- 1Section of Developmental Neurobiology, Department of Neurology, University Hospital Würzburg, Würzburg, Germany
- 2Division of Neurodegenerative Diseases, Department of Neurology, Heidelberg University Hospital and Faculty of Medicine, Heidelberg, Germany
- 3Center of Mental Health, Department of Psychiatry, Psychosomatics and Psychotherapy, University of Würzburg, Würzburg, Germany
- 4TSE Systems GmbH, Berlin, Germany
- 5Institut du Cerveau – Paris Brain Institute, Inserm, Sorbonne Université, Paris, France
- 6EVerZom, Paris, France
- 7INCIA, CNRS, EPHE, Université de Bordeaux, Bordeaux, France
- 8Department of Neurology, University Hospital Essen, Essen, Germany
- 9Institute of Neuronal Cell Biology, Technical University Munich, Munich, Germany
We have previously demonstrated that neuroinflammation by the adaptive immune system acts as a robust and targetable disease amplifier in a mouse model of Spastic Paraplegia, type 11 (SPG11), a complicated form of Hereditary Spastic Paraplegia (HSP). While we identified an impact of neuroinflammation on distinct neuropathological changes and gait performance, neuropsychological features, typical and clinically highly relevant symptoms of complicated HSPs, were not addressed. Here we show that the corresponding SPG11 mouse model shows distinct behavioral abnormalities, particularly related to social behavior thus partially reflecting the neuropsychological changes in patients. We provide evidence that some behavioral abnormalities can be mitigated by genetic inactivation of the adaptive immune system. Translating this into a clinically applicable approach, we show that treatment with the established immunomodulators fingolimod or teriflunomide significantly attenuates distinct behavioral abnormalities, with the most striking effect on social behavior. This study links neuroinflammation to behavioral abnormalities in a mouse model of SPG11 and may thus pave the way for using immunomodulators as a treatment approach for SPG11 and possibly other complicated forms of HSP with neuropsychological involvement.
Introduction
Genetically mediated disorders of the central nervous system (CNS) are usually orphan diseases with poor treatment options (Groh and Martini, 2017). Lack of knowledge of the basic pathomechanism is often the cause of limited therapeutic options. The situation is even more complicated since many genetically mediated diseases are characterized by not only physical manifestations, like motor symptoms, but display additional psychological involvement. Neuropsychological features are often present in many CNS diseases like Alzheimer’s disease (AD), Parkinson’s disease (PD), Multiple Sclerosis (MS), and multiple system atrophy (Becker et al., 1988; St Clair et al., 1988; Brassington and Marsh, 1998; Barcelos et al., 2018). However, despite its substantial relevance for patients, relatives, and treating clinicians, the pathomechanisms leading to neuropsychological symptoms remain mainly elusive. Interestingly, primary neuropsychological disorders are often associated with low-grade secondary inflammation, as seen for example in depressive disorder (Miller and Raison, 2016; Beurel et al., 2020; Majd et al., 2020), schizophrenia (Kirkpatrick and Miller, 2013; Upthegrove and Khandaker, 2020; Sun et al., 2022), bipolar disorder (Pereira et al., 2021; Saccaro et al., 2021), and borderline personality disorder (MacDowell et al., 2020; Saccaro et al., 2021). In a mouse mutant related to abnormal CNS myelin, displaying catatonic features, secondary inflammation has also been shown to lead to behavioral changes implicated in schizophrenia, bipolar and depressive disorders, suggesting that inflammation can be causally involved in the development of abnormal behavior in mice (Hagemeyer et al., 2012; Janova et al., 2018).
While the leading feature of Hereditary Spastic Paraplegia (HSP) is spasticity of the lower limbs due to axon degeneration in the corticospinal tract, there are complicated forms of HSPs, like HSP type 11 (SPG11), that show superimposed clinical and neurological features of high variance (Klebe et al., 2015; Boutry et al., 2019). Cognitive impairment and neuropsychological symptoms including anxiety, learning and memory difficulties, and changes in social behavior (Denora et al., 2009; Wijemanne et al., 2015; Faber et al., 2018; Utz et al., 2022) are usually present in patients with SPG11. We could previously demonstrate that secondary neuroinflammation by the adaptive immune system acts as a robust disease amplifier in a mouse model of SPG11, leading to remarkable aggravation of histopathological and gait alterations (Hörner et al., 2022). In the present study, we aimed to (i) characterize possible neuropsychological symptoms in the corresponding mouse model and (ii) investigate the possibility whether these symptoms are, at least partially, mediated or aggravated by neuroinflammation and may, thus, be treatable by immunomodulators.
We provide evidence that our SPG11 model shows distinct behavioral abnormalities, including abnormal social, impulsivity-like, anxiety-like, and hyperactivity-like behavior, which partially reflects neuropsychological changes seen in patients (Wijemanne et al., 2015; Faber et al., 2018; Utz et al., 2022; Klebe et al., unpublished data). Importantly, we also show that genetically inactivating the adaptive immune system ameliorates distinct disease-related behavioral abnormalities. Translating this into a clinically applicable approach, we show that treatment with the established immunomodulators fingolimod (FTY720) or teriflunomide, that are in use for treatment of MS, leads to an attenuation of distinct behavioral abnormalities. Together with our previous findings, this may be the base for the clinical use of approved immunomodulators in SPG11 patients and, possibly, other patients with complicated forms of HSP.
Materials and methods
Animals
All mice were on a C57BL/6J genetic background. Animals were group-housed and kept in individually ventilated cages under barrier conditions at the Center of Experimental Molecular Medicine, University of Würzburg with a 14 h/10 h day/night rhythm (<300 lux during day). Mice were group-housed with sex-matched littermates (3–5 mice/cage). The number of mice housed together was comparable among all experimental groups. No aggressive behavior between the individuals was observed. Colonies were maintained at 20–24°C and 40–60% humidity, with free access to food and water. All animal experiments were approved by the local authority of the Government of Lower Franconia, Germany.
Spg11-knockout (Spg11−/−) mice were used as an SPG11 disease model, and age-matched wild-type (wt, Spg11+/+) littermates were used as control animals (Branchu et al., 2017). B6J refers to C57BL/6 J mice bought from Charles River Laboratories. Mice of either sex were analyzed for histopathological readout parameters, as we did not detect sex-related differences in Spg11+/+ or Spg11−/− mice. Genotypes were determined by conventional PCR using isolated DNA from ear punch biopsies as previously described (Branchu et al., 2017). In experiments aimed to genetically inactivate the adaptive immune reactions, Spg11−/− mice were crossbred with Rag1-deficient (Rag1−/−) mice (Mombaerts et al., 1992), devoid of mature T- and B-lymphocytes, according to previously published protocols (Ip et al., 2006; Groh et al., 2013). Rag1−/− mice did not display any abnormalities in longevity and body weight under the standardized conditions applied (specific pathogen-free). For behavioral analysis Spg11+/+, Spg11−/−, Spg11−/−Rag1−/− and B6J mice were divided by sex to minimize sex-bias. For each group a minimum of 3–5 animals per genotype and sex were tested (Tables 1, 2).
Immunomodulatory treatment
Fingolimod (FTY720; Sigma-Aldrich; SML0700) was dissolved in autoclaved water at 3 μg per milliliter and provided ad libitum, corresponding to a dose of 0.5 mg/kg body weight in a mouse with an average body weight of 30 g and an approximate water consumption of 5 ml per day. Teriflunomide (Biorbyt, orb146201) was dissolved in autoclaved drinking water containing 0.6% Tween 80 at 60 μg per milliliter and provided ad libitum, corresponding to a dose of 10 mg/kg body weight per day. These concentrations are based on previous animal experiments of our group (Groh et al., 2017; Hörner et al., 2022; Abdelwahab et al., 2023) and other laboratories (Metzler et al., 2008; Merrill et al., 2009), implicating dose conversion scaling (Nair and Jacob, 2016). Similar to previous studies, Spg11−/− mice receiving water containing only 0.6% Tween 80 did not show any abnormalities in inflammation, longevity, gross behavior, or body weight (data not shown; Groh et al., 2016, 2017, 2018, 2021a; Hörner et al., 2022). Autoclaved drinking water without the compounds was provided to non-treated controls. The water with or without compounds was changed weekly. Mice were treated for 450 days, from 3 months of age until the end of the last series of tests and monitored daily regarding defined burden criteria and phenotypic abnormalities. No obvious side effects were detectable during treatment.
Behavioral evaluation
A behavioral test battery was designed to longitudinally investigate mice (Figure 1). The test battery consisted of the cliff avoidance reaction (CAR) analysis for investigation of impulsivity-like behavior (Figure 1A), the dark/light box (DLB) analysis for investigation of anxiety-like behavior (Figure 1B), the open field (OF) analysis for investigation of exploratory and hyperactivity-like behavior (Figure 1C), the novel object recognition (NOR) analysis for investigation of memory and recognition (Figure 1D), and the social interaction and novelty (SI) analysis for investigation of social interest and novelty behavior (Figure 1E). The tests were performed starting with less stress-inducing tests, followed by more stress-inducing tests, while in the results section, the order of presentation reflects the relevance of our findings for possible translatability to patients. Two tests per week were performed, with at least two and a maximum of four test-free days in between. All tests were recorded using a camera and evaluated using the EthoVisionXT (Noldus Information Technology) video tracking software v.116. All tests were performed in a room with only the investigator present. Mice were placed in the testing room to habituate 30 min prior to each test. Between each trial, arenas, objects and/or cages, were cleaned using 10% ethanol and the arena was aired out for approximately 3 min to minimize behavioral disturbance by olfaction. No more than two tests per week were performed to minimize stress bias. The investigator was unaware of genotypes and possible treatment of the mice during testing.
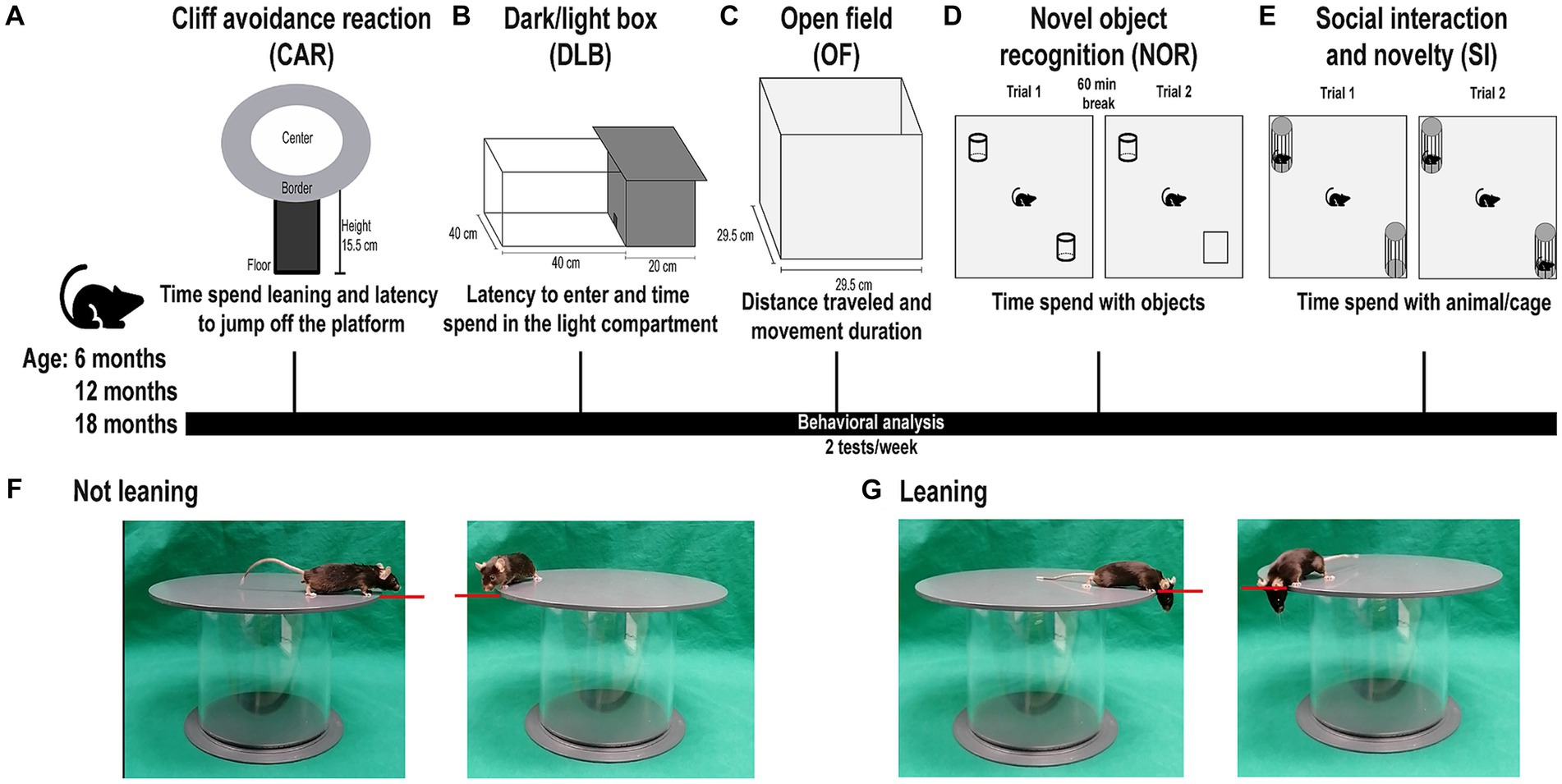
Figure 1. Behavioral test battery for mice. Representative image of the behavioral test battery mice were subjected to in the following order: cliff avoidance reaction (CAR), dark/light box (DLB) analysis, open field (OF) analysis, novel object recognition (NOR), and social interaction and novelty (SI) analysis at the age of 6, 12, and 18 months. The order is structured from less stress-inducing to more stress-inducing tests. Each week a maximum of two tests was performed with a minimum two-day break between tests. (A) Graphic depiction of CAR analysis. The platform is approximately 15.5 cm high, non-transparent, and divided into a center (white) and a border (gray) zone. A mouse is placed in the middle of the platform and the time spend leaning and latency to jump off the platform is measured. (B) Graphic depiction of DLB analysis. The DLB consists of a light compartment (<300 lx) and a dark compartment, connected by a doorway through which the mouse can walk freely. A mouse is placed in the dark compartment and the latency to enter, and time spend in the light compartment is measured. (C) Graphic depiction of OF analysis. A mouse is placed in a wooden and non-transparent OF box. The distance traveled and movement duration of mice is measured. (D) Graphic depiction of NOR analysis. A mouse is placed in an OF box, containing two identical objects. After a 60-min break, one object is replaced by an unfamiliar object of similar proportions and the mouse is placed back into the box. The time mice spend with the objects is measured. (E) Graphic depiction of SI analysis. A mouse is placed in an OF box containing two small cages. In the social interest trial (trial 1), one cage contains an unfamiliar mouse, while the other cage remains empty. In the social novelty trial (trial 2) a second unfamiliar mouse is placed in the empty cage. The time mice spend with the animal/cage is measured. (F) Representative images of mice not leaning over the edge of the CAR platform: A horizontal axis (red line) defines if a mouse is leaning. If the eyes of the mouse do not cross the horizontal axis of the platform rim the mouse is not considered leaning. (G) Representative images of mice leaning over the edge of the CAR platform: If the eyes of the mouse cross the horizontal axis of the platform rim the movement is considered leaning. Note that the order of presentation of tests in the results section is different as there it reflects the relevance of our findings for possible translatability to patients.
Cliff avoidance reaction
To assess impulsivity-like behavior, the CAR analysis was used. CAR was evaluated by using a round, non-transparent, plastic platform (diameter: 25 cm; thickness: 2 cm), supported by a plastic rod (height: 15.5 cm) (Figure 1A). The platform was divided into a center zone (diameter: 20 cm) and a border zone (width: 2.5 cm). To initiate the test, one mouse was gently placed into the center of the platform. The test lasted 10 min. The investigator recorded the latency to jump off the platform and the time the mouse spent leaning over the edge of the platform. A behavior was defined as “jumping behavior,” when a mouse voluntarily jumped off the platform. If a mouse jumped off the platform during the test, it was gently placed back in the middle of the platform. The definition of “leaning over the edge of the platform” is depicted in Figure 1. A behavior was considered “leaning over the edge of the platform” when the eyes of the mouse crossed the horizontal axis of the platform rim (Figures 1F,G).
