- 1Key Laboratory of Biorheological Science and Technology (Ministry of Education), College of Bioengineering, Chongqing University, Chongqing, China
- 2Key Laboratory of Hepatobiliary and Pancreatic Surgery, Institute of Hepatobiliary Surgery, Southwest Hospital, Third Military Medical University (Army Medical University), Chongqing, China
Chronic stress has a substantial influence on the tumor microenvironment (TME), leading to compromised effectiveness of anti-cancer therapies through diverse mechanisms. It disrupts vital functions of immune cells that play a critical role in anti-tumor immunity, such as the inhibition of dendritic cells (DCs) and lymphocytes, while simultaneously enhancing the activity of immune cells that support tumor growth, such as myeloid-derived suppressor cells and tumor-associated macrophages. Furthermore, chronic stress exerts a significant impact on crucial mechanisms within the TME, including angiogenesis, DNA repair, hypoxia, extracellular matrix deposition, and tumor metabolism. These alterations in the TME, induced by stress, result from the activation of the hypothalamic–pituitary–adrenal axis and sympathetic nervous system, in conjunction with epigenetic modifications. In conclusion, chronic stress significantly influences the TME and impedes the efficacy of anti-cancer treatments, underscoring the importance of targeting stress pathways to improve therapeutic results.
1 Introduction
Chronic stress has been identified as a significant factor in the initiation and advancement of tumors, as it fosters inflammation and leads to adverse cancer-related consequences such as depression, fatigue, sleep disturbances, and unfavorable prognosis (Kruk et al., 2019; Russell and Lightman, 2019; Conceição et al., 2021). This phenomenon can be ascribed to the enduring stimulation of the hypothalamic–pituitary–adrenal (HPA) axis and the sympathetic nervous system (SNS) during prolonged episodes of chronic stress. Consequently, stress hormones such as catecholamines [including norepinephrine (NE) and epinephrine (E)] are discharged from sympathetic nerves, while cortisol is liberated from the adrenal cortex (Wang et al., 2022; Wu et al., 2022b). In cancer patients during diagnosis and treatment, various neuroendocrine factors such as dopamine, prolactin, nerve growth factor, BDNF, substance P, and oxytocin are commonly observed and undergo central regulation in response to stress (McEwen and Boyd, 2018; Mravec et al., 2020). These neurotransmitters possess the ability to impact immune and endothelial cells within the TME, thereby facilitating tumor progression (Jiang et al., 2020; Battaglin et al., 2022). The nervous and immune systems establish communication through shared soluble mediators and receptors, enabling the brain to detect inflammation and modulate the immune response (Dantzer, 2018). The SNS exerts a dual effect on the immune system, enhancing humoral immune responses while concurrently suppressing cell-mediated immune responses through the inhibition of cytotoxic activity in T lymphocytes and NK cells. This ultimately results in an immunosuppressive TME mediated by stress hormones, particularly glucocorticoids (Qin et al., 2021).
The TME has gained recognition as a crucial element in the pathogenesis of cancer, encompassing stromal cells, immune cells, endothelial cells, and other resident cell types. These cells, previously considered passive observers in tumor development, are now acknowledged to have a pivotal role in driving cancer progression. A multitude of factors, encompassing intrinsic attributes of cancer cells, the location of tumor formation, the stage of the tumor, and variables specific to the patient, exert an influence on the composition and functional state of the TME, resulting in notable variations (Winkler et al., 2023). The dynamic interplay between cancer cells and the TME, comprising stromal cells and extracellular matrix constituents, plays a crucial role in fostering cancer cell heterogeneity, clonal evolution, and the development of multidrug resistance. Ultimately, these interactions expedite the advancement and dissemination of cancer (Kamiya et al., 2021).
Chronic stress has been shown to affect the effectiveness of anticancer treatments by exerting an influence on the TME (Hong et al., 2022). The SNS plays a role in the formation of pre-metastatic niches (Ieguchi et al., 2022). Neurological signals and pathways have an impact on various cancer characteristics, such as metabolism and (epi)genomic stability, as well as supporting microenvironments that promote tumor growth, including immune infiltration and the extracellular matrix (Vaes et al., 2022). Numerous studies have provided evidence that chronic stress can induce alterations in the TME, affecting tumor cells, cancer stromal cells, and the extracellular matrix, thereby facilitating the progression of cancer.
This article provides an overview of the impact of chronic stress on the TME, elucidates the underlying mechanisms responsible for TME alterations induced by chronic stress, and underscores the detrimental consequences of chronic stress on anticancer treatment efficacy. These findings underscore the imperative for the implementation of comprehensive anticancer strategies.
2 Mechanisms of TME alterations under chronic stress
Chronic stress significantly impacts the tumor microenvironment through two primary mechanisms: the activation of the HPA axis and the SNS. The HPA axis involves the secretion of CRH and adrenocorticotropic hormone (ACTH), which then triggers the release of cortisol by the adrenal gland. Similarly, the SNS can stimulate the secretion of E and NE by the adrenal medulla or neurons.
When triggered by chronic stress, these neuroendocrine systems exert diverse effects on immune and tumor cells within the tumor microenvironment. Chronic stress alters the composition and status of immune cells, impairs immune responses, and enables tumor cells to circumvent immune surveillance and develop resistance to drugs. Furthermore, chronic stress disrupts crucial processes such as angiogenesis, cell apoptosis, and metabolism, ultimately fostering tumor growth and progression (Figure 1; Tian et al., 2021).
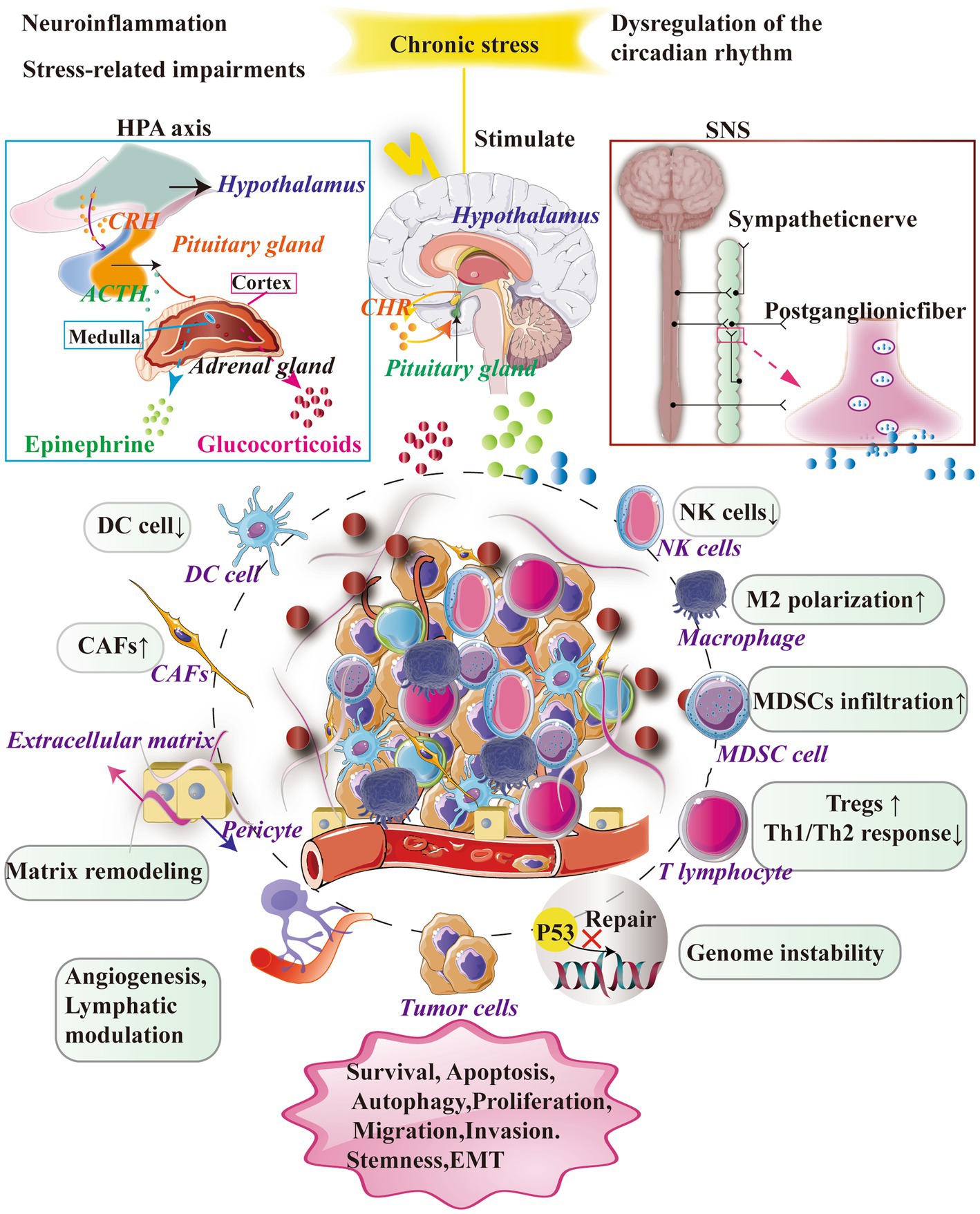
Figure 1. Chronic stress exerts a significant influence on the TME by primarily activating essential neuroendocrine response systems, namely the SNS and the HPA axis. The activation of the SNS leads to the secretion of NE and facilitates the synthesis and release of adrenaline and noradrenaline. However, the activation of the HPA axis results in the release of CRH from the hypothalamus, which in turn stimulates the secretion of adrenocorticotropic hormone ACTH and glucocorticoids (GCs) from the pituitary gland. It is worth noting that this complex cascade plays a significant role in facilitating the secretion and release of GCs from the adrenal cortex. Furthermore, the impact of stress-related hormones on cancer cells is mediated through their interaction with receptors on immune cells, thereby facilitating crucial biological processes including cell survival, programmed cell death, cellular self-degradation, cell division, cellular movement, and infiltration.
2.1 Immune cells
Extended periods of stress can hinder the immune system’s capacity to safeguard the body through the suppression of T cells, natural killer cells, and macrophages (Antoni and Dhabhar, 2019; Wieduwild et al., 2020; Thapa and Cao, 2023). Furthermore, chronic stress can enhance the accumulation of immune-inhibitory cells, specifically regulatory T and B cells, as well as tumor-associated macrophages. Additionally, persistent stress triggers inflammation in the TME, facilitating reciprocal interactions between cancerous and inflammatory immune cells. Upon infiltration into the TME, immune cells undergo metabolic adaptations (Bondar and Medzhitov, 2013; Bader et al., 2020). Tumor-associated macrophages (TAMs), tumor-infiltrating lymphocytes (TILs), DCs, and myeloid-derived suppressor cells (MDSCs) employ paracrine signaling to regulate cancer growth by releasing pro-inflammatory cytokines. These cellular components establish communication through direct interactions and the secretion of cytokines and chemokines, utilizing autocrine and paracrine mechanisms (Lorton and Bellinger, 2015; Chen and Mellman, 2017; Greten and Grivennikov, 2019).
2.1.1 Dendritic cells
DCs are essential in initiating adaptive immunity against cancer through the presentation of tumor antigens (Gardner and Ruffell, 2016; Sommershof et al., 2017). Nevertheless, chronic stress can undermine their functionality and result in immune suppression among recipients of cancer vaccines. This impairment is associated with the increased expression of stress-induced hormones and Tsc22d3, which diminish dendritic cell maturation and hinder their capacity to activate TCD8+ cells. To address this issue, reactivating the β2-AR receptor pathway and increasing Phosphorylated cAMP response element binding protein (pCREB) levels is crucial to restore CD40 signal transduction in DCs (Singh and Ranjan, 2023), and enhance the effectiveness of basic dendritic cell-based cancer vaccine therapy (Yang et al., 2019).
2.1.2 Lymphocytes/tumor-infiltrating lymphocytes
Chronic stress harms supportive T cells and increases immune-suppressive T cells, especially in breast cancer patients (Kamiya et al., 2019). Stress also upregulates TIGIT, an immune receptor critical in regulating anti-tumor and antiviral immune responses (Rudak et al., 2019). However, stress has been found to have a preserving effect on mucosal-associated invariant T cells, while simultaneously impairing their TH1/TH2-type responses (Rudak et al., 2021). T cells are typically categorized based on central markers such as CD8 and CD4, as well as receptor subunits (Paijens et al., 2021).
2.1.3 CD8 +t
The exploration of signaling mechanisms that influence the phenotype of CD8+ T cells within the TME carries substantial implications, as it offers valuable insights for the advancement of novel approaches in cancer treatment. β-adrenergic receptors (β-ARs) exert a significant regulatory function through the modulation of immune checkpoint molecules, resulting in the suppression of anti-tumor immune responses and the simultaneous upregulation of PD-1, TIM-3, and Lag3, ultimately leading to T cell exhaustion (Nissen et al., 2018; Acharya et al., 2020). Additionally, the endogenous signaling of glucocorticoids within the TME impacts the differentiation of CD8+T cells, thereby compromising the effectiveness of immune checkpoint blockade (Qiao et al., 2021). Activation of the SNS via β-ARs has been observed to modulate the metabolism of CD8+ T cells within the TME, resulting in hindered infiltration of these cells in murine models of melanoma and colon cancer (Geng et al., 2023). Moreover, the inhibition of CD8+ T cell infiltration and function caused by NE has been found to confer resistance to anti-PD-1 monoclonal antibodies in cases of lung adenocarcinoma and gastric cancer (Muthuswamy et al., 2017; Vaes et al., 2022). Additionally, the release of calcitonin gene-related peptide (CGRP) by tumor-associated neurons has been directly implicated in the exhaustion of CD8+ T cells, thereby impairing their ability to eliminate melanoma cells (Balood et al., 2022).
2.1.4 CD4 +t
CD4 +T cells are essential components of the anti-tumor immune response as they stimulate CD8+ T cells and induce DCs to express CD40 ligand (Borst et al., 2018). The differentiation of CD4 +T cells is influenced by specific combinations of cytokines, which can also lead to the generation of regulatory Tregs. Tregs have been linked to tumor growth due to their ability to produce immunosuppressive cytokines (Knochelmann et al., 2018). The glucocorticoid-induced leucine zipper (GILZ) plays a crucial role in promoting the development of regulatory T cells (Tregs) by inducing the expression of FoxP3. Furthermore, the activation of the β2-AR in Tregs impedes their ability to produce inhibitory cytokines (Cannarile et al., 2019). However, the presence of chronic stress has been observed to result in an elevation of CD4+T cells within tumor tissues, thereby potentially facilitating the differentiation of cancer stem cells (Thapa and Cao, 2023). According to T-cell-extrinsic mechanisms, stress hormones cause CD4+T cells to differentiate toward a Th2 response (Capelle et al., 2022). The utilization of αGITR antibodies to mitigate Tregs functionality has been discovered to induce the differentiation of CD4+T cells into effector T cells, diminish Tregs-mediated immune suppression, and generate anti-tumor impacts (Zenga et al., 2022). The potential alleviation of certain negative effects of chronic stress can be achieved through β-ARs antagonism (Partecke et al., 2016).
