- 1Department of Neurosurgery, The First Affiliated Hospital of Nanjing Medical University, Nanjing, China
- 2Department of Neurosurgery, The Affiliated Huaian No. 1 People's Hospital of Nanjing Medical University, Huaian, Jiangsu, China
- 3Department of Rehabilitation Medicine, The Affiliated Lianyungang Oriental Hospital of Kangda College of Nanjing Medical University, Lianyungang, Jiangsu, China
- 4Department of Neurosurgery, Sir Run Run Hospital, Nanjing Medical University, Nanjing, China
Background: Cognitive impairment is a common sequela following traumatic brain injury (TBI). This study aimed to identify risk factors for cognitive impairment after 3 and 12 months of TBI and to create nomograms to predict them.
Methods: A total of 305 mild-to-moderate TBI patients admitted to the First Affiliated Hospital with Nanjing Medical University from January 2018 to January 2022 were retrospectively recruited. Risk factors for cognitive impairment after 3 and 12 months of TBI were identified by univariable and multivariable logistic regression analyses. Based on these factors, we created two nomograms to predict cognitive impairment after 3 and 12 months of TBI, the discrimination and calibration of which were validated by plotting the receiver operating characteristic (ROC) curve and calibration curve, respectively.
Results: Cognitive impairment was detected in 125/305 and 52/305 mild-to-moderate TBI patients after 3 and 12 months of injury, respectively. Age, the Glasgow Coma Scale (GCS) score, >12 years of education, hyperlipidemia, temporal lobe contusion, traumatic subarachnoid hemorrhage (tSAH), very early rehabilitation (VER), and intensive care unit (ICU) admission were independent risk factors for cognitive impairment after 3 months of mild-to-moderate TBI. Meanwhile, age, GCS score, diabetes mellitus, tSAH, and surgical treatment were independent risk factors for cognitive impairment after 12 months of mild-to-moderate TBI. Two nomograms were created based on the risk factors identified using logistic regression analyses. The areas under the curve (AUCs) of the two nomograms to predict cognitive impairment after 3 and 12 months of mild-to-moderate TBI were 0.852 (95% CI [0.810, 0.895]) and 0.817 (95% CI [0.762, 0.873]), respectively.
Conclusion: Two nomograms are created to predict cognitive impairment after 3 and 12 months of TBI. Age, GCS score, >12 years of education, hyperlipidemia, temporal lobe contusion, tSAH, VER, and ICU admission are independent risk factors for cognitive impairment after 3 months of TBI; meanwhile, age, the GCS scores, diabetes mellitus, tSAH, and surgical treatment are independent risk factors of cognitive impairment after 12 months of TBI. Two nomograms, based on both groups of factors, respectively, show strong discriminative abilities.
Introduction
New cases of traumatic brain injury (TBI) are estimated to be 20 million per year, posing a heavy global burden of disease (Injury and Spinal Cord Injury, 2019). Based on the Glasgow Coma Scale (GCS) scores, TBI is classified as mild-to-moderate (GCS, 9–15 points) and severe (GCS,≤8 points). The incidence of mild-to-moderate TBI is much higher than that of severe TBI. Despite its low mortality, mild-to-moderate TBI still causes multiple neurological deficits in survivors (Pavlovic et al., 2019).
Cognitive impairment, a common type of neurological dysfunction after mild-to-moderate TBI, markedly worsens the quality of life and the long-term neurological outcomes in survivors (McHugh et al., 2006). Cognitive impairment after mild-to-moderate TBI is usually manifested as the impairment of executive function, memory, attention, speech, and naming (Miotto et al., 2010; Panwar et al., 2019). Cognitive function can be recovered in some TBI patients within 1–3 months post-TBI, but cognitive impairment may stay for a long term in some cases (McHugh et al., 2006). A previous study has reported that 19.2% of mild TBI patients and 39.3% of moderate TBI patients still experience cognitive impairment after 3 months of injury (Othman et al., 2022). Draper and Ponsford (2008) have revealed that cognitive impairment may persist in some patients even after 10 years of TBI, the degree of which is positively correlated with the severity of the injury.