Dark/light box analysis
To evaluate anxiety-like behavior in mice, DLB analysis was used. The DLB consists of a dark compartment (20 cm × 40 cm), and a light compartment (40 cm × 40 cm; <300 lx), connected by a small doorway (Figure 1B). To begin the experiment, one mouse was placed in a random corner of the dark compartment of the box, facing the wall and away from the doorway. Corners were randomly alternated between mice to minimize bias. The mouse was allowed to explore freely and was recorded in the light, but not dark compartment. After 10 min, the mouse was taken out of the arena, disregarding if it had entered the light compartment or not. The investigator recorded the time until entering the light compartment of the arena and the time spent in the light compartment.
Open field analysis
To evaluate the general exploratory and hyperactivity-like behavior of mice, the OF analysis was used. To begin the experiment, one mouse was gently placed in a corner of a white, non-transparent, wooden box (29.5 cm × 29.5 cm), facing the wall (Figure 1C). The corners were randomly selected and alternated between different mice. One trial lasted a total of 10 min. Total walking distance and movement duration was assessed by the EthoVisionXT software.
Novel object recognition
For assessment of non-spatial learning and recognition, the NOR analysis was used as previously described (Groh et al., 2021b). The test was performed in an OF box (29.5 cm × 29.5 cm), which was divided into quadrants of the same size. For the training trial, two identical objects were placed in opposite quadrants of the arena (Figure 1D). The quadrants were randomly alternated between tests to minimize bias. To start the experiment, one mouse was gently placed into a corner of a quadrant not containing an object, facing the wall. The corners were randomly selected and alternated between mice. After the training trial, the mouse was taken out of the arena and placed back in its home cage for 60 min. For the test trial, one of the objects was replaced by an unfamiliar object that was of similar dimensions and colors but yielded enough discrimination properties and did not show spontaneous preference in prior testing (data not shown). The mouse was placed back into a corner of the arena, in a quadrant not containing an object, facing the wall. During both trials, the mouse was allowed to explore freely for 10 min while being recorded. In both trials, the time spend actively sniffing or touching each object was recorded by the investigator. The object preference was calculated for training and test trial: [time spent with object 1/time spent with object 2] × 100. In the test trial object 1 refers to the unfamiliar object.
Social interaction and novelty analysis
For assessment of social interaction and novelty behavior, the SI analysis was used. Two small, identical cages were placed in an OF box (29.5 cm × 29.5 cm) in diagonal corners of the arena (Figure 1E). The corners containing the cages were chosen randomly and alternated between different tests to minimize bias. In the social interest trial (trial 1), one of the cages contained a sex-matched mouse that was unfamiliar to the test mouse. The cage containing the mouse was chosen randomly and alternated between tests to minimize bias. The test mouse was gently placed in one of the corners of the arena, not containing a cage, facing the wall. The corners were alternated randomly between mice. The social novelty trial (trial 2) was carried out directly following the social interest trial (trial 1). A second, unknown, sex-matched mouse was placed in the empty cage of the arena. To start the social novelty trial (trial 2), the test mouse was gently placed in a corner, not containing a cage, facing the wall. The test mouse was allowed to explore freely for 10 min. The time spent with the unfamiliar mice or the empty cage (direct interaction; sniffing or touching) was recorded by the investigator. The social preference was calculated for both trials. Social interest preference (trial 1): [time spent with animal 1/time spent with empty cage] × 100. Social novelty preference (trial 2): [time spent with animal 2/time spent with animal1] × 100. Animal 1 being the first unfamiliar animal, and animal 2 being the second unfamiliar animal. When the unfamiliar mice were taken out of the cages, the cages were cleaned using 10% ethanol and given time to air out for approximately 3 min. The mice used as unfamiliar mice were placed in the cages for 5 min each day starting 3 days prior to testing day for habituation.
Dissection and processing of nervous tissue
Mice were euthanized using CO2 (according to guidelines by the State Office of Health and Social Affairs Berlin) and transcardially perfused with phosphate-buffered saline (PBS) containing heparin followed by 2% paraformaldehyde (PFA) in PBS. Subsequently, tissue was harvested, post-fixed, dehydrated and processed as previously described (Groh et al., 2016). Before embedding of the brain, the olfactory bulb was removed, the cerebrum and cerebellum were separated at defined positions and embedded in TissueTek ® O.C.T.™ compound.
Histochemistry and immunofluorescence
Immunohistochemistry was performed on 40 μm thick coronal cerebellum cryo-sections (Bregma −6.00 to −6.30 mm). Sections were washed and blocked using 5% bovine serum albumin/1% normal goat serum/0.3% Triton X100 in PBS. Sections were incubated over night at 4°C with the following primary antibody: mouse anti-calbindin (1: 500, Swant Inc., 300). Primary antibody binding was visualized using a fluorescently labeled anti-mouse secondary antibody (1:300, Dianova). Fluorescence images were acquired using an Axio Imager M2 microscope (Zeiss; Objective: 20x/0.8 Ph2 M27) with ApoTome.2 structured illumination equipment, attached Axiocam cameras and corresponding software (ZEN v.2.3 blue edition). Images were minimally processed (rotation and cropping) using Photoshop CS6 (Adobe). For quantification of Purkinje cells (PC), calbindin+ cells were counted in at in at least three coronal cerebellum cryo-sections for each animal and related to the area of the section using the cell counter plugin in ImageJ (National Institutes of Health, Bethesda USA).
Experimental design and statistical analysis
Quantifications and clinical analyses were performed by investigators unaware of the genotype and potential treatments of mice, by assigning uniquely coded labels to animals. Mice were randomly placed into experimental and control groups according to genotyping results and by using a random generator.1 For biometrical sample size estimation, the G*Power program (version 3.1.3) was used (Faul et al., 2007). Calculation of the appropriate sample size groups was performed in a priori power analysis by comparing the mean of three to four groups with a defined adequate power of 0.8 (1-beta-error) and an α-error of 0.05. To determine the prespecified effect size d, previously published data were considered comparable reference values (Groh et al., 2017, 2021a; Hörner et al., 2022). Statistical analysis was performed using Prism 8 (GraphPad). The Shapiro–Wilk test was used to assess data sets for normal distribution and the F test was used to check for variance homology. If more than two groups or multiple timepoints (behavioral analysis) were compared, differences were evaluated by one-way ANOVA, followed by Sidak’s post-hoc test. Significance levels are indicated by asterisks (*) and considered significant according to the following scheme: *p < 0.05; **p < 0.01; ***p < 0.001. Measurements and quantifications are shown as individual values (circles, squares, triangles = mean value of one mouse) and mean ± standard deviation (SD). Graphs were generated using Prism 8.
Results
Spg11−/− mice display distinct behavioral abnormalities.
Patients suffering from complex HSP forms often present with distinct neuropsychological features, including anxiety, hyperactivity, impulsivity, learning and memory difficulties, and changes in social behavior (Osmolak et al., 2012; Wijemanne et al., 2015; Faber et al., 2018; Utz et al., 2022). We here examined whether similar behavioral alterations are detectable in Spg11−/− mice by using a behavioral test battery comprising tests for social interaction and novelty behavior, novel object recognition, general exploratory, and hyperactivity-like behavior, anxiety-like behavior, and impulsivity-like behavior (Figure 1).
The social interaction and novelty (SI) analysis comprises a social interest (trial 1) and novelty trial (trial 2) (Figure 1E). In both trials, the time the test mice spend directly sniffing or touching the unfamiliar mice or the empty cage is recorded (Figure 1E). Male and female Spg11−/− mice showed a significant reduction of social interest and novelty preference compared to wt mice at 18 months of age (Figures 2A,B,D). Furthermore, Spg11−/− mice of both sexes spent less time with the unfamiliar animals during the social novelty trial (trial 2) compared to their wt littermates (Figures 2C,E), reflecting abnormal social behavior. Interestingly, while male Spg11−/− mice showed a reduction in time spent with the unfamiliar animal only at 18 months of age, female Spg11−/− mice spent less time with the unfamiliar animals already at 12 months, though the social novelty preference was still comparable to wt mice (Figures 2A–E). Of note, the novel object recognition, a measure of non-spatial memory and recognition (Figure 1D), was not altered in male or female Spg11−/− mice at any investigated age (Supplementary Figures S1a,b).
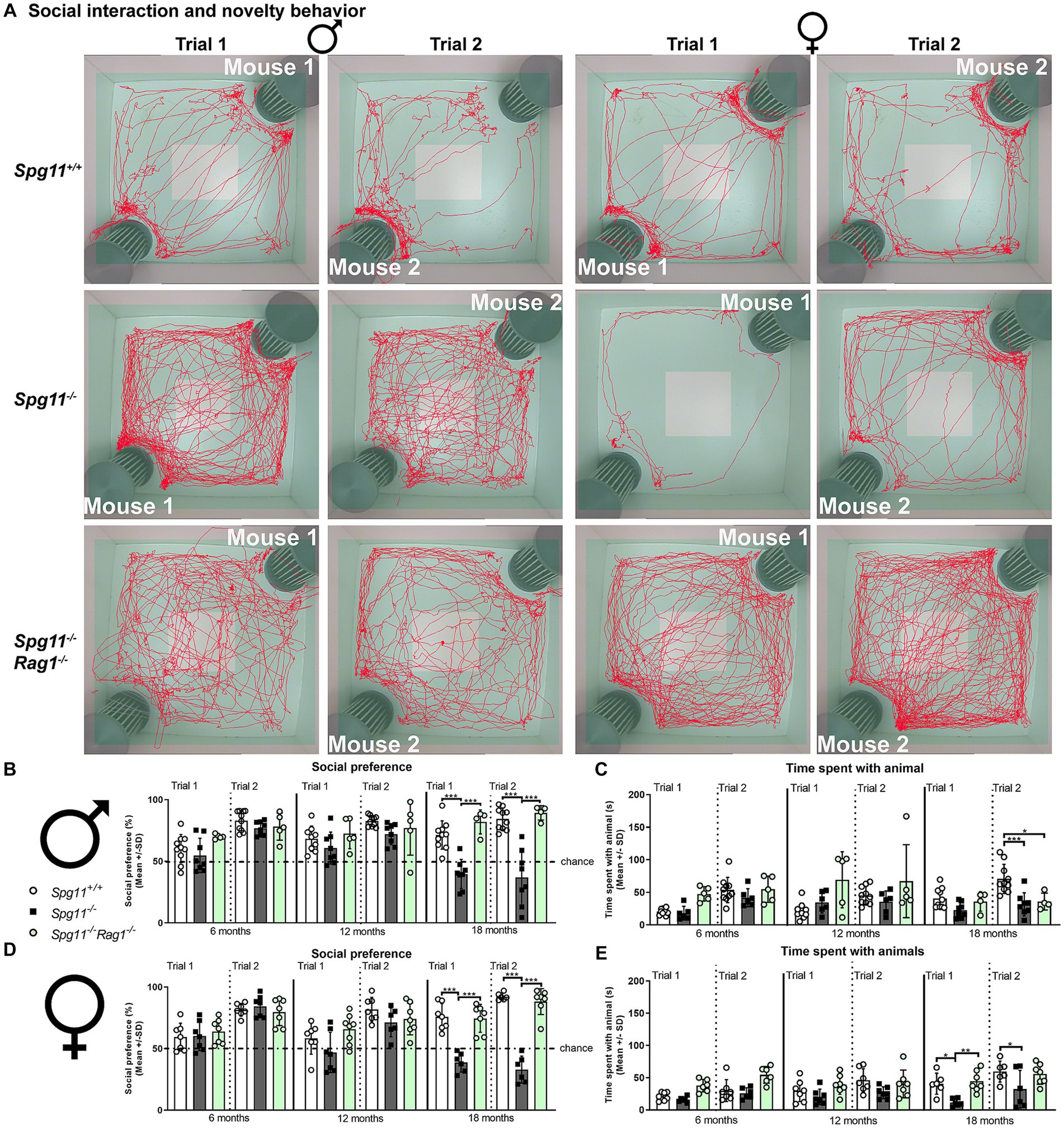
Figure 2. Spg11−/− mice show social abnormalities, which are ameliorated by Rag1-deficiency. (A) Representative images of social interest (trial 1, 1st, and 3rd column) and novelty (trial 2, 2nd, and 4th column) analysis of 18-month-old male (1st and 2nd column) and female (3rd and 4th column) Spg11+/+ (top row), Spg11−/− mice (2nd row), and Spg11−/−Rag1−/− mice (bottom row). Light square indicates the center zone of the arena, green area indicates the border zone. Red line represents the walking track. Mouse 1 indicates the cage in which the first unfamiliar mouse is placed, mouse 2 in which the second unfamiliar mouse is placed. Empty indicates the empty cage. (B) Social interest and novelty preference of 6-, 12-, and 18-month-old male mice. Male Spg11−/− mice show lower social interest and novelty preference compared to wt mice at 18 months, which is restored to wt levels by Rag1-deficiency [F(17, 117) = 12.14, p < 0.0001]. (C) Time spent with unfamiliar animals of male mice at 6, 12, and 18 months. Male Spg11−/− mice less time with the unfamiliar animals during the social novelty trial (trial 2) compared to wt littermates at 18 months. This is not altered by Rag1-deficiency [F(17, 110) = 5.077, p < 0.0001]. (D) Social interest and novelty preference of 6-, 12-, and 18-month-old female mice. Female Spg11−/− mice show lower social interest and novelty preference compared to their wt littermates at 18 months, which is restored to wt levels by Rag1-deficiency [F(23, 131) = 10.55, p < 0.0001]. (E) Time spent with unfamiliar animals of female mice at 6, 12, and 18 months. Female Spg11−/− mice spend less time with the unfamiliar animals compared to their wt littermates in the social interest trial (trial 1) at 18 months, and in the social novelty trial (trial 2) at 12 and 18 months. Rag1-deficiency increases the time female Spg11−/− mice spend with the unfamiliar animals in the social interest (trial 1) and novelty trial (trial 2) [F(17, 94) = 4.875, p < 0.0001]. Chance indicates equal time spent with the empty cage/animals (50%). Error bars represent standard deviations (circles, squares = value of one mouse). Significance Spg11−/− compared to Spg11+/+ and Spg11−/−Rag1−/− compared to Spg11+/+ and Spg11−/− mice is determined by one-way ANOVA and Sidak’s post hoc test (*p < 0.05, **p < 0.01, ***p < 0.001).
To investigate exploratory and hyperactivity-like behavior, we performed the open field (OF) analysis, measuring the distance mice traveled and their movement duration (Figure 1C). Male Spg11−/− mice walked longer distances and showed a higher movement duration at 6 and 18 months of age compared to wt mice (Figures 3A–C), indicating hyperactivity-like behavior. Of note, the time male Spg11−/− mice spent in the center zone of the arena remained unaffected (data not shown). In contrast, female Spg11−/− showed an increased walking distance and movement duration only at 18 months of age (Figures 3A,D,E) and the center time remained unaffected compared to wt mice at all ages investigated (data not shown).