2.1.5 Natural killer cells
The role of NK cells in preventing and treating infections and cancer has long been recognized (Myers and Miller, 2021). Adrenaline and noradrenaline inhibit DNA-dependent mechanisms and protein synthesis, resulting in a reduction in cytokine production, including IL-2 and IFN-γ, and IL-12 (Neeman and Ben-Eliyahu, 2013). In addition to its role in regulating humoral immune responses, the SNS also plays a role in limiting the cytotoxic functions of T lymphocytes and NK cells in cellular responses (Jiang et al., 2017). The increased expression of programmed cell death receptor-1 (PD-1) in tumor-infiltrating NK cells, induced by glucocorticoid-mediated signaling, ultimately contributes to the progression of hepatocellular carcinoma in individuals with depression (Zhao et al., 2019).
2.1.6 Myeloid-derived suppressor cells
The immunosuppressive effects of cancer therapy involve the active involvement of MDSCs in mediating immune suppression (Bruno et al., 2019). MDSCs represent a specific subset of immature myeloid cells (Iñigo-Marco and Alonso, 2019). The process of chronic stress has been observed to enhance the accumulation of MDSCs via the IL-6/STAT3 signaling pathway, thereby creating a conducive environment for metastasis (Cheng et al., 2019; Mohammadpour et al., 2019; An et al., 2021). Additionally, chronic pressure-induced adrenal signals effectively stimulate the proliferation and activation of MDSCs, leading to the establishment of an immunosuppressive microenvironment (Mohammadpour et al., 2021). The activation of the CXCL5-CXCR2-Erk cascade, induced by chronic restraint stress, plays a crucial role in driving the regulatory and recruiting functions of MDSCs (Cao et al., 2021). Furthermore, it is important to note that psychological stress serves as a catalyst for the activation of MDSCs within the spleen via TAM/CXCL1 signaling. This process plays a pivotal role in facilitating the development of pre-metastatic niches in breast cancer (Zheng et al., 2023).
2.1.7 Macrophages
Additionally, macrophages play a vital role in modulating the TME, thereby influencing various aspects such as angiogenesis, remodeling of the extracellular matrix, proliferation of cancer cells, metastasis, immune suppression, and resistance to treatment (DeNardo and Ruffell, 2019; Mantovani et al., 2022). Psychological depression has been observed to exert a notable influence on the infiltration of macrophages in tumors, thereby significantly modulating their activity and inhibitory effects, ultimately impacting prognosis and treatment outcomes (Cassetta and Pollard, 2018, 2023). It has been found that the exposure of tumor cells to NE elicits the secretion of neuropeptide Y (NPY), which in turn facilitates the recruitment of macrophages and subsequent release of interleukin 6 (IL6). This phenomenon leads to the activation of the STAT3 signaling pathway in prostate cancer cells (Cheng et al., 2019). Furthermore, NE exerts control over the CCL2/CCR2 pathway through the stimulation of the β-ARs, thereby facilitating the migration and colonization of tumor cells in the lungs prior to metastasis (Chen et al., 2018). Moreover, the β3-AR receptor plays a crucial role in maintaining the equilibrium between M1/M2 macrophages and N1 granulocytes within the TME (Calvani et al., 2019). Glucocorticoids (GC) disrupt the ability of macrophages to effectively eliminate tumor cells by modulating the equilibrium between the “eat me” signal receptor (LRP1) and the “do not eat me” signal receptor (SIRPα) (Wu et al., 2022a). Additionally, the SNS expedites the activation of Kupffer cells via the ADRα1 receptor, thereby maintaining an inflammatory microenvironment that facilitates the progression of liver cancer (Huan et al., 2017).
2.1.8 Cancer-associated fibroblasts
CAFs play a pivotal and multifaceted role in the TME, influencing various aspects such as tumor cell survival, metastasis, angiogenesis, immunosuppression, and resistance to therapeutic interventions (Zhang Y. et al., 2023). Empirical evidence substantiates the hypothesis that the commencement of α2-AR acts as a trigger for the proliferation and dissemination of CAFs, thus leading to an augmentation of the concentration of TGF-β within the confined TME (Shan et al., 2014). These signaling molecules effectively facilitate the division of tumor cells and the formation of new blood vessels, thereby promoting the supply of essential nutrients and oxygen to sustain tumor growth (Chen and Song, 2019; Bejarano et al., 2021; Rimal et al., 2022). Additionally, catecholamines, such as NE, proficiently regulate CAFs by activating β-ARs, thereby inducing heightened collagen synthesis. The presence of reinforced structural support within the tumor tissue facilitates the migration, invasion, and metastatic progression of tumor cells to distant sites (Nagaraja et al., 2021).
2.2 Cytokines
Scientific evidence unequivocally links cancer progression with the presence of inflammatory mediators like TNF-α, IL-6, TGF-β, and IL-10 (Liu N. et al., 2021). This correlation has intricated and widespread effects, as chronic stress disrupts cytokine secretion and activates multiple cellular pathways that significantly impact the occurrence and progression of malignant tumors. Furthermore, chronic stress actively promotes tumor development by affecting specific cellular pathways (Märkl et al., 2022; Vignjević Petrinović et al., 2023). For instance, the CXCL3-mediated Wnt/β-catenin pathway alters fatty acid metabolism (Lou et al., 2023), while dopamine-induced nuclear translocation of DRD2 impairs HIF1α degradation (Bernabé, 2021), thus promoting malignant cancer progression. Notably, chronic restraint stress triggers hepatocellular carcinoma growth via the β-ARs signaling pathway, and acute myeloid leukemia is linked to the HMGB1/NLRP3/IL-1β signaling pathway (Liu N. et al., 2021). Interestingly, rapid activation of β2-ARs skillfully regulates inflammation by inducing IL-10 secretion (Ağaç et al., 2018). However, this activation also has the potential to exacerbate cytokine and chemokine secretion, thereby compromising immune cell functionality (Thapa and Cao, 2023).
2.3 Angiogenesis
Additionally, sympathetic NE has been shown to promote tumor progression and angiogenesis through interaction with β-ARs in endothelial cells (Hondermarck and Jobling, 2018; Zhang et al., 2019; Cervantes-Villagrana et al., 2020; March et al., 2020). Chronic stress has been found to have a detrimental effect on cancer metastasis and the expression of various factors such as VEGF, MMP-2, MMP-7, and MMP-9 (Harjes, 2017; Stone, 2018). The activation of β2-ARs signaling has been shown to inhibit PPARγ activity, thereby promoting the growth of breast cancer and VEGF/FGF2-mediated angiogenesis (Zahalka et al., 2017; Zhou et al., 2020; Jeong et al., 2022). Additionally, β- ARs signaling has been found to suppress HDAC2, contributing to tumor angiogenesis and the progression of prostate cancer (Hulsurkar et al., 2017). Lastly, chronic stress has been observed to enhance angiogenesis through the activation of the PlexinA1/VEGFR2-JAK2-STAT3 pathway (Lu et al., 2021).
2.4 DNA damage
It is important to note that chronic stress has a significant impact on DNA integrity, regardless of the presence of carcinogens (Eckerling et al., 2021; Valente et al., 2021). Stress hormones are integral to the process of DNA damage repair, concurrently producing reactive oxygen species (ROS) and reactive nitrogen species (RNS) (Iftikhar et al., 2021). It is worth noting that adrenaline can induce DNA damage even in ovarian cancer cells (Lamboy-Caraballo et al., 2020). Moreover, prolonged stress reduces antioxidant activity, resulting in the buildup of free radicals, impeding DNA damage repair, and fostering the progression of skin cancer (Muqbil et al., 2020).
2.5 EMT
The chronic presence of stress facilitates tumor metastasis and invasion through the activation of diverse pathways (Blaes et al., 2020). For example, the miR-337-3p/STAT3 pathway has been observed to stimulate EMT in breast cancer cells and lung adenocarcinoma cells, thereby promoting invasion and migration (Du et al., 2020; Jiao et al., 2023). The activation of B2AR, MOR, and GSK3 by adrenaline and opioid drugs has been identified as a contributing factor to drug resistance, particularly in triple-negative breast cancer cases (Rousseau et al., 2022). EMT induction in both tongue squamous cell carcinoma and colorectal cancer is facilitated by the activation of distinct signaling pathways (Liu et al., 2018; Zhou et al., 2022). The impact of the β2-AR agonist salbutamol on EMT, migration, and invasion has been substantiated through the activation of the ERK phosphorylation pathway (Lu et al., 2022). Additionally, melatonin has been proven to inhibit the metastasis of epithelial ovarian cancer induced by chronic stress by modulating the NE/AKT/β-catenin/SLUG axis (Bu et al., 2020).
2.6 Extracellular matrix
ECM plays a pivotal role in tumors, providing essential functions such as structural support, regulation of the microenvironment, and provision of signaling molecules (Huang et al., 2021). The continuous activation of FAK, which is facilitated by the chronic stress-induced signaling transduction of β-ARs through the cAMP/PKA pathway, initiates a consequential cascade in which Erk1/2-MMP orchestrates the remodeling of the ECM (Cheng et al., 2018). Importantly, this remodeling phenomenon plays a significant role in enhancing invasive properties and metastatic potential in the pathogenesis of melanoma and prostate cancer (Ji et al., 2019).
2.7 Metabolic disorder
Furthermore, chronic stress exerts a profound influence on the immune system, leading to disruptions in mitochondrial function and cellular metabolism (Fan et al., 2019; Ruan et al., 2021). It serves as a potent stimulus for the activation of specific adrenaline receptors (β1ARs and β3ARs), initiating a series of events that result in the accumulation of lipid droplets in MCF-7 breast cancer cells. This accumulation disrupts the normal metabolic processes to a significant extent (Silva et al., 2023). The activation of β-ARs additionally alters cellular metabolism, hindering oxidative phosphorylation and encouraging angiogenesis in prostate cancer (Faulkner et al., 2019). Importantly, prolonged stress stimulates LDHA-dependent glucose metabolism via USP28, thereby enhancing the stability of MYC (Cui et al., 2019).
2.8 Hypoxia
Pressure plays a crucial role in regulating the function of immune cells by influencing tissue perfusion (He et al., 2022). The activation of sympathetic nerves or the administration of adrenergic receptor agonists induces a state of tumor hypoxia within the TME, which impedes the migration of leukocytes and promotes tumor progression. The simultaneous activation of the α1d-AR by chronic stress and Helicobacter pylori infection initiates the ubiquitination process of the SerpinA1 complex, leading to hypoxia and facilitating its interaction with IL-1α. This interaction ultimately promotes the development of gastric tumors (Devi et al., 2021; Aziz et al., 2022; Sullivan et al., 2023).
3 The chronic stress affects the efficacy of cancer treatment
The effectiveness of cancer treatments is negatively impacted by chronic stress (Wu et al., 2022b; Table 1).
3.1 Chemotherapy
Chronic stress disrupts the cellular response to DNA damage, thereby exerting detrimental effects on chemotherapy. It is worth noting that NE not only influences the resistance of ovarian cancer cells to cisplatin but also compromises DNA integrity (Lamboy-Caraballo et al., 2020).
Furthermore, chronic stress weakens the apoptotic response of tumor cells triggered by chemotherapy, ultimately impairing the efficacy of cytotoxic agents. This impairment is exacerbated by stress-induced glucocorticoids, which increase cancer cell resistance and reduce the efficacy of multiple chemotherapy drugs, such as paclitaxel, doxorubicin, TRAIL, and fluorouracil (Kanai et al., 2020; Cui et al., 2021; Butz and Patócs, 2022; Mitre-Aguilar et al., 2022). Additionally, NE inhibits c-Jun phosphorylation, mediated by JNK, through activation of the β 2-AR pathway, thereby attenuating the apoptosis of ovarian cancer cells induced by paclitaxel (Kang et al., 2016; Chen et al., 2021).
3.2 Radiotherapy
Radiation oncology is primarily responsible for the occurrence of distant effects that can result in long-lasting damage to nearby radiation-sensitive nerves. The incidence of distant events following radiotherapy closely correlates with the level of adrenergic stress experienced by the host. Chronic stress has been found to have detrimental effects on T-cell function, gene expression, and signaling pathways, leading to a reduction in immune factor secretion. Adrenergic agonists exacerbate immune suppression and promote the presence of M2 macrophages, further compromising the immune system’s ability to regulate tumor growth. Furthermore, adrenergic agonists also impede the activity and function of CD8 +T cells, directly affecting their effectiveness in targeting tumors (Chen et al., 2020). Of particular significance is the decrease in expression of IFN-γ and Granzyme B in T cells caused by adrenergic agonists, which limits their effector phenotype. This reduction ultimately promotes resistance among tumor cells to radiation-induced cell death, thereby disrupting the anticancer effects of radiation (MacDonald et al., 2019). Additionally, β2-ARs agonists amplify the expression of Wnt1 and Drosha in LLC-1 cells, hindering radiation-induced cell apoptosis (Zhang X. et al., 2020).
3.3 Immunotherapy
Extensive preclinical research has supported the adverse effects of prolonged stress on the effectiveness of immune checkpoint inhibitors, tumor vaccines, and immune stimulants (Liu et al., 2023). It has also been demonstrated that psychological pressure reduces the therapeutic efficacy of IL-2 immunotherapy among patients with renal cell carcinoma, resulting in decreased expression of IL-2 receptors in peripheral blood leukocytes (Zhang L. et al., 2020). Additionally, scientists have observed that manipulation of glucocorticoid receptors in pancreatic cancer cells enhances immune evasion and resistance to immunotherapy, leading to changes in expression of PD-L1 and MHC-I genes (Deng et al., 2021). Furthermore, the β2-AR receptor has been found to disrupt the mitochondria’s adaptation of T-cells, impair T-cell activation, proliferation, cytokine release, and hinder the effectiveness of CAR-T cell therapy (Farooq et al., 2023). Also, chronic stress from social disruption suppresses CD8+ T-cell responses to cancer vaccines when administered via PLGA-MS, due to impaired dendritic cell maturation, migration, and CD8+ T-cell response initiation (Daher et al., 2019).
