light conditions during daytime sleep.
The relative power of slow waves in daytime sleep with dim light compared to daytime sleep with 30 lux light was significantly higher (p < 0.05) in N3 sleep of the last sleep cycle only (Figure 3B). Frequency bands in sleep stages other than N3 did not show significant differences between the dim and 30 lux light conditions during daytime sleep.
In this study, the analysis of sleep EEG power showed a lower relative power of slow or delta waves in all NREM sleep stages (N1: slow, N2, and N3: slow and delta) during daytime sleep in shift workers compared to nighttime sleep in day workers, whereas a higher power in the theta wave in the N2 and N3 stages was observed during daytime sleep compared to nighttime sleep. We found that during daytime sleep, a lower sigma wave power in N2 was observed under light exposure conditions compared to no light exposure. Moreover, daytime sleepers had lower slow wave power in the N3 stage in all three cycles (first, second, and last) than nighttime sleepers. During daytime sleep, a lower slow wave power in the N3 stage of the last cycle was observed under light exposure than under no light exposure.
Rotating shifts are common among nurses to ensure the continuity of care. One of the most harmful effects of night-shift work is the deterioration of sleep patterns caused by circadian rhythm disruption and increased sleep homeostatic pressure (Wright et al., 2013; Alfonsi et al., 2021). Despite the increase in sleep pressure as a result of night shifts, it has been reported that the effect of light exposure during night work can lead to poor-quality daytime sleep (Geiger-Brown et al., 2012; Wright et al., 2013; Kaliyaperumal et al., 2017; Sletten et al., 2017; Sunde et al., 2020). Persistent poor sleep may lead to increased fatigue, which can negatively impact patient and staff safety. Therefore, it is essential to create a low-risk environment for sleep, possibly through the use of eye masks, earplugs, and light-blocking curtains or shades (Wright et al., 2013).
Our comparison of other PSG parameters between daytime and nighttime sleep under the same dim light conditions showed shorter sleep latency, higher percentage of WASO, lower sleep efficiency, reduced percentage of N2 sleep, and higher percentage of N3 sleep during daytime sleep than during nighttime sleep. This finding is reasonable because when sleep deprivation occurs owing to night work, the homeostatic drive for sleep increases (Boivin et al., 2022), which may further decrease sleep latency and increase the percentage of N3 sleep. Similar results were also found in a simulation study by Pedersen et al. (2022). On the contrary, our findings of higher percentage of WASO and lower sleep efficiency during daytime sleep suggest that sleep disturbances are common in shift workers, likely due to circadian rhythm misalignment, resulting in low melatonin levels and high cortisol levels during daytime sleep (Boivin et al., 2022).
When comparing the relative spectral power of sleep EEG between daytime and nighttime sleep, the daytime sleep group had a significantly lower power of slow waves in the entire NREM sleep period (N1, N2, and N3) and a lower power of delta waves in N3 sleep. This is supported by previous studies, which showed that shift workers’ daytime sleep inhibits slow-wave activity compared with normal nighttime sleep (Grønli et al., 2017).
The daytime sleep group also presented a significantly higher theta wave power during N2 and N3 sleep. In contrast, the percentage of N3 sleep during daytime sleep was greater than that during nighttime sleep. Despite this increased percentage of longer N3 sleep during daytime sleep, it is noteworthy that spectral power analysis indicated that the quality of N3 sleep in daytime sleepers may be compromised due to the lower power in slow and delta waves, as demonstrated in Figure 1C. The impact of this altered power pattern of N3 sleep during daytime sleep on night-shift work performance remains to be clarified.
There were no significant differences in PSG parameters between dim light (<5 lux) and overhead room light (30 lux) conditions during daytime sleep, except for the mean time in bed and the REM arousal index. The first night shift in a sequence often leads to prolonged wakefulness (Ganesan et al., 2019). By the end of the first night, the continuous time awake often reaches 24 h or longer and coincides with the circadian nadir for alertness (Ganesan et al., 2019). For this reason, it is possible that light stimuli have only subtle effects on PSG parameters. Thus, these subtle changes in PSG are reasonably different from the strong effects of light exposure on normal nighttime sleep in day workers (Cho et al., 2013).
