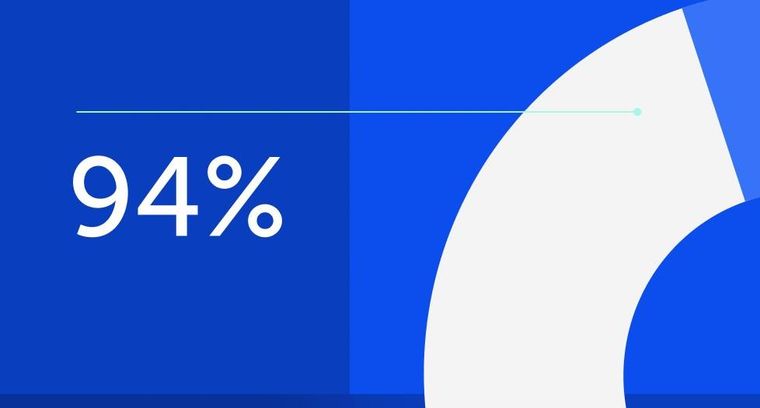
94% of researchers rate our articles as excellent or good
Learn more about the work of our research integrity team to safeguard the quality of each article we publish.
Find out more
ORIGINAL RESEARCH article
Front. Neurosci., 05 December 2023
Sec. Visual Neuroscience
Volume 17 - 2023 | https://doi.org/10.3389/fnins.2023.1298468
This article is part of the Research TopicThe Operationalization of Cognitive Systems in the Comprehension of Visual StructuresView all 5 articles
Objective: To explore the characteristics and mechanisms of working memory impairment in patients with frontal lobe epilepsy (FLE) through a memory game paradigm combined with eye tracking technology.
Method: We included 44 patients with FLE and 50 healthy controls (HC). All participants completed a series of neuropsychological scale assessments and a short-term memory game on an automated computer-based memory evaluation platform with an eye tracker.
Results: Memory scale scores of FLE patients including digit span (U = 747.50, p = 0.007), visual recognition (U = 766.50, p = 0.010), and logical memory (U = 544.00, p < 0.001) were significantly lower than HC. The patients with FLE took longer to complete the four levels of difficulty of the short-term memory game than healthy controls (level 1: U = 2974.50, p = 0.000; level 2: U = 3060.50, p = 0.000; level 3: U = 2465.00, p = 0.000; level 4: U = 2199.00, p = 0.000). During the memory decoding period, first fixation on the targets took significantly longer for FLE patients for all difficulty levels compared to controls (level 1: U = 3407.00, p = 0.008; level 2: U = 3618.00, p = 0.036; level 3: U = 3345.00, p = 0.006; level 4: U = 2781.00, p = 0.000). The average fixation duration per target among patients with FLE was found to be significantly longer compared to HC (level 1: U = 2994.50, p = 0.000; level 2: U = 3101.00, p = 0.000; level 3: U = 2559.50, p = 0.000; level 4: U = 2184.50, p = 0.000). The total fixation duration on AOI/total completion time of FLE patients was significantly lower than those of HC for levels 1 to 3 (level 1: U = 1557.00, p = 0.000; level 2: U = 2333.00, p = 0.000; level 3: U = 2757.00, p = 0.000). Furthermore, the eye tracking data during the memory decoding phase were correlated with neuropsychological scale scores (p < 0.05).
Conclusion: Patients with FLE exhibited short-term memory impairment probably due to deficits in attentional maintenance, especially during the memory decoding phase. Eye tracking technology provided the possibility to help separate and quantify visual attention from memory processing, contributing to exploring underlying mechanisms of memory impairment in FLE.
Frontal lobe epilepsy (FLE) is the second most prevalent focal epilepsy in adults, accounting for approximately 20–30% of cases (Giovagnoli et al., 2020; McGonigal, 2022). It is increasingly recognized as a brain network disorder, which impacts a broad range of cognitive domains (Rayner et al., 2015; Nair and Szaflarski, 2020). Although attention deficits and impaired executive functioning are relatively common, memory deficits have also been commonly described among patients with FLE (Kibby et al., 2019). However, the frontal lobe is not traditionally the brain area responsible for memory function (Buschman and Miller, 2007; Centeno et al., 2010; Al-Aidroos et al., 2012; Fiebelkorn and Kastner, 2020). Therefore, how memory can be impaired in FLE and whether patients have specific memory characteristics remain inconclusive.
Previous studies have demonstrated that attention plays a crucial role in the process of memory formation (Zelinsky and Loschky, 2005; Chun and Johnson, 2011; Bahmani et al., 2019). More specifically, visual attention fundamentally determines which information is inputted and encoded into memory (Hollingworth et al., 2001; Voss et al., 2017). Rock and Gutman (1981) have proven that people can better recall items that have received more visual attention. Bahmani et al. (2019) also confirmed the inextricable relationship between memory and visual attention at the electrophysiological, behavioral, and anatomical levels. However, the conventional scales of memory evaluations, which include the Wechsler Memory Scale (WMS), the Hopkins Verbal Learning Test, and the Rey Auditory Verbal Learning Test, are unable to separate visual attention from memory and lack the ability to gauge and accurately measure it (Sherman et al., 2012; McAuley et al., 2015; Sveikata et al., 2019). Consequently, a tool that can quantitatively measure visual attention during memory processes and assist us in investigating the influence of attention deficit on memory impairment is urgently needed.
