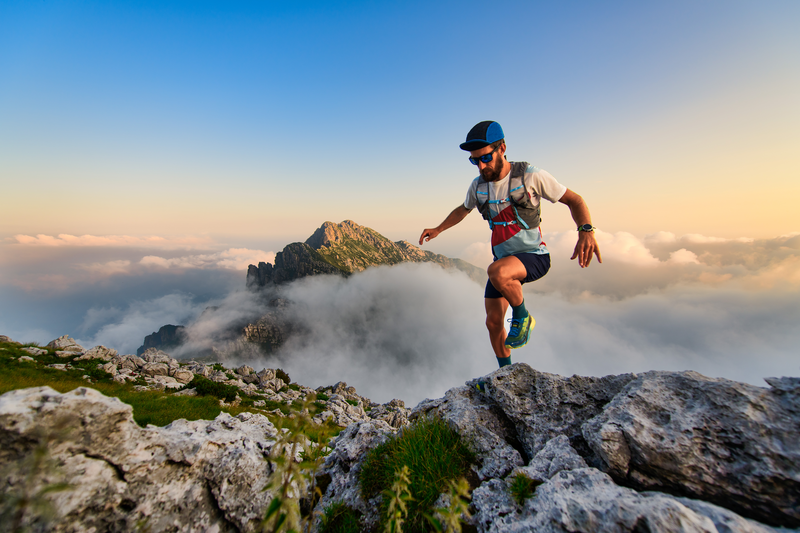
94% of researchers rate our articles as excellent or good
Learn more about the work of our research integrity team to safeguard the quality of each article we publish.
Find out more
ORIGINAL RESEARCH article
Front. Neurosci. , 04 September 2023
Sec. Autonomic Neuroscience
Volume 17 - 2023 | https://doi.org/10.3389/fnins.2023.1256067
This article is part of the Research Topic Understanding the Role of the Autonomic Nervous System in Health and Disease View all 14 articles
Background: Prior research suggests that cardiovascular autonomic dysfunction might be an early marker of cardiotoxicity induced by antitumor treatment and act as an early predictor of cardiovascular disease-related morbidity and mortality. The impact of thoracic radiotherapy on the parasympathetic and sympathetic nervous systems, however, remains unclear. Therefore, this study aimed to evaluate the short-term effects of thoracic radiotherapy on the autonomic nervous system, using deceleration capacity (DC), acceleration capacity (AC) of heart rate, and heart rate variability (HRV) as assessment tools.
Methods: A 5 min electrocardiogram was collected from 58 thoracic cancer patients before and after thoracic radiotherapy for DC, AC, and HRV analysis. HRV parameters employed included the standard deviation of the normal-normal interval (SDNN), root mean square of successive interval differences (RMSSD), low frequency power (LF), high frequency power (HF), total power (TP), and the LF to HF ratio. Some patients also received systemic therapies alongside radiotherapy; thus, patients were subdivided into a radiotherapy-only group (28 cases) and a combined radiotherapy and systemic therapies group (30 cases) for additional subgroup analysis.
Results: Thoracic radiotherapy resulted in a significant reduction in DC (8.5 [5.0, 14.2] vs. 5.3 [3.5, 9.4], p = 0.002) and HRV parameters SDNN (9.9 [7.03, 16.0] vs. 8.2 [6.0, 12.4], p = 0.003), RMSSD (9.9 [6.9, 17.5] vs. 7.7 [4.8, 14.3], p = 0.009), LF (29 [10, 135] vs. 24 [15, 50], p = 0.005), HF (35 [12, 101] vs. 16 [9, 46], p = 0.002), TP (74 [41, 273] vs. 50 [33, 118], p < 0.001), and a significant increase in AC (−8.2 [−14.8, −4.9] vs. -5.8 [−10.1, −3.3], p = 0.003) and mean heart rate (79.8 ± 12.6 vs. 83.9 ± 13.6, p = 0.010). Subgroup analysis indicated similar trends in mean heart rate, DC, AC, and HRV parameters (SDNN, RMSSD, LF, HF, TP) in both the radiotherapy group and the combined treatment group post-radiotherapy. No statistically significant difference was noted in the changes observed in DC, AC, and HRV between the two groups pre- and post-radiotherapy.
Conclusion: Thoracic radiotherapy may induce cardiovascular autonomic dysfunction by reducing parasympathetic activity and enhancing sympathetic activity. Importantly, the study found that the concurrent use of systemic therapies did not significantly amplify or contribute to the alterations in autonomic function in the short-term following thoracic radiotherapy. DC, AC and HRV are promising and feasible biomarkers for evaluating autonomic dysfunction caused by thoracic radiotherapy.