Thus, our in-depth analysis explored the possibility of alterations in the spectral power of sleep EEG waves for various sleep stages and cycles in relation to light exposure during daytime sleep Indeed, we identified decreased power of sleep spindles (represented by the sigma wave) in N2 sleep for night-shift workers with light exposure during daytime sleep compared to when they slept without light exposure during daytime. Sleep spindles serve as a transitional phase between the light sleep of stage 1 and the deep sleep stages 3, known as slow-wave sleep. Their contribution to the cyclical pattern of sleep stages is crucial for upholding a stable sleep architecture (De Gennaro and Ferrara, 2003; Andrillon et al., 2011; Purcell et al., 2017). Sleep spindles result from an interaction between the thalamus and the cortex. This interaction is crucial for the synchronization of neuronal activity, which is important for the transition between sleep stages and the maintenance of a stable sleep state (Steriade, 2006; Astori et al., 2013; Lüthi, 2014). Any disruptions to this interaction can result in fragmented sleep and diminished sleep quality, which suggests that light exposure may disturb sleep stability. Furthermore, sleep spindle activity is highly associated with memory consolidation (Bergmann TOMolle et al., 2012; Leminen et al., 2017). Future studies are required to clarify whether light-exposed daytime sleep leads to impaired memory consolidation.
We did not observe differences in slow waves during N3 sleep between the dim light and 30 lux light conditions. This is likely explained by our finding that slow waves significantly decrease during daytime sleep with dim light. Therefore, daytime sleep strongly promotes poor deep sleep, and subsequently, no further decrease in slow waves could be exerted by light exposure.
However, our in-depth analysis of different sleep cycles showed that light exposure during daytime sleep significantly decreased the relative power of slow waves in the last cycle, but not in the early cycles (i.e., first and second cycles).
The current study had some limitations. First, the sample size was relatively small, with 31 night shift nurses and 24 daytime workers. Secondly, there was no significant difference in TST between daytime sleepers (5.4 ± 0.44 h) and nighttime sleepers (5.5 ± 1.41 h). In contrast, previous research has reported an average nighttime TST of approximately 6.5 h (Patel et al., 2009; Åkerstedt et al., 2022), which is longer than that observed for nighttime sleepers. The shorter TST in our results for nighttime sleepers might be attributed to the fact that they were recruited from hospitals and were predominantly hospital employees. Their average nighttime TST was reported to be 5.75 ± 0.74 h, aligning with our observations (Choi et al., 2021). Thirdly, the electrophysiological activity changes during daytime sleep may vary depending on the chronotype. Previous studies on nighttime sleep have shown that morning sleepers had fewer slow waves and more sigma wave activities in NREM compared to evening sleepers (Mongrain et al., 2005, 2006). In the current study, the relationship between chronotype and night shift work, circadian rhythm shift, or exposure to ambient light was not investigated; therefore, future studies may need to take this into account. Fourth, our study was based solely on young female participants working in a hospital environment, leading to a potential bias in our analysis. Furthermore, it is noteworthy that these nurses were not fixed night-shift workers but were in a 12-h rotation system. The generalizability of this study may be improved by including male participants, other age groups, fixed night-shift workers, and other occupations. Fifth, the effects of night-shift work (where nighttime sleep deprivation occurs and light exposure occurs during nighttime work) and circadian rhythm shifts could not be separately analyzed, although both can cause EEG power changes during daytime sleep. Finally, although we compared daytime sleep with and without light exposure, our analysis did not compare the effects of light exposure on daytime or nighttime sleep. This may not completely distinguish the effects of light exposure from the effects of circadian rhythm shift or night-shift work.