Eye tracking technology has the ability to capture visual behaviors and track the path of an individual’s eye movements in real time as patients engage in cognitive tasks (Boraston and Blakemore, 2007; Oyama et al., 2019; Tao et al., 2020). When integrated with memory tasks, it can accurately and comprehensively quantify visual attention (Boraston and Blakemore, 2007; Blais et al., 2008). Sylvia B. Guillory applied eye tracking-based measurement in the study of memory function among individuals with Autism Spectrum Disorder (ASD), specifically those with Phelan-McDermid Syndrome (PMS) (Guillory et al., 2021). They revealed that patients with PMS and co-morbid ASD diagnoses have poorer memory and lower attentional engagement with social images (Guillory et al., 2021). Using oculomotor tracking combined with a visual paired-comparison paradigm, patients with Rett syndrome showed restricted and immature visual attention characteristics during recognition memory tasks (Rose et al., 2013). In our previous work on an automated computer-based memory assessment platform with an eye tracker, we found that TLE patients experienced memory retrieval difficulties with relative sparing of attention (Zhu et al., 2021; Xiao et al., 2023).
In the present study, we hypothesize that patients with FLE might have a specific memory impairment profile, which is different from those of TLE, and attention might contribute to deficits in the memory process in FLE. To this end, we first applied the digit span, visual recognition, and logical memory scales, as per the Wechsler Memory Scales-Chinese Revision (WMS-RC), in patients with FLE. To further delineate the patterns of memory impairment, we employed an automated memory assessment platform, which, relying on eye tracking technology, recorded and analyzed associated parameters in both memory encoding and decoding phases, including total completion time, first fixation time on target, average fixation duration per target, etc. We made a correlation analysis between the eye tracking index and the performance of memory scales. Our work showed that patients with FLE exhibited impaired short-term memory function, which might be mostly due to deficits in attention maintenance, especially during the memory decoding phase.
A total of 44 FLE patients [22 male, age 28.11 (9.15)] and 50 healthy controls (HC) [22 male, age 30.22 (12.39)] were enrolled in our study and were matched for age, sex (male/female), and years of education. All FLE patients were recruited from September 2020 to August 2021 at Xiangya Hospital, Central South University, and were diagnosed with FLE according to the definition of the International League Against Epilepsy (ILAE) by two trained epileptologists (Fisher et al., 2017). The diagnostic criteria for FLE were based on history (typical symptoms of seizures suggestive of frontal lobe origin), EEG, and neuroimaging. Exclusion criteria: (1) those aged under 16 years or over 60 years; (2) those who had a history of neurological disorders other than epilepsy and a history of neurosurgery; (3) those who claimed to have subjective memory impairment; (4) those who also had psychiatric disorders or comorbidities; (5) those who failed the eye tracking calibration procedure or were unable to understand the experimental procedure.
All the participants undertook a memory scale assessment. We applied digit span, visual recognition, and logical memory scales, as per the Wechsler Memory Scales-Chinese Revision (WMS-RC), to assess the memory ability of the participants.
Digit span aims to measure verbal working memory and can be conducted forward and backward. In digit span forward, the participants are required to repeat a string of digits in sequence after hearing them, starting by repeating 3 digits, with a maximum of 11 digits, and there are two chances for each digit length. In digit span backward, the participants are required to repeat the digits in reverse, starting with 2 digits, with a maximum of 10. The test was terminated when 11 digits were correctly recalled or incorrectly recalled twice in a row.
Visual recognition measures visuospatial memory. In this task, participants were shown eight cards for 30 s and were required to recall the eight cards in 28 cards. The cards included graphics, Chinese characters, and mathematical symbols. The more cards the participant recognized, the higher the score.
Logical memory task measured verbal episodic memory. The participants were asked to browse a short story, and the investigator would read it aloud at the same time. When the investigator finished reading, the story card would be taken back and the participants were required to immediately recall the story in as much detail as possible. There were two different short stories for each trial. The more details the participant recalled, the higher the score.
An automated computer-based memory assessment platform was adapted from Li et al., as reported in our previous study, for measuring short-term memory. The stimuli included 38 front-facing human images downloaded from the Chinese University of Hong Kong (CUHK) student database1 and 38 images of fractals downloaded from the Web.
The participants were presented with the image(s) for 5 s (referred to as the encoding phase); then, the image(s) would disappear and 12 images in 3 rows and 4 columns would be presented on the screen (referred to as the decoding phase). They were required to choose the image they memorized by clicking the mouse, they could not proceed with the next trial until all the correct targets had been clicked. There are four difficulty levels in the short-term memory game, starting with memorizing one target (level 1) and ending with memorizing four memory targets (level 4), with four trials in each level (Figure 1). The terms “YES” and “NO” are presented as feedback for the correct selections and incorrect selections, respectively.
Figure 1. During the trial, a target was shown on the screen for 5 s (encoding), followed by the appearance of 12 (4 × 3) potential answers. Participants were required to recall and click on the correct target from the 12 objects (decoding). The targets were numbered from 1 to 4, and participants were required to choose all the correct targets during the decoding phase in order to proceed to the next level. The orange circle indicates the target and the white circle in the decoding phase is the interference graphic.