Thoracic radiotherapy serves as a pivotal element in the treatment of thoracic cancer (TC), including breast cancer, esophageal cancer, lung cancer, and other thoracic malignancies (Raghunathan et al., 2017). Existing research has indicated that thoracic radiotherapy can precipitate vascular endothelial dysfunction, leading to accelerated atherosclerosis and inflammatory activation (Sylvester et al., 2018; Venkatesulu et al., 2018; Baselet et al., 2019). Numerous studies have investigated the amplified risk resulting from thoracic radiotherapy, implicating myocardial, coronary, valvular, and pericardial diseases, along with arrhythmias, as factors contributing to increased mortality (Jacob et al., 2016; Armanious et al., 2018). In this context, the cardiovascular autonomic nervous system (ANS)—a key regulator of heart rate, myocardial function, and myocardial blood flow—assumes significant importance. The initiation of cardiovascular toxicity, as well as early indications of diastolic/systolic dysfunction and disease severity, may stem from cardiovascular autonomic dysfunction (AD) caused by radiation and chemotherapy (Tjeerdsma et al., 1999; Guimarães et al., 2015; Teng et al., 2021). Therefore, examining the impact of thoracic radiotherapy on ANS could provide substantial insights into both short and long-term cardiovascular adversities associated with radiotherapy.
The cardiovascular ANS is constituted by the sympathetic nervous system (SNS) and the parasympathetic nervous system (PNS) (Schwartz and De Ferrari, 2011). ANS functionality can be assessed through several clinically viable measures, such as deceleration capacity (DC), acceleration capacity (AC) of heart rate, and heart rate variability (HRV) (Bauer et al., 2006a; Lombardi and Stein, 2011; Zou et al., 2016). Previous research has underscored the prognostic value of DC, AC, and HRV in the early detection of cardiovascular disease onset and sudden cardiac death rates in patients with conditions like myocardial infarction, heart failure, dilated cardiomyopathy, or coronary artery disease (Arsenos et al., 2016; Zou et al., 2016; Wang et al., 2017; Rizas et al., 2018; Fang et al., 2020). More recent observational studies have established that DC is a potent predictor of cardiovascular toxicity stemming from treatments like epirubicin or trastuzumab in breast cancer patients (Feng and Yang, 2015; Feng et al., 2021). Hence, the incorporation of DC, AC, and HRV in clinical studies investigating potential cardiovascular toxicity resulting from antitumor therapy could prove beneficial.
Earlier studies have provided preliminary insights into the effects of antitumor treatment on cardiovascular ANS in patients with malignant tumors (Ekholm et al., 2000; Groarke et al., 2015; Stachowiak et al., 2018; Caru et al., 2019). Early identification of cardiovascular AD has the potential to enhance preventative strategies to mitigate clinically significant cardiac toxicity subsequent to thoracic radiotherapy. It may be necessary to identify novel biomarkers in order to facilitate the early detection of heart damage resulting from radiation exposure. Therefore, the objective of this study is to elucidate the alterations in DC, AC, and HRV before and after thoracic radiotherapy in TC patients, contributing novel insights into the short-term radiotherapy effects on cardiovascular ANS.
This study included TC patients who underwent thoracic radiotherapy at the Department of Tumor Radiotherapy, the First Affiliated Hospital of Bengbu Medical College. Exclusion criteria were as follows: (1) presence of a pacemaker, (2) prior receipt of thoracic radiotherapy, (3) incomplete thoracic radiotherapy, and (4) poor electrocardiogram (ECG) quality. The study was approved by the local hospital’s Clinical Medical Research Ethics Board (registration number: 2019KY031). All participants were voluntary contributors who provided informed consent.
Patients were administered thoracic radiotherapy via a linear accelerator (Siemens or Elekta Synergy Platform) using 6-MV photon beams. The median cumulative radiation dose was 50 Gray, while the median individual radiation dose was 2 Gray. Certain patients also received potentially cardiotoxic systemic therapies, such as taxanes, platinum compounds, or trastuzumab, which may instigate variations in the cardiovascular ANS (Coumbe and Groarke, 2018; Teng et al., 2021). Consequently, we partitioned TC patients into two groups: the radiotherapy-only group, and the combined radiotherapy and systemic therapies group.
An ECG was captured before and after radiotherapy using an ECG recorder (HeaLink-R211B; HeaLink Ltd., Bengbu, China). A V6-lead was employed, and ECGs were collected at a sampling rate of 400 Hz. Participants remained in a supine position, motionless for 5 min during ECG collection. The same operator conducted measurements both pre- and post-radiotherapy.
The Pan-Tompkins algorithm was employed in this study to extract the R-peaks from the ECG readings (Pan and Tompkins, 1985). The Kubios software’s threshold-based automatic artifact correction algorithm was used to rectify both technical and physiological artifacts (Niskanen et al., 2004). The mean heart rate (HR) was defined as the average resting heart rate over a period of 5 min. The phase-rectified signal averaging technique was utilized for the computation of the DC and AC. Initially, the R-R intervals time series were examined to detect the decelerating and accelerating anchors that were characterized by a longer or shorter value than the preceding value, respectively. Subsequently, R-R intervals segments surrounding the decelerating and accelerating anchors were evaluated. Lastly, the aforementioned segments were aligned at the decelerating and accelerating anchors, and the signals of segments were averaged to derive the phase-rectified signal averaging signals (Bauer et al., 2006a,b; Nasario-Junior et al., 2014). Several commonly used HRV parameters were engaged in this study: the standard deviation of the normal-normal intervals (SDNN), root mean square of successive interval differences (RMSSD), low frequency power (LF, 0.04–0.15 Hz), high frequency power (HF, 0.15–0.4 Hz), total power (TP, 0–0.4 Hz), and the ratio of LF to HF (LF/HF). For the frequency domain HRV analysis, R-R interval sequences were transformed into evenly sampled time series using a 4 Hz resampling rate, with the aid of a cubic spline interpolation method. The fast Fourier transform algorithm was applied in tandem with Welch’s periodogram method (150 s window width and 50% overlap window) to calculate HRV spectra.