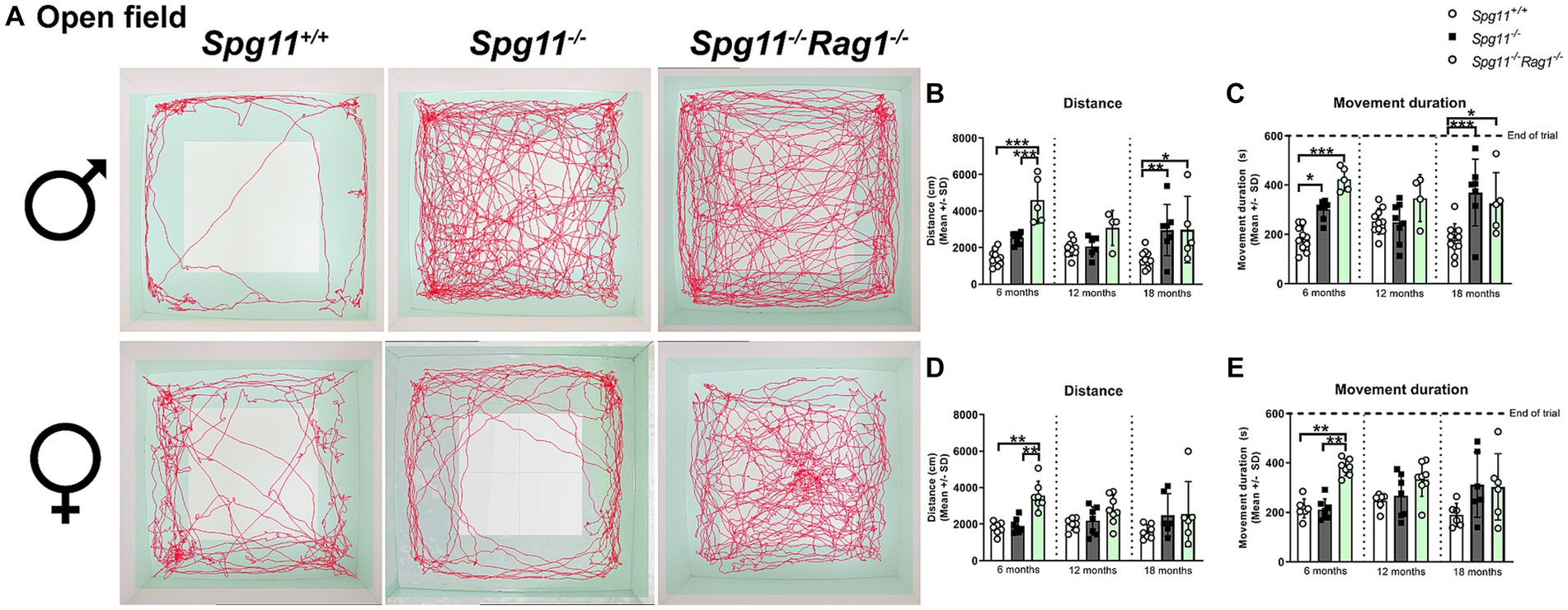
Figure 3. Spg11−/− mice show hyperactivity-like behavior, which is not ameliorated by Rag1-deficiency. (A) Representative images of OF analysis of 18-month-old male (top row) and female (bottom row) Spg11+/+, Spg11−/−, and Spg11−/−Rag1−/− mice. Light square indicates the center zone of the arena, green area indicates the border zone. Red line represents the walking track. (B) Male Spg11−/− mice walk a greater distance and (C) show a higher movement duration than their wt littermates at 6 and 18 months. This is not reduced by Rag1-deficiency. Of note, male Rag1-deficienct Spg11−/− mice walk a greater distance and show a higher movement duration compared to wt and Spg11−/− mice at 6 months [distance: F(11, 79) = 6.021, p < 0.0001; movement duration: F(11, 81) = 5.389, p < 0.0001]. (D) Female Spg11−/− mice walk a greater distance and (E) show a higher movement duration compared to their wt littermates at 18 months. This is not reduced by Rag1-deficiency. Of note, female Rag1-deficienct Spg11−/− mice walk a greater distance and show a higher movement duration compared to wt and Spg11−/− mice at 6 months [distance: F(11, 65) = 3.037, p = 0.0025; movement duration: F(11, 61) = 3.455, p = 0.0009]. Error bars represent standard deviations (circles, squares = value of one mouse). Significance of Spg11−/− compared to Spg11+/+ mice and of Spg11−/−Rag1−/− compared to Spg11+/+ and Spg11−/− mice is determined by one-way ANOVA and Sidak’s post hoc test (*p < 0.05, **p < 0.01, ***p < 0.001).
To assess the anxiety-like behavior in this mouse model, we performed the commonly used dark/light box (DLB) analysis, recording the latency to enter and the time mice spend in the light compartment (Figure 1B). While we did not see differences in the behavior of male Spg11−/− mice compared to their wt littermates (Figures 4A–C), we detected distinct differences between female Spg11−/− and Spg11+/+ mice at 18 months of age (Figures 4A,D,E). While female Spg11−/− mice did not show an altered latency to enter the light compartment, they showed a strong tendency to spend more time in the light compartment than their wt littermates (Figures 4D,E), indicating reduced anxiety-like behavior.
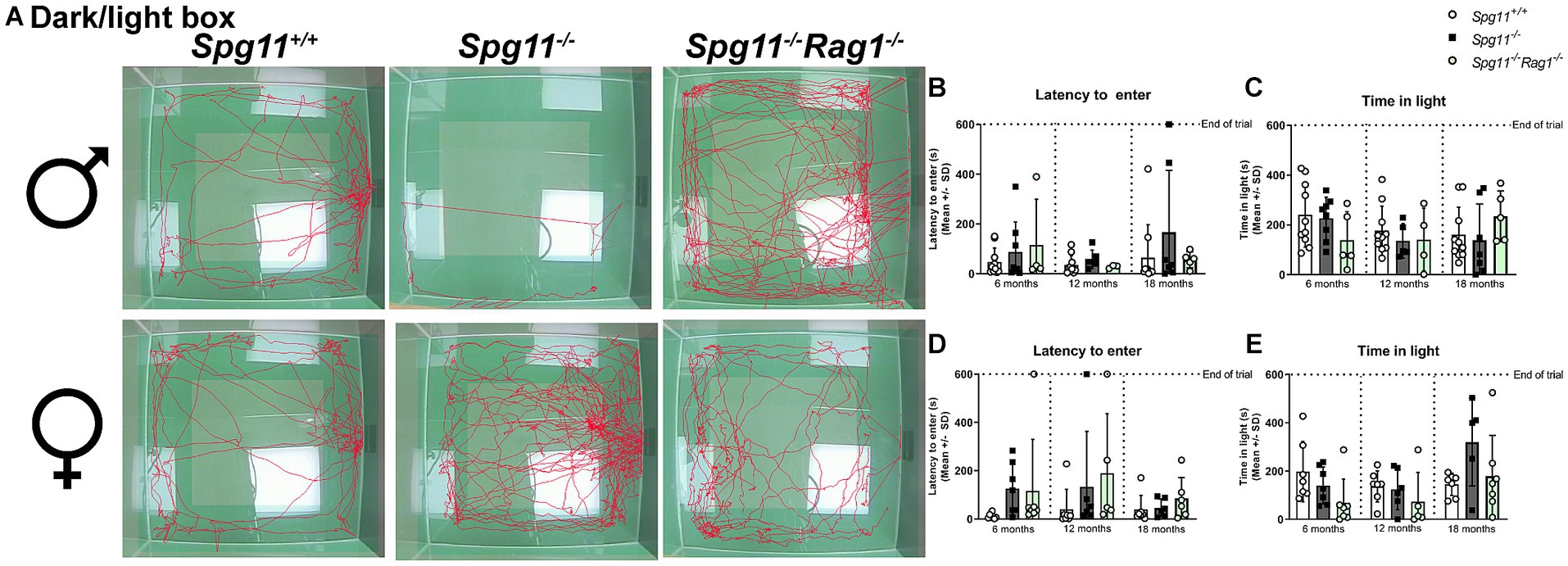
Figure 4. Female Spg11−/− mice show reduced anxiety-like behavior, which is restored by Rag1-deficiency. (A) Representative images of DLB analysis of 18-month-old male (top row) and female (bottom row) Spg11+/+, Spg11−/−, and Spg11−/−Rag1−/− mice. Light square indicates the center zone of the arena. Red line represents the walking track. (B) Male Spg11−/− or Spg11−/–Rag1−/− mice do not show an altered latency to enter the light compartment, or (C) time spend in the light compartment compared to wt mice at any investigated age [latency to enter: F(11, 73) = 1.675, p = 0.0964; time spend in light compartment: F(11, 74) = 2.084, p = 0.0319]. (D) Female Spg11−/− or Spg11−/−Rag1−/− mice do not show an altered latency to enter the light compartment at any investigated age [F(11, 61) = 1.098, p = 0.3785]. (E) Female Spg11−/− show a strong tendency towards more time spend in the light compartment than their wt littermates at 18 months. This is restored by Rag1-deficiency [F(11, 62) = 2.095, p = 0.0339]. Error bars represent standard deviations (circles, squares = mean value of one mouse). Significance of Spg11−/− compared to Spg11+/+ and Spg11−/−Rag1−/− compared to Spg11+/+ and Spg11−/− mice is determined by one-way ANOVA and Sidak’s post hoc test.
To assess the impulsivity-like behavior of Spg11−/− mice, we used the cliff avoidance reaction (CAR) analysis (Figure 1A). Here, the time mice spend leaning over the edge of the platform and the latency to jump off the platform is recorded (Figures 1A,F,G). Male Spg11−/− mice spent more time leaning over the edge of the platform at 18 months compared to wt mice (Figures 5A,B). At the same age, they showed a reduced latency to jump off the platform compared to wt littermates, indicating impulsivity-like behavior (Figure 5C). Interestingly, female Spg11−/− mice showed only a tendency to spend more time leaning over the edge and to jump off the platform compared to their wt littermates at 18 months (Figures 5A,D,E). Wt mice of either sex rarely jumped off the platform at any investigated age (Figures 5C,E). Of note, Spg11−/− did not show an increased risk to involuntarily fall off the platform compared to wt littermates.
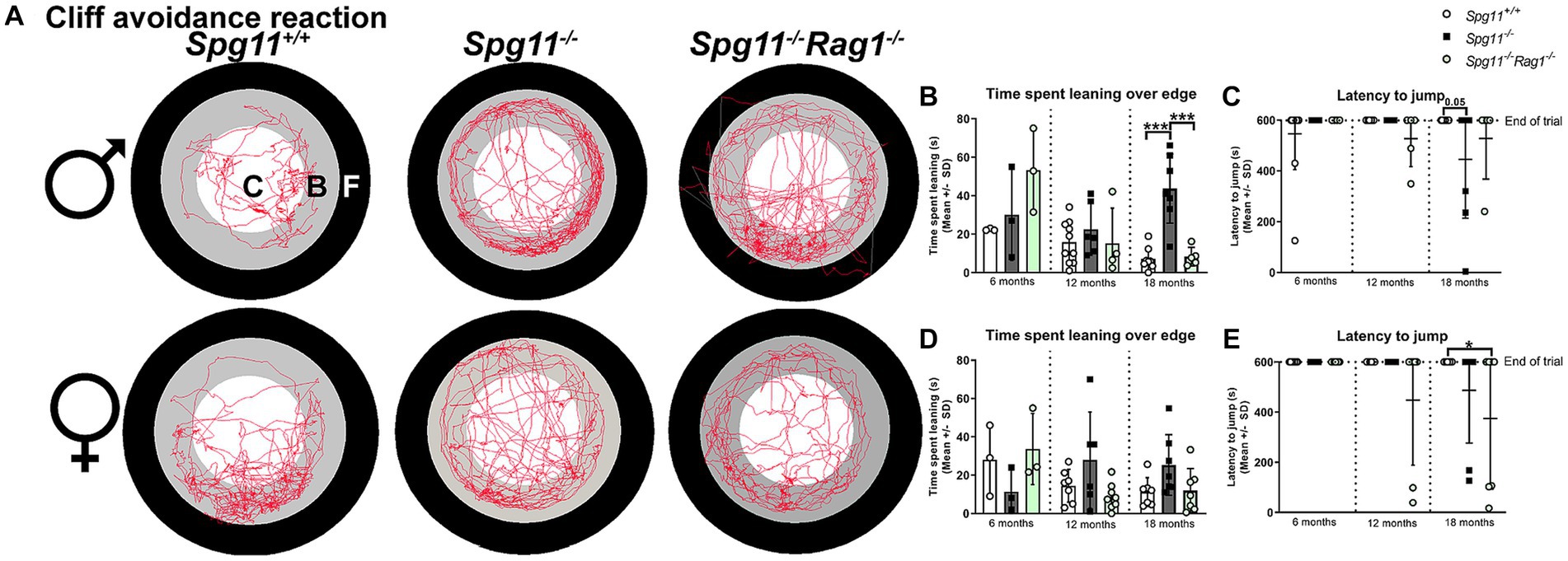
Figure 5. Spg11−/− mice show impulsivity-like behavior, which is partially reduced by Rag1-deficiency. (A) Representative images of CAR analysis of 18-month-old male (top row) and female (bottom row) Spg11+/+, Spg11−/−, and Spg11−/−Rag1−/− mice. Red line represents the walking track (C = center zone, white; B = border zone, grey; F = floor zone, black). (B) Male Spg11−/− mice spend more time leaning over the edge of the platform at 18 months. This is reduced by Rag1-deficiency [F(11, 55) = 8.024, p < 0.0001]. (C) Male Spg11−/− and Spg11−/−Rag1−/− mice show a lower latency to jump off the platform compared to their wt littermates at 18 months [F(8, 54) = 1.643, p = 0.1343]. (D) Female Spg11−/− show a tendency to spend more time leaning over the edge of the platform at 18 months. This is reduced by Rag1-deficiency [F(8, 42) = 2.219, p = 0.0451]. (E) Female Spg11−/− and Spg11−/−Rag1−/− mice show a lower latency to jump off the platform compared to their wt littermates at 18 months [F(8, 59) = 2.684, p = 0.0138]. Error bars represent standard deviations (circles, squares = value of one mouse). Significance of Spg11−/− compared to Spg11+/+ and Spg11−/−Rag1−/− compared to Spg11+/+ and Spg11−/− mice is determined by one-way ANOVA and Sidak’s post hoc test (*p < 0.05, ***p < 0.001).
In summary, we here demonstrate that male and female Spg11−/− mice show distinct behavioral abnormalities, comprising reduced social interest and novelty behavior, as well as hyperactivity-like and impulsivity-like behavior. Additionally, female Spg11−/− mice show reduced anxiety-like behavior. Non-spatial memory and recognition remains unaffected in Spg11−/− mice. Importantly, the detected social abnormalities were not due to a general reduction of exploratory behavior or recognition, or increased anxiety-like behavior. This suggests that this mouse model displays the neuropsychological characteristics of SPG11 to a large degree.
Genetic inactivation of the adaptive immune system ameliorates behavioral abnormalities of Spg11−/− mice
Based on our previous observation that adaptive immune reactions substantially amplify neuropathological features and disturbed gait coordination in this mouse model for SPG11 (Hörner et al., 2022), we here investigated whether immune reactions could mediate or aggravate behavioral abnormalities as well. As a proof-of-principle approach, we evaluated the putative impact of lymphocytes in Spg11−/− mice by crossbreeding them with Rag1-deficient (Rag1−/−) mice, lacking mature T- and B-lymphocytes (Mombaerts et al., 1992). We found that in Spg11−/− mice, Rag1-deficiency restored the social interest and novelty preference of male Spg11−/− mice to wt levels at 18 months of age (Figures 2A,B). Interestingly, even though the social novelty preference (trial 2) was restored, the time spent with the unfamiliar animals during this trial remained reduced (Figure 2C). In female Spg11−/− mice, Rag1-deficiency restored the social interest and novelty preference (Figures 2A,D), and increased the time 18-month-old female knockout mice spent with the unfamiliar animals in both trials (Figure 2E). Thus, abnormalities in the social behavior of female Spg11−/− mice were completely prevented. Rag1-deficiency did not affect the social interest and novelty behavior of male or female Spg11+/+ mice at any investigated age (Supplementary Figures S2a–d). Of note, the novel object recognition of male or female wt or knockout mice was not altered by Rag1-deficiency (Supplementary Figures S1a,b, S2e,f).
Rag1-deficiency did not reduce hyperactivity-like behavior of male or female Spg11−/− mice, as revealed by OF analysis (Figures 3A–E). Surprisingly, male and female Spg11−/−Rag1−/− mice walked a greater distance and showed a higher movement duration than Spg11−/− mice at 6 months of age (Figures 3A–E). While the walking distance and movement duration increased in Spg11−/− mice between 6 and 18 months, it remained unchanged in Rag1-deficient Spg11−/− mice, leading to similar values at 18 months (Figures 3A–E). Rag1-deficiency did not alter the exploratory behavior of male or female Spg11+/+ mice at any investigated age (Supplementary Figures S2g–j).
Regarding anxiety-related parameters, Rag1-deficiency did not lead to any behavioral changes of male Spg11−/− mice in the DLB (Figures 4A–C). While Rag1-deficiency did not alter the latency to enter the light compartment of female Spg11−/− mice (Figures 4A,D), it led to a tendency towards less time spent in the light compartment, a parameter typically increased in 18-month-old female Spg11−/− mice (Figures 4A,E). Of note, Rag1-deficiency led to an increased latency to enter and a tendency to spend less time in the light compartment in male and female (Supplementary Figures S2k–n).
In the CAR, Rag1-deficiency reduced the time spent leaning over the edge of the platform, typically increased in 18-month-old male Spg11−/− mice (Figures 5A,B), and showed the same tendency for female Spg11−/−Rag1−/− mice (Figures 5A,D). However, it did not ameliorate the latency to jump off the platform of male or female Spg11−/− mice (Figures 5C,E). While male Rag1-deficient Spg11+/+ mice spent more time leaning over the platform at 6 months, all other parameters remained unaffected at all investigated ages (Supplementary Figures S2o–r).
The previously presented data indicate that neuropsychological abnormalities are, at least partially, driven by the adaptive immune system. Complementary to our previous study, these findings show that secondary inflammation does not only contribute to neuropathological and gait alterations (Hörner et al., 2022) but also to behavioral abnormalities.