Concurrently with the assessment of short-term memory, we gathered real-time eye tracking information utilizing the EyeLink 1,000 Plus eye tracker to observe the visual search conducted by the participants throughout the task. The eye tracking data was captured in monocular remote mode with a sampling rate of 500 Hz. To ensure accuracy, participants were instructed to minimize movement of their upper body while using the remote mode. Minor movements of the head and upper body were permitted, as the oculomotor system can be automatically calibrated. A standard 9-point calibration procedure was implemented to guarantee the precision and accuracy of the collected data. Participants who were unable to pass the calibration process were excluded from the subsequent experiment.
Eye tracking data were analyzed with DataViewer (version 4.2). We extracted the eye tracking indicators for the encoding and decoding phases separately. Fixation, saccade, and other behaviors were directly calculated using the EyeLink system. The EyeLink system uses three thresholds to detect saccades and fixation: motion, velocity, and acceleration, corresponding to 0.15 degrees, 30 degrees/s, and 8,000 degrees/s2, respectively. The update interval and data accumulation period of the whole system are set at 50 milliseconds. The eye tracking indicators included total completion time, first fixation time on target, average fixation duration per target, average fixation count per target, total fixation duration on area of interest (AOI)/total completion time, and average fixation duration per target/total fixation duration on AOI. First fixation time on target refers to the time point when the participants notice the presented target. The reaction time refers to the duration between the introduction of a stimulus and the subsequent response. The first fixation time on target in the encoding phase can be used to reflect the subject’s response and alertness when participating in the experiment. Average fixation duration per target is the average fixation durations within per target. Average fixation count per target is the average number of fixations that fall within the target. Total fixation duration on AOI/total completion time is the percentage of total fixation duration on AOI to total completion time. Average fixation duration per target/total fixation duration on AOI is the percentage of average fixation duration per target to the total fixation duration on AOI. We used Python (version 3.7.10) to process the eye tracking data. For each participant, the eye tracking indicators were averaged over the trials to obtain the average indicator corresponding to each difficulty level. We also extracted the above eye tracking indicators under memorized fractal images and memorized front-facing images.
The statistical analysis was conducted using the SPSS software package (version 27.0; SPSS Inc., Chicago, Illinois, United States). In order to analyze categorical variables such as sex in both FLE patients and healthy controls, χ2 analysis was employed. Continuous variables such as age and years of education, which passed the normality test, were analyzed using an independent samples t-test. The data were presented as mean and standard deviation (Mean ± SD). For memory scale scores and eye tracking data that significantly deviated from normal distribution, the Mann–Whitney U test was used to compare the differences between the two groups. Spearman’s correlation analysis was used to determine the correlation between the eye tracking indicators and the memory scale score. Two-tailed p-values were calculated for all tests, and p-values less than 0.05 were considered statistically significant.
A total of 44 adult FLE patients and 50 HC eligible for this study were included. The demographic and clinical characteristics of the participants are shown in Table 1. No statistically significant differences were found between FLE patients and HC regarding sex (p = 0.561), age (p = 0.341), and years of education (p = 0.079).
As shown in Table 2, there were significant differences between FLE patients and HC in the scores of scales reflecting memory function, including digit span (U = 747.50, p = 0.007), visual recognition (U = 766.50, p = 0.010), and logical memory (U = 544.00, p < 0.001). The FLE patients’ scores in these scales were significantly lower than those of HC.
There was a significant difference in total completion time between the two groups in the decoding phase in the four difficulty levels (level 1: U = 2974.50, p = 0.000; level 2: U = 3060.50, p = 0.000; level 3: U = 2465.00, p = 0.000; level 4: U = 2199.00, p = 0.000). The total completion time of the FLE patients in the four difficulty levels was significantly longer than that of the HC (Figure 2A).
Figure 2. Comparison of (A) total completion time of the Short-Term Memory task, (B) total completion time under fractal image stimuli, and (C) total completion time under front-facing image stimuli between the HC group and FLE group at four difficulty levels. Statistical significance for each task between groups is indicated by asterisk(s) (*p < 0.05, **p < 0.01, ***p < 0.001). HC, healthy control; FLE, frontal lobe epilepsy.
The total completion time of FLE patients under fractal image stimuli was significantly longer than that of HC in levels 1–4 (level 1: U = 653.00, p = 0.001; level 2: U = 697.00, p = 0.002; level 3: U = 509.00, p = 0.000; level 4: U = 569.00, p = 0.000) (Figure 2B). The total completion time of FLE patients under front-facing image stimuli was also significantly longer than that of HC in levels 1–4 (level 1: U = 794.00, p = 0.020; level 2: U = 732.00, p = 0.005; level 3: U = 710.00, p = 0.003; level 4: U = 535.00, p = 0.000) (Figure 2C).
In the memory encoding phase, the first fixation time on targets of FLE patients was significantly longer than that of HC in level 4 (U = 3603.5, p = 0.032), while no significant difference was found in the remaining difficulty levels. There were no differences in average fixation duration per target and average fixation count per target in levels 1 to 4 (all p > 0.05). When it comes to different image stimuli, no differences were found in all eye tracking indicators in the four difficulty levels under both fractal image stimuli and front-facing image stimuli (Table 3).
