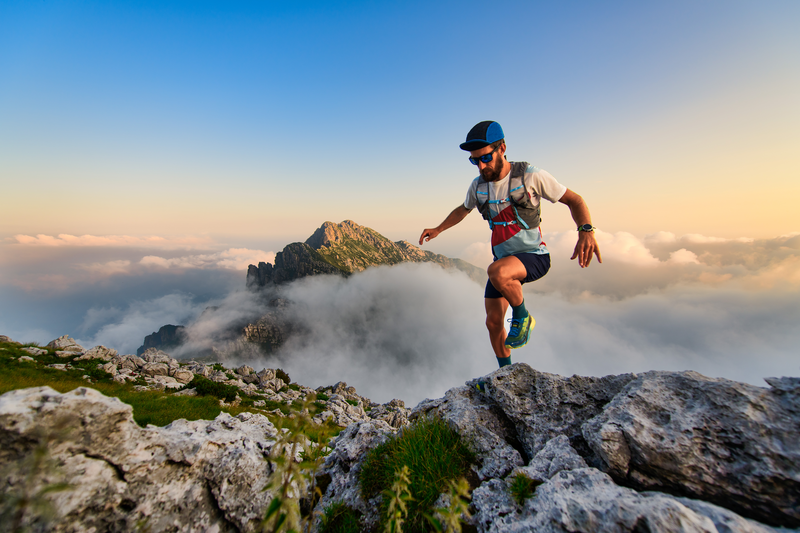
95% of researchers rate our articles as excellent or good
Learn more about the work of our research integrity team to safeguard the quality of each article we publish.
Find out more
EDITORIAL article
Front. Neurosci. , 13 October 2023
Sec. Neural Technology
Volume 17 - 2023 | https://doi.org/10.3389/fnins.2023.1303574
This article is part of the Research Topic Invertebrate Neurophysiology - of Currents, Cells, and Circuits View all 15 articles
Editorial on the Research Topic
Invertebrate neurophysiology—of currents, cells, and circuits
Exploring the intricacies of the brain and comprehending how it gives rise to behavior, still stands as one of the foremost challenges for the scientific community. To investigate the functioning of the nervous system directly, researchers have often employed electrophysiological techniques. Nonetheless, the complexity and sheer abundance of neurons, along with their myriad connections, present significant obstacles. Consequently, scientists have turned to the study of simpler organisms with intricate behavioral patterns but a substantially lower number of neurons. Thus, over the years, invertebrates have been extensively employed in neuroscience research owing to their relative simplicity, accessibility, and lower ethical concerns compared to vertebrate models. Their significant contributions and pioneering role in advancing our understanding of neuroscience are evident through the numerous discoveries made using these organisms. As a result, the central neural circuits of worms, molluscs, insects, and crustaceans were characterized well before similar findings emerged from vertebrate preparations, highlighting the extensive and valuable history of invertebrate research in neuroscience (Clarac and Pearlstein, 2007). These ground-breaking achievements have unraveled numerous fundamental mechanisms underlying neuronal function and this Research Topic comprising nine original research articles and four reviews, emphasizes the continuing breakthroughs made by researchers investigating invertebrates like molluscs, insects, crustaceans, and others.
Invertebrate nervous systems offer several advantages over their vertebrate counterparts for trying to investigate and understand a range of different neurobiological phenomena. These advantages include large, individually identifiable neurons in some species, such as molluscs (Croll, 1987; Bullock, 2000). These relatively large neuronal somata facilitate intracellular electrophysiology, dynamic clamp, cell isolation and culture, DNA/RNA sequencing of individual neurons, and much more (Katz and Quinlan, 2019). In this Research Topic, Zhuo et al. review many of these techniques used in the infamous Aplysia californica nervous system, including focused ultrasound, optical recording and stimulation, and especially the more recent infrared neural inhibition, spearheaded by these authors over the last decade. Also in this topic, Lee and Watson use a molluscan nervous system to better understand the neural mechanisms underlying modulation of feeding behavior in the nudibranch, Melibe leonina. This work on feeding complements prior work in this species, on identified neurons involved in locomotion (Thompson and Watson, 2005; Sakurai et al., 2014).
Having access to identifiable neurons has facilitated the study of central pattern generators (CPGs) in numerous invertebrates, including crayfish swimmerets, feeding and swimming in gastropods, the leech heartbeat and swimming, the stomatogastric ganglion of crustaceans, and walking in stick insects (reviewed in Marder et al., 2005; Selverston, 2010). In this topic, Pirtle reviews over four decades of research on the CPG controlling locomotion in the pteropod Clione limacina, particularly mechanisms that contribute to acceleration of swimming, which is achieved through neuromodulation of the swim CPG. Blitz reviews neuromodulation in a number of invertebrate CPGs, especially the circuits in the crustacean stomatogastric ganglion that are responsible for regulating gastric activity. Both of these reviews highlight many of the neuromodulatory principles that have been learned from these invertebrate preparations, including co-transmitters, the roles of neuromodulatory projection neurons, state dependence, the role of sensory feedback, communication between CPG circuits, and many others.