Our research clearly indicates that night shift work and the resulting disruption of the body’s natural sleep–wake cycle have a significant impact on sleep quality. Specifically, we found that these factors lead to a decrease in slow wave and delta wave activities during NREM sleep. Additionally, we discovered that exposure to light during daytime sleep further reduced N2 sleep spindle activity and decreased N3 wave activity, particularly during the final sleep cycle. These findings highlight the negative effects of shift work and daytime light exposure on the sleep quality of individuals working night shifts.
The datasets for this article are not publicly available due to concerns regarding participant/patient anonymity. Requests to access the datasets should be directed to the corresponding author.
The studies involving humans were approved by Institutional Review Board of Samsung Medical Center (IRB No:2018-05-120 for daytime sleep in shift workers and 2018-10-037 for nighttime sleep in healthy adults). The studies were conducted in accordance with the local legislation and institutional requirements. The participants provided their written informed consent to participate in this study.
SY: Formal analysis, Methodology, Validation, Writing – original draft. SC: Conceptualization, Data curation, Validation, Writing – original draft. CZ: Formal analysis, Methodology, Software, Writing – original draft. EJ: Funding acquisition, Project administration, Supervision, Writing – review & editing. HK: Conceptualization, Supervision, Writing – review & editing.
The author(s) declare that financial support was received for the research, authorship, and/or publication of this article. This research was supported by grants from the National Institutes of Health (P41EB015922) and the Samsung Medical Center (OTC1190671).
The authors declare that the research was conducted in the absence of any commercial or financial relationships that could be construed as a potential conflict of interest.
The author(s) declared that they were an editorial board member of Frontiers, at the time of submission. This had no impact on the peer review process and the final decision.
All claims expressed in this article are solely those of the authors and do not necessarily represent those of their affiliated organizations, or those of the publisher, the editors and the reviewers. Any product that may be evaluated in this article, or claim that may be made by its manufacturer, is not guaranteed or endorsed by the publisher.
The Supplementary material for this article can be found online at: https://www.frontiersin.org/articles/10.3389/fnins.2024.1306070/full#supplementary-material
1. ^GitHub link: https://github.com/ZCONG2025/Sleep-Cycle-Separation/tree/main
Åkerstedt, T., Schwarz, J., Lindberg, E., and Theorell-Haglöw, J. (2022). Total sleep time, sleep efficiency, and next day subjective sleepiness in a large group of women. Sleep Adv. 3:zpac028. doi: 10.1093/sleepadvances/zpac028
Alfonsi, V., Scarpelli, S., Gorgoni, M., Pazzaglia, M., Giannini, A. M., and De Gennaro, L. (2021). Sleep-related problems in night shift nurses: towards an individualized interventional practice. Front. Hum. Neurosci. 15:644570. doi: 10.3389/fnhum.2021.644570
Alshahrani, S. M., Baqays, A. A., Alenazi, A. A., AlAngari, A. M., and AlHadi, A. N. (2017). Impact of shift work on sleep and daytime performance among health care professionals. Saudi Med. J. 38, 846–851. doi: 10.15537/smj.2017.8.19025
Andrillon, T., Nir, Y., Staba, R. J., Ferrarelli, F., Cirelli, C., Tononi, G., et al. (2011). Sleep spindles in humans: insights from intracranial EEG and unit recordings. J. Neurosci. 31, 17821–17834. doi: 10.1523/JNEUROSCI.2604-11.2011
Astori, S., Wimmer, R. D., and Lüthi, A. (2013). Manipulating sleep spindles–expanding views on sleep, memory, and disease. Trends Neurosci. 36, 738–748. doi: 10.1016/j.tins.2013.10.001
Bastien, C. H., Vallieres, A., and Morin, C. M. (2001). Validation of the insomnia severity index as an outcome measure for insomnia research. Sleep Med. 2, 297–307. doi: 10.1016/S1389-9457(00)00065-4
Bergmann TOMolle, M., Diedrichs, J., Born, J., and Siebner, H. R. (2012). Sleep spindle-related reactivation of category-specific cortical regions after learning face-scene associations. NeuroImage 59, 2733–2742. doi: 10.1016/j.neuroimage.2011.10.036
Berry, R. B., Brooks, R., Gamaldo, C., Harding, S. M., Lloyd, R. M., Quan, S. F., et al. (2017). AASM scoring manual updates for 2017 (version 2.4). JCSM. 13, 665–666. doi: 10.5664/jcsm.6576
Boivin, D. B., Boudreau, P., and Kosmadopoulos, A. (2022). Disturbance of the circadian system in shift work and its health impact. J. Biol. Rhythm. 37, 3–28. doi: 10.1177/07487304211064218
Carskadon, M. A., and Rechtschaffen, A. (2011). Monitoring and staging human sleep. Princ. Pract. Sleep Med. 5, 16–26.