The first fixation time on targets of FLE patients was significantly longer than that of HC in the four difficulty levels (level 1: U = 3407.00, p = 0.008; level 2: U = 3618.00, p = 0.036; level 3: U = 3345.00, p = 0.006; level 4: U = 2781.00, p = 0.000). The average fixation duration per target of FLE patients was significantly longer than that of HC (level 1: U = 2994.50, p = 0.000; level 2: U = 3101.00, p = 0.000; level 3: U = 2559.50, p = 0.000; level 4: U = 2184.50, p = 0.000), the average fixation count per target of FLE patients were also significantly higher than that of HC (level 1: U = 3206.50, p = 0.001; level 2: U = 3320.00, p = 0.004; level 3: U = 2855.50, p = 0.000; level 4: U = 2571.50, p = 0.000). The total fixation duration on AOI/total completion time of FLE patients was significantly lower than that of HC in levels 1 to 3 (level 1: U = 1557.00, p = 0.000; level 2: U = 2333.00, p = 0.000; level 3: U = 2757.00, p = 0.000), while no difference was found in level 4 (level 4: U = 3683.00, p = 0.054). The average fixation duration per target/total fixation duration on AOI of FLE patients was significantly higher than that of HC in level 1 (U = 3987.00, p = 0.020), while no differences were found in the remaining difficulty levels (Table 4 and Figure 3).
Figure 3. Comparison of (A) first fixation time on target, (B) average fixation duration per target, (C) average fixation count per target, and (D) total fixation duration on AOI/total completion time of the decoding phase in all tasks between the HC group and FLE group. Statistical significance for each task between groups is indicated by asterisk(s) (*p < 0.05, **p < 0.01, ***p < 0.001). HC, healthy control; FLE, frontal lobe epilepsy.
The first fixation time on target of FLE patients was significantly longer than that of HC (level 1: U = 695.00, p = 0.002; level 2: U = 798.50, p = 0.022; level 3: U = 728.00, p = 0.007; level 4: U = 652.00, p = 0.001). The average fixation duration per target of FLE patients was significantly longer than that of HC (level 1: U = 720.00, p = 0.004; level 2: U = 709.00, p = 0.003; level 3: U = 578.00, p = 0.000; level 4: U = 459.00, p = 0.000), and the average fixation count per target of FLE patients was significantly higher than that of HC (level 1: U = 829.00, p = 0.039; level 2: U = 761.50, p = 0.010; level 3: U = 655.00, p = 0.001; level 4: U = 597.00, p = 0.000). The total fixation duration on AOI/total completion time of FLE patients was significantly lower than that of HC (level 1: U = 369.00, p = 0.000; level 2: U = 463.00, p = 0.000; level 3: U = 544.00, p = 0.000) (Figure 4).
Figure 4. Comparison of (A) first fixation time on target, (B) average fixation duration per target, (C) average fixation count per target, and (D) total fixation duration on AOI/total completion time of the decoding phase in the fractal image stimuli task between the HC group and FLE group. Statistical significance for each task between groups is indicated by asterisk(s) (*p < 0.05, **p < 0.01, ***p < 0.001). HC, healthy control; FLE, frontal lobe epilepsy.
The first fixation time on target of FLE patients was significantly longer than that of HC (level 4: U = 745.00, p = 0.010), the average fixation duration per target of FLE patients was significantly longer than that of HC (level 1: U = 774.50, p = 0.014; level 2: U = 836.00, p = 0.045; level 3: U = 723.50, p = 0.004; level 4: U = 636.50, p = 0.000), the average fixation count per target of FLE patients was significantly higher than that of HC (level 1: U = 783,50, p = 0.016; level 3: U = 783.00, p = 0.016, level 4: U = 636.50, p = 0.000). The total fixation duration on AOI/total completion time of FLE patients was significantly lower than that of HC (level 1: U = 395.00, p = 0.000; level 2: U = 695.00, p = 0.002; level 3: U = 799.00, p = 0.023) (Figure 5).
Figure 5. Comparison of (A) average fixation duration per target, (B) average fixation count per target, and (C) total fixation duration on AOI/total completion time of the decoding phase in the front-facing image stimuli task between the HC group and FLE group. Statistical significance for each task between groups is indicated by asterisk(s) (*p < 0.05, **p < 0.01, ***p < 0.001). HC, healthy control; FLE, frontal lobe epilepsy.
The total completion time of the decoding phase was negatively correlated with the scores of the digit span (rs = −0.525, p = 0.000), visual recognition (rs = −0.315, p = 0.002), and logical memory (rs = −0.398, p = 0.000) (Figure 6).
Figure 6. Correlations between the scores of memory scale assessment (digit span, visual recognition, logical memory) and TCT, FFT on AOI, FFT on target, AFD per target, AFC per target, TFD on AOI/TCT, AFD per target/TFD on AOI. Statistical significance for each task between groups is indicated by asterisk(s) (*p < 0.05, **p < 0.01, ***p < 0.001). TCT, total completion time; FFT, first fixation time; AOI, area of interest; AFD, average fixation duration; AFC, average fixation count; TFD, total fixation duration.