Another advantage to invertebrates for unraveling elements of the nervous system, is that invertebrate taxa cover a far wider phylogenetic spread than vertebrates. Therefore, comparing the nervous systems of phylogenetically disparate invertebrates can be very informative in learning about conserved neurobiological principles and evolution of nervous systems. In this topic, there are articles on a wide array of bilaterian invertebrates, including ecdysozoans (Arthropoda—Au et al.; Cillov and Stumpner; Au et al.; Blitz; Megwa et al.; Powell et al.) and lophotrochozoans (Mollusca—Lee and Watson; Pirtle; Zhuo et al.; Gribkova et al.). However, research on cellular signaling and neurons in metazoans that diverged from bilaterians can provide additional understanding about the evolution of nervous systems. Moroz et al. and Norekian and Moroz investigate the use of various peptidergic and nitrergic signaling compounds in ctenophores, which may have independently evolved a nervous “system” (Moroz et al., 2014; Moroz and Kohn, 2016; Burkhardt et al., 2023). Going even further afield in the metazoan clade, Nikitin et al. report on the use of amino acids in nerveless placozoans, to integrate various behaviors, such as feeding and locomotion. Ultimately, one of the goals of this type of research in these invertebrate systems is to determine if certain principles can be applied to other organisms, such as mammals. Here, Gribkova et al. attempt to do just that, pointing out similar parallels in modular arrangement of nervous systems between soft-bodied invertebrates and vertebrates. These similarities in arrangement suggest that continued work in these simpler circuits may shed interesting light on vertebrate nervous systems.
Insect preparations with their simple nervous systems with well-defined neural circuits have been instrumental to study the neural basis of complex behaviors observed in their natural environments, providing valuable insights into general principles of neural processing and decision-making (e.g., Menzel, 2012). For example, crickets, bush crickets, and grasshoppers exhibit highly specialized auditory sensory systems and central circuits, allowing them to engage in acoustic communication (Huber et al., 1989). Their relatively larger and fewer identifiable neurons facilitate the consistent identification of individual homologous neurons across different animals and species. Exploiting this unique advantage, Cillov and Stumpner review and describe some novel elements in local prothoracic auditory neurons, investigating the evolution of nervous systems and deepening our understanding of their development and function.
The circadian clock is another well-researched behavior in insects, and the fruit fly Drosophila melanogaster has played a crucial role in understanding the molecular clock over the past five decades since the discovery of the first clock mutant (Konopka and Benzer, 1971), as recognized by the Nobel Prize in 2017. In addition to its small size, short generation time, rapid life cycle, and easy breeding at a low cost, Drosophila's simple and well-characterized genetics have made it an invaluable and highly tractable tool for studying neural function and behavior as well as human disease (Bier, 2005). Researchers use heterologous gene expression, utilizing the binary GAL4/UAS system from yeast to introduce genes from different species (Brand and Perrimon, 1993). By exploiting this genetic flexibility and the significant genetic similarities to other insects, such as mosquitoes, Au et al. revealed that the Cryptochrome 1 proteins from nocturnal and diurnal mosquitos can mediate distinct physiological and behavioral responses to blue light in flies, aligning with the specific behaviors of the different mosquito species. Consequently, the authors were able to manipulate the behavior of the fruit flies based on the mosquito version of the protein expressed. More recently, fruit flies have also become accessible for electrophysiological studies, particularly patch clamp recordings, enabling researchers to understand and define the clock circuit responsible for the fly's time perception using just 150 neurons. Notably, the light input and arousal LNv neurons are well-studied, and here Au et al., using a similar experimental approach to above, showed that different photoreceptor systems are integrated in the LNvs to provide functional redundancy for wavelength-dependent light perception that triggers behavioral arousal in the flies.
Lastly, since the seminal work of Hodgkin and Huxley in the 1950s (Hodgkin and Huxley, 1952), computational modeling in neuroscience has become increasingly important and impactful, especially with the ever-increasing computational power available to researchers. In this collection, utilizing computational modeling, Megwa et al. study the mechanism of the sodium potassium ATPase ion pump affecting neuronal plasticity in the well-established larval fly motoneuron system. Accomplishing this task experimentally would have been exceedingly challenging; moreover, its application to vertebrate systems is facilitated by the models' universality.
The objective of this Research Topic was to emphasize and advocate the valuable role of invertebrate preparations for neuroscience research. We received a diverse array of contributions studying a range of different species and techniques and encompassing both original research studies and comprehensive reviews on invertebrate electrophysiology. Our aim is to inspire and motivate more researchers to embrace and study a broad range of invertebrate species using a wide spectrum of electrophysiological techniques once again.
JN: Conceptualization, Writing—original draft, review, and editing. KT: Writing—review and editing. EB: Conceptualization, Writing—original draft, review, and editing.
The author(s) declare that no financial support was received for the research, authorship, and/or publication of this article.