Chang, A. M., Aeschbach, D., Duffy, J. F., and Czeisler, C. A. (2015). Evening use of light-emitting eReaders negatively affects sleep, circadian timing, and next-morning alertness. Proc. Natl. Acad. Sci. USA 112, 1232–1237. doi: 10.1073/pnas.1418490112
Cho, J. R., Joo, E. Y., Koo, D. L., and Hong, S. B. (2013). Let there be no light: the effect of bedside light on sleep quality and background electroencephalographic rhythms. Sleep Med. 14, 1422–1425. doi: 10.1016/j.sleep.2013.09.007
Choi, S. J., Jo, H., Kim, D., and Joo, E. Y. (2021). Sleep-wake pattern, sleep quality and daytime status in fixed day-shift hospital workers. J. Sleep Med. 18, 167–174. doi: 10.13078/jsm.210021
Choi, S. J., and Joo, E. Y. (2016). Light exposure and sleep-wake pattern in rapidly rotating shift nurses. J. Sleep Med. 13, 8–14. doi: 10.13078/jsm.16002
Choi, S. J., Park, H. R., and Joo, E. Y. (2019). Effects of light on daytime sleep in 12 hours night shift workers: a field study. J. Sleep Med. 16, 26–35. doi: 10.13078/jsm.19026
De Gennaro, L., and Ferrara, M. (2003). Sleep spindles: an overview. Sleep Med. Rev. 7, 423–440. doi: 10.1053/smrv.2002.0252
Esaki, Y., Kitajima, T., Obayashi, K., Saeki, K., Fujita, K., and Iwata, N. (2019). Light exposure at night and sleep quality in bipolar disorder: the APPLE cohort study. J. Affect. Disord. 257, 314–320. doi: 10.1016/j.jad.2019.07.031
Gage, NM, and Baars, BJ. Fundamentals of cognitive neuroscience: a beginner's guide. 2nd San Diego, CA: Academic Press (2018)
Ganesan, S., Magee, M., Stone, J. E., Mulhall, M. D., Collins, A., Howard, M. E., et al. (2019). The impact of shift work on sleep, alertness and performance in healthcare workers. Sci. Rep. 9:4635. doi: 10.1038/s41598-019-40914-x
Geiger-Brown, J., Rogers, V. E., Trinkoff, A. M., Kane, R. L., Bausell, R. B., and Scharf, S. M. (2012). Sleep, sleepiness, fatigue, and performance of 12-hour-shift nurses. Chronobiol. Int. 29, 211–219. doi: 10.3109/07420528.2011.645752
Gramfort, A., Luessi, M., Larson, E., Engemann, D. A., Strohmeier, D., Brodbeck, C., et al. (2014). MNE software for processing MEG and EEG data. NeuroImage 86, 446–460. doi: 10.1016/j.neuroimage.2013.10.027
Grønli, J., Meerlo, P., Pedersen, T. T., Pallesen, S., Skrede, S., Marti, A. R., et al. (2017). A rodent model of night-shift work induces short-term and enduring sleep and electroencephalographic disturbances. J. Biol. Rhythm. 32, 48–63. doi: 10.1177/0748730416675460
Horne, J. A., and Ostberg, O. (1976). A self-assessment questionnaire to determine morningness-eveningness in human circadian rhythms. Int. J. Chronobiol. 4, 97–110.