First fixation time on targets in the decoding phase was negatively correlated with the scores of the digit span (rs = −0.406, p = 0.000), visual recognition (rs = −0.370, p = 0.000), and logical memory (rs = −0.374, p = 0.000). The average fixation duration per target in the decoding phase was negatively correlated with the digit span (rs = −0.511, p = 0.000), visual recognition (rs = −0.367, p = 0.000), and logical memory (rs = −0.378, p = 0.000) scores. The average fixation count per target was also negatively correlated with the scores of digit span (rs = −0.515, p = 0.000), visual recognition (rs = −0.291, p = 0.004), and logical memory (rs = −0.361, p = 0.000). There was a positive correlation between the total fixation duration on AOI/total completion time and logical memory score (rs = 0.262, p = 0.011) (Figure 6).
In this study, we confirmed the presence of memory deficits among FLE patients in combination with scales and short-term memory tests by eye tracking technology. We found that FLE patients showed intact attentional alertness but impaired attentional retaining in memory processes. In addition, these differences in eye tracking metrics appeared mainly during the memory decoding phase and performed worse with both fractal and front-facing image stimuli. Poor performance in memory scales associated with the eye tracking data suggests that eye tracking technology has the potential to be used as a supplementary neuropsychological tool for memory evaluation.
It has been widely accepted that patients with FLE do possess memory impairments, showing dysfunction during encoding, free recall, and retrieval (Exner et al., 2002, Nolan et al., 2004), and there is also evidence of memory deficit following frontal lobe resection for epilepsy (McDonald et al., 2001). In our study, FLE patients performed worse on both the WMS-RC test and the eye tracking-based short-term memory tests, confirming the view that memory deficit is one of the long-term effects of FLE on the neural networks involved in memory function. The medial prefrontal cortex, along with an intact connection with the medial temporal lobe and hippocampus, has been involved in memory encoding and retrieval (Centeno et al., 2010; Squire et al., 2015; van den Berg et al., 2021). Petrides and Pandya (1988) have identified a larger group of fiber projections in the frontal cortex that originated from the various areas of the temporal region, which might be the anatomic basis of the memory circuit. The rich interconnectivity between the temporal and frontal lobes may facilitate epileptic activity propagation and subsequent dysfunction in distant structures. Additionally, a series of functional neuroimaging findings have revealed that the frontal lobe participated in supporting effective memory in conjunction with memory-associated structures like the hippocampus (Centeno et al., 2012; Braakman et al., 2013). Therefore, we inferred that the memory deficits in FLE might be partly due to the dysfunction of different regions within the frontal lobes contributing to long-term memory functioning (Patrikelis et al., 2009; Kibby et al., 2019; Caciagli et al., 2023).
The frontal lobe is the hub of the attentional cognitive control network, also involved in the orchestrating functions of attention. Thus, whether the memory impairment among patients with FLE was dependent on attention deficit was further investigated. We found no difference in performance between FLE and HC in the first fixation time on target in the memory encoding phase, which means the attentional alerting is mostly retained in FLE to guarantee that they are in a heightened sensitive state and have the ability to respond fast and correctly (Posner and Petersen, 1990). However, the following sustained attention during the retrieval period has been identified as obviously impaired due to a lower ratio of the total fixation duration on AOI/total completion time among patients with FLE. Previous studies have shown that the frontal cortex not only produces regulatory signals related to attention but also functions to maintain and control spatial attention (Fiebelkorn and Kastner, 2020). Thus, we inferred that the recurrent epileptic discharges and pathology in different frontal regions would interfere with the attentional network and further negatively affect higher cognitive functions such as attention and memory (Rayner et al., 2015; Fiebelkorn and Kastner, 2020).
We also noticed that the patients with FLE showed impairments primarily in recognition memory, which aligns with previous reports by Centeno et al. (2010). They observed that poor memory performance in FLE was caused by difficulties in information retrieving, rather than encoding dysfunction (Centeno et al., 2010), resulting in repeatedly searching for the correct target, corresponding to longer fixation duration and more fixation counts on the target captured. Why these difficulties in recalling memories mostly contributed to memory deficits in FLE patients is of interest. We believe a possible reason is that the damaged prefrontal cortex from FLE disturbs the memory decoding process. The hippocampus is responsible for the initial memory encoding, while the prefrontal cortex takes charge of memory retrieval and consolidation (Preston and Eichenbaum, 2013; Hainmueller and Bartos, 2020). During the memory recall phase, the prefrontal cortex receives visual input, integrates prior knowledge and short-term memory from the hippocampus, and ultimately regulates attentional allocation (Buschman and Miller, 2007; Katsuki and Constantinidis, 2014). Consequently, when the frontal lobe and affective cognitive network exhibit structural or functional abnormalities, memory recollection in the decoding phase suffers. Accumulating evidence has supported this hypothesis by showing that patients with right frontal lobe tumors performed poorly in the retrieval process (Anderson et al., 2011). Our study involved six patients diagnosed with FLE, specifically experiencing frontal lobe absence seizures. Drawing from previous research on absence seizures, we hypothesize that abnormal discharges originating in the frontal lobe contribute to cognitive impairment and disturbances in consciousness among these patients (Lenkov et al., 2013; Wang et al., 2023). In forthcoming research, the integration of eye movement techniques with advanced methods like functional magnetic resonance imaging (fMRI) and magnetoencephalography (MEG) could further explore the influence of the frontal lobe on absence seizures.