Treatment with fingolimod or teriflunomide ameliorates behavioral abnormalities of Spg11−/− mice
Since we could show that behavioral abnormalities of Spg11−/− mice are, at least partially, driven by the adaptive immune system, we investigated whether immunomodulatory treatment, as a more clinically relevant approach, would lead to similar beneficial effects.
Indeed, we found striking benefits of treatment, starting at 3 months of age, with either fingolimod or teriflunomide on the social behavior of Spg11−/− mice (Figures 6A–E). Treatment restored social interest (trial 1) and novelty preference (trial 2) of 18-month-old male Spg11−/− mice to wt levels (Figures 6A,B). Interestingly, and resembling the findings in Rag1-deficient Spg11−/− mice, treatment did not increase the time male Spg11−/− mice spent with the unfamiliar animals in the social novelty trial at this age (Figure 6C). Remarkably, treatment with teriflunomide did not only restore the social interest (trial 1) and novelty preference (trial 2) of female Spg11−/− mice to wt levels at 18 months, but treated mice also spent significantly more time with the unfamiliar animal in the social interest trial (trial 1), showing the same tendency in the social novelty trial (trial 2) (Figures 6A,D,E). Treatment with fingolimod also increased the social interest and novelty preference of 18-month-old female Spg11−/− mice, albeit not to the same degree as treatment with teriflunomide (Figures 6A,D). Interestingly, treatment with fingolimod did not increase the time female Spg11−/− mice spent with the unfamiliar mice (Figure 6E). The novel object recognition of male or female Spg11−/− mice was not altered by treatment with either immunomodulator (Supplementary Figures S3a,b). To ensure that treatment alone did not change the behavior of mice, a limited number of B6J mice were treated with fingolimod or teriflunomide using the same treatment approach as previously described. Treatment did not alter the social interest (trial 1) or novelty preference (trial 2) of male or female B6J mice compared to untreated littermates (Figures 7A–E). The time treated mice spent with the unfamiliar animals also remained unaffected by either treatment (Figures 7A–E). Moreover, the novel object recognition of male or female B6J mice was not altered by treatment with either immunomodulator (Supplementary Figures S4a,b).
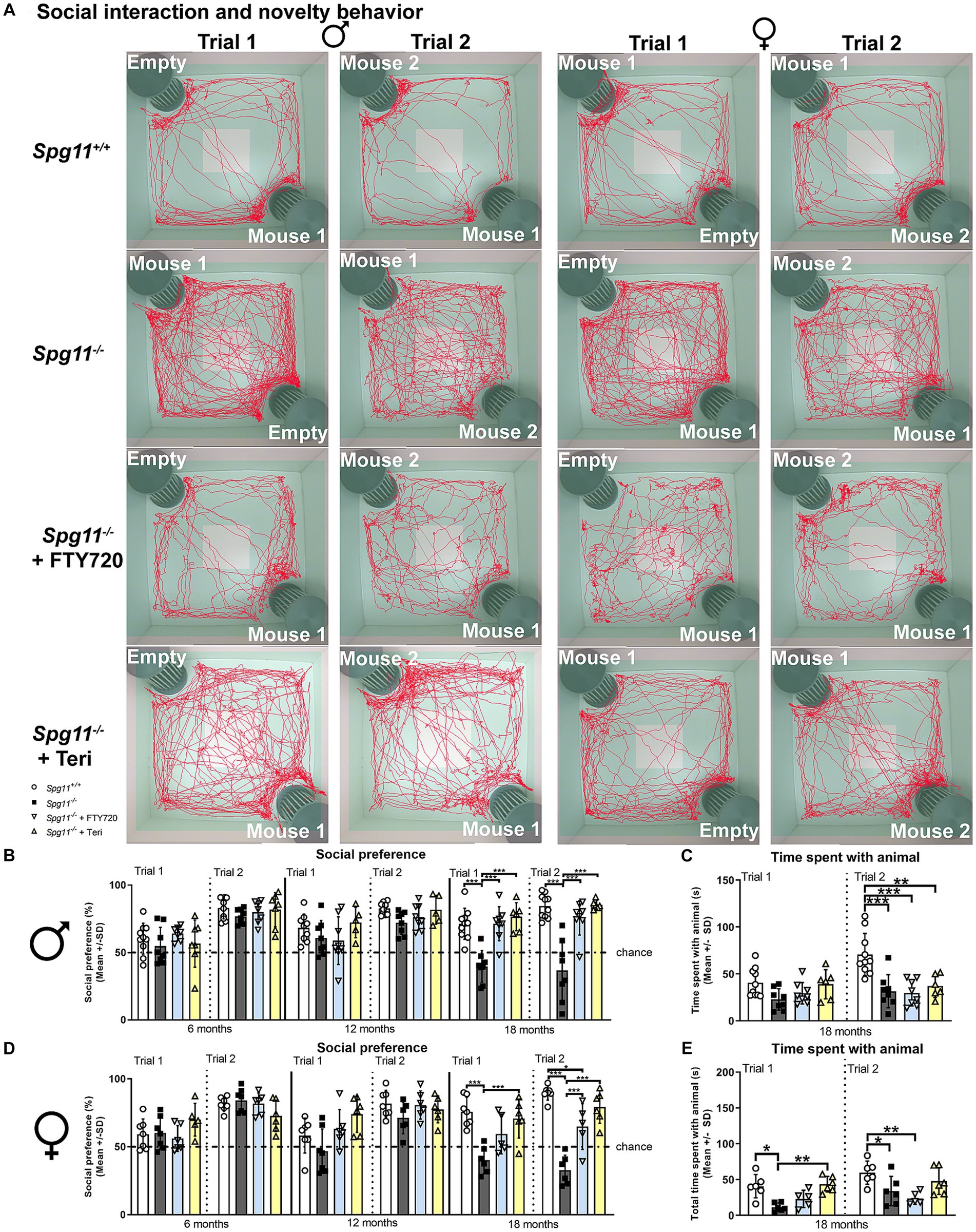
Figure 6. Treatment with fingolimod or teriflunomide ameliorates social abnormalities of Spg11−/− mice. (A) Representative images of social interest (trial 1; 1st and 3rd column) and novelty (trial 2; 2nd and 4th column) analysis of 18-month-old male (1st and 2nd column) and female (3rd and 4th column) Spg11+/+ (top row), Spg11−/− mice (2nd row), and Spg11−/− mice treated with fingolimod (FTY720) (3rd row) or teriflunomide (bottom row). Light square indicates the center zone of the arena, green area indicates the border zone. Red line represents the walking track. Mouse 1 indicates the cage in which the first unfamiliar mouse is placed, mouse 2 in which the second unfamiliar mouse is placed. Empty indicates the empty cage. (B) Social preference of male Spg11+/+, Spg11−/− and treated Spg11−/− mice at 6, 12, and 18 months. Treatment with either immunomodulator restores social interest and novelty preference of 18-month-old male Spg11−/− mice [social preference: F(11, 82) = 4.826, p < 0.0001; time spent with animal: F(7, 56) = 8.078, p < 0.0001]. (C) Treatment does not increase the time male Spg11−/− mice spend with the unfamiliar animals in the social novelty trial (trial 2). (D) Social preference of female Spg11+/+, Spg11−/− or treated Spg11−/− mice at 6, 12, and 18 months. Treatment with either immunomodulator increases the social interest and novelty preference of 18-month-old female Spg11−/− mice, while the effect of teriflunomide treatment is more pronounced [F(23, 126) = 9.881, p < 0.0001]. (E) Treatment with teriflunomide, but not fingolimod, increases the time female Spg11−/− mice spend with the unfamiliar animal in the social interest trial (trial 1) and shows the same tendency in the social novelty trial (trial 2) at 18 months [F(7, 38) = 6.643, p < 0.0001]. Chance indicates equal time spent with the empty cage/animals (50%). Error bars represent the standard deviations (circles, squares, triangles = mean value of one mouse). Significance of treated knockout mice compared to Spg11+/+ and untreated Spg11−/− mice is determined by one-way ANOVA and Sidak’s post hoc test (*p < 0.05, **p < 0.01, ***p < 0.001). Corresponding data from Spg11+/+ and Spg11−/− mice, as shown in Figure 2, are presented here again.
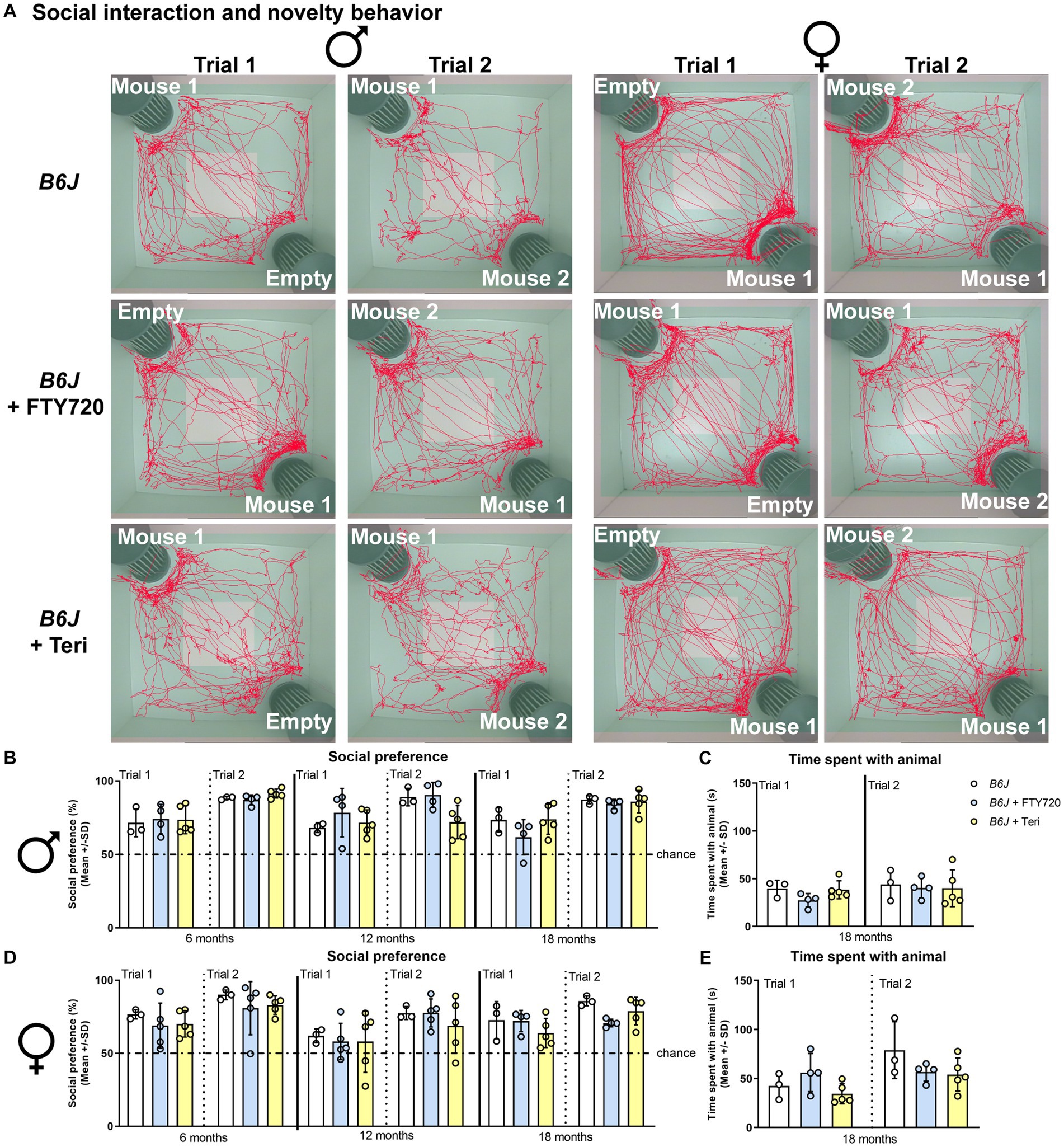
Figure 7. Treatment with fingolimod or teriflunomide does not alter social behavior of B6J mice. (A) Representative images of social interest (trial 1; 1st and 3rd column) and novelty (trial 2; 2nd and 4th column) analysis of 18-month-old male (1st and 2nd column) and female (3rd and 4th column) B6J (top row) and B6J mice treated with fingolimod (FTY720) (2nd row) or teriflunomide (bottom row). Light square indicates the center zone of the arena, green area indicates the border zone. Red line represents the walking track. Mouse 1 indicates in which cage the first unfamiliar mouse was placed, mouse 2 in which the second unfamiliar mouse was placed. Empty indicates the empty cage. (B) Social preference of B6J or treated B6J mice at 6-, 12-, and 18- months. Treatment does not alter social interest and novelty preference or (C) the time spend with the unfamiliar animals of male B6J mice [social preference: F(17, 54) = 4.146, p < 0.0001; time spent with animal: F(5, 18) = 0.7371, p = 0.6054]. (D) Social preference of female B6J or treated B6J mice at 6-, 12-, and 18- months. Treatment does not alter social interest and novelty preference, or (E) the time spend with the unfamiliar animals of female B6J mice [social preference: F(17, 58) = 2.382, p = 0.0074; time spent with animal: F(5, 18) = 3.001, p = 0.0384]. Chance indicates equal time spent with the empty cage/animals (50%). Error bars represent standard deviations (circles = value of one mouse). Significance of treated B6J mice compared to B6J mice is determined by one-way ANOVA and Sidak’s post hoc test.
Using the OF analysis, we found that treatment with fingolimod, but not teriflunomide, reduced the walking distance and showed the same tendency for the movement duration, typically increased in male Spg11−/− mice, at 6 months of age (Figures 8A–C). No effect of treatment with either immunomodulator on these parameters was detectable at later ages (Figures 8A–C). Treatment of female Spg11−/− mice with fingolimod, but not teriflunomide, led to a trend towards a reduced walking distance and movement duration at 18 months (Figures 8A,D,E). While treatment with fingolimod did not change the exploratory behavior of male B6J mice, female B6J mice showed an increased walking distance and a tendency towards increased movement duration compared to untreated mice at 18 months (Figures 9A–E). Treatment with teriflunomide had no effect on the exploratory behavior of male or female B6J mice (Figures 9A–E).
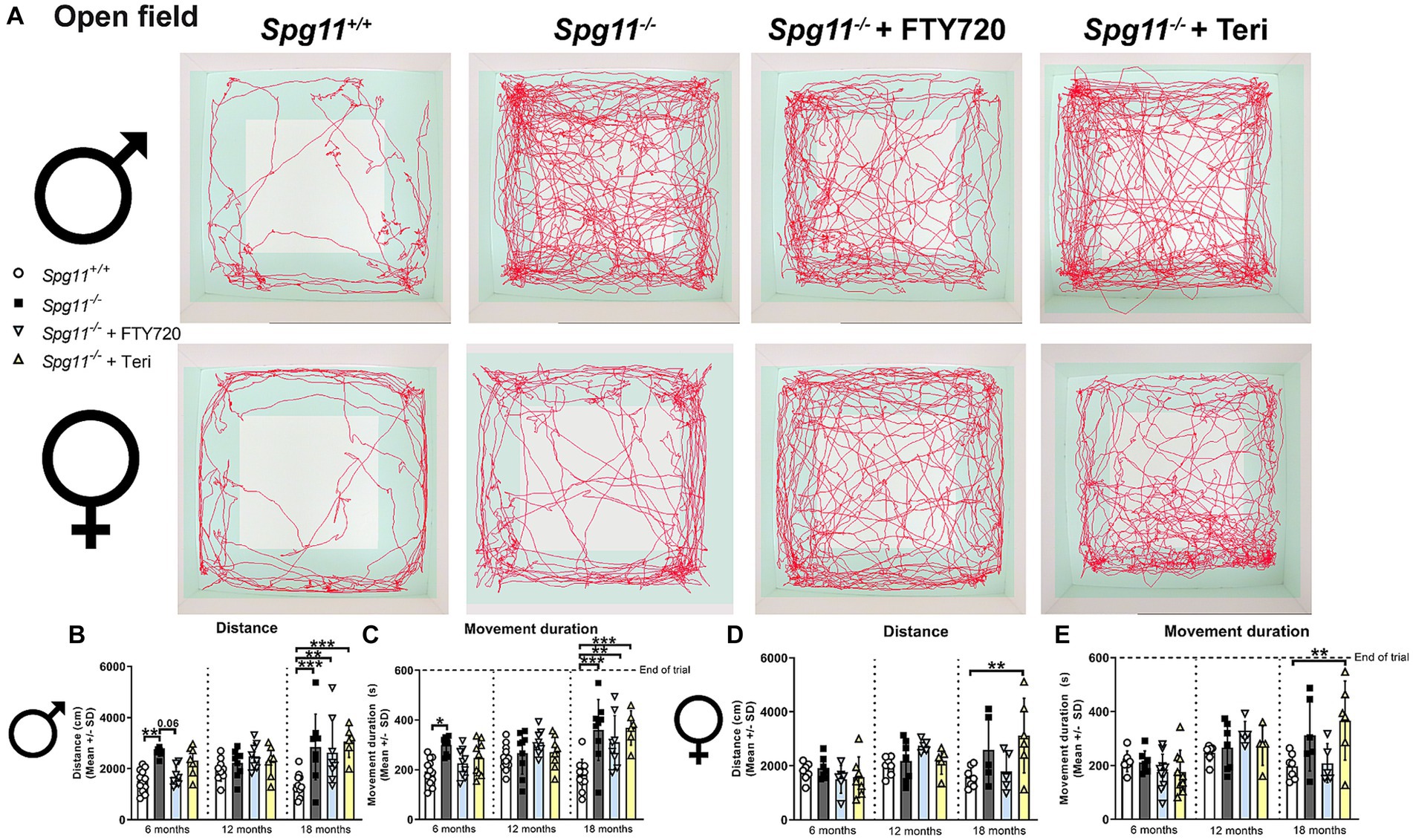
Figure 8. Treatment with fingolimod or teriflunomide does not reduce hyperactivity-like behavior of Spg11−/− mice. (A) Representative images of OF analysis of 18-month-old male (top row) and female (bottom row) Spg11+/+, Spg11−/−, and Spg11−/− mice treated with fingolimod (FTY720) or teriflunomide. Light square indicates the center zone of the arena, green area indicates the border zone. Red line represents the walking track. (B) While treatment with fingolimod reduces the walking distance and (C) movement duration of male Spg11−/− mice at 6 months neither treatment reduces them at 18 months [distance: F(11, 90) = 5.215, p < 0.0001; movement duration: F(11, 98) = 6.190, p < 0.0001]. (D) Treatment with fingolimod, but not teriflunomide, leads to a trend towards a reduced walking distance and (E) movement duration of 18-month-old female Spg11−/− mice [distance: F(11, 62) = 3.047, p = 0.0026; movement duration: F(11, 69) = 4.110, p = 0.0001]. Error bars represent standard deviations (circles, squares, triangles = value of one mouse). Significance of treated Spg11−/− mice compared to Spg11+/+ and Spg11−/− mice is determined by one-way ANOVA and Sidak’s post hoc test (**p < 0.01, ***p < 0.001). Corresponding data from Spg11+/+ and Spg11−/− mice, as shown in Figure 3, are presented here again.