3.4 Molecularly targeted therapy
The research findings indicate that chronic stress impedes the inhibitory effects of EGFR, leading to a decrease in the effectiveness of the treatment. Additionally, the stress hormone NE increases the expression of Cx32 and enhances the transcription levels of proteins associated with resistance to EGFR-TKI, such as MET and IGF-1R (Xie et al., 2019). At the same time, it slows down the degradation rate of these proteins, further worsening the impact on the efficacy of Afatinib. Moreover, the activation of β2-AR has been found to inhibit LKB1 and promote the expression of IL-6, resulting in the development of resistance against EGFR TKI in non-small cell lung cancer (NSCLC) through the MAPK pathway (Nilsson et al., 2017; Chang et al., 2020).
4 Targeting chronic stress for enhanced anticancer therapies
The significant influence of chronic stress on the initiation and progression of cancer has been consistently demonstrated in multiple studies. Therefore, it is imperative to prioritize targeted management of chronic stress in order to enhance the efficacy of anticancer therapies (Figure 2).
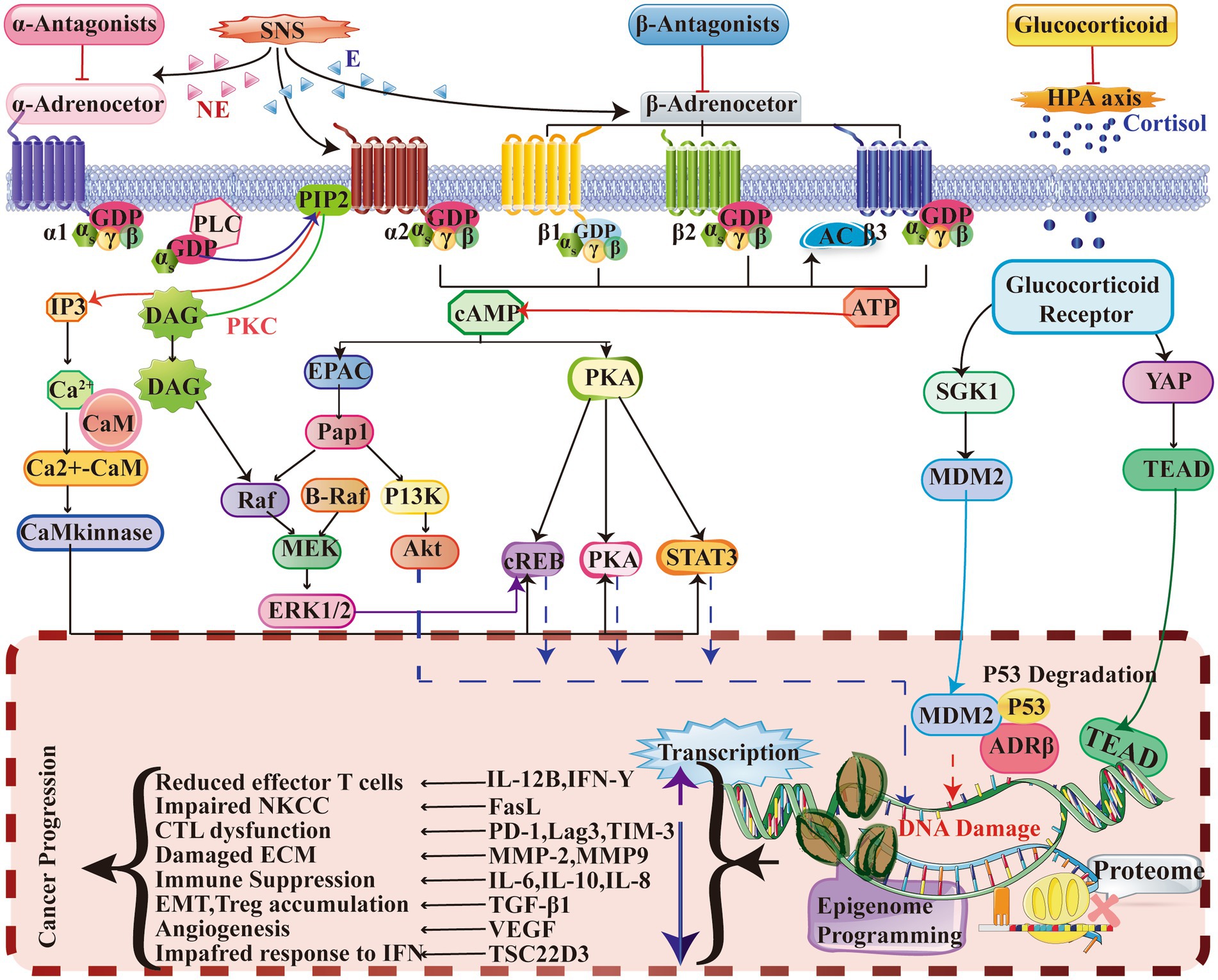
Figure 2. This study provides evidence that chronic stress induces the activation of a signaling pathway that significantly impacts the TME. ARs, specifically α-AR, β2-AR, and β3-AR, play a crucial role in this process. ARs function as G-protein coupled receptors (GPCRs) and bind to agonists such as adrenaline and noradrenaline. This binding activates intracellular Gαs protein, initiating a series of reactions that result in increased levels of intracellular IP3, DAG, and cAMP. Additionally, this activation simultaneously triggers second messenger pathways, including PKA, PKC, EPAC, and Ca2+-CaM. Furthermore, the involvement of glucocorticoid receptors (GRs), which are part of the nuclear receptor family, is also noteworthy. When GCs permeate the cell membrane and bind to GRs, the Hsp protein dissociates, enabling the primary subunit of GRs to relocate to the cell nucleus and initiate gene transcription. Consequently, the regulation of cytokine and ligand gene expression occurs, thereby impacting protein synthesis programming and epigenetic gene expression. Collectively, these signaling mechanisms induce changes that contribute to the deterioration of the TME and ultimately promote tumor development. E, Epinephrine; EPAC, Exchange protein directly activated by cAMP; DAG, Diacylglycerol; ERK, Extracellular signal-regulated kinase; PI3K, Phosphatidylinositol 3-kinase; Hsp., Heat shock protein; PKA, Protein kinase A; AC, Adenylyl cyclase; MDR, Multidrug resistance; GATA1, GATA-binding protein 1; STAT3, Signal transducer and activator of transcription 3; NE, Norepinephrine; CaM, Calmodulin; IP3, Inositol trisphosphate; PIP2, Phosphatidylinositol 4,5-bisphosphate; EMT, Epithelial-mesenchymal transition; PKC, Protein kinase C; PLC, Phospholipase C; CREB, cAMP response element-binding protein; GR, Glucocorticoid receptor; CTL, Cytotoxic T lymphocyte; ECM, Extracellular matrix; NKCC, NK cell cytotoxicity; IFN, Interferon; ATP, Adenosine triphosphate; cAMP, Cyclic adenosine monophosphate; MEK, Mitogen-activated protein kinase; Pap1, Pathway activator protein 1.
4.1 Medications targeting adrenergic receptors
The efficacy of ARs antagonists in the treatment of tumor formation and growth induced by chronic stress has been demonstrated (Nilsson et al., 2020). These antagonists encompass both alpha and beta blockers, as outlined in Table 2.
4.1.1 β-Blockers
The presence of catecholamines and β-ARs has been observed to exhibit correlation with various types of cancer, such as breast, bladder, glioma, prostate, colorectal, gastric, and melanoma cancers (Barathova et al., 2020; Matzner et al., 2020; Jayachandran et al., 2023; Li R.Q. et al., 2023). Increased sympathetic nervous system activity leading to elevated levels of catecholamines has been demonstrated to contribute to tumor growth, angiogenesis, and metastasis. Nevertheless, the mitigation of these carcinogenic effects can be achieved by inhibiting the interaction between catecholamines and β-ARs. For instance, research conducted on breast cancer cells subjected to the β-AR antagonist propranolol has revealed heightened levels of the tumor suppressor protein p53 and augmented cellular demise (Montoya et al., 2019). Propranolol has also exhibited efficacy in alleviating immune suppression facilitated by IFN-γ-induced PD-L1 expression in ovarian cancer (Falcinelli et al., 2023). Additional β1-AR blockers such as Nebivolol, Bisoprolol, and Landiolol have similarly exhibited potential in bolstering antitumor immune responses across various cancer categories, thereby potentially enhancing patient prognoses (Niu et al., 2021; Farhoumand et al., 2023; Jin et al., 2023; Li F. et al., 2023; Shahid et al., 2023; Springer et al., 2023; Yuan et al., 2023).
4.1.2 α-Blockers
α-AR blockers, such as quinazoline, phentolamine, silodosin, doxazosin, prazosin, and naproxen, have demonstrated significant potential as effective cancer treatments (Suzuki et al., 2019). These medications have successfully impeded tumor growth and the development of new blood vessels in advanced prostate, bladder, and renal cancers. Silodosin, for instance, has been found to suppress the growth of prostate cancer cells by blocking α 1-AR. Similarly, doxazosin has shown the ability to hinder the multiplication of bladder cancer cells and restrict tumor expansion. The combination of these receptor blockers with traditional chemotherapy, radiotherapy, and anti-EGFR treatment has resulted in improved overall survival rates for prostate cancer patients (Ashrafi et al., 2017). Furthermore, they enhance autophagy, which facilitates the breakdown of damaged cellular components, thereby intensifying their anticancer effects (Archer et al., 2021). Additionally, α 1-AR blockers have the potential to mitigate adverse effects and enhance the efficacy of CAR-T cell therapy (Liu D. et al., 2021). Quinazoline and phentolamine have been found to alleviate symptoms of cytokine release syndrome in CAR-T cell therapy patients (Li et al., 2020; Sun et al., 2020; Liu D. et al., 2021). These findings emphasize the use of α-AR blockers as promising agents in cancer therapy, highlighting their capability to enhance treatment outcomes (Shimizu et al., 2020; Florent et al., 2020a,b; Zhong et al., 2021).
4.2 Psychosocial intervention for stress-related tumors
Interventions that directly target both physical and psychological stressors can effectively improve the TME, leading to enhanced efficacy of cancer treatments (Li et al., 2019; Tack et al., 2021, 2022). These interventions include carefully structured exercise programs (Zhang et al., 2016; Marker et al., 2018; McGettigan et al., 2020), the use of music therapy (Batty et al., 2017), dietary improvements (Goto et al., 2017; Jacka et al., 2017; Yang et al., 2020; Van Blarigan et al., 2023) and the establishment of healthier sleep patterns (Jacob et al., 2018; Chen Y.C. et al., 2022). It has been firmly established that chronic stress has a significant impact on the progression of cancer and is closely linked to the gut-brain axis (Jiang et al., 2023; Radford-Smith and Anthony, 2023). By regulating the gut microbiota, stress responses in individuals with tumors can be alleviated (Zhang Z. et al., 2020; Kumar et al., 2023; Li C. et al., 2023). A range of interventions, such as probiotics, prebiotics, postbiotics, and 5-HTP, can effectively achieve this outcome (Zhang Z. et al., 2020; Jiang et al., 2023; Kumar et al., 2023; Li C. et al., 2023; Radford-Smith and Anthony, 2023). Moreover, the incorporation of conventional, complementary, and integrative approaches, including acupuncture (von Trott et al., 2020; Han et al., 2021; Chen et al., 2023), meditation (Cramer et al., 2017; Kinkead et al., 2018), herbal medicine (e.g., Si-Ni-San) (Zhang J. et al., 2023) and Tai Chi (Irwin et al., 2017), has demonstrated efficacy in suppressing the proliferation and spread of tumors caused by chronic psychological stress.
4.3 Therapeutics for nerve fibers
The treatment of stress-induced tumors necessitates the implementation of antineuronal therapy and neural ablation (Magnon and Hondermarck, 2023). Neural ablation, which combines surgical techniques and pharmacological intervention, effectively alleviates symptoms associated with tumor growth (Zhao et al., 2014; Rabben et al., 2016). The inhibition of nerve growth factor (NGF) serves as a crucial approach to hinder the advancement of diseases by impeding the growth and restructuring of neural fibers linked to the tumor (Lin et al., 2023; Yan et al., 2023). Moreover, research has demonstrated a substantial association between the existence of nociceptor neurons and enhanced rates of survival among individuals afflicted with high-grade ovarian tumors (HGSOC) (Restaino et al., 2023). To summarize, interventions such as antineuronal therapy, neural ablation, and NGF inhibition play a significant role in the management of tumors induced by chronic stress (Logotheti et al., 2020).
4.4 Immune system regulation
Targeting immune-suppressive neurotransmitters represents a potential approach for overcoming immune resistance and augmenting the anti-tumor immune response (Xiao et al., 2023). Notably, benzodiazepine-class drugs, functioning as GABA(A)R activators, have been observed to enhance the response to radiation and immune checkpoint blockade (ICB) by facilitating direct anti-tumor effects and the infiltration of CD8+ T cells. Additionally, dopamine signaling has demonstrated potential in promoting the differentiation of tissue-resident memory CD8+ T cells, thereby enhancing the effectiveness of chemotherapy in pancreatic cancer and regulating macrophage-mediated inflammation (Liu Q. et al., 2021; Chen Y. et al., 2022). The investigation of GITR in Tregs as a potential target has revealed that the use of antibodies can facilitate the transformation of Tregs into CD4 + T cells, thereby diminishing their inhibitory impact on tumor immunity (Amoozgar et al., 2021). Additionally, the administration of fluoxetine, an antidepressant, has been found to suppress the mechanisms induced by chronic stress in lung cancer, consequently augmenting the cellular immune response and leading to notable antitumor effects (Yang et al., 2021; Figure 3).