DC is a quantitative metric of the PNS regulatory ability, while AC symbolizes the SNS tone. SDNN represents the overall variability of HRV, reflecting the combined activity of the PNS and SNS. RMSSD is indicative of vagus nerve activity, LF is influenced by both PNS and SNS, and HF corresponds to the PNS tone. TP is representative of the activities of the PNS and SNS, while the LF/HF ratio indicates the interplay between the SNS and PNS (Bauer et al., 2006a; Vanderlei et al., 2009; Lombardi and Stein, 2011; Zou et al., 2016; Thomas et al., 2019).
All DC, AC, and HRV indicators were analyzed using Kubios HRV Premium software (version 3.5, Magi Kubios Oy, Kuopio, Finland1) (Niskanen et al., 2004).
The Shapiro–Wilk test was applied to verify the normality of the data. The independent sample t-test or Mann–Whitney U test was utilized to compare the differences in each continuous variable, and the Chi-square test was employed to compare the differences in each counting variable among the subgroups before thoracic radiotherapy. The differences in DC, AC, and HRV before and after radiotherapy were analyzed by the Paired Sample t-test or Wilcoxon sign-rank test. Cohen’s d value characterized the effect sizes of the differences in DC, AC, and HRV before and after radiotherapy in the subgroups. The independent sample t-test or Mann–Whitney U test was used to compare the differences in DC, AC, and HRV before and after radiotherapy among the subgroups. All of these statistical analyses were conducted using SPSS Statistics 25.0 (IBM Corp., Chicago, Illinois, United States of America). All tests were two-tailed, with p values of <0.05 considered statistically significant.
A total of 58 patients diagnosed with TC were considered, consisting of 25 males and 33 females, with an average age of 58.6 ± 12.1 years. The prevalence of specific cancer types varied among the participants, with esophageal cancer being the most common (20/58), followed by breast (19/58), lung (16/58), Hodgkin’s lymphoma (1/58), thymic cancer (1/58), and lung metastasis (1/58). The patients were categorized into two cohorts based on their treatment regimen during thoracic radiotherapy: one received solely radiotherapy (28 patients) while the other received a combination of radiotherapy and systemic therapies (30 patients). The overall patient characteristics and a comparison of variables for subgroups pre-radiotherapy are detailed in Table 1.
Table 1. General characteristics of patients with comparisons of variables for subgroups prior to radiotherapy.
Our statistical analysis revealed significant variations in mean HR, DC, AC, SDNN, RMSSD, LF, HF, and TP across all patients when data prior to radiotherapy were compared. Specific changes included a significant decrease in DC (p = 0.002), SDNN (p = 0.003), RMSSD (p = 0.009), LF (p = 0.005), HF (p = 0.002), and TP (p < 0.001) when compared with pre-radiotherapy data. Contrastingly, AC (p = 0.003) and mean HR (p = 0.010) increased post-radiotherapy. A higher LF/HF ratio was observed post-radiotherapy, although this difference was not statistically significant (Table 2).
The subgroup analysis demonstrated that mean HR, DC, AC, as well as HRV parameters SDNN, RMSSD, LF, HF, and TP followed a consistent trend of increase or decrease post-thoracic radiotherapy in both the radiotherapy and combined therapy groups (Figure 1). The effect sizes of DC, AC, and HRV within subgroups are illustrated in Figure 2.
To examine whether the short-term effects of combined thoracic radiotherapy and systemic therapies on the cardiovascular ANS were either synergistic or additive, we analyzed the variations in DC, AC, and HRV before and after radiotherapy for subgroup patients. However, no statistically significant differences were found in the changes of DC, AC, and HRV between the two groups pre- and post-radiotherapy (Figure 3).
Figure 3. Change in DC, AC, and HRV, calculated as post-radiotherapy value minus pre-radiotherapy value for subgroups of patients.
This study evaluated the immediate effects of thoracic radiotherapy on DC, AC, and HRV in TC patients. Our findings demonstrated that thoracic radiotherapy could lead to significant alterations in mean HR, DC, AC, and HRV parameters including SDNN, RMSSD, LF, HF, and TP. Additionally, a subgroup analysis was performed, taking into account patients who were also receiving systemic therapies concurrently with radiotherapy. The outcomes implied that mean HR, DC, AC, SDNN, RMSSD, LF, HF, and TP demonstrated identical trends of increase or decrease post-radiotherapy, in both the radiotherapy-only group and the combined radiotherapy and systemic therapies group. Importantly, there was no substantial difference observed in the alterations of DC, AC, and HRV between the two groups before and after the radiotherapy.