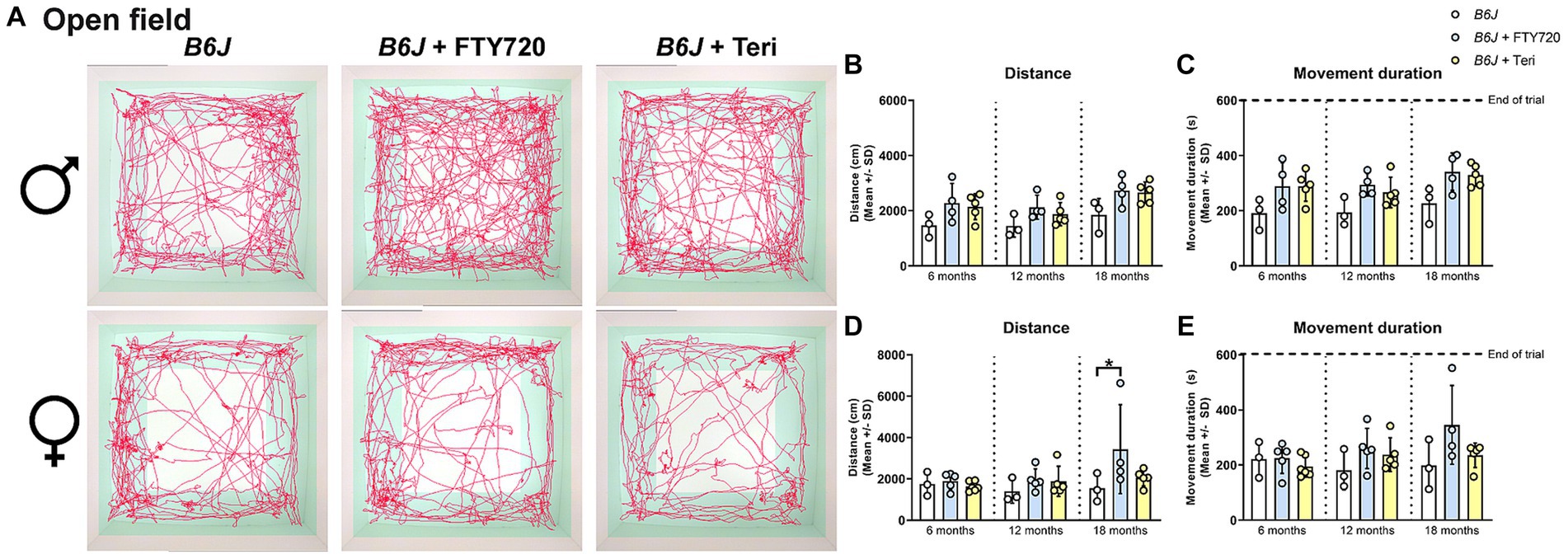
Figure 9. Treatment with fingolimod or teriflunomide increases aspects of hyperactivity-like behavior of B6J mice. (A) Representative images of OF analysis of 18-month-old male (top row) and female (bottom row) B6J and B6J mice treated with fingolimod (FTY720) or teriflunomide. Light square indicates the center zone of the arena, green area indicates the border zone. Red line represents the walking track. (B) Treatment with either immunomodulator leads to a tendency towards an increased walking distance and (C) movement duration of 18-month-old male B6J mice [distance: F(8, 27) = 3.246, p = 0.0102; movement duration: F(8, 27) = 3.073, p = 0.0135]. (D) Treatment with fingolimod, but not teriflunomide, increases the walking distance and (E) movement duration of 18-month-old female B6J mice [distance: F(8, 30) = 1.975, p = 0.0848; movement duration: F(8, 30) = 1.781, p = 0.1204]. Error bars represent standard deviations (circles = value of one mouse). Significance of treated B6J mice compared to B6J mice is determined by one-way ANOVA and Sidak’s post hoc test (*p < 0.05).
Regarding the anxiety-like behavior, neither treatment changed the behavior of male Spg11−/− mice compared to wt mice in the DLB analysis (Figures 10A–C). In female knockout mice, which typically show reduced anxiety-like behavior under non-treatment conditions, treatment with fingolimod led to an increased time to enter and less time spent in the light compartment compared to untreated Spg11−/− mice (Figures 10A,D,E). Of note, treatment of Spg11−/− mice with fingolimod also increased these parameters compared to wt mice (Figures 10A,D,E). Treatment with teriflunomide showed the same tendencies, albeit not to the same degree as fingolimod (Figures 10A,D,E). Treatment of male or female B6J mice with fingolimod or teriflunomide did not alter the latency to enter or time spent in light compartment (Figures 11A–E).
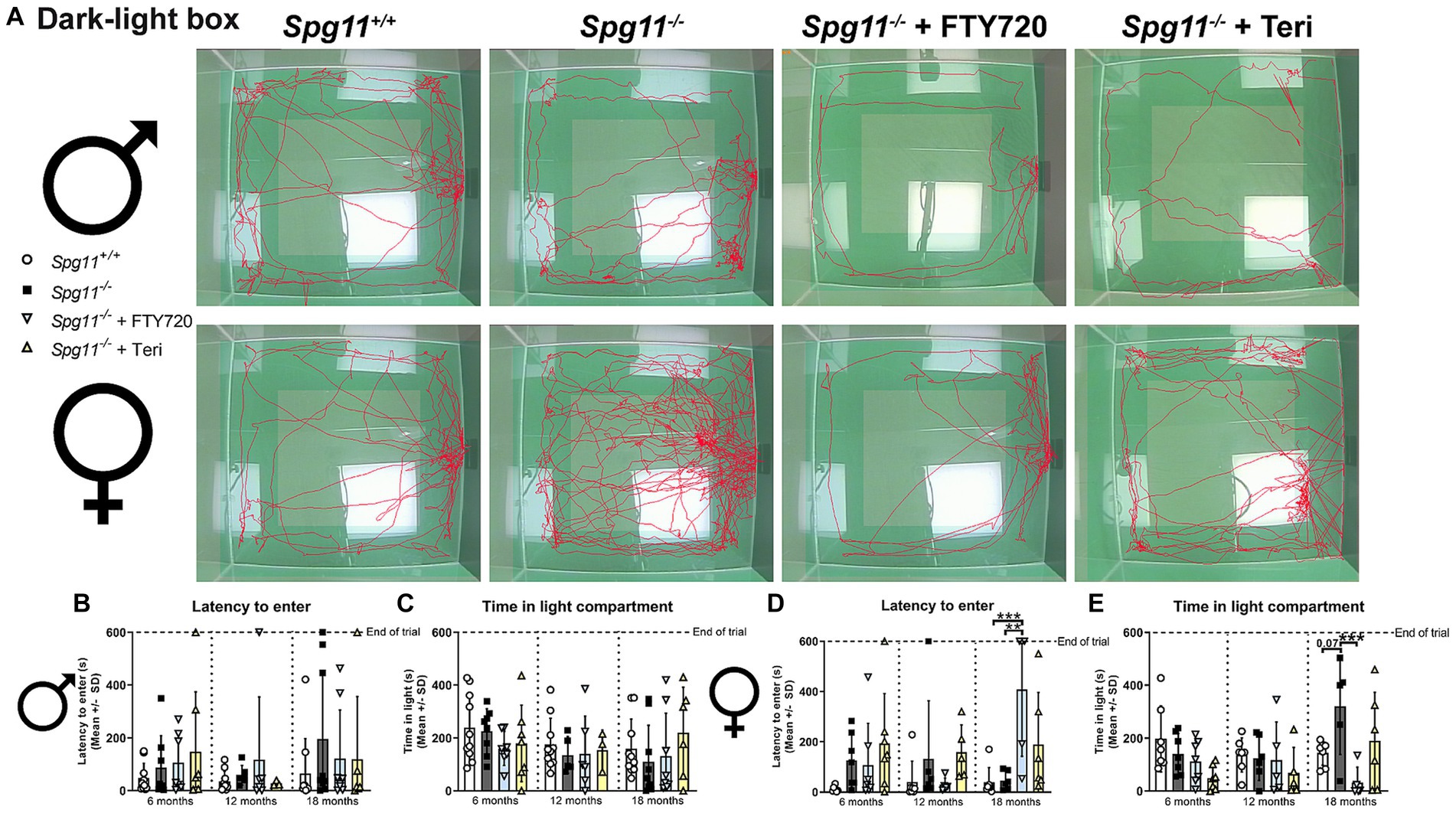
Figure 10. Treatment with fingolimod or teriflunomide restores anxiety-like behavior, typically reduced in female Spg11−/− mice. (A) Representative images of DLB analysis of 18-month-old male (top row) and female (bottom row) Spg11+/+, Spg11−/−, and Spg11−/− mice treated with fingolimod (FTY720) or teriflunomide. Light square indicates the center zone of the arena. Red line represents the walking track. (B) Treatment does not alter latency to enter, or (C) time spend in the light compartment of male Spg11−/− mice [latency to enter: F(11, 78) = 0.7479, p = 0.6896; time spend in light compartment: F(8, 30) = 1.781, p = 0.1204]. (D) Treatment with fingolimod, but not teriflunomide, increases the latency to enter of female Spg11−/− mice compared to wt and untreated knockout mice at 18 months [F(11, 62) = 2.880, p = 0.0041]. (E) Treatment with fingolimod reduces the time spend in the light compartment of 18-month-old female Spg11−/− mice, while teriflunomide shows the same tendency. Error bars represent standard deviations (circles, squares, triangles = value of one mouse). Significance of treated Spg11−/− mice compared to Spg11+/+ and Spg11−/− mice is determined by one-way ANOVA and Sidak’s post hoc test (**p < 0.01, ***p < 0.001). Corresponding data from Spg11+/+ and Spg11−/− mice, as shown in Figure 4, are presented here again.
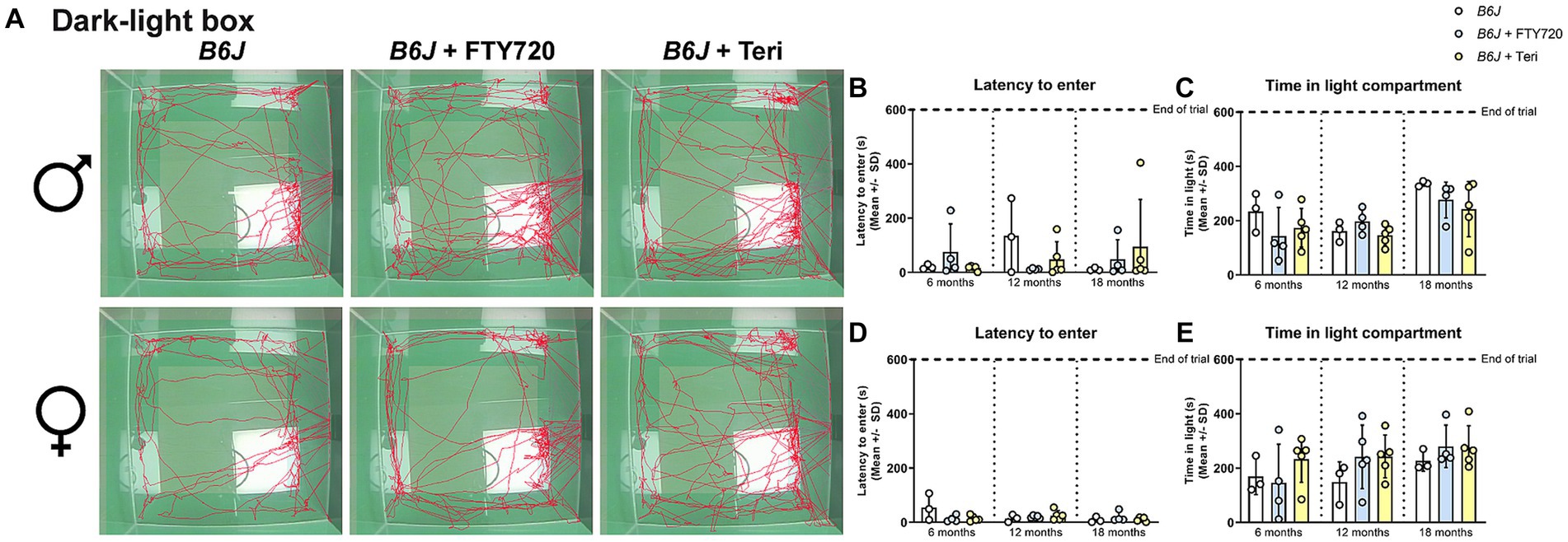
Figure 11. Treatment with fingolimod or teriflunomide does not alter anxiety-like behavior of B6J mice. (A) Representative images of DLB analysis of 18-month-old male (top row) and female (bottom row) B6J mice. (B,C) Treatment does not alter the latency to enter, or (D,E) time spend in the light compartment of male or female B6J mice [male: latency to enter: F(8, 27) = 0.8045, p = 0.6043; time spend in light compartment: F(8, 27) = 3.204, p = 0.0109; female: latency to enter: F(8, 28) = 1.926, p = 0.0955; time spend in light compartment: F(8, 28) = 1.193, p = 0.3386]. Error bars represent standard deviations (circles = value of one mouse). Significance of treated B6J mice compared to untreated B6J mice is determined by one-way ANOVA and Sidak’s post hoc test.
Similar to our findings in Rag1-deficient mice, treatment with fingolimod or teriflunomide reduced the time male Spg11−/− mice spent leaning over the edge of the platform, while the latency to jump remained unchanged in the CAR analysis (Figures 12A–C). In female Spg11−/− mice, treatment with fingolimod, but not teriflunomide, led to a tendency towards a reduced time spent leaning over the edge of the platform, while the latency to jump remained unaffected by both treatments (Figures 12A,D,E). Conversely, treatment of male B6J mice with fingolimod increased the time spent leaning over the edge of the platform at 18 months (Figures 13A,B). Surprisingly, we detected a reduced latency to jump off the platform in untreated male B6J mice, that was not altered by treatment with either immunomodulator (Figure 13C). In female B6J mice, the time spent leaning over the edge of the platform remained unaffected, but treatment with teriflunomide led to a reduced latency to jump off the platform when compared to untreated mice at 12 months (Figures 13A,D,E). Treatment with fingolimod did not lead to behavioral changes of female B6J mice in the CAR analysis (Figures 13A,D,E).