There is also a significant correlation between the total completion time of the memory game and the scores of the scales. Previous studies have found that the eye tracking index correlates well with cognitive performance. Therefore, we believe that eye tracking technology combined with cognitive tasks could be applied as an alternative option for neuropsychological assessment in clinical practice and long-term monitoring. Considering our finding of short-term memory deficit, probably due to attentional maintenance dysfunction during memory retrieval, future psychological training strategies, which focus on cognitive adjusting to sustain attentional focus, are suggested to cope with those problems and to improve memory.
Nevertheless, there are several limitations in our current study. Firstly, it was a clinical study conducted at a single center with small sample size; prospective studies with larger participation with long-term follow-up are required. Secondly, lateralization of FLE would have an effect on cognitive performance. For better screening of the memory deficit profile of FLE, future studies should take lateralization into consideration. Moreover, the impact of anti-seizure medications on cognition is an inevitable confounding factor that limits our statistical power, newly diagnosed patients should be enrolled to exclude the interfering effects of drugs. Additionally, we can design new memory game paradigms that combine fractal pictures and frontal-facing images, which can facilitate further investigation of the connection and difference between general and social cognition.
Our study innovatively combined traditional scales and eye tracking short-term memory tasks, to explore the characteristics and mechanisms of working memory impairment in patients with FLE. We found that short-term memory deficits in FLE patients are probably due to attentional maintenance dysfunction during the memory retrieval phase. Eye tracking technology with cognitive tasks could be a reliable potential supplementary neuropsychological tool for cognition evaluation and precise process intervention.
The raw data supporting the conclusions of this article will be made available by the authors, without undue reservation.
The studies involving humans were approved by Ethics Committee of Xiangya Hospital of Central South University. The studies were conducted in accordance with the local legislation and institutional requirements. The participants provided their written informed consent to participate in this study.
QZ: Data curation, Formal analysis, Investigation, Writing – original draft, Writing – review & editing. WS: Formal analysis, Methodology, Software, Visualization, Writing – review & editing. KH: Data curation, Writing – review & editing. LQ: Writing – review & editing. SW: Data curation, Writing – review & editing. XL: Writing – review & editing. QW: Software, Writing – review & editing. LF: Conceptualization, Project administration, Supervision, Writing – review & editing.
The author(s) declare financial support was received for the research, authorship, and/or publication of this article. This study was supported by the National Key Research and Development Program of China (2022YFC2503804), National Natural Science Foundation of China (82071461; 82271503), “From 0 to 1” Original Innovation Project of the Basic Frontier Scientific Research Program of the Chinese Academy of Sciences (29 J20-015-III), Natural Science Foundation of Hunan Province (2021JJ31060; 2023JJ30919).
We are very grateful to all of the participants in this study.
The authors declare that the research was conducted in the absence of any commercial or financial relationships that could be construed as a potential conflict of interest.
All claims expressed in this article are solely those of the authors and do not necessarily represent those of their affiliated organizations, or those of the publisher, the editors and the reviewers. Any product that may be evaluated in this article, or claim that may be made by its manufacturer, is not guaranteed or endorsed by the publisher.
Al-Aidroos, N., Said, C. P., and Turk-Browne, N. B. (2012). Top-down attention switches coupling between low-level and high-level areas of human visual cortex. Proc. Natl. Acad. Sci. U. S. A. 109, 14675–14680. doi: 10.1073/pnas.1202095109
Anderson, N. D., Davidson, P. S. R., Mason, W. P., Gao, F., Binns, M. A., and Winocur, G. (2011). Right frontal lobe mediation of recollection-and familiarity-based verbal recognition memory: evidence from patients with tumor resections. J. Cogn. Neurosci. 23, 3804–3816. doi: 10.1162/jocn_a_00050
Bahmani, Z., Clark, K., Merrikhi, Y., Mueller, A., Pettine, W., Isabel Vanegas, M., et al. (2019). Prefrontal contributions to attention and working memory. Curr. Top. Behav. Neurosci. 41, 129–153. doi: 10.1007/7854_2018_74
Blais, C., Jack, R. E., Scheepers, C., Fiset, D., and Caldara, R. (2008). Culture shapes how we look at faces. PLoS One 3:e3022. doi: 10.1371/journal.pone.0003022