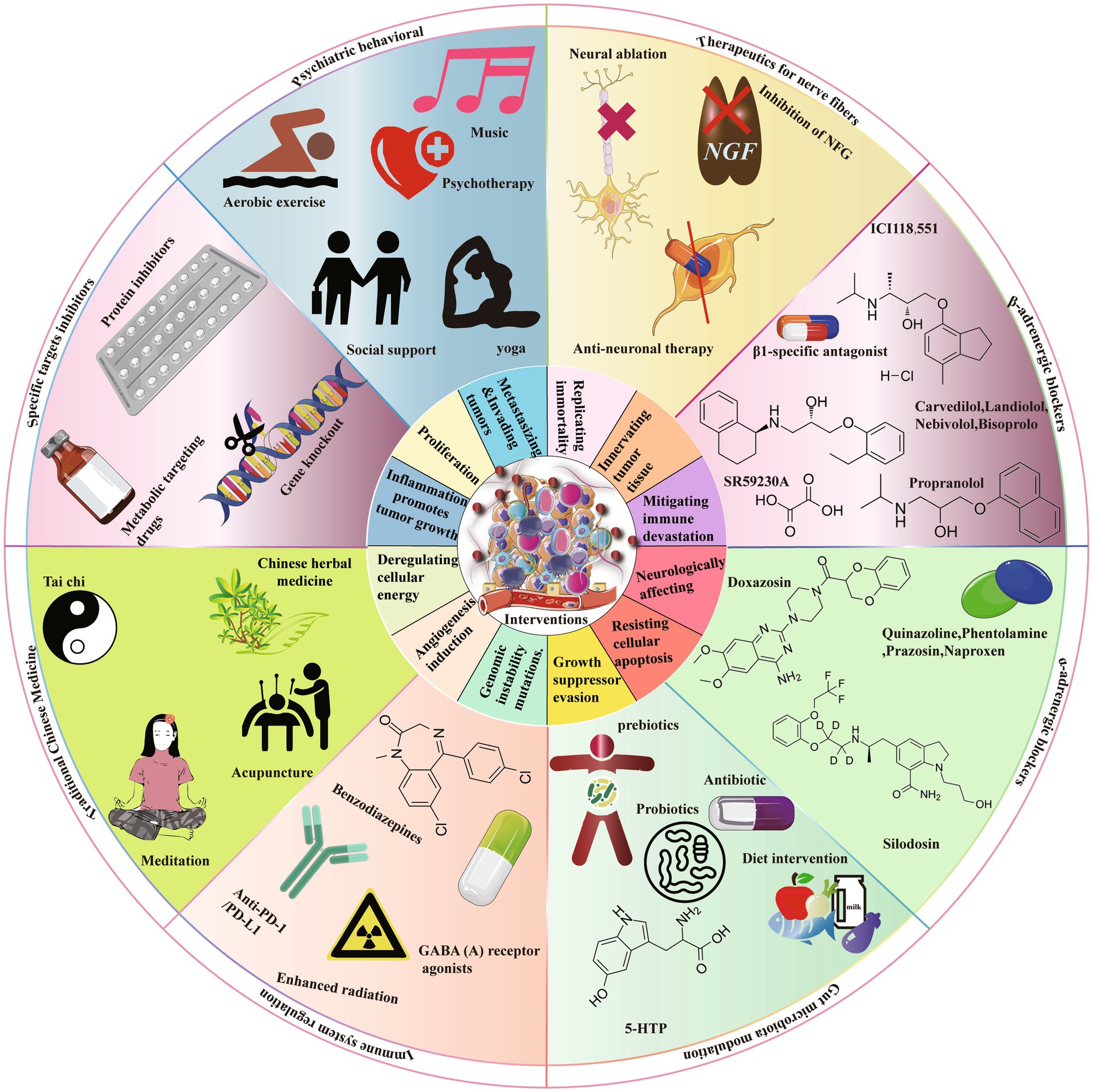
Figure 3. There are several strategies that can be implemented to mitigate the advancement of cancer resulting from chronic stress. These strategies encompass the use of targeted inhibitors, psychosocial interventions, neurofiber therapy, receptor blockers (including both β-ARs and α-ARs blockers), modulation of gut microbiota, modulation of the immune system, and the incorporation of traditional Chinese medicine. Targeted inhibitors encompass the utilization of pharmacological compounds, protein inhibitors, and other substances that specifically target metabolic processes. Psychosocial interventions encompass a diverse array of activities, including aerobic exercise, psychotherapy, yoga therapy, music therapy, and mindfulness training. Neurofiber therapy incorporates a multitude of treatment modalities, such as nerve ablation, inhibition of nerve growth factor, and interventions for anti-neural diseases. The classification of β-ARs blockers includes subcategories such as β2-AR blockers and β3-AR blockers, whereas α-ARs blockers encompass both α2-AR blockers and α1-AR blockers.
5 Conclusion
The examination of prolonged stress in cancer therapy holds significant significance due to its direct influence on the neoplastic microenvironment, ultimately compromising treatment effectiveness and impairing immune responses. It is imperative to acquire a comprehensive comprehension of the complex interaction between persistent stress, stromal cells, and the immune system to formulate effective treatment approaches. Additionally, elucidating the impact of prolonged stress on neoplastic genetics and expression patterns can yield valuable insights into treatment response and prognosis. The advent of innovative technologies, such as artificial intelligence and optogenetics, offers promising opportunities for addressing chronic stress and improving cancer therapies, thereby enabling personalized and targeted treatment strategies. Furthermore, the potential synergy between β-blockers and anti-angiogenic agents, immunotherapy, radiotherapy, or chemotherapy holds promise for enhancing treatment efficacy. The potential for alleviating the adverse impacts of prolonged stress lies in the implementation of mindfulness and meditation interventions, in conjunction with meticulous evaluation of drug dosage and timing. To advance cancer care and the field of personalized medicine, it is imperative to undertake a comprehensive examination of the interplay between persistent stress, the neoplastic microenvironment, stromal cells, the immune system, and treatment.
Author contributions
YRL: Conceptualization, Methodology, Writing – original draft. FHL: Funding acquisition, Resources, Writing – original draft. YCT: Project administration, Writing – original draft. YNW: Formal analysis, Writing – original draft. FX: Supervision, Writing – review & editing. JHW: Funding acquisition, Validation, Writing – review & editing.
Funding
The author(s) declare financial support was received for the research, authorship, and/or publication of this article. This work was supported by the National Major Scientific Research Instrument Development Project of NSFC (21827812) and the Natural Science Foundation of Chong-Qing (CSTB2023NSCQ-MSX0563).
Conflict of interest
The authors declare that the research was conducted in the absence of any commercial or financial relationships that could be construed as a potential conflict of interest.
Publisher’s note
All claims expressed in this article are solely those of the authors and do not necessarily represent those of their affiliated organizations, or those of the publisher, the editors and the reviewers. Any product that may be evaluated in this article, or claim that may be made by its manufacturer, is not guaranteed or endorsed by the publisher.
References
Acharya, N., Madi, A., Zhang, H., Klapholz, M., Escobar, G., Dulberg, S., et al. (2020). Endogenous glucocorticoid signaling regulates CD8(+) T cell differentiation and development of dysfunction in the tumor microenvironment. Immunity 53, 658–671.e656. doi: 10.1016/j.immuni.2020.08.005
Ağaç, D., Estrada, L. D., Maples, R., Hooper, L. V., and Farrar, J. D. (2018). The β2-adrenergic receptor controls inflammation by driving rapid IL-10 secretion. Brain Behav. Immun. 74, 176–185. doi: 10.1016/j.bbi.2018.09.004
Amoozgar, Z., Kloepper, J., Ren, J., Tay, R. E., Kazer, S. W., Kiner, E., et al. (2021). Targeting Treg cells with GITR activation alleviates resistance to immunotherapy in murine glioblastomas. Nat. Commun. 12:2582. doi: 10.1038/s41467-021-22885-8
An, J., Feng, L., Ren, J., Li, Y., Li, G., Liu, C., et al. (2021). Chronic stress promotes breast carcinoma metastasis by accumulating myeloid-derived suppressor cells through activating β-adrenergic signaling. Onco Targets Ther 10:2004659. doi: 10.1080/2162402x.2021.2004659
Antoni, M. H., and Dhabhar, F. S. (2019). The impact of psychosocial stress and stress management on immune responses in patients with cancer. Cancer 125, 1417–1431. doi: 10.1002/cncr.31943
Arbour, K. C., Mezquita, L., Long, N., Rizvi, H., Auclin, E., Ni, A., et al. (2018). Impact of baseline steroids on efficacy of programmed cell Death-1 and programmed death-ligand 1 blockade in patients with non-small-cell lung Cancer. J. Clin. Oncol. 36, 2872–2878. doi: 10.1200/JCO.2018.79.0006
Archer, M., Dogra, N., Dovey, Z., Ganta, T., Jang, H. S., Khusid, J. A., et al. (2021). Role of α- and β-adrenergic signaling in phenotypic targeting: significance in benign and malignant urologic disease. Cell Commun. Signal 19:78. doi: 10.1186/s12964-021-00755-6
Ashrafi, S., Shapouri, R., and Mahdavi, M. (2017). Immunological consequences of immunization with tumor lysate vaccine and propranolol as an adjuvant: a study on cytokine profiles in breast tumor microenvironment. Immunol. Lett. 181, 63–70. doi: 10.1016/j.imlet.2016.11.014
Aston, W. J., Hope, D. E., Cook, A. M., Boon, L., Dick, I., Nowak, A. K., et al. (2019). Dexamethasone differentially depletes tumour and peripheral blood lymphocytes and can impact the efficacy of chemotherapy/checkpoint blockade combination treatment. Onco Targets Ther 8:e1641390. doi: 10.1080/2162402X.2019.1641390
Aziz, F., Li, X., Chakraborty, A., Zheng, Y., Xin, M., Liu, K., et al. (2022). Ubiquitination of ADRα1d/SerpinA1 complex stimulates hypoxia to induce gastric tumorigenesis with a combination of helicobacter pylori and chronic stress through IL-1α. Gastric Cancer 25, 726–740. doi: 10.1007/s10120-022-01297-7
Bader, J. E., Voss, K., and Rathmell, J. C. (2020). Targeting metabolism to improve the tumor microenvironment for cancer immunotherapy. Mol. Cell 78, 1019–1033. doi: 10.1016/j.molcel.2020.05.034
Balood, M., Ahmadi, M., Eichwald, T., Ahmadi, A., Majdoubi, A., Roversi, K., et al. (2022). Nociceptor neurons affect cancer immunosurveillance. Nature 611, 405–412. doi: 10.1038/s41586-022-05374-w
Barathova, M., Grossmannova, K., Belvoncikova, P., Kubasova, V., Simko, V., Skubla, R., et al. (2020). Impairment of hypoxia-induced CA IX by beta-blocker propranolol—impact on progression and metastatic potential of colorectal cancer cells. Int. J. Mol. Sci. 21:8760. doi: 10.3390/ijms21228760
Battaglin, F., Jayachandran, P., Strelez, C., Lenz, A., Algaze, S., Soni, S., et al. (2022). Neurotransmitter signaling: a new frontier in colorectal cancer biology and treatment. Oncogene 41, 4769–4778. doi: 10.1038/s41388-022-02479-4
Batty, G. D., Russ, T. C., Stamatakis, E., and Kivimäki, M. (2017). Psychological distress in relation to site specific cancer mortality: pooling of unpublished data from 16 prospective cohort studies. BMJ 356:j108. doi: 10.1136/bmj.j108
Bejarano, L., Jordāo, M. J. C., and Joyce, J. A. (2021). Therapeutic targeting of the tumor microenvironment. Cancer Discov. 11, 933–959. doi: 10.1158/2159-8290.CD-20-1808
Bernabé, D. G. (2021). Catecholamines mediate psychologic stress-induced cancer progression. Cancer Res. 81, 5144–5146. doi: 10.1158/0008-5472.CAN-21-3077
Blaes, A. H., Domingo-Musibay, E., and Kalinsky, K. (2020). Propranolol: what is BLOCKing its clinical investigation in breast cancer? Clin. Cancer Res. 26, 1781–1783. doi: 10.1158/1078-0432.CCR-19-3818
Bondar, T., and Medzhitov, R. (2013). The origins of tumor-promoting inflammation. Cancer Cell 24, 143–144. doi: 10.1016/j.ccr.2013.07.016
Borst, J., Ahrends, T., Bąbała, N., Melief, C. J. M., and Kastenmüller, W. (2018). CD4(+) T cell help in cancer immunology and immunotherapy. Nat. Rev. Immunol. 18, 635–647. doi: 10.1038/s41577-018-0044-0
Bruno, G., Cencetti, F., Pini, A., Tondo, A., Cuzzubbo, D., Fontani, F., et al. (2020). β3-adrenoreceptor blockade reduces tumor growth and increases neuronal differentiation in neuroblastoma via SK2/S1P(2) modulation. Oncogene 39, 368–384. doi: 10.1038/s41388-019-0993-1
Bruno, A., Mortara, L., Baci, D., Noonan, D. M., and Albini, A. (2019). Myeloid derived suppressor cells interactions with natural killer cells and pro-angiogenic activities: roles in tumor progression. Front. Immunol. 10:771. doi: 10.3389/fimmu.2019.00771
Bruno, G., Nastasi, N., Subbiani, A., Boaretto, A., Ciullini Mannurita, S., Mattei, G., et al. (2023). β3-adrenergic receptor on tumor-infiltrating lymphocytes sustains IFN-γ-dependent PD-L1 expression and impairs anti-tumor immunity in neuroblastoma. Cancer Gene Ther. 30, 890–904. doi: 10.1038/s41417-023-00599-x
Bu, S., Wang, Q., Sun, J., Li, X., Gu, T., and Lai, D. (2020). Melatonin suppresses chronic restraint stress-mediated metastasis of epithelial ovarian cancer via NE/AKT/β-catenin/SLUG axis. Cell Death Dis. 11:644. doi: 10.1038/s41419-020-02906-y
Butz, H., and Patócs, A. (2022). Mechanisms behind context-dependent role of glucocorticoids in breast cancer progression. Cancer Metastasis Rev. 41, 803–832. doi: 10.1007/s10555-022-10047-1
Calvani, M., Bruno, G., Dabraio, A., Subbiani, A., Bianchini, F., Fontani, F., et al. (2020). β3-Adrenoreceptor blockade induces stem cells differentiation in melanoma microenvironment. Int. J. Mol. Sci. 21:1420. doi: 10.3390/ijms21041420
Calvani, M., Bruno, G., Dal Monte, M., Nassini, R., Fontani, F., Casini, A., et al. (2019). β(3) -Adrenoceptor as a potential immuno-suppressor agent in melanoma. Br. J. Pharmacol. 176, 2509–2524. doi: 10.1111/bph.14660
Cannarile, L., Delfino, D. V., Adorisio, S., Riccardi, C., and Ayroldi, E. (2019). Implicating the role of GILZ in glucocorticoid modulation of T-cell activation. Front. Immunol. 10:1823. doi: 10.3389/fimmu.2019.01823
Cao, M., Huang, W., Chen, Y., Li, G., Liu, N., Wu, Y., et al. (2021). Chronic restraint stress promotes the mobilization and recruitment of myeloid-derived suppressor cells through β-adrenergic-activated CXCL5-CXCR2-Erk signaling cascades. Int. J. Cancer 149, 460–472. doi: 10.1002/ijc.33552
Capelle, C. M., Chen, A., Zeng, N., Baron, A., Grzyb, K., Arns, T., et al. (2022). Stress hormone signalling inhibits Th1 polarization in a CD4 T-cell-intrinsic manner via mTORC1 and the circadian gene PER1. Immunology 165, 428–444. doi: 10.1111/imm.13448
Cassetta, L., and Pollard, J. W. (2018). Targeting macrophages: therapeutic approaches in cancer. Nat. Rev. Drug Discov. 17, 887–904. doi: 10.1038/nrd.2018.169
Cassetta, L., and Pollard, J. W. (2023). A timeline of tumour-associated macrophage biology. Nat. Rev. Cancer 23, 238–257. doi: 10.1038/s41568-022-00547-1
Cervantes-Villagrana, R. D., Albores-García, D., Cervantes-Villagrana, A. R., and García-Acevez, S. J. (2020). Tumor-induced neurogenesis and immune evasion as targets of innovative anti-cancer therapies. Signal Transduct. Target. Ther. 5:99. doi: 10.1038/s41392-020-0205-z