The pathophysiology of cardiovascular AD induced by thoracic radiotherapy and systemic therapies is multifaceted and complex. The primary mechanisms underlying thoracic radiotherapy-associated AD encompass direct neural damage caused by radiation and the pro-inflammatory state inherent in malignancies (Teng et al., 2021). The direct radiation exposure to the vagal nerve and carotid regions may instigate inflammation and subsequent fibrosis, leading to potential damage to the vagal nerve and carotid sinus baroreflexes (Sharabi et al., 2003; Goodman and Schrader, 2009; Kong et al., 2011). An alternate explanation for the incidence of cardiovascular AD in cancer survivors could be the modification of the ANS as a consequence of a pro-inflammatory state, as opposed to intrinsic neural damage. This inflammatory state is frequently observed in cancer settings, particularly at the initiation of radiotherapy, triggering an overproduction of inflammatory cytokines such as interleukin-1, interleukin-6, and tumor necrosis factor-alpha. These factors may suppress vagal nerve activity, which in turn results in an elevation of the resting heart rate and a shift in the balance between the PNS and SNS toward SNS predominance (Thayer and Lane, 2007). Systemic therapies, such as chemotherapy or targeted therapy, may also affect the ANS during and after cancer treatment. The etiology of chemotherapy-induced AD, involving agents like anthracyclines, taxanes, and platinum compounds, may involve inflammatory pathways, cellular injury, and oxidative stress (Coumbe and Groarke, 2018; Teng et al., 2021).
The stability of heart rhythm is primarily determined by the combined effects of the PNS and SNS (Lahiri et al., 2008). Recent research demonstrated that patients with Hodgkin’s lymphoma who underwent thoracic radiotherapy presented a higher mean HR in contrast to those who did not undergo such treatment (Groarke et al., 2015). Moreover, previous findings strongly correlated an elevated mean HR with increased morbidity and mortality rates of cardiovascular diseases (Cooney et al., 2010; Anker et al., 2016; Lee et al., 2016). In this study, thoracic radiotherapy was found to increase the mean HR of all TC patients. A subgroup analysis revealed that patients who underwent radiotherapy demonstrated a higher mean HR post-treatment, although the difference was not statistically significant. This observation suggests that an elevated mean HR could be an efficient, albeit not entirely accurate, indicator for a rapid assessment of cardiovascular AD.
Numerous studies have emphasized the close correlation between neck or chest irradiation and cardiovascular AD (Hoca et al., 2012; Huang et al., 2013; Goyal et al., 2017). For instance, an investigation involving 14 TC patients discovered that mediastinal radiotherapy resulted in a decrease in HF values and an increase in the LF/HF in HRV frequency domain parameters (Hoca et al., 2012). Our research corroborated that thoracic radiotherapy negatively impacts HRV indicators, including SDNN, RMSSD, LF, HF, and TP. These findings suggest that thoracic radiotherapy may contribute to AD by diminishing PNS activity. Nevertheless, our study found no statistically significant difference in LF/HF values pre- and post-radiotherapy, which might be attributable to the complex physiological basis of LF/HF. Pagani et al. (1984) proposed the use of LF/HF to measure cardiac sympatho-vagal balance. However, several studies have contested this approach, asserting that LF/HF fails to accurately quantify the dynamic relationship between SNS and PNS activities and cannot confidently depict the physiological basis of LF/HF (Billman, 2011; Billman, 2013). DC and AC represent emerging noninvasive techniques for evaluating autonomic modulation (Bauer et al., 2006a; Zou et al., 2016). Recent observational studies have shown that DC was an effective predictor of epirubicin-related or trastuzumab-related cardiotoxicity development in patients with breast cancer (Feng and Yang, 2015; Feng et al., 2021). Our study determined that thoracic radiotherapy can significantly decrease DC while simultaneously increasing AC. This indicates that thoracic radiotherapy may lead to a reduction in PNS tone, as reflected by DC, and an increase in SNS activity, as assessed by AC, in TC patients. The changes in DC and AC precipitated by thoracic radiotherapy may correlate with the incidence of cardiovascular disease and sudden cardiac death rates in TC patients post-radiotherapy. However, these potential relationships warrant verification through long-term follow-up studies.
Prior research has established the potential cardiovascular toxicity of taxanes, platinum compounds, anthracyclines, and trastuzumab (Jain et al., 2017; Coumbe and Groarke, 2018; Dong and Chen, 2018). For instance, Dermitzakis et al. (2016) found that concurrent paclitaxel and carboplatin chemotherapy significantly influenced the PNS and SNS in ovarian cancer patients, predominantly affecting parasympathetic heart innervation. Liu et al. (2023) showed that the combination of taxane and carboplatin chemotherapy could impact early ANS status in patients with cervical cancer. Meinardi et al. (2001) discovered that even moderate doses of epirubicin were not associated with persistent alterations in HRV for breast cancer patients, suggesting a possible dose–response relationship for anthracycline-induced ANS damage. Our findings revealed no statistically significant difference in the alterations of DC, AC, and HRV pre- and post-radiotherapy between the radiotherapy-only group and the combined radiotherapy and systemic therapies group. This implies that, in the short term, thoracic radiotherapy coupled with systemic therapies does not significantly exacerbate or add to ANS changes. Currently, while radiotherapy and systemic therapies may exert cardiac toxic effects, the precise mechanism of their interaction remains elusive.