James, L., James, S. M., Wilson, M., Brown, N., Dotson, E. J., Edwards, C. D., et al. (2020). Sleep health and predicted cognitive effectiveness of nurses working 12-hour shifts: an observational study. Int. J. Nurs. Stud. 112:103667. doi: 10.1016/j.ijnurstu.2020.103667
Jo, H., Park, H. R., Choi, S. J., Lee, S. Y., Kim, S. J., and Joo, E. Y. (2021). Effects of organic light-emitting diodes on circadian rhythm and sleep. Psychiatry Investig. 18, 471–477. doi: 10.30773/pi.2020.0348
Johns, M. W. (1991). A new method for measuring daytime sleepiness: the Epworth sleepiness scale. Sleep 14, 540–545. doi: 10.1093/sleep/14.6.540
Kaliyaperumal, D., Elango, Y., Alagesan, M., and Santhanakrishanan, I. (2017). Effects of sleep deprivation on the cognitive performance of nurses working in shift. J. Clin. Diagn. Res. 11, CC01–CC03. doi: 10.7860/JCDR/2017/26029.10324
Kecklund, G., and Axelsson, J. (2016). Health consequences of shift work and insufficient sleep. BMJ 355:i5210. doi: 10.1136/bmj.i5210
Knufinke, M., Nieuwenhuys, A., Geurts, S. A. E., Most, E. I. S., Moen, M. H., Maase, K., et al. (2021). Dim light, sleep tight, and wake up bright - sleep optimization in athletes by means of light regulation. Eur. J. Sport Sci. 21, 7–15. doi: 10.1080/17461391.2020.1722255
Leminen, M. M., Virkkala, J., Saure, E., Paajanen, T., Zee, P. C., Santostasi, G., et al. (2017). Enhanced memory consolidation via automatic sound stimulation during non-REM sleep. Sleep 40:zsx0003. doi: 10.1093/sleep/zsx0003
Lüthi, A. (2014). Sleep spindles: where they come from, what they do. Neuroscientist 20, 243–256. doi: 10.1177/1073858413500854
Manoach, D. S., and Shinn, R. S. (2013). “Schizophrenia, other neuropsychiatric disorders, and sleep” in Encyclopedia of sleep. ed. C. A. Kushida (San Diego, CA: Academic Press)
Martin, J. S., Laberge, L., Sasseville, A., Bérubé, M., Alain, S., Houle, J., et al. (2015). Day and night shift schedules are associated with lower sleep quality in evening-types. Chronobiol. Int. 32, 627–636. doi: 10.3109/07420528.2015.1033425
Mason, I. C., Grimaldi, D., Reid, K. J., Warlick, C. D., Malkani, R. G., Abbott, S. M., et al. (2022). Light exposure during sleep impairs cardiometabolic function. Proc. Natl. Acad. Sci. U. S. A. 119:e2113290119. doi: 10.1073/pnas.2113290119
May, J. F. (2011). “Driver Fatigue” in Handbook of traffic psychology. ed. B. E. Porter (San Diego: Academic Press), 287–297.