Boraston, Z., and Blakemore, S.-J. (2007). The application of eye-tracking technology in the study of autism. J. Physiol. 581, 893–898. doi: 10.1113/jphysiol.2007.133587
Braakman, H. M. H., Vaessen, M. J., Jansen, J. F. A., Debeij-van Hall, M. H. J. A., de Louw, A., Hofman, P. A. M., et al. (2013). Frontal lobe connectivity and cognitive impairment in pediatric frontal lobe epilepsy. Epilepsia 54, 446–454. doi: 10.1111/epi.12044
Buschman, T. J., and Miller, E. K. (2007). Top-down versus bottom-up control of attention in the prefrontal and posterior parietal cortices. Science 315, 1860–1862. doi: 10.1126/science.1138071
Caciagli, L., Paquola, C., He, X., Vollmar, C., Centeno, M., Wandschneider, B., et al. (2023). "disorganization of language and working memory systems in frontal versus temporal lobe epilepsy." brain: a. J. Neurol. 146, 935–953. doi: 10.1093/brain/awac150
Centeno, M., Thompson, P. J., Koepp, M. J., Helmstaedter, C., and Duncan, J. S. (2010). Memory in frontal lobe epilepsy. Epilepsy Res. 91, 123–132. doi: 10.1016/j.eplepsyres.2010.07.017
Centeno, M., Vollmar, C., O'Muircheartaigh, J., Stretton, J., Bonelli, S. B., Symms, M. R., et al. (2012). Memory in frontal lobe epilepsy: an fMRI study. Epilepsia 53, 1756–1764. doi: 10.1111/j.1528-1167.2012.03570.x
Chun, M. M., and Johnson, M. K. (2011). Memory: enduring traces of perceptual and reflective attention. Neuron 72, 520–535. doi: 10.1016/j.neuron.2011.10.026
Exner, C., Boucsein, K., Lange, C., Winter, H., Weniger, G., Steinhoff, B. J., et al. (2002). Neuropsychological performance in frontal lobe epilepsy. Seizure 11, 20–32. doi: 10.1053/seiz.2001.0572
Fiebelkorn, I. C., and Kastner, S. (2020). Functional specialization in the attention network. Annu. Rev. Psychol. 71, 221–249. doi: 10.1146/annurev-psych-010418-103429
Fisher, R. S., Cross, J. H., French, J. A., Higurashi, N., Hirsch, E., Jansen, F. E., et al. (2017). Operational classification of seizure types by the international league against epilepsy: position paper of the ILAE Commission for Classification and Terminology. Epilepsia 58, 522–530. doi: 10.1111/epi.13670
Giovagnoli, A. R., Tallarita, G. M., Parente, A., Pastori, C., and de Curtis, M. (2020). The understanding of mental states and the cognitive phenotype of frontal lobe epilepsy. Epilepsia 61, 747–757. doi: 10.1111/epi.16457
Guillory, S. B., Baskett, V. Z., Grosman, H. E., McLaughlin, C. S., Isenstein, E. L., Wilkinson, E., et al. (2021). Social visual attentional engagement and memory in Phelan-McDermid syndrome and autism spectrum disorder: a pilot eye tracking study. J. Neurodev. Disord. 13:58. doi: 10.1186/s11689-021-09400-2
Hainmueller, T., and Bartos, M. (2020). Dentate gyrus circuits for encoding, retrieval and discrimination of episodic memories. Nat. Rev. Neurosci. 21, 153–168. doi: 10.1038/s41583-019-0260-z
Hollingworth, A., Williams, C. C., and Henderson, J. M. (2001). To see and remember: visually specific information is retained in memory from previously attended objects in natural scenes. Psychon. Bull. Rev. 8, 761–768. doi: 10.3758/BF03196215
Katsuki, F., and Constantinidis, C. (2014). Bottom-up and top-down attention: different processes and overlapping neural systems. Neuroscientist 20, 509–521. doi: 10.1177/1073858413514136
Kibby, M. Y., Cohen, M. J., Stanford, L., and Park, Y. D. (2019). Are frontal and temporal lobe epilepsy dissociable in their memory functioning? Epilepsy Behav. 99:106487. doi: 10.1016/j.yebeh.2019.106487
Lenkov, D. N., Volnova, A. B., Pope, A. R. D., and Tsytsarev, V. (2013). Advantages and limitations of brain imaging methods in the research of absence epilepsy in humans and animal models. J. Neurosci. Methods 212, 195–202. doi: 10.1016/j.jneumeth.2012.10.018
McAuley, J. W., Passen, N., Prusa, C., Dixon, J., Cotterman-Hart, S., and Shneker, B. F. (2015). An evaluation of the impact of memory and mood on antiepileptic drug adherence. Epilepsy Behav. 43, 61–65. doi: 10.1016/j.yebeh.2014.11.017
McDonald, C. R., Bauer, R. M., Grande, L., Gilmore, R., and Roper, S. (2001). The role of the frontal lobes in memory: evidence from unilateral frontal resections for relief of intractable epilepsy. Arch. Clin. Neuropsychol. 16, 571–585. doi: 10.1093/arclin/16.6.571
McGonigal, A. (2022). Frontal lobe seizures: overview and update. J. Neurol. 269, 3363–3371. doi: 10.1007/s00415-021-10949-0
Nair, S., and Szaflarski, J. P. (2020). Neuroimaging of memory in frontal lobe epilepsy. Epilepsy Behav. 103:106857. doi: 10.1016/j.yebeh.2019.106857
Nolan, M. A., Redoblado, M. A., Lah, S., Sabaz, M., Lawson, J. A., Cunningham, A. M., et al. (2004). Memory function in childhood epilepsy syndromes. J. Paediatr. Child Health 40, 20–27. doi: 10.1111/j.1440-1754.2004.00284.x
Oyama, A., Takeda, S., Ito, Y., Nakajima, T., Takami, Y., Takeya, Y., et al. (2019). Novel method for rapid assessment of cognitive impairment using high-performance eye-tracking technology. Sci. Rep. 9:12932. doi: 10.1038/s41598-019-49275-x