Emerging evidence has shown that patients receiving thoracic radiotherapy have an increased risk for developing cardiovascular disease (Groarke et al., 2015; Jacob et al., 2016; Armanious et al., 2018). According to the American Society of Clinical Oncology’s clinical practice guideline, baseline assessment of the left ventricular ejection fraction could feasibly stratify risk in patients potentially susceptible to cardiovascular toxicity (Armenian et al., 2017). However, relying solely on left ventricular ejection fraction as an indicator of cardiac performance has faced substantial criticism (Konstam and Abboud, 2017; Marwick, 2018). Prior research has demonstrated that cardiovascular ANS function may be a more accurate mortality predictor following myocardial infarction than left ventricular ejection fraction (Bauer et al., 2006a). Additional studies have emphasized the significance of alterations in serum biomarkers, notably cardiac troponin, for forecasting cardiovascular toxicity (Cardinale et al., 2010; Pavo et al., 2015). Therefore, we propose a baseline assessment encompassing ANS function, left ventricular ejection fraction, and troponin measurement for cardiovascular toxicity risk stratification, with subsequent combined measurements for longitudinal cardiac monitoring.
Our study’s primary limitation is the heterogeneity of the study population. Given our limited sample size, it was unattainable to discern the distinct performance of DC, AC, and HRV in patients with varying types of TC. We strongly advocate for future studies to augment the sample size and prioritize these differences (e.g., tumor type, chemotherapy drugs and doses). Furthermore, due to limited statistical power, some background variables potentially influencing cardiovascular ANS, such as physical activity, were omitted.
Our study elucidates the modifications of DC, AC, and HRV pre- and post-thoracic radiotherapy in patients diagnosed with TC. These results suggest that thoracic radiotherapy prompts cardiovascular AD by diminishing PNS activity and augmenting SNS tone. Additionally, the data implies that combining radiotherapy with systemic therapies may not yield a substantial synergetic or additive impact on the ANS in the short term. We hypothesize that the simultaneous measurement of DC, AC, and HRV could be helpful in developing an integrated biomarker for identifying both short- and long-term radiotherapy-induced cardiac autonomic modulation impairments.
The raw data supporting the conclusions of this article will be made available by the authors, without undue reservation.
The studies involving humans were approved by the Medical Ethics Committee of the First Affiliated Hospital of Bengbu Medical College. The studies were conducted in accordance with the local legislation and institutional requirements. The participants provided their written informed consent to participate in this study.
SW: Data curation, Writing – original draft. WG: Formal analysis, Writing – original draft. HZ: Writing – original draft. GL: Writing – original draft. YZ: Resources, Supervision, Writing – review & editing. BS: Conceptualization, Methodology, Resources, Writing – review & editing, XZ: Resources, Supervision, Writing – review & editing.
This research was funded by the “512” Outstanding Talents Fostering Project of Bengbu Medical College (grant number BY51201312), the Natural Science Research Project of Anhui Educational Committee (grant number KJ2021A0803), and the Scientific Research Innovation Project of Bengbu Medical College (grant number BYKC201905).
An immediate family member of BS owns stock in HeaLink Ltd., Bengbu, China.
The remaining authors declare that the research was conducted in the absence of any commercial or financial relationships that could be construed as a potential conflict of interest.
All claims expressed in this article are solely those of the authors and do not necessarily represent those of their affiliated organizations, or those of the publisher, the editors and the reviewers. Any product that may be evaluated in this article, or claim that may be made by its manufacturer, is not guaranteed or endorsed by the publisher.
Anker, M. S., Ebner, N., Hildebrandt, B., Springer, J., Sinn, M., Riess, H., et al. (2016). Resting heart rate is an independent predictor of death in patients with colorectal, pancreatic, and non-small cell lung cancer: results of a prospective cardiovascular long-term study. Eur. J. Heart Fail. 18, 1524–1534. doi: 10.1002/ejhf.670