Post-TBI cognitive impairment has been well-studied. At present, therapeutic strategies mainly include medications, rehabilitation exercises, and transcranial magnetic stimulation (TMS) (Neville et al., 2015; Jenkins et al., 2019; Martinez-Molina et al., 2022). However, efficient predictive factors for identifying high-risk populations are scant, so intervention strategies are not started promptly. In the present retrospective study, we analyzed risk factors for cognitive impairment after 3 and 12 months of mild-to-moderate TBI. Based on these factors, two nomograms were created to predict cognitive impairment in mild-to-moderate TBI patients after 3 and 12 months of injury.
Methods
Subjects
Mild-to-moderate TBI patients admitted to the Neurosurgery Department, the First Affiliated Hospital with Nanjing Medical University from January 2018 to January 2022 were retrospectively recruited. The inclusion criteria were as follows: (i) aged over 16 years old and under 90 years old; (ii) an interval time <24 h from TBI to admission; (iii) the lowest score of GCS at admission, 3 h after admission, and 6 h after admission was ≥9; and (iv) computed tomography (CT) of the head performed within 6 h of admission and 48 h before discharge. The exclusion criteria were as follows: (i) death during hospitalization; (ii) severe compound injuries; (iii) history of mental illnesses or cognitive impairments; (iv) hormonal disorders during the course of disease; and (v) status epilepticus during the course of the disease. Written informed consent was obtained from all subjects or their guardians. This study was approved by the Ethics Committee of the First Affiliated Hospital of Nanjing Medical University (No. 2022-SR-354).
Data collection
The following data were recorded: (i) baseline characteristics, including sex, age, and years of education; (ii) medical history, including the history of hypertension, diabetes mellitus, and hyperlipidemia; (iii) clinical characteristics of TBI, including the GCS score and causes of injury; (iv) imaging features of TBI, including injury site (right, left, and both); contusions in the temporal, frontal, parietal, and occipital lobes; epidural, subdural, or traumatic subarachnoid hemorrhage (tSAH); and subdural effusion on discharge; and (v) treatment, including surgical treatment, ICU admission, and very early rehabilitation (VER). VER was defined as rehabilitation exercises that commenced within 3 days after admission or immediately after postoperative vital signs were stable. The GCS score was selected and recorded as the lowest score of GCS at admission, 3 h after admission, and 6 h after admission. The computed tomography (CT) examination of the TBI patient showed the presence of high-density shadow in the subarachnoid space, which was defined as tSAH. Subdural effusion was defined as effusion that appeared within 10 days of TBI with a similar uniform low-density area, width >3 mm, and CT value <20 Hu. ICU admission was defined as treatment in the intensive care unit during hospitalization regardless of the length of stay in the ICU.
Assessment of cognitive impairment
The survivors of mild-to-moderate TBI patients were followed up for 12 months after the injury. A widely used tool to detect cognitive impairment with high sensitivity and specificity, the Montreal Cognitive Assessment (MoCA), was performed at 3 and 12 months post-TBI. The MoCA score increased by 1 point when traumatic brain injury patients had <12 years of education. Ranging from 0 to 30 points, a lower MoCA score indicated a worse cognitive function (Nasreddine et al., 2005). In the present study, cognitive impairment was defined as those with a maximal MoCA score of 26 points.