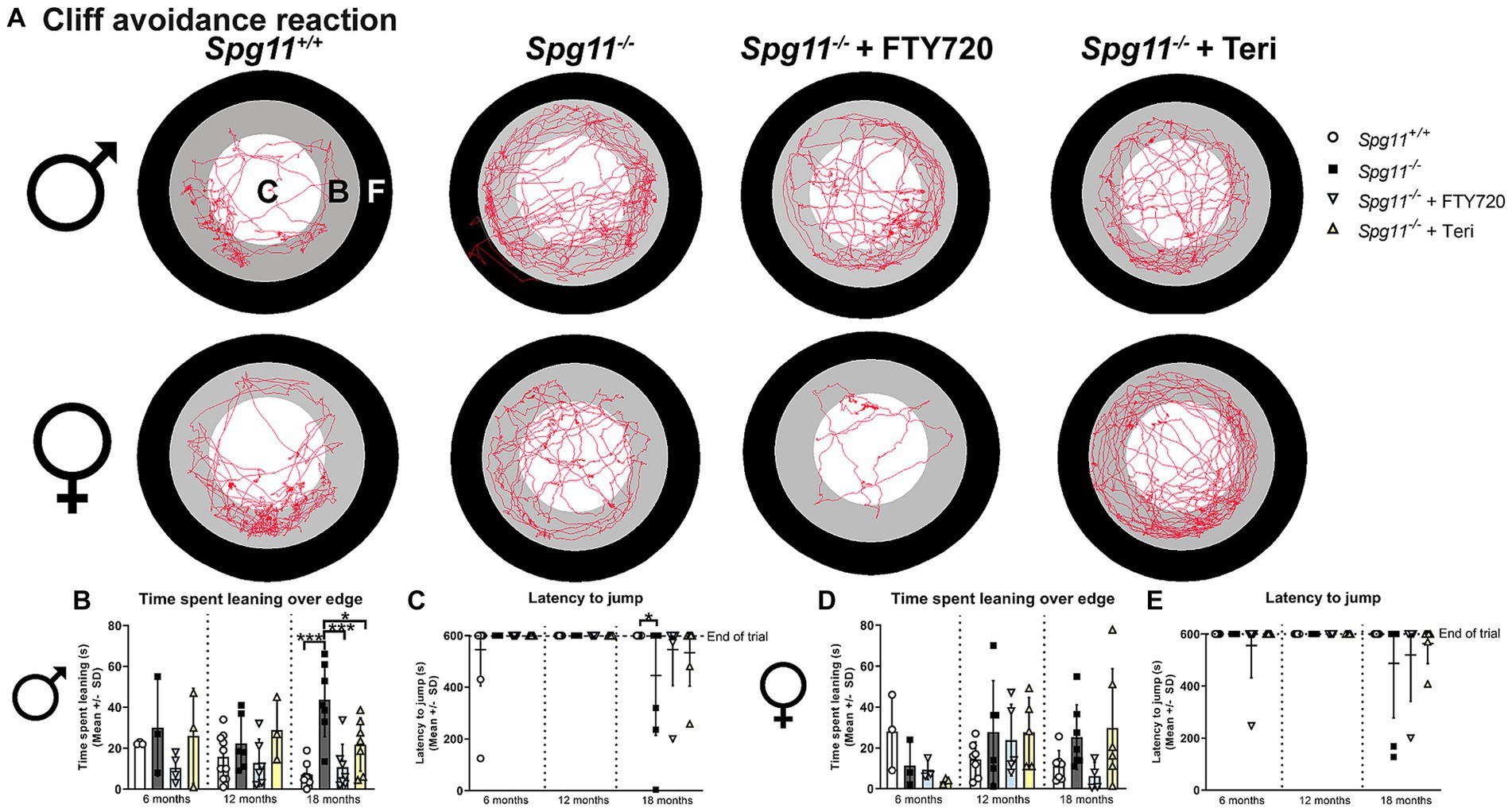
Figure 12. Treatment with fingolimod or teriflunomide reduces aspects of impulsivity-like behavior of Spg11−/− mice. (A) Representative images of CAR analysis of 18-month-old male (top row) and female (bottom row) Spg11+/+, Spg11−/− and Spg11−/− mice treated with fingolimod (FTY720) or teriflunomide. Red line represents the walking track (C = center zone, white; B = border zone, grey; F = floor zone, black). (B) Treatment reduces the time spend leaning over the edge of the platform, (C) but does not ameliorate the latency to jump off the platform of 18-month-old male Spg11−/− mice [time spend leaning: F(11, 55) = 3.931, p = 0.0003; latency to jump: F(11, 80) = 1.717, p = 0.0845] (D). Treatment of female Spg11−/− mice with fingolimod, but not teriflunomide, shows a tendency towards a reduced time spend leaning over the edge of the platform at 18 months [F(11, 47) = 1.488, p = 0.1678]. (E) Treatment does not ameliorate the latency to jump off the platform of 18-month-old female Spg11−/− mice [F(11, 71) = 1.298, p = 0.2434]. Error bars represent standard deviations (circles, squares, triangles = value of one mouse). Significance of treated knockout mice compared to Spg11+/+ and Spg11−/− mice is determined by one-way ANOVA and Sidak’s post hoc test (*p < 0.05, ***p < 0.001). Corresponding data from Spg11+/+ and Spg11−/− mice, as shown in Figure 5, are presented here again.
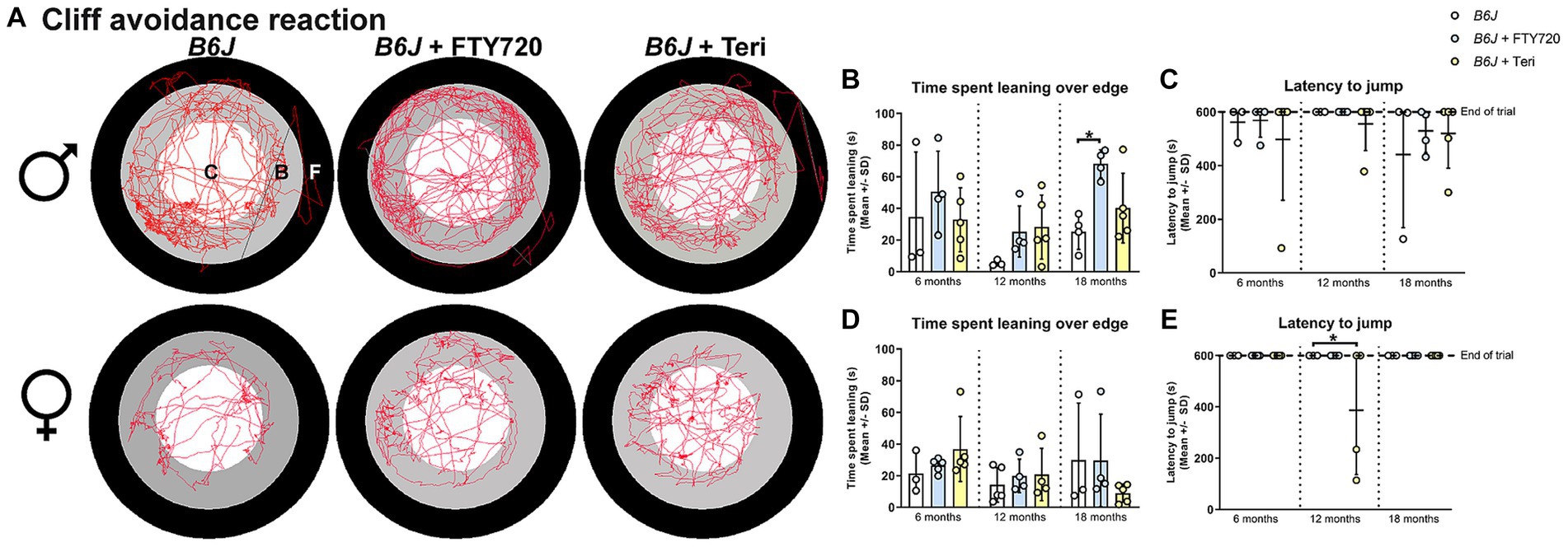
Figure 13. Treatment with fingolimod or teriflunomide increases aspects of impulsivity-like behavior of B6J mice. (A) Representative images of CAR analysis of 18-month-old male (top row) and female (bottom row) B6J and B6J mice treated with fingolimod (FTY720) or teriflunomide. Red line represents the walking track (C = center zone, white; B = border zone, grey; F = floor zone, black). (B) Treatment with fingolimod increases the time male B6J spend leaning over the edge of the platform compared to untreated mice at 18 months [F(8, 28) = 2.682, p = 0.0252]. (C) Untreated male B6J mice show jumping behavior and treatment does not alter this [F(8, 27) = 0.4806, p = 0.8591]. (D) Treatment does not alter the time female B6J mice spend leaning over the edge of the platform [F(8, 29) = 1.117, p = 0.3808]. (E) Treatment with teriflunomide, but not fingolimod, reduces the latency to jump off the platform of 12-month-old female B6J mice compared to untreated mice [F(8, 27) = 2.886, p = 0.0185]. Error bars represent standard deviations (circles = value of one mouse). Significance of treated B6J mice compared to untreated B6J mice is determined by one-way ANOVA and Sidak’s post hoc test (*p < 0.05).
In summary, we here show that pharmacological immunomodulation improves distinct behavioral abnormalities, especially related to social behavior, of Spg11−/− mice.
Degeneration and preservation of Purkinje cells correlate with development and mitigation, respectively, of behavioral abnormalities of Spg11−/− mice
Correct function of cerebellar Purkinje cells (PC) is implicated in social behavior of humans and mice (Bauman and Kemper, 2005; Amaral et al., 2008; Tsai et al., 2012; Reith et al., 2013; Cutando et al., 2022). It has been previously demonstrated that Spg11−/− mice show loss of PC (Branchu et al., 2017), and we detected high levels of inflammation in the cerebellum (Hörner et al., 2022). Therefore, we wanted to investigate whether genetic inactivation of the adaptive immune system affects PC loss, possibly explaining the ameliorated social behavior of Spg11−/−Rag1−/− mice. Agreeing with the previously reported findings, we saw a pronounced loss of PC in Spg11−/− mice compared to wt littermates at 18 months (Figures 14A–C), and indeed, Rag1-deficieny prevented this loss (Figures 14B,C). Importantly, treatment with fingolimod or teriflunomide also significantly attenuated the loss of PC in the cerebellum of Spg11−/− mice (Figures 14B,D).
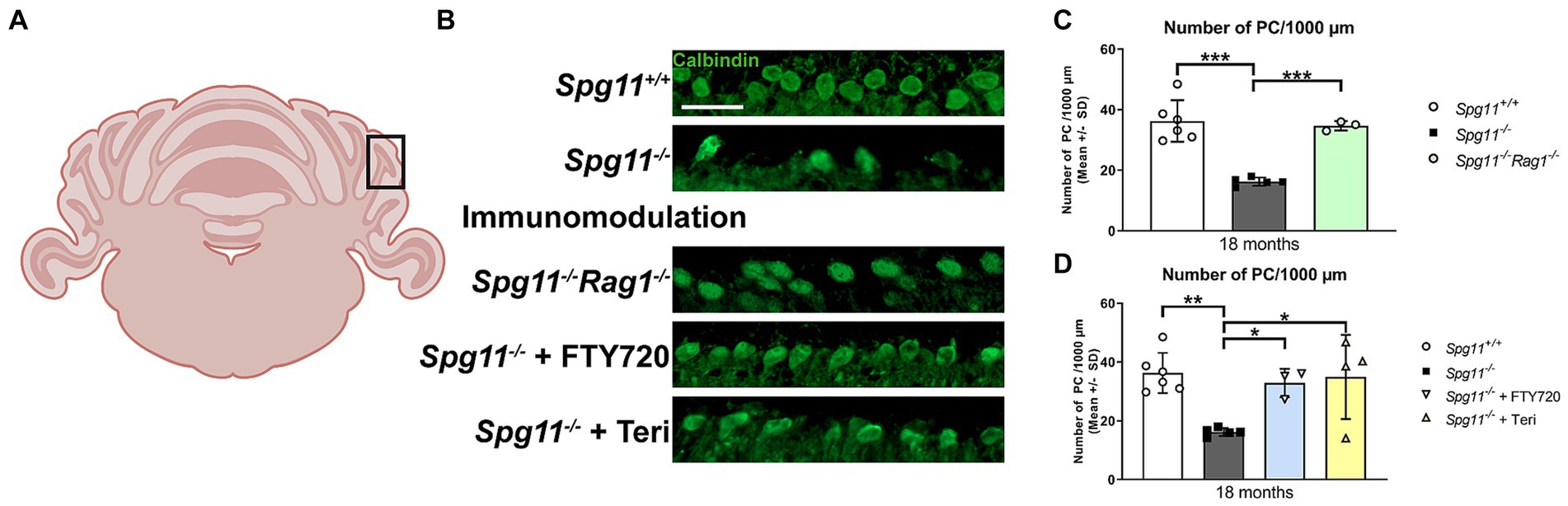
Figure 14. Genetic or pharmacological immunomodulation preserves Purkinje cell numbers of Spg11−/− mice. (A) Schematic representation of the mouse cerebellum. Black box indicates the area in which Purkinje cells (PC) were counted. Created with BioRender. (B) Representative images of Calbindin+ PC in the cerebellum of 18-month-old Spg11+/+ (top row), Spg11−/−(2nd row), Spg11−/−Rag1−/−(3rd row) and Spg11−/− mice treated with fingolimod (FTY720) (4th row) or teriflunomide (bottom row). Scale bar: 50 μm. (C) Spg11−/− mice show loss of PC compared to wt littermates at 18 months and Rag1-deficiency [F(2, 11) = 27.52, p < 0.0001] or (D) treatment with fingolimod (FTY720) or teriflunomide preserves PC numbers [F(3, 14) = 6.759, p = 0.0048]. Error bars represent standard deviations (circles, squares, triangles = mean value of one mouse). Significance of Spg11−/−Rag1−/− and treated Spg11−/− mice compared to Spg11+/+ and Spg11−/− mice are determined by one-way ANOVA and Sidak’s post hoc test (*p < 0.05, **p < 0.01, ***p < 0.001). (Spg11+/+ mice = 6; Spg11−/− mice = 5; Spg11−/−Rag1−/− mice = 3; Spg11−/− mice+ FTY720 = 3; Spg11−/− mice + Teri = 4). Corresponding data from Spg11+/+ and Spg11−/− mice are presented in (C,D).
In summary, we here show that genetic or pharmacological immunomodulation preserves PC numbers in the cerebellum of Spg11−/− mice.
Discussion
We previously showed that secondary inflammation by the adaptive immune system contributes to disease progression in a mouse model of SPG11 (Hörner et al., 2022). This comprised histopathological changes and distinct gait abnormalities related to coordination, partially reflecting abnormal gait parameters seen in patients (Hörner et al., 2022). Here, we further extend our analysis by shifting the research focus to the impact of secondary inflammation on behavioral abnormalities related to neuropsychological features typically detected in SPG11 patients.
Spg11−/− mice display distinct behavioral alterations, partially reflecting neuropsychological features of patients
In humans, neuropsychological abnormalities are accompanying symptoms in many CNS diseases like AD, PD, MS, multiple system atrophy and HSPs (Becker et al., 1988; St Clair et al., 1988; Brassington and Marsh, 1998; Osmolak et al., 2012; Barcelos et al., 2018; Faber et al., 2018). However, behavioral involvement remains an insufficiently researched area and the underlying pathomechanisms remain mainly elusive. Here, we established a behavioral test battery that revealed abnormalities in social, hyperactivity-like, anxiety-like, and impulsivity-like behavior of Spg11−/− mice, all being relevant for SPG11 patients (Wijemanne et al., 2015; Faber et al., 2018; Utz et al., 2022; Klebe et al., unpublished data). Generally, we noted that most behavioral abnormalities were detected at higher ages (e.g., from month 12 or 18 onwards), faithfully reflecting the relatively later onset of many behavioral features in patients and confirming the suitability of the test battery selected.