Armanious, M. A., Mohammadi, H., Khodor, S., Oliver, D. E., Johnstone, P. A., and Fradley, M. G. (2018). Cardiovascular effects of radiation therapy. Curr. Probl. Cancer 42, 433–442. doi: 10.1016/j.currproblcancer.2018.05.008
Armenian, S. H., Lacchetti, C., Barac, A., Carver, J., Constine, L. S., Denduluri, N., et al. (2017). Prevention and monitoring of cardiac dysfunction in survivors of adult cancers: American Society of Clinical Oncology clinical practice guideline. J. Clin. Oncol. 35, 893–911. doi: 10.1200/JCO.2016.70.5400
Arsenos, P., Manis, G., Gatzoulis, K. A., Dilaveris, P., Gialernios, T., Angelis, A., et al. (2016). Deceleration capacity of heart rate predicts arrhythmic and total mortality in heart failure patients. Ann. Noninvasive Electrocardiol. 21, 508–518. doi: 10.1111/anec.12343
Baselet, B., Sonveaux, P., Baatout, S., and Aerts, A. (2019). Pathological effects of ionizing radiation: endothelial activation and dysfunction. Cell. Mol. Life Sci. 76, 699–728. doi: 10.1007/s00018-018-2956-z
Bauer, A., Kantelhardt, J. W., Barthel, P., Schneider, R., Mäkikallio, T., Ulm, K., et al. (2006a). Deceleration capacity of heart rate as a predictor of mortality after myocardial infarction: cohort study. Lancet 367, 1674–1681. doi: 10.1016/S0140-6736(06)68735-7
Bauer, A., Kantelhardt, J. W., Bunde, A., Barthel, P., Schneider, R., Malik, M., et al. (2006b). Phase-rectified signal averaging detects quasi-periodicities in non-stationary data. Physica A. 364, 423–434. doi: 10.1016/j.physa.2005.08.080
Billman, G. E. (2011). Heart rate variability – a historical perspective. Front. Physiol. 2:86. doi: 10.3389/fphys.2011.00086
Billman, G. E. (2013). The LF/HF ratio does not accurately measure cardiac sympatho-vagal balance. Front. Physiol. 4:26. doi: 10.3389/fphys.2013.00026
Cardinale, D., Colombo, A., Torrisi, R., Sandri, M. T., Civelli, M., Salvatici, M., et al. (2010). Trastuzumab-induced cardiotoxicity: clinical and prognostic implications of troponin I evaluation. J. Clin. Oncol. 28, 3910–3916. doi: 10.1200/JCO.2009.27.3615
Caru, M., Corbin, D., Périé, D., Lemay, V., Delfrate, J., Drouin, S., et al. (2019). Doxorubicin treatments induce significant changes on the cardiac autonomic nervous system in childhood acute lymphoblastic leukemia long-term survivors. Clin. Res. Cardiol. 108, 1000–1008. doi: 10.1007/s00392-019-01427-9
Cooney, M. T., Vartiainen, E., Laatikainen, T., Juolevi, A., Dudina, A., and Graham, I. M. (2010). Elevated resting heart rate is an independent risk factor for cardiovascular disease in healthy men and women. Am. Heart J. 159, 612–619.e3. doi: 10.1016/j.ahj.2009.12.029
Coumbe, B. G. T., and Groarke, J. D. (2018). Cardiovascular autonomic dysfunction in patients with cancer. Curr. Cardiol. Rep. 20:69. doi: 10.1007/s11886-018-1010-y
Dermitzakis, E. V., Kimiskidis, V. K., Lazaridis, G., Alexopoulou, Z., Timotheadou, E., Papanikolaou, A., et al. (2016). The impact of paclitaxel and carboplatin chemotherapy on the autonomous nervous system of patients with ovarian cancer. BMC Neurol. 16:190. doi: 10.1186/s12883-016-0710-4
Dong, J., and Chen, H. (2018). Cardiotoxicity of anticancer therapeutics. Front. Cardiovasc. Med. 5:9. doi: 10.3389/fcvm.2018.00009
Ekholm, E. M., Salminen, E. K., Huikuri, H. V., Jalonen, J., Antila, K. J., Salmi, T. A., et al. (2000). Impairment of heart rate variability during paclitaxel therapy. Cancer 88, 2149–2153. doi: 10.1002/(sici)1097-0142(20000501)88:9<2149::aid-cncr22>3.0.co;2-z
Fang, S. C., Wu, Y. L., and Tsai, P. S. (2020). Heart rate variability and risk of all-cause death and cardiovascular events in patients with cardiovascular disease: a meta-analysis of cohort studies. Biol. Res. Nurs. 22, 45–56. doi: 10.1177/1099800419877442
Feng, Y., Qin, Z., and Yang, Z. (2021). Deceleration capacity of heart rate predicts trastuzumab-related cardiotoxicity in patients with HER2-positive breast cancer: a prospective observational study. J. Clin. Pharm. Ther. 46, 93–98. doi: 10.1111/jcpt.13258
Feng, Y. Y., and Yang, Z. J. (2015). Clinical application of the heart rate deceleration capacity test to predict Epirubicin-induced cardiotoxicity. J. Cardiovasc. Pharmacol. 66, 371–375. doi: 10.1097/FJC.0000000000000289
Goodman, B. P., and Schrader, S. L. (2009). Radiation-induced cranial neuropathies manifesting as baroreflex failure and progressive bulbar impairment. Neurologist 15, 102–104. doi: 10.1097/NRL.0b013e31817ba3a6