Statistical analysis
Categorical variables were expressed as number of cases (n) and percentage (%). Continuous variables in a normal distribution were expressed as mean ± standard deviation ( SD); otherwise, they were expressed as median (M) and interquartile boundary values (P25, P75). Variables with a p-value of <0.10 in the univariable logistic regression model were introduced into the multivariable logistic regression model using the backward elimination method. The odds ratio (OR) and the corresponding 95% confidence interval (95% CI) and p-value were calculated. Nomograms were created using an R package by incorporating variables with a p-value of <0.05 identified using the multivariable logistic regression analysis. The discriminative ability of the nomogram was assessed by plotting the receiver operating characteristic (ROC) curve and calculating the C-statistics, which was equal to the area under the curve (AUC). The C-statistics ranged from 0.5 to 1.0, and a higher C-statistics indicated better discrimination of the nomogram. Internal validation of the nomogram was performed within 1,000 bootstrap resampling. A linear calibration curve indicated an acceptable goodness-of-fit of the nomogram. Statistical analysis was performed using SPSS 23.0 and packages of rms, readr, pROC, formula, and ggplot2 in R, and figures were programmatically created using R 3.6.1. A p-value of <0.05 was considered statistically significant.
Results
Clinical characteristics of subjects
A total of 365 patients with mild-to-moderate TBI were recruited. After excluding 12 deaths and 48 subjects who were lost to follow-up, 305 eligible patients were finally included in this study, including 190 with mild TBI (GCS 13–15 points) and 115 with moderate TBI (GCS 9–12 points).
After 3 months of TBI, 125 (41.0%) patients developed cognitive impairment. The incidences of cognitive impairment in mild and moderate TBI patients after 3 months of injury were 28.9% and 60.9%, respectively. Later, 52 (17.0%) TBI patients still suffered from cognitive impairment after 12 months of injury, with incidences of cognitive impairment in mild and moderate TBI patients of 8.4% and 31.3%, respectively.
Independent risk factors for cognitive impairment after 3 and 12 months of mild-to-moderate TBI
The univariable logistic regression analysis revealed that age (P < 0.001), GCS scores (P < 0.001), >12 years of education (P < 0.001), hyperlipidemia (P < 0.001), injury side (P = 0.005), temporal lobe contusion (P < 0.001), subdural hematoma (P < 0.001), tSAH (P < 0.001), surgical treatment (P = 0.016), subdural effusion (P = 0.035), and ICU admission (P < 0.001) were significantly correlated with cognitive impairment after 3 months of mild-to-moderate TBI (Table 1). In addition, age (P = 0.003), GCS score (P < 0.001), >12 years of education (P = 0.047), diabetes mellitus (P = 0.005), hyperlipidemia (P = 0.004), injury side (bilateral sides vs. right side, P = 0.045), temporal lobe contusion (P = 0.001), subdural hematoma (P = 0.003), tSAH (P < 0.001), surgical treatment (P = 0.001), subdural effusion (P = 0.015), and ICU admission (P < 0.001) were significantly correlated with cognitive impairment after 12 months of mild-to-moderate TBI (Table 2).