The authors declare that the research was conducted in the absence of any commercial or financial relationships that could be construed as a potential conflict of interest.
All claims expressed in this article are solely those of the authors and do not necessarily represent those of their affiliated organizations, or those of the publisher, the editors and the reviewers. Any product that may be evaluated in this article, or claim that may be made by its manufacturer, is not guaranteed or endorsed by the publisher.
Bier, E. (2005). Drosophila, the golden bug, emerges as a tool for human genetics. Nat. Rev. Genet. 6, 9–23. doi: 10.1038/nrg1503
Brand, A. H., and Perrimon, N. (1993). Targeted gene expression as a means of altering cell fates and generating dominant phenotypes. Development 118, 401–415.
Bullock, T. H. (2000). Revisiting the concept of identifiable neurons. Brain Behav. Evol. 55, 236–240. doi: 10.1159/000006657
Burkhardt, P., Colgren, J., Medhus, A., Digel, L., Naumann, B., Soto-Angel, J. J., et al. (2023). Syncytial nerve net in a ctenophore adds insights on the evolution of nervous systems. Science 380, 293–297. doi: 10.1126/science.ade5645
Clarac, F., and Pearlstein, E. (2007). Invertebrate preparations and their contribution to neurobiology in the second half of the 20th century. Brain Res. Rev. 54, 113–161. doi: 10.1016/j.brainresrev.2006.12.007
Croll, R. P. (1987). “Identified neurons and cellular homologies,” in Nervous Systems in Invertebrates, ed M. A. Ali (Boston, MA: Springer), 41–59.
Hodgkin, A. L., and Huxley, A. F. (1952). A quantitative description of membrane current and its application to conduction and excitation in nerve. J. Physiol. 117, 500–544.
Huber, F., Moore, T. E., and Loher, W. (1989). Cricket Behavior and Neurobiology. Ithaca, NY: Cornell University Press.
Katz, P. S., and Quinlan, P. D. (2019). The importance of identified neurons in gastropod molluscs to neuroscience. Curr. Opin. Neurobiol. 56, 1–7. doi: 10.1016/j.conb.2018.10.009
Konopka, R. J., and Benzer, S. (1971). Clock mutants of Drosophila melanogaster. Proc. Natl. Acad. Sci. U.S.A. 68, 2112–2116.
Marder, E., Bucher, D., Schulz, D. J., and Taylor, A. L. (2005). Invertebrate central pattern generation moves along. Curr. Biol. 15, R685–R699. doi: 10.1016/j.cub.2005.08.022
Menzel, R. (2012). The honeybee as a model for understanding the basis of cognition. Nat. Rev. Neurosci. 13, 758–768. doi: 10.1038/nrn3357
Moroz, L. L., Kocot, K. M., Citarella, M. R., Dosung, S., Norekian, T. P., Povolotskaya, I. S., et al. (2014). The ctenophore genome and the evolutionary origins of neural systems. Nature 510, 109–114. doi: 10.1038/nature13400
Moroz, L. L., and Kohn, A. B. (2016). Independent origins of neurons and synapses: insights from ctenophores. Philos. Trans. R Soc. B 371, 20150041. doi: 10.1098/rstb.2015.0041
Sakurai, A., Gunaratne, C. A., and Katz, P. S. (2014). Two interconnected kernels of reciprocally inhibitory interneurons underlie alternating left-right swim motor pattern generation in the mollusk Melibe leonina. J. Neurophysiol. 112, 1317–1328. doi: 10.1152/jn.00261.2014
Selverston, A. I. (2010). Invertebrate central pattern generator circuits. Philos. Trans. R Soc. B 365, 2329–2345. doi: 10.1098/rstb.2009.0270
Keywords: electrophysiological techniques, neuronal modeling, invertebrates, synapse, neural circuits
Citation: Newcomb JM, Todd K and Buhl E (2023) Editorial: Invertebrate neurophysiology—of currents, cells, and circuits. Front. Neurosci. 17:1303574. doi: 10.3389/fnins.2023.1303574
Received: 28 September 2023; Accepted: 03 October 2023;
Published: 13 October 2023.
Edited and reviewed by: Laura Ballerini, International School for Advanced Studies (SISSA), Italy
Copyright © 2023 Newcomb, Todd and Buhl. This is an open-access article distributed under the terms of the Creative Commons Attribution License (CC BY). The use, distribution or reproduction in other forums is permitted, provided the original author(s) and the copyright owner(s) are credited and that the original publication in this journal is cited, in accordance with accepted academic practice. No use, distribution or reproduction is permitted which does not comply with these terms.
*Correspondence: Edgar Buhl, ZS5idWhsQGJyaXN0b2wuYWMudWs=
Disclaimer: All claims expressed in this article are solely those of the authors and do not necessarily represent those of their affiliated organizations, or those of the publisher, the editors and the reviewers. Any product that may be evaluated in this article or claim that may be made by its manufacturer is not guaranteed or endorsed by the publisher.
Research integrity at Frontiers
Learn more about the work of our research integrity team to safeguard the quality of each article we publish.