Goyal, M., Shukla, P., Gupta, D., Bisht, S., Verma, N. S., Tiwari, S., et al. (2017). Cardiovascular sequel of neck irradiation in head and neck cancer patients. Int. J. Radiat. Biol. 93, 711–716. doi: 10.1080/09553002.2017.1303217
Groarke, J. D., Tanguturi, V. K., Hainer, J., Klein, J., Moslehi, J. J., Ng, A., et al. (2015). Abnormal exercise response in long-term survivors of hodgkin lymphoma treated with thoracic irradiation: evidence of cardiac autonomic dysfunction and impact on outcomes. J. Am. Coll. Cardiol. 65, 573–583. doi: 10.1016/j.jacc.2014.11.035
Guimarães, S. L., Brandão, S. C., Andrade, L. R., Maia, R. J., and Markman Filho, B. (2015). Cardiac sympathetic hyperactivity after chemotherapy: early sign of cardiotoxicity? Arq. Bras. Cardiol. 105, 228–234. doi: 10.5935/abc.20150075
Hoca, A., Yildiz, M., and Ozyigit, G. (2012). Evaluation of the effects of mediastinal radiation therapy on autonomic nervous system. Med. Oncol. 29, 3581–3586. doi: 10.1007/s12032-012-0237-5
Huang, C. C., Huang, T. L., Hsu, H. C., Chen, H. C., Lin, H. C., Chien, C. Y., et al. (2013). Long-term effects of neck irradiation on cardiovascular autonomic function: a study in nasopharyngeal carcinoma patients after radiotherapy. Muscle Nerve 47, 344–350. doi: 10.1002/mus.23530
Jacob, S., Pathak, A., Franck, D., Latorzeff, I., Jimenez, G., Fondard, O., et al. (2016). Early detection and prediction of cardiotoxicity after radiation therapy for breast cancer: the BACCARAT prospective cohort study. Radiat. Oncol. 11:54. doi: 10.1186/s13014-016-0627-5
Jain, D., Russell, R. R., Schwartz, R. G., Panjrath, G. S., and Aronow, W. (2017). Cardiac complications of cancer therapy: pathophysiology, identification, prevention, treatment, and future directions. Curr. Cardiol. Rep. 19:36. doi: 10.1007/s11886-017-0846-x
Kong, L., Lu, J. J., Liss, A. L., Hu, C., Guo, X., Wu, Y., et al. (2011). Radiation-induced cranial nerve palsy: a cross-sectional study of nasopharyngeal cancer patients after definitive radiotherapy. Int. J. Radiat. Oncol. Biol. Phys. 79, 1421–1427. doi: 10.1016/j.ijrobp.2010.01.002
Konstam, M. A., and Abboud, F. M. (2017). Ejection fraction: misunderstood and overrated (changing the paradigm in categorizing heart failure). Circulation 135, 717–719. doi: 10.1161/CIRCULATIONAHA.116.025795
Lahiri, M. K., Kannankeril, P. J., and Goldberger, J. J. (2008). Assessment of autonomic function in cardiovascular disease: physiological basis and prognostic implications. J. Am. Coll. Cardiol. 51, 1725–1733. doi: 10.1016/j.jacc.2008.01.038
Lee, D. H., Park, S., Lim, S. M., Lee, M. K., Giovannucci, E. L., Kim, J. H., et al. (2016). Resting heart rate as a prognostic factor for mortality in patients with breast cancer. Breast Cancer Res. Treat. 159, 375–384. doi: 10.1007/s10549-016-3938-1
Liu, J., Guan, W., Sun, Y., Wang, Y., Li, G., Zhang, S., et al. (2023). Early detection of the impact of combined taxane and carboplatin treatment on autonomic nerves in patients with cervical cancer: measurement of heart rate variability. Front. Physiol. 14:1126057. doi: 10.3389/fphys.2023.1126057
Lombardi, F., and Stein, P. K. (2011). Origin of heart rate variability and turbulence: an appraisal of autonomic modulation of cardiovascular function. Front. Physiol. 2:95. doi: 10.3389/fphys.2011.00095
Marwick, T. H. (2018). Ejection fraction pros and cons: JACC state-of-the-art review. J. Am. Coll. Cardiol. 72, 2360–2379. doi: 10.1016/j.jacc.2018.08.2162
Meinardi, M. T., van Veldhuisen, D. J., Gietema, J. A., Dolsma, W. V., Boomsma, F., van den Berg, M. P., et al. (2001). Prospective evaluation of early cardiac damage induced by epirubicin-containing adjuvant chemotherapy and locoregional radiotherapy in breast cancer patients. J. Clin. Oncol. 19, 2746–2753. doi: 10.1200/JCO.2001.19.10.2746
Nasario-Junior, O., Benchimol-Barbosa, P. R., and Nadal, J. (2014). Refining the deceleration capacity index in phase-rectified signal averaging to assess physical conditioning level. J. Electrocardiol. 47, 306–310. doi: 10.1016/j.jelectrocard.2013.12.006
Niskanen, J. P., Tarvainen, M. P., Ranta-Aho, P. O., and Karjalainen, P. A. (2004). Software for advanced HRV analysis. Comput. Methods Progr. Biomed. 76, 73–81. doi: 10.1016/j.cmpb.2004.03.004
Pagani, M., Lombardi, F., Guzzetti, S., Sandrone, G., Rimoldi, O., Malfatto, G., et al. (1984). Power spectral density of heart rate variability as an index of sympatho-vagal interaction in normal and hypertensive subjects. J. Hypertens. Suppl. 2, S383–S385.