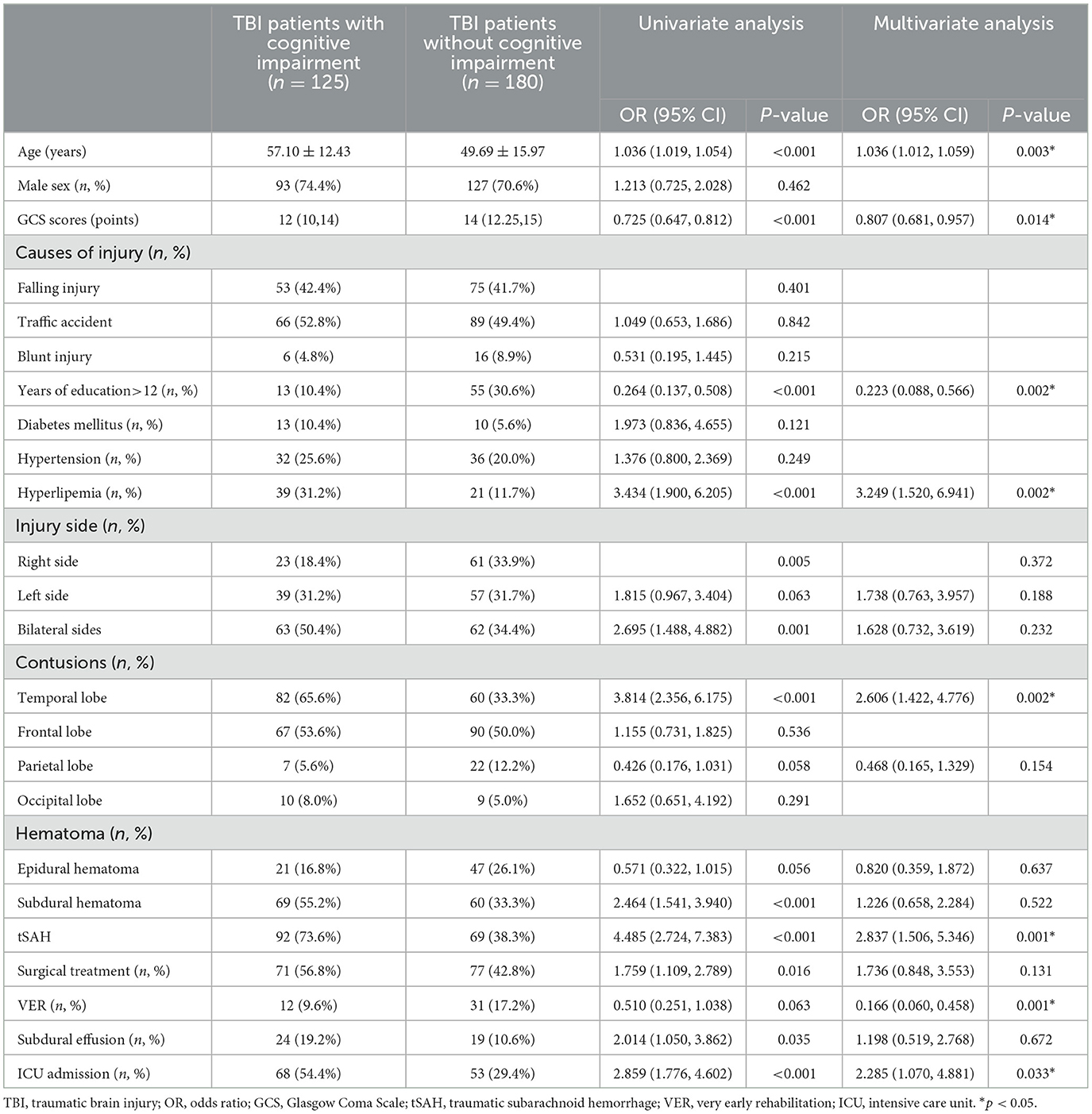
Table 1. Univariable and multivariable logistic regression analyses on cognitive impairment after 3 months of mild-to-moderate TBI (n = 305).