Chang, C. H., Lee, C. H., Ko, J. C., Chang, L. Y., Lee, M. C., Zhang, J. F., et al. (2020). Effect of β-blocker in treatment-Naïve patients with advanced lung adenocarcinoma receiving first-generation EGFR-TKIs. Front. Oncol. 10:583529. doi: 10.3389/fonc.2020.583529
Chen, Y. C., Chen, W. M., Chiang, M. F., Shia, B. C., and Wu, S. Y. (2022). Association between pre-existing sleep disorders and survival rates of patients with breast Cancer. Cancers 14:798. doi: 10.3390/cancers14030798
Chen, N., Ge, M. M., Li, D. Y., Wang, X. M., Liu, D. Q., Ye, D. W., et al. (2021). β2-adrenoreceptor agonist ameliorates mechanical allodynia in paclitaxel-induced neuropathic pain via induction of mitochondrial biogenesis. Biomed. Pharmacother. 144:112331. doi: 10.1016/j.biopha.2021.112331
Chen, H., Liu, D., Guo, L., Cheng, X., Guo, N., and Shi, M. (2018). Chronic psychological stress promotes lung metastatic colonization of circulating breast cancer cells by decorating a pre-metastatic niche through activating β-adrenergic signaling. J. Pathol. 244, 49–60. doi: 10.1002/path.4988
Chen, D. S., and Mellman, I. (2017). Elements of cancer immunity and the cancer-immune set point. Nature 541, 321–330. doi: 10.1038/nature21349
Chen, M., Qiao, G., Hylander, B. L., Mohammadpour, H., Wang, X. Y., Subjeck, J. R., et al. (2020). Adrenergic stress constrains the development of anti-tumor immunity and abscopal responses following local radiation. Nat. Commun. 11:1821. doi: 10.1038/s41467-020-15676-0
Chen, X., and Song, E. (2019). Turning foes to friends: targeting cancer-associated fibroblasts. Nat. Rev. Drug Discov. 18, 99–115. doi: 10.1038/s41573-018-0004-1
Chen, Y., Yan, S. M., Pu, Z., Feng, J., Tan, L., Li, Y., et al. (2022). Dopamine signaling promotes tissue-resident memory differentiation of CD8+ T cells and antitumor immunity. Cancer Res. 82, 3130–3142. doi: 10.1158/0008-5472.Can-21-4084
Chen, W., Zhang, X. N., Su, Y. S., Wang, X. Y., Li, H. C., Liu, Y. H., et al. (2023). Electroacupuncture activated local sympathetic noradrenergic signaling to relieve synovitis and referred pain behaviors in knee osteoarthritis rats. Front. Mol. Neurosci. 16:1069965. doi: 10.3389/fnmol.2023.1069965
Cheng, Y., Gao, X. H., Li, X. J., Cao, Q. H., Zhao, D. D., Zhou, J. R., et al. (2018). Depression promotes prostate cancer invasion and metastasis via a sympathetic-cAMP-FAK signaling pathway. Oncogene 37, 2953–2966. doi: 10.1038/s41388-018-0177-4
Cheng, Y., Tang, X. Y., Li, Y. X., Zhao, D. D., Cao, Q. H., Wu, H. X., et al. (2019). Depression-induced neuropeptide Y secretion promotes prostate cancer growth by recruiting myeloid cells. Clin. Cancer Res. 25, 2621–2632. doi: 10.1158/1078-0432.CCR-18-2912
Conceição, F., Sousa, D. M., Paredes, J., and Lamghari, M. (2021). Sympathetic activity in breast cancer and metastasis: partners in crime. Bone Res 9:9. doi: 10.1038/s41413-021-00137-1
Cramer, H., Lauche, R., Klose, P., Lange, S., Langhorst, J., and Dobos, G. J. (2017). Yoga for improving health-related quality of life, mental health and cancer-related symptoms in women diagnosed with breast cancer. Cochrane Database Syst. Rev. 1:Cd010802. doi: 10.1002/14651858.CD010802.pub2
Cui, Y., Han, X., Liu, H., Xie, Q., Guan, Y., Yin, B., et al. (2023). Impact of endogenous glucocorticoid on response to immune checkpoint blockade in patients with advanced cancer. Front. Immunol. 14:1081790. doi: 10.3389/fimmu.2023.1081790
Cui, Q., Jiang, D., Zhang, Y., and Chen, C. (2023). The tumor-nerve circuit in breast cancer. Cancer Metastasis Rev. 42, 543–574. doi: 10.1007/s10555-023-10095-1
Cui, B., Luo, Y., Tian, P., Peng, F., Lu, J., Yang, Y., et al. (2019). Stress-induced epinephrine enhances lactate dehydrogenase A and promotes breast cancer stem-like cells. J. Clin. Invest. 129, 1030–1046. doi: 10.1172/JCI121685
Cui, B., Peng, F., Lu, J., He, B., Su, Q., Luo, H., et al. (2021). Cancer and stress: NextGen strategies. Brain Behav. Immun. 93, 368–383. doi: 10.1016/j.bbi.2020.11.005
Daher, C., Vimeux, L., Stoeva, R., Peranzoni, E., Bismuth, G., Wieduwild, E., et al. (2019). Blockade of β-adrenergic receptors improves CD8(+) T-cell priming and cancer vaccine efficacy. Cancer Immunol. Res. 7, 1849–1863. doi: 10.1158/2326-6066.CIR-18-0833
Dantzer, R. (2018). Neuroimmune interactions: from the brain to the immune system and vice versa. Physiol. Rev. 98, 477–504. doi: 10.1152/physrev.00039.2016
DeNardo, D. G., and Ruffell, B. (2019). Macrophages as regulators of tumour immunity and immunotherapy. Nat. Rev. Immunol. 19, 369–382. doi: 10.1038/s41577-019-0127-6
Deng, Y., Xia, X., Zhao, Y., Zhao, Z., Martinez, C., Yin, W., et al. (2021). Glucocorticoid receptor regulates PD-L1 and MHC-I in pancreatic cancer cells to promote immune evasion and immunotherapy resistance. Nat. Commun. 12:7041. doi: 10.1038/s41467-021-27349-7
Devi, S., Alexandre, Y. O., Loi, J. K., Gillis, R., Ghazanfari, N., Creed, S. J., et al. (2021). Adrenergic regulation of the vasculature impairs leukocyte interstitial migration and suppresses immune responses. Immunity 54, 1219–1230.e1217. doi: 10.1016/j.immuni.2021.03.025
Du, P., Zeng, H., Xiao, Y., Zhao, Y., Zheng, B., Deng, Y., et al. (2020). Chronic stress promotes EMT-mediated metastasis through activation of STAT3 signaling pathway by miR-337-3p in breast cancer. Cell Death Dis. 11:761. doi: 10.1038/s41419-020-02981-1
Eckerling, A., Ricon-Becker, I., Sorski, L., Sandbank, E., and Ben-Eliyahu, S. (2021). Stress and cancer: mechanisms, significance and future directions. Nat. Rev. Cancer 21, 767–785. doi: 10.1038/s41568-021-00395-5
Falcinelli, M., Al-Hity, G., Baron, S., Mampay, M., Allen, M. C., Samuels, M., et al. (2023). Propranolol reduces IFN-γ driven PD-L1 immunosuppression and improves anti-tumour immunity in ovarian cancer. Brain Behav. Immun. 110, 1–12. doi: 10.1016/j.bbi.2023.02.011
Fan, K. Q., Li, Y. Y., Wang, H. L., Mao, X. T., Guo, J. X., Wang, F., et al. (2019). Stress-induced metabolic disorder in peripheral CD4(+) T cells leads to anxiety-like behavior. Cells 179, 864–879.e819. doi: 10.1016/j.cell.2019.10.001
Farhoumand, L. S., Liu, H., Tsimpaki, T., Hendgen-Cotta, U. B., Rassaf, T., Bechrakis, N. E., et al. (2023). Blockade of ß-adrenergic receptors by Nebivolol enables tumor control potential for uveal melanoma in 3D tumor spheroids and 2D cultures. Int. J. Mol. Sci. 24:5894. doi: 10.3390/ijms24065894
Farooq, M. A., Ajmal, I., Hui, X., Chen, Y., Ren, Y., and Jiang, W. (2023). β2-adrenergic receptor mediated inhibition of T cell function and its implications for CAR-T cell therapy. Int. J. Mol. Sci. 24:12837. doi: 10.3390/ijms241612837
Faulkner, S., Jobling, P., March, B., Jiang, C. C., and Hondermarck, H. (2019). Tumor neurobiology and the war of nerves in cancer. Cancer Discov. 9, 702–710. doi: 10.1158/2159-8290.CD-18-1398
Florent, R., Poulain, L., and N'Diaye, M. (2020a). Drug repositioning of the α(1)-adrenergic receptor antagonist Naftopidil: a potential new anti-cancer drug? Int. J. Mol. Sci. 21:5339. doi: 10.3390/ijms21155339
Florent, R., Weiswald, L. B., Lambert, B., Brotin, E., Abeilard, E., Louis, M. H., et al. (2020b). Bim, Puma and Noxa upregulation by Naftopidil sensitizes ovarian cancer to the BH3-mimetic ABT-737 and the MEK inhibitor Trametinib. Cell Death Dis. 11:380. doi: 10.1038/s41419-020-2588-8
Fu, S., Liu, M., Zhu, C., Zhang, H., Zhao, C., Xie, Y., et al. (2023). Regulatory mucosa-associated invariant T cells controlled by β1 adrenergic receptor signaling contribute to hepatocellular carcinoma progression. Hepatology 78, 72–87. doi: 10.1097/HEP.0000000000000014
Gardner, A., and Ruffell, B. (2016). Dendritic cells and cancer immunity. Trends Immunol. 37, 855–865. doi: 10.1016/j.it.2016.09.006
Geng, Q., Li, L., Shen, Z., Zheng, Y., Wang, L., Xue, R., et al. (2023). Norepinephrine inhibits CD8(+) T-cell infiltration and function, inducing anti-PD-1 mAb resistance in lung adenocarcinoma. Br. J. Cancer 128, 1223–1235. doi: 10.1038/s41416-022-02132-7
Globig, A. M., Zhao, S., Roginsky, J., Maltez, V. I., Guiza, J., Avina-Ochoa, N., et al. (2023). The β(1)-adrenergic receptor links sympathetic nerves to T cell exhaustion. Nature 622, 383–392. doi: 10.1038/s41586-023-06568-6
Goto, T., Tomonaga, S., and Toyoda, A. (2017). Effects of diet quality and psychosocial stress on the metabolic profiles of mice. J. Proteome Res. 16, 1857–1867. doi: 10.1021/acs.jproteome.6b00859
Greten, F. R., and Grivennikov, S. I. (2019). Inflammation and Cancer: triggers, mechanisms, and consequences. Immunity 51, 27–41. doi: 10.1016/j.immuni.2019.06.025
Guan, Y., Yao, W., Yu, H., Feng, Y., Zhao, Y., Zhan, X., et al. (2023). Chronic stress promotes colorectal cancer progression by enhancing glycolysis through β2-AR/CREB1 signal pathway. Int. J. Biol. Sci. 19, 2006–2019. doi: 10.7150/ijbs.79583
Haldar, R., Ricon-Becker, I., Radin, A., Gutman, M., Cole, S. W., Zmora, O., et al. (2020). Perioperative COX2 and β-adrenergic blockade improves biomarkers of tumor metastasis, immunity, and inflammation in colorectal cancer: a randomized controlled trial. Cancer 126, 3991–4001. doi: 10.1002/cncr.32950
Haldar, R., Shaashua, L., Lavon, H., Lyons, Y. A., Zmora, O., Sharon, E., et al. (2018). Perioperative inhibition of β-adrenergic and COX2 signaling in a clinical trial in breast cancer patients improves tumor Ki-67 expression, serum cytokine levels, and PBMCs transcriptome. Brain Behav. Immun. 73, 294–309. doi: 10.1016/j.bbi.2018.05.014
Han, K., Kim, M., Kim, E. J., Park, Y. C., Kwon, O., Kim, A. R., et al. (2021). Moxibustion for treating cancer-related fatigue: a multicenter, assessor-blinded, randomized controlled clinical trial. Cancer Med. 10, 4721–4733. doi: 10.1002/cam4.4020
Harjes, U. (2017). Tumour angiogenesis: controlling nerves. Nat. Rev. Cancer 17:708. doi: 10.1038/nrc.2017.115
He, G., Peng, X., Wei, S., Yang, S., Li, X., Huang, M., et al. (2022). Exosomes in the hypoxic TME: from release, uptake and biofunctions to clinical applications. Mol. Cancer 21:19. doi: 10.1186/s12943-021-01440-5
Hiller, J. G., Cole, S. W., Crone, E. M., Byrne, D. J., Shackleford, D. M., Pang, J. B., et al. (2020). Preoperative β-blockade with propranolol reduces biomarkers of metastasis in breast cancer: a phase II randomized trial. Clin. Cancer Res. 26, 1803–1811. doi: 10.1158/1078-0432.CCR-19-2641
Hondermarck, H., and Jobling, P. (2018). The sympathetic nervous system drives tumor angiogenesis. Trends Cancer 4, 93–94. doi: 10.1016/j.trecan.2017.11.008
Hong, Y., Zhang, L., Liu, N., Xu, X., Liu, D., and Tu, J. (2022). The central nervous mechanism of stress-promoting cancer progression. Int. J. Mol. Sci. 23:12653. doi: 10.3390/ijms232012653
Huan, H. B., Wen, X. D., Chen, X. J., Wu, L., Wu, L. L., Zhang, L., et al. (2017). Sympathetic nervous system promotes hepatocarcinogenesis by modulating inflammation through activation of alpha1-adrenergic receptors of Kupffer cells. Brain Behav. Immun. 59, 118–134. doi: 10.1016/j.bbi.2016.08.016
Huang, J., Zhang, L., Wan, D., Zhou, L., Zheng, S., Lin, S., et al. (2021). Extracellular matrix and its therapeutic potential for cancer treatment. Signal Transduct. Target. Ther. 6:153. doi: 10.1038/s41392-021-00544-0
Hulsurkar, M., Li, Z., Zhang, Y., Li, X., Zheng, D., and Li, W. (2017). Beta-adrenergic signaling promotes tumor angiogenesis and prostate cancer progression through HDAC2-mediated suppression of thrombospondin-1. Oncogene 36, 1525–1536. doi: 10.1038/onc.2016.319
Ieguchi, K., Funakoshi, M., Mishima, T., Takizawa, K., Omori, T., Nakamura, F., et al. (2022). The sympathetic nervous system contributes to the establishment of pre-metastatic pulmonary microenvironments. Int. J. Mol. Sci. 23:10652. doi: 10.3390/ijms231810652
Iftikhar, A., Islam, M., Shepherd, S., Jones, S., and Ellis, I. (2021). Cancer and stress: does it make a difference to the patient when these two challenges collide? Cancers 13:163. doi: 10.3390/cancers13020163