Pan, J., and Tompkins, W. J. (1985). A real-time QRS detection algorithm. IEEE Trans. Biomed. Eng. BME-32, 230–236. doi: 10.1109/TBME.1985.325532
Pavo, N., Raderer, M., Hülsmann, M., Neuhold, S., Adlbrecht, C., Strunk, G., et al. (2015). Cardiovascular biomarkers in patients with cancer and their association with all-cause mortality. Heart 101, 1874–1880. doi: 10.1136/heartjnl-2015-307848
Raghunathan, D., Khilji, M. I., Hassan, S. A., and Yusuf, S. W. (2017). Radiation-induced cardiovascular disease. Curr. Atheroscler. Rep. 19:22. doi: 10.1007/s11883-017-0658-x
Rizas, K. D., Eick, C., Doller, A. J., Hamm, W., von Stuelpnagel, L., Zuern, C. S., et al. (2018). Bedside autonomic risk stratification after myocardial infarction by means of short-term deceleration capacity of heart rate. Europace 20, f129–f136. doi: 10.1093/europace/eux167
Schwartz, P. J., and De Ferrari, G. M. (2011). Sympathetic-parasympathetic interaction in health and disease: abnormalities and relevance in heart failure. Heart Fail. Rev. 16, 101–107. doi: 10.1007/s10741-010-9179-1
Sharabi, Y., Dendi, R., Holmes, C., and Goldstein, D. S. (2003). Baroreflex failure as a late sequela of neck irradiation. Hypertension 42, 110–116. doi: 10.1161/01.HYP.0000077441.45309.08
Stachowiak, P., Milchert-Leszczyńska, M., Falco, M., Wojtarowicz, A., Kaliszczak, R., Safranow, K., et al. (2018). Heart rate variability during and after chemotherapy with anthracycline in patients with breast cancer. Kardiol. Pol. 76, 914–916. doi: 10.5603/KP.2018.0098
Sylvester, C. B., Abe, J. I., Patel, Z. S., and Grande-Allen, K. J. (2018). Radiation-induced cardiovascular disease: mechanisms and importance of linear energy transfer. Front. Cardiovasc. Med. 5:5. doi: 10.3389/fcvm.2018.00005
Teng, A. E., Noor, B., Ajijola, O. A., and Yang, E. H. (2021). Chemotherapy and radiation-associated cardiac autonomic dysfunction. Curr. Oncol. Rep. 23:14. doi: 10.1007/s11912-020-01013-7
Thayer, J. F., and Lane, R. D. (2007). The role of vagal function in the risk for cardiovascular disease and mortality. Biol. Psychol. 74, 224–242. doi: 10.1016/j.biopsycho.2005.11.013
Thomas, B. L., Claassen, N., Becker, P., and Viljoen, M. (2019). Validity of commonly used heart rate variability markers of autonomic nervous system function. Neuropsychobiology 78, 14–26. doi: 10.1159/000495519
Tjeerdsma, G., Meinardi, M. T., van Der Graaf, W. T., van Den Berg, M. P., Mulder, N. H., Crijns, H. J., et al. (1999). Early detection of anthracycline induced cardiotoxicity in asymptomatic patients with normal left ventricular systolic function: autonomic versus echocardiographic variables. Heart 81, 419–423. doi: 10.1136/hrt.81.4.419
Vanderlei, L. C., Pastre, C. M., Hoshi, R. A., Carvalho, T. D., and Godoy, M. F. (2009). Basic notions of heart rate variability and its clinical applicability. Rev. Bras. Cir. Cardiovasc. 24, 205–217. doi: 10.1590/s0102-76382009000200018
Venkatesulu, B. P., Mahadevan, L. S., Aliru, M. L., Yang, X., Bodd, M. H., Singh, P. K., et al. (2018). Radiation-induced endothelial vascular injury: a review of possible mechanisms. JACC Basic Transl. Sci. 3, 563–572. doi: 10.1016/j.jacbts.2018.01.014
Wang, C. G., Luo, X. C., Yao, T., Liu, Y. Y., Zhao, S. H., Cheng, J. Y., et al. (2017). Clinical value of DC and DRs in warning sudden cardiac death of patients with coronary artery disease. Zhongguo Ying Yong Sheng Li Xue Za Zhi 33, 244–247. doi: 10.12047/j.cjap.5509.2017.060
Keywords: autonomic modulation, cardiovascular toxicity, deceleration/acceleration capacities of heart rate, heart rate variability, thoracic radiotherapy
Citation: Wu S, Guan W, Zhao H, Li G, Zhou Y, Shi B and Zhang X (2023) Assessment of short-term effects of thoracic radiotherapy on the cardiovascular parasympathetic and sympathetic nervous systems. Front. Neurosci. 17:1256067. doi: 10.3389/fnins.2023.1256067
Received: 10 July 2023; Accepted: 22 August 2023;
Published: 04 September 2023.
Edited by:
Vitor Engracia Valenti, São Paulo State University, BrazilReviewed by:
Felipe Ribeiro, São Paulo State University, BrazilCopyright © 2023 Wu, Guan, Zhao, Li, Zhou, Shi and Zhang. This is an open-access article distributed under the terms of the Creative Commons Attribution License (CC BY). The use, distribution or reproduction in other forums is permitted, provided the original author(s) and the copyright owner(s) are credited and that the original publication in this journal is cited, in accordance with accepted academic practice. No use, distribution or reproduction is permitted which does not comply with these terms.
*Correspondence: Xiaochun Zhang, Y2VpcUBzaW5hLmNvbQ==; Bo Shi, c2hpYm9AYmJtYy5lZHUuY24=
Disclaimer: All claims expressed in this article are solely those of the authors and do not necessarily represent those of their affiliated organizations, or those of the publisher, the editors and the reviewers. Any product that may be evaluated in this article or claim that may be made by its manufacturer is not guaranteed or endorsed by the publisher.
Research integrity at Frontiers
Learn more about the work of our research integrity team to safeguard the quality of each article we publish.