Learning and memory difficulties are a prominent feature of SPG11 patients (Faber et al., 2018; Utz et al., 2022) and previously published work provided evidence that Spg11−/− mice show cognitive deficits (Branchu et al., 2017). We could not detect defects in memory and recognition of Spg11−/− mice. However, the Y-maze test used by Branchu et al. relied on spatial memory, while the here used NOR investigates recognition without a spatial memory component. It is therefore possible, that the two forms of memory acquisition are affected differently in this mouse model. Furthermore, Spg11−/− mice showed signs of hyperactivity-like behavior and previous studies could provide evidence that SPG11 patients show signs of attention deficit/hyperactivity syndrome (ADHD) (Wijemanne et al., 2015). Interestingly, female Spg11−/− mice showed reduced anxiety-like behavior, that was not detectable in male knockout mice, while male Spg11−/− mice showed more pronounced impulsivity-like behavior. While the exact mechanisms responsible for the sex differences in behavioral changes of Spg11−/− mice remain to be identified, previous studies offered multiple putative explanations for sex differences in neuropsychological disease manifestations, including CNS-associated immune reactions (Osborne et al., 2018; Bangasser and Cuarenta, 2021). Regarding the here identified altered features of social behavior, it is tempting to speculate that impaired olfactory function in the mutants might be a possible reason, as impaired olfaction is an established symptom of other neurodegenerative disorders, like AD (Murphy, 2019). Further studies in patients and the corresponding mouse model are required to address this question.
In summary, our SPG11 mouse model reflects many aspects of mostly later-onset neuropsychological features detected in SPG11 patients.
Immunomodulation improves distinct behavioral abnormalities of Spg11−/− mice
We found that Rag1-deficiency and treatment with the immunomodulators fingolimod (FTY720) or teriflunomide robustly attenuated the abnormal social behavior of male and female Spg11−/− mice. A study by McGowan et al. (2011) indicated that genuine Rag1-deficient mice show an impaired social recognition memory. Importantly, these experiments focused largely on social memory, and social behavior of Rag1-deficient mice was comparable to those of wt mice after a retention phase of 30 min (McGowan et al., 2011), indicating that Rag1-deficiency does not generally alter social behavior. Of note, especially treatment with fingolimod has been shown to be beneficial in other disease models regarding social abnormalities, e.g., it attenuated social deficits in a rat model of autism spectrum disorder (Wu et al., 2017), and in mouse models of experimental autoimmune encephalomyelitis (EAE) (Baert et al., 2019), and systemic lupus erythematosus (de Bruin et al., 2016; Shi et al., 2018).
Regarding anxiety-related parameters, genetic and immunomodulatory approaches counteracted the reduced anxiety-like behavior, typically detected in female Spg11−/− mice. Some studies indicate that Rag1-deficiency leads to increased anxiety- and depression-like behavior in mice (Rattazzi et al., 2013; Smith et al., 2014), and indeed Rag1-deficiency increased anxiety-like behavior of wt mice in our study. Conversely, fingolimod treatment has been shown to reduce anxiety-like behavior in mouse EAE (Bonfiglio et al., 2017), while other studies provided evidence that treatment with fingolimod did not affect anxiety-like behavior in other mouse or rat models (di Nuzzo et al., 2015; Leo et al., 2017). In line with these findings, we did not detect an effect of treatment with immunomodulators on anxiety-like behavior of B6J mice, although we acknowledge the low number of mice as a possible limitation of this result. It has to be noted that there is evidence of a mechanistic link between anxiety-like behavior and microglial activation, especially in the amygdala and the hippocampus (Sawada et al., 2014; Stein et al., 2017; Wang et al., 2018). However, we did not analyze microglia in these compartments. It should be subject of future experiments to determine the connection of Rag1-deficiency/pharmacological immune modulation, microglial activation, and anxiety-like behavior.
Regarding impulsivity-like behavior, Rag1-deficiency and pharmacological treatment could reduce the time Spg11−/− mice spent leaning over the edge of the platform. Indeed, it has been shown that inflammatory markers are elevated in humans and mice that display traits linked to impulsivity and aggression (Beurel and Jope, 2014; Logsdon et al., 2016; Kim et al., 2020), and reducing neuroinflammation in a rat model of traumatic brain injury led to a significant reduction of impulsivity-like behavior in the elevated platform maze test (Logsdon et al., 2016).
The role of T-lymphocytes as pathogenic amplifiers in genetically mediated diseases has been well-documented (Groh et al., 2013, 2016, 2017, 2018, 2023; Groh and Martini, 2017; Hörner et al., 2022; Abdelwahab et al., 2023). Additionally, research has implicated microglia and their activation states in disease progression, particularly in neurodegenerative and neurodevelopmental disorders (Lukens and Eyo, 2022; Matuleviciute et al., 2023). Notably, prior investigations of the Spg11−/− mouse model revealed elevated numbers and activation of microglia, suggesting a potential contribution to behavioral abnormalities (Hörner et al., 2022). While the present study focused on the impact of targetable adaptive immune cells, their interaction with microglia should be explored in future experiments.
Taken together, the here presented data provide evidence that behavioral abnormalities in this mouse model, especially related to social behavior, can indeed be mediated, or aggravated by secondary inflammation.
Preservation of Purkinje cells by immune modulation correlates with ameliorated social behavior of Spg11−/− mice
Structural abnormalities or loss of PC in mice or humans are often associated with altered social behavior (Bauman and Kemper, 2005; Amaral et al., 2008; Tsai et al., 2012; Reith et al., 2013; Sudarov, 2013; Cutando et al., 2022). The here presented mouse model shows axonal damage in the cerebellum (Hörner et al., 2022) and loss of PC (Branchu et al., 2017). According to our previous (Hörner et al., 2022) and present study, these degenerative changes are mediated by disease-amplifying inflammation most likely related to infiltrating CD8+ T-lymphocytes. Thus, mitigation of behavioral changes by immune modulation may be related to dampening of immune-driven damage and loss of PC.
What is the link between SPG11-deficiency and immune-mediated loss of PC causing behavioral changes? It has been previously described that mouse mutants for myelin genes also develop secondary, disease-amplifying inflammation (Ip et al., 2006, 2012; Kroner et al., 2010; Groh et al., 2016; Janova et al., 2018). Similar observations have been made in aging white matter (Groh et al., 2021b) and perturbed myelin has been identified as a risk for axonal degeneration, neuron loss and behavioral decline, implicating inflammation (Schaffner et al., 2023; Groh et al., 2023). Based on the finding that spatacsin is also robustly expressed by oligodendrocytes (Sjostedt et al., 2020), it is plausible to assume that mutant SPG11 or SPG11-deficiency might lead to molecularly altered myelin sheaths in the CNS. Indeed, we previously observed myelin abnormalities in the SPG11 mouse model, in the form of redundant and fragmented myelin profiles, possibly indicative of an altered molecular composition (Hörner et al., 2022). Molecularly altered myelin may be targeted by CD8+ T-lymphocytes, leading to axonal damage and subsequent neuronal loss in case of the PC. Alternatively, or additionally, it is possible that neurons are the primary target of inflammation, as spatacsin is strongly expressed by these cells (Murmu et al., 2011; Perez-Branguli et al., 2014; Pozner et al., 2020). This view is in line with our observation that lymphocytes invade not only white, but also grey matter compartments of SPG11 mutants (Hörner et al., 2022).
Taken together, our findings support the notion that inflammation-mediated cerebellar dysfunction plays a prominent role in SPG11, not only regarding gait abnormalities (Hörner et al., 2022), but also in the development of altered social behavior. Additionally, this study further strengthens the hypothesis that inflammation by CD8+ T-lymphocytes, leading to axonal perturbation and consequent loss of neuronal cell bodies, has an overarching role in the disease progression of many CNS related diseases (Groh et al., 2017; Hörner et al., 2022; Abdelwahab et al., 2023), disorders presenting with neuropsychological features, and aging (Groh et al., 2021b). Nevertheless, future studies should carefully investigate the long-term effects (both putative beneficial and adverse effects) of treatment with immunomodulators in SPG11.
Conclusion
We previously demonstrated that neuroinflammation is a targetable amplifier of neuropathological changes and gait performance in a mouse model for SPG11. As neuropsychological features are also strongly limiting quality of life for both SPG11 patients and their relatives, we here focused on these clinically highly relevant symptoms. We found that some behavioral abnormalities of Spg11−/− mice are indeed mediated or amplified by neuroinflammation and can be mitigated by genetic targeting of lymphocytes and, more importantly, clinically approved immunomodulators. These findings may be of high translational relevance as they could pave the way for using immunomodulators as a treatment option for improving movement and neuropsychological aspects in SPG11.
Data availability statement
The original contributions presented in the study are included in the article/Supplementary material, further inquiries can be directed to the corresponding authors.
Ethics statement
The animal study was approved by Government of Lower Franconia, Germany. The study was conducted in accordance with the local legislation and institutional requirements.
Author contributions
MH: Conceptualization, Methodology, Writing – review & editing, Writing – original draft, Data curation, Formal analysis, Investigation, Project administration, Visualization. SP: Formal analysis, Investigation, Methodology, Writing – review & editing. JB: Methodology, Writing – review & editing, Resources. GS: Methodology, Resources, Writing – review & editing. FD: Methodology, Resources, Writing – review & editing. SK: Conceptualization, Methodology, Writing – review & editing. JG: Conceptualization, Funding acquisition, Methodology, Supervision, Writing – review & editing. RM: Conceptualization, Funding acquisition, Methodology, Project administration, Supervision, Writing – original draft, Writing – review & editing.
Funding
The author(s) declare financial support was received for the research, authorship, and/or publication of this article. This work was supported by the Federal Ministry of Education and Research (BMBF) [TreatHSP.net, Project 9, 01GM1905F (RM und JG)], and the Elite Network of Bavaria “Translational Neuroscience” (to RM).
Acknowledgments
The authors are grateful to Heinrich Blazyca, Silke Loserth, and Bettina Meyer for expert technical assistance, to Anja Weidner, Beverly Pfeiffer, Thomas Bimmerlein, and Jacqueline Schreiber for attentive animal care, and to PD Angelika Schmitt and Katharina Krause for discussions and advice.
Conflict of interest
JB was employed by EVerZOM. SP was employed by TSE systems.
The remaining authors declare that the research was conducted in the absence of any commercial or financial relationships that could be construed as a potential conflict of interest.
The author(s) declared that they were an editorial board member of Frontiers, at the time of submission. This had no impact on the peer review process and the final decision.
Publisher’s note
All claims expressed in this article are solely those of the authors and do not necessarily represent those of their affiliated organizations, or those of the publisher, the editors and the reviewers. Any product that may be evaluated in this article, or claim that may be made by its manufacturer, is not guaranteed or endorsed by the publisher.
Supplementary material
The Supplementary material for this article can be found online at: https://www.frontiersin.org/articles/10.3389/fnins.2024.1299554/full#supplementary-material
Abbreviations
AD, Alzheimer’s disease; ADHD, Attention deficit/hyperactivity syndrome; B6J, C57BL/6 J; CAR, Cliff avoidance reaction; CNS, Central nervous system; DLB, Dark/light box; EAE, Experimental autoimmune encephalomyelitis; FTY720, Fingolimod; HSP, Hereditary spastic paraplegia; MS, Multiple sclerosis; NOR, Novel object recognition; OF, Open field; SI, Social interaction and novelty; SPG11, Hereditary spastic paraplegia type 11; PBS, Phosphate-buffered saline; PC, Purkinje cell; PD, Parkinson’s disease; PFA, Paraformaldehyde; WT, Wild-type.
Footnotes
1. ^https://www.randomizer.org/, last accessed 2023/04/29.
References
Abdelwahab, T., Stadler, D., Knopper, K., Arampatzi, P., Saliba, A. E., Kastenmuller, W., et al. (2023). Cytotoxic CNS-associated T cells drive axon degeneration by targeting perturbed oligodendrocytes in PLP1 mutant mice. iScience 26:106698. doi: 10.1016/j.isci.2023.106698
Amaral, D. G., Schumann, C. M., and Nordahl, C. W. (2008). Neuroanatomy of autism. Trends Neurosci. 31, 137–145. doi: 10.1016/j.tins.2007.12.005
Baert, L., Benkhoucha, M., Popa, N., Ahmed, M. C., Manfroi, B., Boutonnat, J., et al. (2019). A proliferation-inducing ligand-mediated anti-inflammatory response of astrocytes in multiple sclerosis. Ann. Neurol. 85, 406–420. doi: 10.1002/ana.25415
Bangasser, D. A., and Cuarenta, A. (2021). Sex differences in anxiety and depression: circuits and mechanisms. Nat. Rev. Neurosci. 22, 674–684. doi: 10.1038/s41583-021-00513-0
Barcelos, L. B., Saad, F., Giacominelli, C., Saba, R. A., de Carvalho Aguiar, P. M., Silva, S. M. A., et al. (2018). Neuropsychological and clinical heterogeneity of cognitive impairment in patients with multiple system atrophy. Clin. Neurol. Neurosurg. 164, 121–126. doi: 10.1016/j.clineuro.2017.10.039
Bauman, M. L., and Kemper, T. L. (2005). Neuroanatomic observations of the brain in autism: a review and future directions. Int. J. Dev. Neurosci. 23, 183–187. doi: 10.1016/j.ijdevneu.2004.09.006
Becker, J. T., Huff, F. J., Nebes, R. D., Holland, A., and Boller, F. (1988). Neuropsychological function in Alzheimer's disease. Pattern of impairment and rates of progression. Arch. Neurol. 45, 263–268. doi: 10.1001/archneur.1988.00520270037018
Beurel, E., and Jope, R. S. (2014). Inflammation and lithium: clues to mechanisms contributing to suicide-linked traits. Transl. Psychiatry 4:e488. doi: 10.1038/tp.2014.129
Beurel, E., Toups, M., and Nemeroff, C. B. (2020). The bidirectional relationship of depression and inflammation: double trouble. Neuron 107, 234–256. doi: 10.1016/j.neuron.2020.06.002
Bonfiglio, T., Olivero, G., Merega, E., Di Prisco, S., Padolecchia, C., Grilli, M., et al. (2017). Prophylactic versus therapeutic Fingolimod: restoration of presynaptic defects in mice suffering from experimental autoimmune encephalomyelitis. PLoS One 12:e0170825. doi: 10.1371/journal.pone.0170825
Boutry, M., Pierga, A., Matusiak, R., Branchu, J., Houllegatte, M., Ibrahim, Y., et al. (2019). Loss of spatacsin impairs cholesterol trafficking and calcium homeostasis. Commun. Biol. 2:380. doi: 10.1038/s42003-019-0615-z