Iñigo-Marco, I., and Alonso, M. M. (2019). Destress and do not suppress: targeting adrenergic signaling in tumor immunosuppression. J. Clin. Invest. 129, 5086–5088. doi: 10.1172/JCI133115
Irwin, M. R., Olmstead, R., Carrillo, C., Sadeghi, N., Nicassio, P., Ganz, P. A., et al. (2017). Tai Chi Chih compared with cognitive behavioral therapy for the treatment of insomnia in survivors of breast cancer: a randomized, partially blinded, noninferiority trial. J. Clin. Oncol. 35, 2656–2665. doi: 10.1200/JCO.2016.71.0285
Jabir, N. R., Firoz, C. K., Zughaibi, T. A., Alsaadi, M. A., Abuzenadah, A. M., Al-Asmari, A. I., et al. (2022). A literature perspective on the pharmacological applications of yohimbine. Ann. Med. 54, 2861–2875. doi: 10.1080/07853890.2022.2131330
Jacka, F. N., O'Neil, A., Opie, R., Itsiopoulos, C., Cotton, S., Mohebbi, M., et al. (2017). A randomised controlled trial of dietary improvement for adults with major depression (the 'SMILES' trial). BMC Med. 15:23. doi: 10.1186/s12916-017-0791-y
Jacob, L., Scholten, P. C., Kostev, K., and Kalder, M. (2018). Association between sleep disorders and the presence of breast cancer metastases in gynecological practices in Germany: a case-control study of 11,412 women. Breast Cancer Res. Treat. 171, 443–448. doi: 10.1007/s10549-018-4831-x
Jayachandran, P., Battaglin, F., Strelez, C., Lenz, A., Algaze, S., Soni, S., et al. (2023). Breast cancer and neurotransmitters: emerging insights on mechanisms and therapeutic directions. Oncogene 42, 627–637. doi: 10.1038/s41388-022-02584-4
Jeong, J. H., Park, H. J., Park, S. H., Choi, Y. H., and Chi, G. Y. (2022). β2-adrenergic receptor signaling pathway stimulates the migration and invasion of cancer cells via Src activation. Molecules 27:5940. doi: 10.3390/molecules27185940
Ji, H., Liu, N., Li, J., Chen, D., Luo, D., Sun, Q., et al. (2019). Oxytocin involves in chronic stress-evoked melanoma metastasis via β-arrestin 2-mediated ERK signaling pathway. Carcinogenesis 40, 1395–1404. doi: 10.1093/carcin/bgz064
Ji, L., Xu, F., Zhang, J., Song, T., Chen, W., Yin, X., et al. (2022). ADRB2 expression predicts the clinical outcomes and is associated with immune cells infiltration in lung adenocarcinoma. Sci. Rep. 12:15994. doi: 10.1038/s41598-022-19991-y
Jiang, J., Fu, Y., Tang, A., Gao, X., Zhang, D., Shen, Y., et al. (2023). Sex difference in prebiotics on gut and blood-brain barrier dysfunction underlying stress-induced anxiety and depression. CNS Neurosci. Ther. 29, 115–128. doi: 10.1111/cns.14091
Jiang, S. H., Hu, L. P., Wang, X., Li, J., and Zhang, Z. G. (2020). Neurotransmitters: emerging targets in cancer. Oncogene 39, 503–515. doi: 10.1038/s41388-019-1006-0
Jiang, W., Li, D., Han, R., Zhang, C., Jin, W. N., Wood, K., et al. (2017). Acetylcholine-producing NK cells attenuate CNS inflammation via modulation of infiltrating monocytes/macrophages. Proc. Natl. Acad. Sci. U. S. A. 114, E6202–e6211. doi: 10.1073/pnas.1705491114
Jiao, Y., Kang, G., Pan, P., Fan, H., Li, Q., Li, X., et al. (2023). Acetylcholine promotes chronic stress-induced lung adenocarcinoma progression via α5-nAChR/FHIT pathway. Cell. Mol. Life Sci. 80:119. doi: 10.1007/s00018-023-04742-7
Jin, M. Z., Gao, X. C., and Jin, W. L. (2023). Editorial: Cancer neuroscience: drug repurposing targeting the innervated niche. Front. Pharmacol. 14:1148706. doi: 10.3389/fphar.2023.1148706
Kamiya, A., Hayama, Y., Kato, S., Shimomura, A., Shimomura, T., Irie, K., et al. (2019). Genetic manipulation of autonomic nerve fiber innervation and activity and its effect on breast cancer progression. Nat. Neurosci. 22, 1289–1305. doi: 10.1038/s41593-019-0430-3
Kamiya, A., Hiyama, T., Fujimura, A., and Yoshikawa, S. (2021). Sympathetic and parasympathetic innervation in cancer: therapeutic implications. Clin. Auton. Res. 31, 165–178. doi: 10.1007/s10286-020-00724-y
Kanai, A., McNamara, K. M., Iwabuchi, E., Miki, Y., Onodera, Y., Guestini, F., et al. (2020). Significance of glucocorticoid signaling in triple-negative breast cancer patients: a newly revealed interaction with androgen signaling. Breast Cancer Res. Treat. 180, 97–110. doi: 10.1007/s10549-020-05523-7
Kang, Y., Nagaraja, A. S., Armaiz-Pena, G. N., Dorniak, P. L., Hu, W., Rupaimoole, R., et al. (2016). Adrenergic stimulation of DUSP1 impairs chemotherapy response in ovarian cancer. Clin. Cancer Res. 22, 1713–1724. doi: 10.1158/1078-0432.CCR-15-1275
Kawahara, T., Aljarah, A. K., Shareef, H. K., Inoue, S., Ide, H., Patterson, J. D., et al. (2016). Silodosin inhibits prostate cancer cell growth via ELK1 inactivation and enhances the cytotoxic activity of gemcitabine. Prostate 76, 744–756. doi: 10.1002/pros.23164
Kinkead, B., Schettler, P. J., Larson, E. R., Carroll, D., Sharenko, M., Nettles, J., et al. (2018). Massage therapy decreases cancer-related fatigue: results from a randomized early phase trial. Cancer 124, 546–554. doi: 10.1002/cncr.31064
Knochelmann, H. M., Dwyer, C. J., Bailey, S. R., Amaya, S. M., Elston, D. M., Mazza-McCrann, J. M., et al. (2018). When worlds collide: Th17 and Treg cells in cancer and autoimmunity. Cell. Mol. Immunol. 15, 458–469. doi: 10.1038/s41423-018-0004-4
Kruk, J., Aboul-Enein, B. H., Bernstein, J., and Gronostaj, M. (2019). Psychological stress and cellular aging in cancer: a meta-analysis. Oxidative Med. Cell. Longev. 2019:1270397. doi: 10.1155/2019/1270397
Kumar, A., Pramanik, J., Goyal, N., Chauhan, D., Sivamaruthi, B. S., Prajapati, B. G., et al. (2023). Gut microbiota in anxiety and depression: unveiling the relationships and management options. Pharmaceuticals 16:565. doi: 10.3390/ph16040565
Kwok, H. H., Gao, B., Chan, K. H., Ip, M. S., Minna, J. D., and Lam, D. C. (2021). Nicotinic acetylcholine receptor subunit α7 mediates cigarette smoke-induced PD-L1 expression in human bronchial epithelial cells. Cancers 13:5345. doi: 10.3390/cancers13215345
Lamboy-Caraballo, R., Ortiz-Sanchez, C., Acevedo-Santiago, A., Matta, J., Monteiro, A. N. A., and Armaiz-Pena, G. N. (2020). Norepinephrine-induced DNA damage in ovarian cancer cells. Int. J. Mol. Sci. 21:2250. doi: 10.3390/ijms21062250
Li, C., Cai, Q., Su, Z., Chen, Z., Cao, J., and Xu, F. (2023). Could peripheral 5-HT level be used as a biomarker for depression diagnosis and treatment? A narrative minireview. Front Pharmacol 14:1149511. doi: 10.3389/fphar.2023.1149511
Li, M., Chen, Z., Liu, Z., Zhang, N., Liu, J., Wang, H., et al. (2019). Twelve Chinese herbal preparations for the treatment of depression or depressive symptoms in cancer patients: a systematic review and meta-analysis of randomized controlled trials. BMC Complement. Altern. Med. 19:28. doi: 10.1186/s12906-019-2441-8
Li, Z., Lin, Y., Song, H., Qin, X., Yu, Z., Zhang, Z., et al. (2020). First small-molecule PROTACs for G protein-coupled receptors: inducing α (1A)-adrenergic receptor degradation. Acta Pharm. Sin. B 10, 1669–1679. doi: 10.1016/j.apsb.2020.01.014
Li, F., Niu, M., Qin, K., Guo, R., Yi, Y., Xu, J., et al. (2023). FBXL2 promotes E47 protein instability to inhibit breast cancer stemness and paclitaxel resistance. Oncogene 42, 339–350. doi: 10.1038/s41388-022-02559-5
Li, W., Wan, J., Chen, C., Zhou, C., Liao, P., Hu, Q., et al. (2022). Dissecting the role of cell signaling versus CD8(+) T cell modulation in propranolol antitumor activity. J. Mol. Med. 100, 1299–1306. doi: 10.1007/s00109-022-02238-8
Li, R. Q., Zhao, X. H., Zhu, Q., Liu, T., Hondermarck, H., Thorne, R. F., et al. (2023). Exploring neurotransmitters and their receptors for breast cancer prevention and treatment. Theranostics 13, 1109–1129. doi: 10.7150/thno.81403
Liang, Y., Li, H., Gan, Y., and Tu, H. (2021). Shedding light on the role of neurotransmitters in the microenvironment of pancreatic cancer. Front. Cell Dev. Biol. 9:688953. doi: 10.3389/fcell.2021.688953
Liao, P., Song, K., Zhu, Z., Liu, Z., Zhang, W., Li, W., et al. (2020). Propranolol suppresses the growth of colorectal cancer through simultaneously activating autologous CD8(+) T cells and inhibiting tumor AKT/MAPK pathway. Clin. Pharmacol. Ther. 108, 606–615. doi: 10.1002/cpt.1894
Lin, P. J., Altman, B. J., Gilmore, N. J., Loh, K. P., Dunne, R. F., Bautista, J., et al. (2023). Effect of yoga and mediational influence of fatigue on walking, physical activity, and quality of life among cancer survivors. J. Natl. Compr. Cancer Netw. 21, 153–162.e152. doi: 10.6004/jnccn.2022.7080
Liu, B., Liu, Z., Wang, Y., Lian, X., Han, Z., Cheng, X., et al. (2021). Overexpression of GINS4 is associated with poor prognosis and survival in glioma patients. Mol. Med. 27:117. doi: 10.1186/s10020-021-00378-0
Liu, S., Sun, Q., and Ren, X. (2023). Novel strategies for cancer immunotherapy: counter-immunoediting therapy. J. Hematol. Oncol. 16:38. doi: 10.1186/s13045-023-01430-8
Liu, H., Wang, C., Xie, N., Zhuang, Z., Liu, X., Hou, J., et al. (2018). Activation of adrenergic receptor β2 promotes tumor progression and epithelial mesenchymal transition in tongue squamous cell carcinoma. Int. J. Mol. Med. 41, 147–154. doi: 10.3892/ijmm.2017.3248
Liu, N., Wu, Y., Wen, X., Li, P., Lu, F., and Shang, H. (2021). Chronic stress promotes acute myeloid leukemia progression through HMGB1/NLRP3/IL-1β signaling pathway. J. Mol. Med. 99, 403–414. doi: 10.1007/s00109-020-02011-9
Liu, D., Xu, X., Dai, Y., Zhao, X., Bao, S., Ma, W., et al. (2021). Blockade of AIM2 inflammasome or α1-AR ameliorates IL-1β release and macrophage-mediated immunosuppression induced by CAR-T treatment. J. Immunother. Cancer 9:e001466. doi: 10.1136/jitc-2020-001466
Liu, C., Yang, Y., Chen, C., Li, L., Li, J., Wang, X., et al. (2021). Environmental eustress modulates β-ARs/CCL2 axis to induce anti-tumor immunity and sensitize immunotherapy against liver cancer in mice. Nat. Commun. 12:5725. doi: 10.1038/s41467-021-25967-9
Liu, Z., Zhang, W., Cheng, X., Wang, H., Bian, L., Wang, J., et al. (2021). Overexpressed XRCC2 as an independent risk factor for poor prognosis in glioma patients. Mol. Med. 27:52. doi: 10.1186/s10020-021-00316-0
Liu, Q., Zhang, R., Zhang, X., Liu, J., Wu, H., Li, Y., et al. (2021). Dopamine improves chemotherapeutic efficacy for pancreatic cancer by regulating macrophage-derived inflammations. Cancer Immunol. Immunother. 70, 2165–2177. doi: 10.1007/s00262-020-02816-0
Logotheti, S., Marquardt, S., Richter, C., Sophie Hain, R., Murr, N., Takan, I., et al. (2020). Neural networks recapitulation by Cancer cells promotes disease progression: a novel role of p73 isoforms in Cancer-neuronal crosstalk. Cancers 12:3789. doi: 10.3390/cancers12123789
Lorton, D., and Bellinger, D. L. (2015). Molecular mechanisms underlying β-adrenergic receptor-mediated cross-talk between sympathetic neurons and immune cells. Int. J. Mol. Sci. 16, 5635–5665. doi: 10.3390/ijms16035635
Lou, F., Long, H., Luo, S., Liu, Y., Pu, J., Wang, H., et al. (2023). Chronic restraint stress promotes the tumorigenic potential of oral squamous cell carcinoma cells by reprogramming fatty acid metabolism via CXCL3 mediated Wnt/β-catenin pathway. Exp. Neurol. 359:114268. doi: 10.1016/j.expneurol.2022.114268
Lu, Y., Zhang, Y., Zhao, H., Li, Q., Liu, Y., Zuo, Y., et al. (2022). Chronic stress model simulated by salbutamol promotes tumorigenesis of gastric cancer cells through β2-AR/ERK/EMT pathway. J. Cancer 13, 401–412. doi: 10.7150/jca.65403
Lu, Y., Zhao, H., Liu, Y., Zuo, Y., Xu, Q., Liu, L., et al. (2021). Chronic stress activates PlexinA1/VEGFR2-JAK2-STAT3 in vascular endothelial cells to promote angiogenesis. Front. Oncol. 11:709057. doi: 10.3389/fonc.2021.709057
MacDonald, C. R., Bucsek, M. J., Qiao, G., Chen, M., Evans, L., Greenberg, D. J., et al. (2019). Adrenergic receptor signaling regulates the response of tumors to ionizing radiation. Radiat. Res. 191, 585–589. doi: 10.1667/RR15193.1