Patrikelis, P., Angelakis, E., and Gatzonis, S. (2009). Neurocognitive and behavioral functioning in frontal lobe epilepsy: a review. Epilepsy Behav. 14, 19–26. doi: 10.1016/j.yebeh.2008.09.013
Petrides, M., and Pandya, D. N. (1988). Association fiber pathways to the frontal cortex from the superior temporal region in the rhesus monkey. J. Comp. Neurol. 273, 52–66. doi: 10.1002/cne.902730106
Posner, M. I., and Petersen, S. E. (1990). The attention system of the human brain. Annu. Rev. Neurosci. 13, 25–42. doi: 10.1146/annurev.ne.13.030190.000325
Preston, A. R., and Eichenbaum, H. (2013). Interplay of hippocampus and prefrontal cortex in memory. Curr. Biol. 23, R764–R773. doi: 10.1016/j.cub.2013.05.041
Rayner, G., Jackson, G. D., and Wilson, S. J. (2015). Behavioral profiles in frontal lobe epilepsy: autobiographic memory versus mood impairment. Epilepsia 56, 225–233. doi: 10.1111/epi.12902
Rock, I., and Gutman, D. (1981). The effect of inattention on form perception. J. Exp. Psychol. Hum. Percept. Perform. 7, 275–285. doi: 10.1037/0096-1523.7.2.275
Rose, S. A., Djukic, A., Jankowski, J. J., Feldman, J. F., Fishman, I., and Valicenti-McDermott, M. (2013). Rett syndrome: an eye-tracking study of attention and recognition memory. Dev. Med. Child Neurol. 55, 364–371. doi: 10.1111/dmcn.12085
Sherman, E. M. S., Brooks, B. L., Fay-McClymont, T. B., and MacAllister, W. S. (2012). Detecting epilepsy-related cognitive problems in clinically referred children with epilepsy: is the WISC-IV a useful tool? Epilepsia 53, 1060–1066. doi: 10.1111/j.1528-1167.2012.03493.x
Squire, L. R., Genzel, L., Wixted, J. T., and Morris, R. G. (2015). Memory consolidation. Cold Spring Harb. Perspect. Biol. 7:a021766. doi: 10.1101/cshperspect.a021766
Sveikata, L., Kavan, N., Pegna, A. J., Seeck, M., Assal, F., Momjian, S., et al. (2019). Postoperative memory prognosis in temporal lobe epilepsy surgery: the contribution of postictal memory. Epilepsia 60, 1639–1649. doi: 10.1111/epi.16281
Tao, L., Wang, Q., Liu, D., Wang, J., Zhu, Z., and Feng, L. (2020). Eye tracking metrics to screen and assess cognitive impairment in patients with neurological disorders. Neurol. Sci. 41, 1697–1704. doi: 10.1007/s10072-020-04310-y
van den Berg, L., de Weerd, A., Reuvekamp, M., Hagebeuk, E., and van der Meere, J. (2021). Working memory in pediatric frontal lobe epilepsy. Appl. Neuropsychol. Child 10, 101–110. doi: 10.1080/21622965.2019.1611431
Voss, J. L., Bridge, D. J., Cohen, N. J., and Walker, J. A. (2017). A closer look at the Hippocampus and memory. Trends Cogn. Sci. 21, 577–588. doi: 10.1016/j.tics.2017.05.008
Wang, Y., Li, Y., Sun, F., Xu, Y., Xu, F., Wang, S., et al. (2023). Altered neuromagnetic activity in default mode network in childhood absence epilepsy. Front. Neurosci. 17:1133064. doi: 10.3389/fnins.2023.1133064
Xiao, L., Zhu, G., Huang, K., Wen, S., Feng, L., Li, B., et al. (2023). Memory characteristics in mesial temporal lobe epilepsy: insights from an eye tracking memory game and neuropsychological assessments. CNS Neurosci. Ther. 29, 2621–2633. doi: 10.1111/cns.14203
Zelinsky, G. J., and Loschky, L. C. (2005). Eye movements serialize memory for objects in scenes. Percept. Psychophys. 67, 676–690. doi: 10.3758/BF03193524
Keywords: eye tracking, memory deficit, frontal lobe epilepsy, visual attention, eye movement
Citation: Zhang Q, Sun W, Huang K, Qin L, Wen S, Long X, Wang Q and Feng L (2023) Frontal lobe epilepsy: an eye tracking study of memory and attention. Front. Neurosci. 17:1298468. doi: 10.3389/fnins.2023.1298468
Received: 21 September 2023; Accepted: 17 November 2023;
Published: 05 December 2023.
Edited by:
Michael Winter, Julius Maximilian University of Würzburg, GermanyReviewed by:
Vassiliy Tsytsarev, University of Maryland, United StatesCopyright © 2023 Zhang, Sun, Huang, Qin, Wen, Long, Wang and Feng. This is an open-access article distributed under the terms of the Creative Commons Attribution License (CC BY). The use, distribution or reproduction in other forums is permitted, provided the original author(s) and the copyright owner(s) are credited and that the original publication in this journal is cited, in accordance with accepted academic practice. No use, distribution or reproduction is permitted which does not comply with these terms.
*Correspondence: Quan Wang, d2FuZ3F1YW5Ab3B0LmFjLmNu; Li Feng, ZmVuZ2xpaHhAMTYzLmNvbQ==
†These authors have contributed equally to this work
Disclaimer: All claims expressed in this article are solely those of the authors and do not necessarily represent those of their affiliated organizations, or those of the publisher, the editors and the reviewers. Any product that may be evaluated in this article or claim that may be made by its manufacturer is not guaranteed or endorsed by the publisher.
Research integrity at Frontiers
Learn more about the work of our research integrity team to safeguard the quality of each article we publish.