Branchu, J., Boutry, M., Sourd, L., Depp, M., Leone, C., Corriger, A., et al. (2017). Loss of spatacsin function alters lysosomal lipid clearance leading to upper and lower motor neuron degeneration. Neurobiol. Dis. 102, 21–37. doi: 10.1016/j.nbd.2017.02.007
Brassington, J. C., and Marsh, N. V. (1998). Neuropsychological aspects of multiple sclerosis. Neuropsychol. Rev. 8, 43–77. doi: 10.1023/A:1025621700003
Cutando, L., Puighermanal, E., Castell, L., Tarot, P., Belle, M., Bertaso, F., et al. (2022). Cerebellar dopamine D2 receptors regulate social behaviors. Nat. Neurosci. 25, 900–911. doi: 10.1038/s41593-022-01092-8
de Bruin, N. M., Schmitz, K., Schiffmann, S., Tafferner, N., Schmidt, M., Jordan, H., et al. (2016). Multiple rodent models and behavioral measures reveal unexpected responses to FTY720 and DMF in experimental autoimmune encephalomyelitis. Behav. Brain Res. 300, 160–174. doi: 10.1016/j.bbr.2015.12.006
Denora, P. S., Schlesinger, D., Casali, C., Kok, F., Tessa, A., Boukhris, A., et al. (2009). Screening of ARHSP-TCC patients expands the spectrum of SPG11 mutations and includes a large scale gene deletion. Hum. Mutat. 30, E500–E519. doi: 10.1002/humu.20945
di Nuzzo, L., Orlando, R., Tognoli, C., Di Pietro, P., Bertini, G., Miele, J., et al. (2015). Antidepressant activity of fingolimod in mice. Pharmacol. Res. Perspect. 3:e00135. doi: 10.1002/prp2.135
Faber, I., Martinez, A. R. M., de Rezende, T. J. R., Martins, C. R. Jr., Martins, M. P., Lourenco, C. M., et al. (2018). SPG11 mutations cause widespread white matter and basal ganglia abnormalities, but restricted cortical damage. Neuroimage Clin. 19, 848–857. doi: 10.1016/j.nicl.2018.05.031
Faul, F., Erdfelder, E., Lang, A. G., and Buchner, A. (2007). G*power 3: a flexible statistical power analysis program for the social, behavioral, and biomedical sciences. Behav. Res. Methods 39, 175–191. doi: 10.3758/BF03193146
Groh, J., Abdelwahab, T., Kattimani, Y., Hörner, M., Loserth, S., Gudi, V., et al. (2023). Microglia-mediated demyelination protects against CD8(+) T cell-driven axon degeneration in mice carrying PLP defects. Nat. Commun. 14:6911. doi: 10.1038/s41467-023-42570-2
Groh, J., Berve, K., and Martini, R. (2017). Fingolimod and Teriflunomide attenuate neurodegeneration in mouse models of neuronal ceroid Lipofuscinosis. Mol. Ther. 25, 1889–1899. doi: 10.1016/j.ymthe.2017.04.021
Groh, J., Berve, K., and Martini, R. (2021a). Immune modulation attenuates infantile neuronal ceroid lipofuscinosis in mice before and after disease onset. Brain Commun. 3:fcab047. doi: 10.1093/braincomms/fcab047
Groh, J., Friedman, H. C., Orel, N., Ip, C. W., Fischer, S., Spahn, I., et al. (2016). Pathogenic inflammation in the CNS of mice carrying human PLP1 mutations. Hum. Mol. Genet. 25, 4686–4702. doi: 10.1093/hmg/ddw296
Groh, J., Hörner, M., and Martini, R. (2018). Teriflunomide attenuates neuroinflammation-related neural damage in mice carrying human PLP1 mutations. J. Neuroinflammation 15:194. doi: 10.1186/s12974-018-1228-z
Groh, J., Knöpper, K., Arampatzi, P., Yuan, X., Lößlein, L., Saliba, A.-E., et al. (2021b). Accumulation of cytotoxic T cells in the aged CNS leads to axon degeneration and contributes to cognitive and motor decline. Nat. Aging 1, 357–367. doi: 10.1038/s43587-021-00049-z
Groh, J., Kuhl, T. G., Ip, C. W., Nelvagal, H. R., Sri, S., Duckett, S., et al. (2013). Immune cells perturb axons and impair neuronal survival in a mouse model of infantile neuronal ceroid lipofuscinosis. Brain J. Neurol. 136, 1083–1101. doi: 10.1093/brain/awt020
Groh, J., and Martini, R. (2017). Neuroinflammation as modifier of genetically caused neurological disorders of the central nervous system: understanding pathogenesis and chances for treatment. Glia 65, 1407–1422. doi: 10.1002/glia.23162
Hagemeyer, N., Goebbels, S., Papiol, S., Kastner, A., Hofer, S., Begemann, M., et al. (2012). A myelin gene causative of a catatonia-depression syndrome upon aging. EMBO Mol. Med. 4, 528–539. doi: 10.1002/emmm.201200230
Hörner, M., Groh, J., Klein, D., Ilg, W., Schols, L., Dos Santos, S., et al. (2022). CNS-associated T-lymphocytes in a mouse model of hereditary spastic paraplegia type 11 (SPG11) are therapeutic targets for established immunomodulators. Exp. Neurol. 355:114119. doi: 10.1016/j.expneurol.2022.114119
Ip, C. W., Kroner, A., Bendszus, M., Leder, C., Kobsar, I., Fischer, S., et al. (2006). Immune cells contribute to myelin degeneration and axonopathic changes in mice overexpressing proteolipid protein in oligodendrocytes. J. Neurosci. 26, 8206–8216. doi: 10.1523/JNEUROSCI.1921-06.2006
Ip, C. W., Kroner, A., Groh, J., Huber, M., Klein, D., Spahn, I., et al. (2012). Neuroinflammation by cytotoxic T-lymphocytes impairs retrograde axonal transport in an oligodendrocyte mutant mouse. PLoS One 7:e42554. doi: 10.1371/journal.pone.0042554
Janova, H., Arinrad, S., Balmuth, E., Mitjans, M., Hertel, J., Habes, M., et al. (2018). Microglia ablation alleviates myelin-associated catatonic signs in mice. J. Clin. Invest. 128, 734–745. doi: 10.1172/JCI97032
Kim, J. S., Kang, E. S., Bahk, Y. C., Jang, S., Hong, K. S., and Baek, J. H. (2020). Exploratory analysis of behavioral impulsivity, pro-inflammatory cytokines, and resting-state frontal EEG activity associated with non-suicidal self-injury in patients with mood disorder. Front. Psych. 11:124. doi: 10.3389/fpsyt.2020.00124
Kirkpatrick, B., and Miller, B. J. (2013). Inflammation and schizophrenia. Schizophr. Bull. 39, 1174–1179. doi: 10.1093/schbul/sbt141
Klebe, S., Stevanin, G., and Depienne, C. (2015). Clinical and genetic heterogeneity in hereditary spastic paraplegias: from SPG1 to SPG72 and still counting. Rev. Neurol. (Paris) 171, 505–530. doi: 10.1016/j.neurol.2015.02.017
Kroner, A., Ip, C. W., Thalhammer, J., Nave, K. A., and Martini, R. (2010). Ectopic T-cell specificity and absence of perforin and granzyme B alleviate neural damage in oligodendrocyte mutant mice. Am. J. Pathol. 176, 549–555. doi: 10.2353/ajpath.2010.090722
Leo, A., Citraro, R., Amodio, N., De Sarro, C., Gallo Cantafio, M. E., Constanti, A., et al. (2017). Fingolimod exerts only temporary Antiepileptogenic effects but longer-lasting positive effects on behavior in the WAG/Rij rat absence epilepsy model. Neurotherapeutics 14, 1134–1147. doi: 10.1007/s13311-017-0550-y
Logsdon, A. F., Lucke-Wold, B. P., Nguyen, L., Matsumoto, R. R., Turner, R. C., Rosen, C. L., et al. (2016). Salubrinal reduces oxidative stress, neuroinflammation and impulsive-like behavior in a rodent model of traumatic brain injury. Brain Res. 1643, 140–151. doi: 10.1016/j.brainres.2016.04.063
Lukens, J. R., and Eyo, U. B. (2022). Microglia and neurodevelopmental disorders. Annu. Rev. Neurosci. 45, 425–445. doi: 10.1146/annurev-neuro-110920-023056
MacDowell, K. S., Marsa, M. D., Buenache, E., Villatoro, J. M. L., Moreno, B., Leza, J. C., et al. (2020). Inflammatory and antioxidant pathway dysfunction in borderline personality disorder. Psychiatry Res. 284:112782. doi: 10.1016/j.psychres.2020.112782
Majd, M., Saunders, E. F. H., and Engeland, C. G. (2020). Inflammation and the dimensions of depression: a review. Front. Neuroendocrinol. 56:100800. doi: 10.1016/j.yfrne.2019.100800
Matuleviciute, R., Akinluyi, E. T., Muntslag, T. A. O., Dewing, J. M., Long, K. R., Vernon, A. C., et al. (2023). Microglial contribution to the pathology of neurodevelopmental disorders in humans. Acta Neuropathol. 146, 663–683. doi: 10.1007/s00401-023-02629-2
McGowan, P. O., Hope, T. A., Meck, W. H., Kelsoe, G., and Williams, C. L. (2011). Impaired social recognition memory in recombination activating gene 1-deficient mice. Brain Res. 1383, 187–195. doi: 10.1016/j.brainres.2011.02.054
Merrill, J. E., Hanak, S., Pu, S. F., Liang, J., Dang, C., Iglesias-Bregna, D., et al. (2009). Teriflunomide reduces behavioral, electrophysiological, and histopathological deficits in the dark Agouti rat model of experimental autoimmune encephalomyelitis. J. Neurol. 256, 89–103. doi: 10.1007/s00415-009-0075-3
Metzler, B., Gfeller, P., Wieczorek, G., Li, J., Nuesslein-Hildesheim, B., Katopodis, A., et al. (2008). Modulation of T cell homeostasis and alloreactivity under continuous FTY720 exposure. Int. Immunol. 20, 633–644. doi: 10.1093/intimm/dxn023
Miller, A. H., and Raison, C. L. (2016). The role of inflammation in depression: from evolutionary imperative to modern treatment target. Nat. Rev. Immunol. 16, 22–34. doi: 10.1038/nri.2015.5
Mombaerts, P., Iacomini, J., Johnson, R. S., Herrup, K., Tonegawa, S., and Papaioannou, V. E. (1992). RAG-1-deficient mice have no mature B and T lymphocytes. Cell 68, 869–877. doi: 10.1016/0092-8674(92)90030-G
Murmu, R. P., Martin, E., Rastetter, A., Esteves, T., Muriel, M. P., El Hachimi, K. H., et al. (2011). Cellular distribution and subcellular localization of spatacsin and spastizin, two proteins involved in hereditary spastic paraplegia. Mol. Cell. Neurosci. 47, 191–202. doi: 10.1016/j.mcn.2011.04.004
Murphy, C. (2019). Olfactory and other sensory impairments in Alzheimer disease. Nat. Rev. Neurol. 15, 11–24. doi: 10.1038/s41582-018-0097-5
Nair, A. B., and Jacob, S. (2016). A simple practice guide for dose conversion between animals and human. J. Basic Clin. Pharm. 7, 27–31. doi: 10.4103/0976-0105.177703
Osborne, B. F., Turano, A., and Schwarz, J. M. (2018). Sex differences in the Neuroimmune system. Curr. Opin. Behav. Sci. 23, 118–123. doi: 10.1016/j.cobeha.2018.05.007
Osmolak, A. M., Wallenberg, R. B., and Caplan, J. P. (2012). Hereditary spastic paraplegia and psychosis: connected by the corpus callosum? Psychosomatics 53, 81–84. doi: 10.1016/j.psym.2011.05.004
Pereira, A. C., Oliveira, J., Silva, S., Madeira, N., Pereira, C. M. F., and Cruz, M. T. (2021). Inflammation in bipolar disorder (BD): identification of new therapeutic targets. Pharmacol. Res. 163:105325. doi: 10.1016/j.phrs.2020.105325
Perez-Branguli, F., Mishra, H. K., Prots, I., Havlicek, S., Kohl, Z., Saul, D., et al. (2014). Dysfunction of spatacsin leads to axonal pathology in SPG11-linked hereditary spastic paraplegia. Hum. Mol. Genet. 23, 4859–4874. doi: 10.1093/hmg/ddu200
Pozner, T., Regensburger, M., Engelhorn, T., Winkler, J., and Winner, B. (2020). Janus-faced spatacsin (SPG11): involvement in neurodevelopment and multisystem neurodegeneration. Brain 143, 2369–2379. doi: 10.1093/brain/awaa099
Rattazzi, L., Piras, G., Ono, M., Deacon, R., Pariante, C. M., and D'Acquisto, F. (2013). CD4(+) but not CD8(+) T cells revert the impaired emotional behavior of immunocompromised RAG-1-deficient mice. Transl. Psychiatry 3:e280. doi: 10.1038/tp.2013.54
Reith, R. M., McKenna, J., Wu, H., Hashmi, S. S., Cho, S. H., Dash, P. K., et al. (2013). Loss of Tsc2 in Purkinje cells is associated with autistic-like behavior in a mouse model of tuberous sclerosis complex. Neurobiol. Dis. 51, 93–103. doi: 10.1016/j.nbd.2012.10.014
Saccaro, L. F., Schilliger, Z., Dayer, A., Perroud, N., and Piguet, C. (2021). Inflammation, anxiety, and stress in bipolar disorder and borderline personality disorder: a narrative review. Neurosci. Biobehav. Rev. 127, 184–192. doi: 10.1016/j.neubiorev.2021.04.017
Sawada, A., Niiyama, Y., Ataka, K., Nagaishi, K., Yamakage, M., and Fujimiya, M. (2014). Suppression of bone marrow-derived microglia in the amygdala improves anxiety-like behavior induced by chronic partial sciatic nerve ligation in mice. Pain 155, 1762–1772. doi: 10.1016/j.pain.2014.05.031
Schaffner, E., Bosch-Queralt, M., Edgar, J. M., Lehning, M., Strauss, J., Fleischer, N., et al. (2023). Myelin insulation as a risk factor for axonal degeneration in autoimmune demyelinating disease. Nat. Neurosci. 26, 1218–1228. doi: 10.1038/s41593-023-01366-9
Shi, D., Tian, T., Yao, S., Cao, K., Zhu, X., Zhang, M., et al. (2018). FTY720 attenuates behavioral deficits in a murine model of systemic lupus erythematosus. Brain Behav. Immun. 70, 293–304. doi: 10.1016/j.bbi.2018.03.009
Sjostedt, E., Zhong, W., Fagerberg, L., Karlsson, M., Mitsios, N., Adori, C., et al. (2020). An atlas of the protein-coding genes in the human, pig, and mouse brain. Science 367:eaay5947. doi: 10.1126/science.aay5947
Smith, C. J., Emge, J. R., Berzins, K., Lung, L., Khamishon, R., Shah, P., et al. (2014). Probiotics normalize the gut-brain-microbiota axis in immunodeficient mice. Am. J. Physiol. Gastrointest. Liver Physiol. 307, G793–G802. doi: 10.1152/ajpgi.00238.2014
St Clair, D., Blackburn, I., Blackwood, D., and Tyrer, G. (1988). Measuring the course of Alzheimer's disease. A longitudinal study of neuropsychological function and changes in P3 event-related potential. Br. J. Psychiatry 152, 48–54. doi: 10.1192/bjp.152.1.48
Stein, D. J., Vasconcelos, M. F., Albrechet-Souza, L., Cereser, K. M. M., and de Almeida, R. M. M. (2017). Microglial over-activation by social defeat stress contributes to anxiety- and depressive-like behaviors. Front. Behav. Neurosci. 11:207. doi: 10.3389/fnbeh.2017.00207
Sudarov, A. (2013). Defining the role of cerebellar Purkinje cells in autism spectrum disorders. Cerebellum 12, 950–955. doi: 10.1007/s12311-013-0490-y
Sun, H. L., Bai, W., Li, X. H., Huang, H., Cui, X. L., Cheung, T., et al. (2022). Schizophrenia and inflammation research: a bibliometric analysis. Front. Immunol. 13:907851. doi: 10.3389/fimmu.2022.907851
Tsai, P. T., Hull, C., Chu, Y., Greene-Colozzi, E., Sadowski, A. R., Leech, J. M., et al. (2012). Autistic-like behaviour and cerebellar dysfunction in Purkinje cell Tsc1 mutant mice. Nature 488, 647–651. doi: 10.1038/nature11310
Upthegrove, R., and Khandaker, G. M. (2020). Cytokines, oxidative stress and cellular markers of inflammation in schizophrenia. Curr. Top. Behav. Neurosci. 44, 49–66. doi: 10.1007/7854_2018_88
Utz, K. S., Kohl, Z., Marterstock, D. C., Doerfler, A., Winkler, J., Schmidt, M., et al. (2022). Neuropsychology and MRI correlates of neurodegeneration in SPG11 hereditary spastic paraplegia. Orphanet J. Rare Dis. 17:301. doi: 10.1186/s13023-022-02451-1
Wang, Y. L., Han, Q. Q., Gong, W. Q., Pan, D. H., Wang, L. Z., Hu, W., et al. (2018). Microglial activation mediates chronic mild stress-induced depressive- and anxiety-like behavior in adult rats. J. Neuroinflamm. 15:21. doi: 10.1186/s12974-018-1054-3
Wijemanne, S., Shulman, J. M., Jimenez-Shahed, J., Curry, D., and Jankovic, J. (2015). SPG11 mutations associated with a complex phenotype resembling Dopa-responsive dystonia. Mov Disord Clin Pract 2, 149–154. doi: 10.1002/mdc3.12144
Keywords: adaptive immune system, neuroinflammation, behavioral abnormalities, repurposing drugs, social behavior
Citation: Hörner M, Popp S, Branchu J, Stevanin G, Darios F, Klebe S, Groh J and Martini R (2024) Clinically approved immunomodulators ameliorate behavioral changes in a mouse model of hereditary spastic paraplegia type 11. Front. Neurosci. 18:1299554. doi: 10.3389/fnins.2024.1299554
Edited by:
Marija Cvetanovic, University of Minnesota Twin Cities, United StatesReviewed by:
Jan Cendelin, Charles University, CzechiaFilippo M. Santorelli, Stella Maris Foundation (IRCCS), Italy
Copyright © 2024 Hörner, Popp, Branchu, Stevanin, Darios, Klebe, Groh and Martini. This is an open-access article distributed under the terms of the Creative Commons Attribution License (CC BY). The use, distribution or reproduction in other forums is permitted, provided the original author(s) and the copyright owner(s) are credited and that the original publication in this journal is cited, in accordance with accepted academic practice. No use, distribution or reproduction is permitted which does not comply with these terms.
*Correspondence: Rudolf Martini, UnVkb2xmLk1hcnRpbmlAdW5pLXd1ZXJ6YnVyZy5kZQ==; Janos Groh, SmFub3MuR3JvaEB0dW0uZGU=; SmFub3MuR3JvaEBkem5lLmRl