Mentink, L. J., Thomas, J., Melis, R. J., Olde Rikkert, M. G., Overeem, S., and Claassen, J. A. (2020). Home-EEG assessment of possible compensatory mechanisms for sleep disruption in highly irregular shift workers–the ANCHOR study. PLoS One 15:e0237622. doi: 10.1371/journal.pone.0237622
Mongrain, V., Carrier, J., and Dumont, M. (2005). Chronotype and sex effects on sleep architecture and quantitative sleep EEG in healthy young adults. Sleep 28, 819–827. doi: 10.1093/sleep/28.7.819
Mongrain, V., Carrier, J., and Dumont, M. (2006). Difference in sleep regulation between morning and evening circadian types as indexed by antero-posterior analyses of the sleep EEG. Eur. J. Neurosci. 23, 497–504. doi: 10.1111/j.1460-9568.2005.04561.x
Park, H. R., Choi, S. J., Jo, H., Cho, J. W., and Joo, E. Y. (2020). Effects of evening exposure to light from organic light-emitting diodes on melatonin and sleep. J. Clin. Neurol. 16, 401–407. doi: 10.3988/jcn.2020.16.3.401
Patel, S. R., Zhu, X., Storfer-Isser, A., Mehra, R., Jenny, N. S., Tracy, R., et al. (2009). Sleep duration and biomarkers of inflammation. Sleep 32, 200–204. doi: 10.1093/sleep/32.2.200
Pedersen, T. T., Sunde, E., Wisor, J., Mrdalj, J., Pallesen, S., and Gronli, J. (2022). Sleep homeostasis and night work: a polysomnographic study of daytime sleep following three consecutive simulated night shifts. Nat Sci Sleep. 14, 243–254. doi: 10.2147/NSS.S339639
Purcell, S., Manoach, D., Demanuele, C., Cade, B., Mariani, S., Cox, R., et al. (2017). Characterizing sleep spindles in 11, 630 individuals from the National Sleep Research Resource. Nat. Commun. 8:15930. doi: 10.1038/ncomms15930
Siclari, F., and Tononi, G. (2016). “Sleep and dreaming” in The neurology of consciousness. eds. S. Laureys, O. Gosseries, and G. Tononi. 2nd ed (San Diego, CA: Academic Press)
Sletten, T. L., Ftouni, S., Nicholas, C. L., Magee, M., Grunstein, R. R., Ferguson, S., et al. (2017). Randomised controlled trial of the efficacy of a blue-enriched light intervention to improve alertness and performance in night shift workers. Occup. Environ. Med. 74, 792–801. doi: 10.1136/oemed-2016-103818
Steriade, M. (2006). Grouping of brain rhythms in corticothalamic systems. Neuroscience 137, 1087–1106. doi: 10.1016/j.neuroscience.2005.10.029
Sunde, E., Pedersen, T., Mrdalj, J., Thun, E., Gronli, J., Harris, A., et al. (2020). Blue-enriched white light improves performance but not subjective alertness and circadian adaptation during three consecutive simulated night shifts. Front. Psychol. 11:2172. doi: 10.3389/fpsyg.2020.02172
Wright, K. P. Jr., Bogan, R. K., and Wyatt, J. K. (2013). Shift work and the assessment and management of shift work disorder (SWD). Sleep Med. Rev. 17, 41–54. doi: 10.1016/j.smrv.2012.02.002
Keywords: shift work, sleep EEG, light exposure, electrophysiology, EEG spectral analysis, circadian misalignment
Citation: Yook S, Choi SJ, Zang C, Joo EY and Kim H (2024) Are there effects of light exposure on daytime sleep for rotating shift nurses after night shift?: an EEG power analysis. Front. Neurosci. 18:1306070. doi: 10.3389/fnins.2024.1306070
Received: 03 October 2023; Accepted: 11 March 2024;
Published: 27 March 2024.
Edited by:
Susana Vacas, Massachusetts General Hospital and Harvard Medical School, United StatesReviewed by:
Yuan Shi, Sichuan University, ChinaCopyright © 2024 Yook, Choi, Zang, Joo and Kim. This is an open-access article distributed under the terms of the Creative Commons Attribution License (CC BY). The use, distribution or reproduction in other forums is permitted, provided the original author(s) and the copyright owner(s) are credited and that the original publication in this journal is cited, in accordance with accepted academic practice. No use, distribution or reproduction is permitted which does not comply with these terms.
*Correspondence: Eun Yeon Joo, ZWpvb0Bza2t1LmVkdQ==
†These authors have contributed equally to this work and share first authorship
Disclaimer: All claims expressed in this article are solely those of the authors and do not necessarily represent those of their affiliated organizations, or those of the publisher, the editors and the reviewers. Any product that may be evaluated in this article or claim that may be made by its manufacturer is not guaranteed or endorsed by the publisher.
Research integrity at Frontiers
Learn more about the work of our research integrity team to safeguard the quality of each article we publish.