Magnon, C., and Hondermarck, H. (2023). The neural addiction of cancer. Nat. Rev. Cancer 23, 317–334. doi: 10.1038/s41568-023-00556-8
Mantovani, A., Allavena, P., Marchesi, F., and Garlanda, C. (2022). Macrophages as tools and targets in cancer therapy. Nat. Rev. Drug Discov. 21, 799–820. doi: 10.1038/s41573-022-00520-5
March, B., Faulkner, S., Jobling, P., Steigler, A., Blatt, A., Denham, J., et al. (2020). Tumour innervation and neurosignalling in prostate cancer. Nat. Rev. Urol. 17, 119–130. doi: 10.1038/s41585-019-0274-3
Marker, R. J., Cox-Martin, E., Jankowski, C. M., Purcell, W. T., and Peters, J. C. (2018). Evaluation of the effects of a clinically implemented exercise program on physical fitness, fatigue, and depression in cancer survivors. Support Care Cancer 26, 1861–1869. doi: 10.1007/s00520-017-4019-7
Märkl, F., Huynh, D., Endres, S., and Kobold, S. (2022). Utilizing chemokines in cancer immunotherapy. Trends Cancer 8, 670–682. doi: 10.1016/j.trecan.2022.04.001
Matzner, P., Sandbank, E., Neeman, E., Zmora, O., Gottumukkala, V., and Ben-Eliyahu, S. (2020). Harnessing cancer immunotherapy during the unexploited immediate perioperative period. Nat. Rev. Clin. Oncol. 17, 313–326. doi: 10.1038/s41571-019-0319-9
McEwen, K., and Boyd, C. M. (2018). A measure of team resilience: developing the resilience at work team scale. J. Occup. Environ. Med. 60, 258–272. doi: 10.1097/JOM.0000000000001223
McGettigan, M., Cardwell, C. R., Cantwell, M. M., and Tully, M. A. (2020). Physical activity interventions for disease-related physical and mental health during and following treatment in people with non-advanced colorectal cancer. Cochrane Database Syst. Rev. 5:Cd012864. doi: 10.1002/14651858.CD012864.pub2
McIlvried, L. A., Atherton, M. A., Horan, N. L., Goch, T. N., and Scheff, N. N. (2022). Sensory neurotransmitter calcitonin gene-related peptide modulates tumor growth and lymphocyte infiltration in Oral squamous cell carcinoma. Adv Biol 6:e2200019. doi: 10.1002/adbi.202200019
Mihalopoulos, M., Dovey, Z., Archer, M., Korn, T. G., Okhawere, K. E., Nkemdirim, W., et al. (2020). Repurposing of α1-adrenoceptor antagonists: impact in renal cancer. Cancers 12:2442. doi: 10.3390/cancers12092442
Mitre-Aguilar, I. B., Moreno-Mitre, D., Melendez-Zajgla, J., Maldonado, V., Jacobo-Herrera, N. J., Ramirez-Gonzalez, V., et al. (2022). The role of glucocorticoids in breast cancer therapy. Curr. Oncol. 30, 298–314. doi: 10.3390/curroncol30010024
Mohammadpour, H., MacDonald, C. R., McCarthy, P. L., Abrams, S. I., and Repasky, E. A. (2021). β2-adrenergic receptor signaling regulates metabolic pathways critical to myeloid-derived suppressor cell function within the TME. Cell Rep. 37:109883. doi: 10.1016/j.celrep.2021.109883
Mohammadpour, H., MacDonald, C. R., Qiao, G., Chen, M., Dong, B., Hylander, B. L., et al. (2019). β2 adrenergic receptor-mediated signaling regulates the immunosuppressive potential of myeloid-derived suppressor cells. J. Clin. Invest. 129, 5537–5552. doi: 10.1172/JCI129502
Montoya, A., Varela-Ramirez, A., Dickerson, E., Pasquier, E., Torabi, A., Aguilera, R., et al. (2019). The beta adrenergic receptor antagonist propranolol alters mitogenic and apoptotic signaling in late stage breast cancer. Biom. J. 42, 155–165. doi: 10.1016/j.bj.2019.02.003
Mravec, B., Horvathova, L., and Hunakova, L. (2020). Neurobiology of cancer: the role of β-adrenergic receptor signaling in various tumor environments. Int. J. Mol. Sci. 21, 1–24. doi: 10.3390/ijms21217958
Muqbil, I., Fatima, S., Azmi, A. S., Alsharidah, A. S., Khan, S. A., Aljaser, F., et al. (2020). Restraint stress abates the antioxidant potential of melatonin on dimethyl benz (a) anthracene (DMBA) induced carcinogenesis. Med. Oncol. 37:96. doi: 10.1007/s12032-020-01422-5
Muthuswamy, R., Okada, N. J., Jenkins, F. J., McGuire, K., McAuliffe, P. F., Zeh, H. J., et al. (2017). Epinephrine promotes COX-2-dependent immune suppression in myeloid cells and cancer tissues. Brain Behav. Immun. 62, 78–86. doi: 10.1016/j.bbi.2017.02.008
Myers, J. A., and Miller, J. S. (2021). Exploring the NK cell platform for cancer immunotherapy. Nat. Rev. Clin. Oncol. 18, 85–100. doi: 10.1038/s41571-020-0426-7
Nagaraja, A. S., Dood, R. L., Armaiz-Pena, G., Kang, Y., Wu, S. Y., Allen, J. K., et al. (2021). Adrenergic-mediated increases in INHBA drive CAF phenotype and collagens. JCI Insight 6:e93076. doi: 10.1172/jci.insight.149895
Nagata, Y., Kawahara, T., Goto, T., Inoue, S., Teramoto, Y., Jiang, G., et al. (2020). Effects of α(1)-adrenergic receptor antagonists on the development and progression of urothelial cancer. Am. J. Cancer Res. 10, 4386–4398.
Neeman, E., and Ben-Eliyahu, S. (2013). Surgery and stress promote cancer metastasis: new outlooks on perioperative mediating mechanisms and immune involvement. Brain Behav. Immun. 30, S32–S40. doi: 10.1016/j.bbi.2012.03.006
Nilsson, M. B., Le, X., and Heymach, J. V. (2020). β-Adrenergic signaling in lung cancer: a potential role for beta-blockers. J. Neuroimmune Pharmacol. 15, 27–36. doi: 10.1007/s11481-019-09891-w
Nilsson, M. B., Sun, H., Diao, L., Tong, P., Liu, D., Li, L., et al. (2017). Stress hormones promote EGFR inhibitor resistance in NSCLC: implications for combinations with β-blockers. Sci. Transl. Med. 9:eaao4307. doi: 10.1126/scitranslmed.aao4307
Nissen, M. D., Sloan, E. K., and Mattarollo, S. R. (2018). β-Adrenergic signaling impairs antitumor CD8(+) T-cell responses to B-cell lymphoma immunotherapy. Cancer Immunol. Res. 6, 98–109. doi: 10.1158/2326-6066.CIR-17-0401
Niu, M., Xu, J., Liu, Y., Li, Y., He, T., Ding, L., et al. (2021). FBXL2 counteracts Grp94 to destabilize EGFR and inhibit EGFR-driven NSCLC growth. Nat. Commun. 12:5919. doi: 10.1038/s41467-021-26222-x
Paijens, S. T., Vledder, A., de Bruyn, M., and Nijman, H. W. (2021). Tumor-infiltrating lymphocytes in the immunotherapy era. Cell. Mol. Immunol. 18, 842–859. doi: 10.1038/s41423-020-00565-9
Partecke, L. I., Speerforck, S., Käding, A., Seubert, F., Kühn, S., Lorenz, E., et al. (2016). Chronic stress increases experimental pancreatic cancer growth, reduces survival and can be antagonised by beta-adrenergic receptor blockade. Pancreatology 16, 423–433. doi: 10.1016/j.pan.2016.03.005
Qiao, G., Bucsek, M. J., Winder, N. M., Chen, M., Giridharan, T., Olejniczak, S. H., et al. (2019). β-Adrenergic signaling blocks murine CD8(+) T-cell metabolic reprogramming during activation: a mechanism for immunosuppression by adrenergic stress. Cancer Immunol. Immunother. 68, 11–22. doi: 10.1007/s00262-018-2243-8
Qiao, G., Chen, M., Mohammadpour, H., MacDonald, C. R., Bucsek, M. J., Hylander, B. L., et al. (2021). Chronic adrenergic stress contributes to metabolic dysfunction and an exhausted phenotype in T cells in the tumor microenvironment. Cancer Immunol. Res. 9, 651–664. doi: 10.1158/2326-6066.CIR-20-0445
Qin, X., Li, J., Wang, S., Lv, J., Luan, F., Liu, Y., et al. (2021). Serotonin/HTR1E signaling blocks chronic stress-promoted progression of ovarian cancer. Theranostics 11, 6950–6965. doi: 10.7150/thno.58956
Rabben, H. L., Zhao, C. M., Hayakawa, Y., Wang, T. C., and Chen, D. (2016). Vagotomy and gastric tumorigenesis. Curr. Neuropharmacol. 14, 967–972. doi: 10.2174/1570159X14666160121114854
Radford-Smith, D. E., and Anthony, D. C. (2023). Prebiotic and probiotic modulation of the microbiota-gut-brain Axis in depression. Nutrients 15:1880. doi: 10.3390/nu15081880
Restaino, A. C., Walz, A., Vermeer, S. J., Barr, J., Kovács, A., Fettig, R. R., et al. (2023). Functional neuronal circuits promote disease progression in cancer. Sci. Adv. 9:eade4443. doi: 10.1126/sciadv.ade4443
Ricon, I., Hanalis-Miller, T., Haldar, R., Jacoby, R., and Ben-Eliyahu, S. (2019). Perioperative biobehavioral interventions to prevent cancer recurrence through combined inhibition of β-adrenergic and cyclooxygenase 2 signaling. Cancer 125, 45–56. doi: 10.1002/cncr.31594
Rimal, R., Desai, P., Daware, R., Hosseinnejad, A., Prakash, J., Lammers, T., et al. (2022). Cancer-associated fibroblasts: origin, function, imaging, and therapeutic targeting. Adv. Drug Deliv. Rev. 189:114504. doi: 10.1016/j.addr.2022.114504
Rousseau, B., Murugan, S., Palagani, A., and Sarkar, D. K. (2022). Beta 2 adrenergic receptor and mu opioid receptor interact to potentiate the aggressiveness of human breast cancer cell by activating the glycogen synthase kinase 3 signaling. Breast Cancer Res. 24:33. doi: 10.1186/s13058-022-01526-y
Ruan, W., Yuan, X., and Eltzschig, H. K. (2021). Circadian rhythm as a therapeutic target. Nat. Rev. Drug Discov. 20, 287–307. doi: 10.1038/s41573-020-00109-w
Rudak, P. T., Choi, J., Parkins, K. M., Summers, K. L., Jackson, D. N., Foster, P. J., et al. (2021). Chronic stress physically spares but functionally impairs innate-like invariant T cells. Cell Rep. 35:108979. doi: 10.1016/j.celrep.2021.108979
Rudak, P. T., Gangireddy, R., Choi, J., Burhan, A. M., Summers, K. L., Jackson, D. N., et al. (2019). Stress-elicited glucocorticoid receptor signaling upregulates TIGIT in innate-like invariant T lymphocytes. Brain Behav. Immun. 80, 793–804. doi: 10.1016/j.bbi.2019.05.027
Russell, G., and Lightman, S. (2019). The human stress response. Nat. Rev. Endocrinol. 15, 525–534. doi: 10.1038/s41574-019-0228-0
Schuster, C., Akslen, L. A., and Straume, O. (2023). β2-adrenergic receptor expression in patients receiving bevacizumab therapy for metastatic melanoma. Cancer Med. 12, 17891–17900. doi: 10.1002/cam4.6424
Shaashua, L., Shabat-Simon, M., Haldar, R., Matzner, P., Zmora, O., Shabtai, M., et al. (2017). Perioperative COX-2 and β-adrenergic blockade improves metastatic biomarkers in breast cancer patients in a phase-II randomized trial. Clin. Cancer Res. 23, 4651–4661. doi: 10.1158/1078-0432.CCR-17-0152
Shahid, A., Chen, M., Lin, C., Andresen, B. T., Parsa, C., Orlando, R., et al. (2023). The β-blocker carvedilol prevents benzo(a)pyrene-induced lung toxicity, inflammation and carcinogenesis. Cancers 15:583. doi: 10.3390/cancers15030583
Shan, T., Cui, X., Li, W., Lin, W., Li, Y., Chen, X., et al. (2014). Novel regulatory program for norepinephrine-induced epithelial-mesenchymal transition in gastric adenocarcinoma cell lines. Cancer Sci. 105, 847–856. doi: 10.1111/cas.12438
Shimizu, T., Yamaguchi, K., Yamamoto, M., Kurioka, R., Kino, Y., Matsunaga, W., et al. (2020). Identification of HUHS190, a human naftopidil metabolite, as a novel anti-bladder cancer drug. Bioorg. Med. Chem. Lett. 30:126744. doi: 10.1016/j.bmcl.2019.126744
Silva, D., Kacprzak, K., Quintas, C., Gonçalves, J., and Fresco, P. (2023). Activation of β-adrenoceptors promotes lipid droplet accumulation in MCF-7 breast cancer cells via cAMP/PKA/EPAC pathways. Int. J. Mol. Sci. 24:767. doi: 10.3390/ijms24010767
Singh, A., and Ranjan, A. (2023). Adrenergic receptor signaling regulates the CD40-receptor mediated anti-tumor immunity. Front. Immunol. 14:1141712. doi: 10.3389/fimmu.2023.1141712
Sommershof, A., Scheuermann, L., Koerner, J., and Groettrup, M. (2017). Chronic stress suppresses anti-tumor T(CD8+) responses and tumor regression following cancer immunotherapy in a mouse model of melanoma. Brain Behav. Immun. 65, 140–149. doi: 10.1016/j.bbi.2017.04.021
Sorski, L., Melamed, R., Matzner, P., Lavon, H., Shaashua, L., Rosenne, E., et al. (2016). Reducing liver metastases of colon cancer in the context of extensive and minor surgeries through β-adrenoceptors blockade and COX2 inhibition. Brain Behav. Immun. 58, 91–98. doi: 10.1016/j.bbi.2016.05.017
Springer, J., Jové, Q., de Lima Junior, E. A., Ladrón, N., López-Soriano, F. J., Busquets, S., et al. (2023). Effects of S-pindolol in mouse pancreatic and lung cancer cachexia models. J. Cachexia. Sarcopenia Muscle 14, 1244–1248. doi: 10.1002/jcsm.13249
Stone, L. (2018). A nervous disposition: the angiometabolic switch. Nat. Rev. Urol. 15:2. doi: 10.1038/nrurol.2017.200
Sullivan, S., Young, A., Garcia, M., Almuwaqqat, Z., Moazzami, K., Hammadah, M., et al. (2023). Sex differences in vascular response to mental stress and adverse cardiovascular events among patients with ischemic heart disease. Arterioscler. Thromb. Vasc. Biol. 43, e112–e120. doi: 10.1161/ATVBAHA.122.318576
Sun, X., Yang, S., and Song, W. (2020). Prazosin inhibits the proliferation and survival of acute myeloid leukaemia cells through down-regulating TNS1. Biomed. Pharmacother. 124:109731. doi: 10.1016/j.biopha.2019.109731
Suzuki, N., Niikura, R., Ihara, S., Hikiba, Y., Kinoshita, H., Higashishima, N., et al. (2019). Alpha-blockers as colorectal cancer chemopreventive: findings from a case-control study, human cell cultures, and in vivo preclinical testing. Cancer Prev. Res. 12, 185–194. doi: 10.1158/1940-6207.CAPR-18-0288
Tack, L., Lefebvre, T., Lycke, M., Langenaeken, C., Fontaine, C., Borms, M., et al. (2021). A randomised wait-list controlled trial to evaluate emotional freedom techniques for self-reported cancer-related cognitive impairment in cancer survivors (EMOTICON). EClinicalMedicine 39:101081. doi: 10.1016/j.eclinm.2021.101081
Tack, L., Schofield, P., Boterberg, T., Chandler, R., Parris, C. N., and Debruyne, P. R. (2022). Psychosocial care after cancer diagnosis: recent advances and challenges. Cancers 14:5882. doi: 10.3390/cancers14235882
Thapa, S., and Cao, X. (2023). Nervous regulation: beta-2-adrenergic signaling in immune homeostasis, cancer immunotherapy, and autoimmune diseases. Cancer Immunol. Immunother. 72, 2549–2556. doi: 10.1007/s00262-023-03445-z
Tian, W., Liu, Y., Cao, C., Zeng, Y., Pan, Y., Liu, X., et al. (2021). Chronic stress: impacts on tumor microenvironment and implications for anti-Cancer treatments. Front. Cell Dev. Biol. 9:777018. doi: 10.3389/fcell.2021.777018
Vaes, N., Idris, M., Boesmans, W., Alves, M. M., and Melotte, V. (2022). Nerves in gastrointestinal cancer: from mechanism to modulations. Nat. Rev. Gastroenterol. Hepatol. 19, 768–784. doi: 10.1038/s41575-022-00669-9
Valente, V. B., de Melo Cardoso, D., Kayahara, G. M., Nunes, G. B., Tjioe, K. C., Biasoli, E. R., et al. (2021). Stress hormones promote DNA damage in human oral keratinocytes. Sci. Rep. 11:19701. doi: 10.1038/s41598-021-99224-w
Van Blarigan, E. L., Ma, C., Ou, F. S., Bainter, T. M., Venook, A. P., Ng, K., et al. (2023). Dietary fat in relation to all-cause mortality and cancer progression and death among people with metastatic colorectal cancer: data from CALGB 80405 (Alliance)/SWOG 80405. Int. J. Cancer 152, 123–136. doi: 10.1002/ijc.34230
van der Heijden, C., Groh, L., Keating, S. T., Kaffa, C., Noz, M. P., Kersten, S., et al. (2020). Catecholamines induce trained immunity in monocytes in vitro and in vivo. Circ. Res. 127, 269–283. doi: 10.1161/CIRCRESAHA.119.315800
Vignjević Petrinović, S., Milošević, M. S., Marković, D., and Momčilović, S. (2023). Interplay between stress and cancer—a focus on inflammation. Front. Physiol. 14:1119095. doi: 10.3389/fphys.2023.1119095
von Trott, P., Oei, S. L., and Ramsenthaler, C. (2020). Acupuncture for breathlessness in advanced diseases: a systematic review and meta-analysis. J. Pain Symptom Manag. 59, 327–338.e323. doi: 10.1016/j.jpainsymman.2019.09.007
Wang, Z., Liu, W., Wang, C., Li, Y., and Ai, Z. (2020). Acetylcholine promotes the self-renewal and immune escape of CD133+ thyroid cancer cells through activation of CD133-Akt pathway. Cancer Lett. 471, 116–124. doi: 10.1016/j.canlet.2019.12.009
Wang, C., Shen, Y., Ni, J., Hu, W., and Yang, Y. (2022). Effect of chronic stress on tumorigenesis and development. Cell. Mol. Life Sci. 79:485. doi: 10.1007/s00018-022-04455-3
Wieduwild, E., Girard-Madoux, M. J., Quatrini, L., Laprie, C., Chasson, L., Rossignol, R., et al. (2020). β2-adrenergic signals downregulate the innate immune response and reduce host resistance to viral infection. J. Exp. Med. 217:e20190554. doi: 10.1084/jem.20190554
Winkler, F., Venkatesh, H. S., Amit, M., Batchelor, T., Demir, I. E., Deneen, B., et al. (2023). Cancer neuroscience: state of the field, emerging directions. Cells 186, 1689–1707. doi: 10.1016/j.cell.2023.02.002
Wu, Y., Luo, X., Zhou, Q., Gong, H., Gao, H., Liu, T., et al. (2022a). The disbalance of LRP1 and SIRPα by psychological stress dampens the clearance of tumor cells by macrophages. Acta Pharm. Sin. B 12, 197–209. doi: 10.1016/j.apsb.2021.06.002
Wu, Y., Zhou, L., Zhang, X., Yang, X., Niedermann, G., and Xue, J. (2022b). Psychological distress and eustress in cancer and cancer treatment: advances and perspectives. Sci. Adv. 8:eabq7982. doi: 10.1126/sciadv.abq7982
Xiao, L., Li, X., Fang, C., Yu, J., and Chen, T. (2023). Neurotransmitters: promising immune modulators in the tumor microenvironment. Front. Immunol. 14:1118637. doi: 10.3389/fimmu.2023.1118637
Xie, J., Wang, X., Ge, H., Peng, F., Zheng, N., Wang, Q., et al. (2019). Cx32 mediates norepinephrine-promoted EGFR-TKI resistance in a gap junction-independent manner in non-small-cell lung cancer. J. Cell. Physiol. 234, 23146–23159. doi: 10.1002/jcp.28881
Xing, D., Li, L., Meng, D., Zhang, Y., and Ma, F. (2023). Anti-cell proliferative mechanism of doxazosin on human oral cancer cells through the modulation of antioxidant and apoptotic pathway. Appl. Biochem. Biotechnol. 195, 6824–6839. doi: 10.1007/s12010-023-04412-1
Yan, J., Chen, Y., Luo, M., Hu, X., Li, H., Liu, Q., et al. (2023). Chronic stress in solid tumor development: from mechanisms to interventions. J. Biomed. Sci. 30:8. doi: 10.1186/s12929-023-00903-9
Yang, Z., Li, Z., Guo, Z., Ren, Y., Zhou, T., Xiao, Z., et al. (2021). Antitumor effect of fluoxetine on chronic stress-promoted lung cancer growth via suppressing kynurenine pathway and enhancing cellular immunity. Front. Pharmacol. 12:685898. doi: 10.3389/fphar.2021.685898
Yang, W., Sui, J., Ma, Y., Simon, T. G., Petrick, J. L., Lai, M., et al. (2020). High dietary intake of vegetable or polyunsaturated fats is associated with reduced risk of hepatocellular carcinoma. Clin. Gastroenterol. Hepatol. 18, 2775–2783.e2711. doi: 10.1016/j.cgh.2020.01.003
Yang, H., Xia, L., Chen, J., Zhang, S., Martin, V., Li, Q., et al. (2019). Stress-glucocorticoid-TSC22D3 axis compromises therapy-induced antitumor immunity. Nat. Med. 25, 1428–1441. doi: 10.1038/s41591-019-0566-4
Yuan, L., Springer, J., Palus, S., Busquets, S., Jové, Q., de Lima, A., et al. (2023). The atypical β-blocker S-oxprenolol reduces cachexia and improves survival in a rat cancer cachexia model. J. Cachexia. Sarcopenia Muscle 14, 653–660. doi: 10.1002/jcsm.13116
Zahalka, A. H., Arnal-Estapé, A., Maryanovich, M., Nakahara, F., Cruz, C. D., Finley, L. W. S., et al. (2017). Adrenergic nerves activate an angio-metabolic switch in prostate cancer. Science 358, 321–326. doi: 10.1126/science.aah5072
Zenga, J., Awan, M. J., Frei, A., Petrie, E., Sharma, G. P., Shreenivas, A., et al. (2022). Chronic stress promotes an immunologic inflammatory state and head and neck cancer growth in a humanized murine model. Head Neck 44, 1324–1334. doi: 10.1002/hed.27028
Zhang, Y., Lv, N., Li, M., Liu, M., and Wu, C. (2023). Cancer-associated fibroblasts: tumor defenders in radiation therapy. Cell Death Dis. 14:541. doi: 10.1038/s41419-023-06060-z
Zhang, B., Ma, S., Rachmin, I., He, M., Baral, P., Choi, S., et al. (2020). Hyperactivation of sympathetic nerves drives depletion of melanocyte stem cells. Nature 577, 676–681. doi: 10.1038/s41586-020-1935-3
Zhang, L., Pan, J., Chen, W., Jiang, J., and Huang, J. (2020). Chronic stress-induced immune dysregulation in cancer: implications for initiation, progression, metastasis, and treatment. Am. J. Cancer Res. 10, 1294–1307.
Zhang, Z., Shao, S., Zhang, Y., Jia, R., Hu, X., Liu, H., et al. (2020). Xiaoyaosan slows cancer progression and ameliorates gut dysbiosis in mice with chronic restraint stress and colorectal cancer xenografts. Biomed. Pharmacother. 132:110916. doi: 10.1016/j.biopha.2020.110916
Zhang, B., Vogelzang, A., Miyajima, M., Sugiura, Y., Wu, Y., Chamoto, K., et al. (2021). B cell-derived GABA elicits IL-10+ macrophages to limit anti-tumour immunity. Nature 599, 471–476. doi: 10.1038/s41586-021-04082-1
Zhang, J., Wang, N., Zheng, Y., Yang, B., Wang, S., Wang, X., et al. (2023). Naringenin in Si-Ni-San formula inhibits chronic psychological stress-induced breast cancer growth and metastasis by modulating estrogen metabolism through FXR/EST pathway. J. Adv. Res. 47, 189–207. doi: 10.1016/j.jare.2022.06.006
Zhang, Y., Zanos, P., Jackson, I. L., Zhang, X., Zhu, X., Gould, T., et al. (2020). Psychological stress enhances tumor growth and diminishes radiation response in preclinical model of lung cancer. Radiother. Oncol. 146, 126–135. doi: 10.1016/j.radonc.2020.02.004
Zhang, X., Zhang, Y., He, Z., Yin, K., Li, B., Zhang, L., et al. (2019). Chronic stress promotes gastric cancer progression and metastasis: an essential role for ADRB2. Cell Death Dis. 10:788. doi: 10.1038/s41419-019-2030-2
Zhang, Q. B., Zhang, B. H., Zhang, K. Z., Meng, X. T., Jia, Q. A., Zhang, Q. B., et al. (2016). Moderate swimming suppressed the growth and metastasis of the transplanted liver cancer in mice model: with reference to nervous system. Oncogene 35, 4122–4131. doi: 10.1038/onc.2015.484
Zhao, C. M., Hayakawa, Y., Kodama, Y., Muthupalani, S., Westphalen, C. B., Andersen, G. T., et al. (2014). Denervation suppresses gastric tumorigenesis. Sci. Transl. Med. 6:250ra115. doi: 10.1126/scitranslmed.3009569
Zhao, Y., Jia, Y., Shi, T., Wang, W., Shao, D., Zheng, X., et al. (2019). Depression promotes hepatocellular carcinoma progression through a glucocorticoid-mediated upregulation of PD-1 expression in tumor-infiltrating NK cells. Carcinogenesis 40, 1132–1141. doi: 10.1093/carcin/bgz017
Zheng, Y., Wang, N., Wang, S., Zhang, J., Yang, B., and Wang, Z. (2023). Chronic psychological stress promotes breast cancer pre-metastatic niche formation by mobilizing splenic MDSCs via TAM/CXCL1 signaling. J. Exp. Clin. Cancer Res. 42:129. doi: 10.1186/s13046-023-02696-z
Zhong, W., Zhang, F., Huang, C., Lin, Y., and Huang, J. (2021). Classification of clear cell renal cell carcinoma based on tumor suppressor genomic profiling. J. Cancer 12, 2359–2370. doi: 10.7150/jca.50462
Zhou, J., Liu, Z., Zhang, L., Hu, X., Wang, Z., Ni, H., et al. (2020). Activation of β2-adrenergic receptor promotes growth and angiogenesis in breast cancer by down-regulating PPARγ. Cancer Res. Treat. 52, 830–847. doi: 10.4143/crt.2019.510
Keywords: chronic stress, tumor microenvironment, immunotherapy, anticancer treatments, sympathetic nervous system, neural-immune interactions, HPA axis, personalized medicine
Citation: Lei Y, Liao F, Tian Y, Wang Y, Xia F and Wang J (2023) Investigating the crosstalk between chronic stress and immune cells: implications for enhanced cancer therapy. Front. Neurosci. 17:1321176. doi: 10.3389/fnins.2023.1321176
Edited by:
Shuai Wang, New York University, United StatesReviewed by:
Rui Wang, The First Affiliated Hospital of Xi'an Jiaotong University, ChinaTengfei Huang, University of Pittsburgh, United States
Copyright © 2023 Lei, Liao, Tian, Wang, Xia and Wang. This is an open-access article distributed under the terms of the Creative Commons Attribution License (CC BY). The use, distribution or reproduction in other forums is permitted, provided the original author(s) and the copyright owner(s) are credited and that the original publication in this journal is cited, in accordance with accepted academic practice. No use, distribution or reproduction is permitted which does not comply with these terms.
*Correspondence: JianHua Wang, d2poQGNxdS5lZHUuY24=; Feng Xia, ZnJhbmtmeGlhQDE2My5jb20=