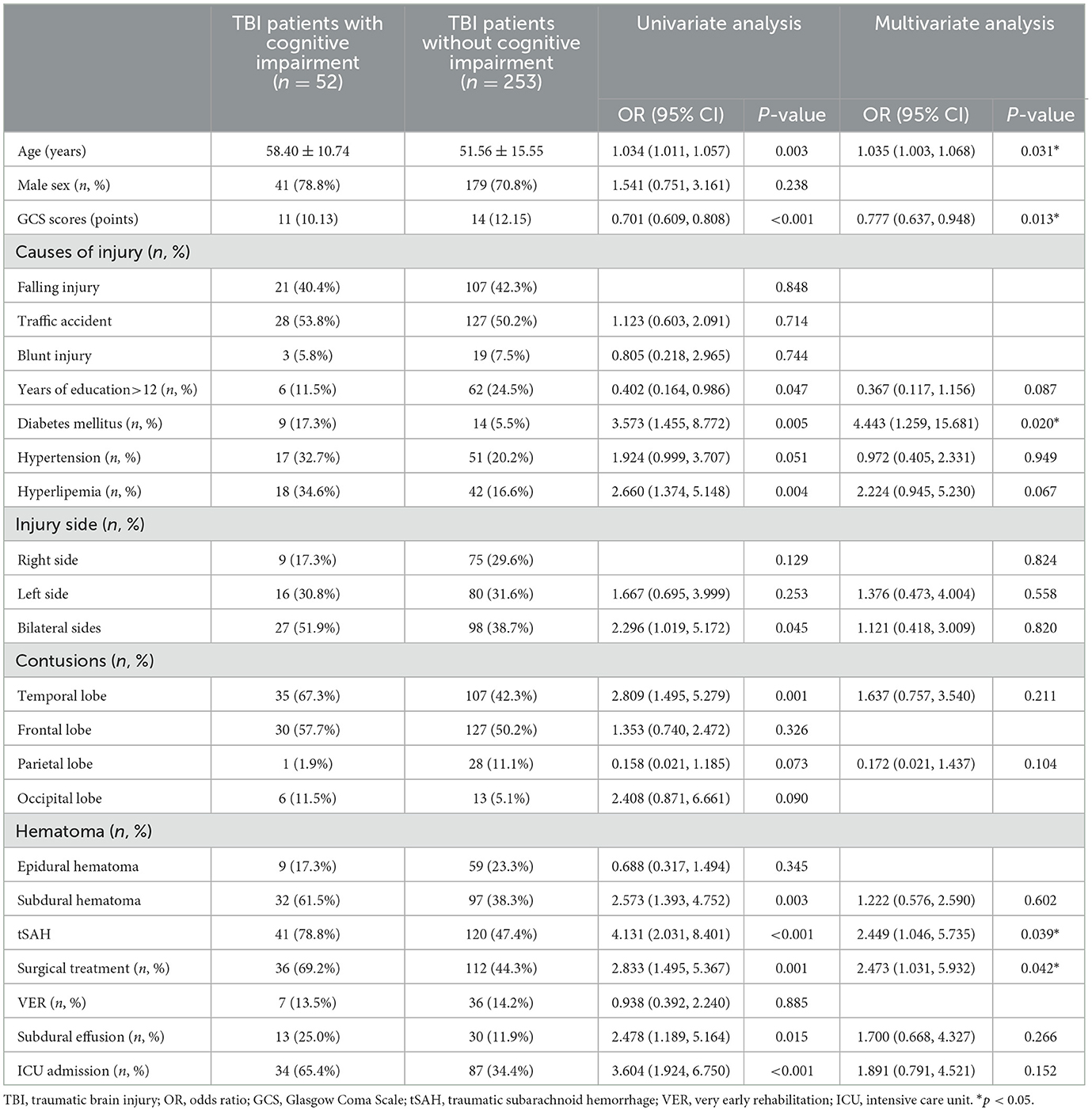
Table 2. Univariable and multivariable logistic regression analyses on cognitive impairment after 12 months of mild-to-moderate TBI (n = 305).
We later introduced variables with a p-value of <0.10 identified using the univariable logistic regression analysis into the multivariable logistic regression analysis. Age (OR, 1.036; 95% CI [1.012,1.059]; P = 0.003), GCS scores (OR, 0.807; 95% CI [0.681, 0.957]; P = 0.014), >12 years of education (OR, 0.223; 95% CI [0.088, 0.566]; P = 0.002), hyperlipidemia (OR, 3.249; 95% CI [1.520, 6.941]; P = 0.002), temporal lobe contusions (OR, 2.606; 95% CI [1.422, 4.776]; P = 0.002), tSAH (OR, 2.837; 95% CI [1.506, 5.346]; P = 0.001), VER (OR, 0.166; 95% CI [0.060, 0.458]; P = 0.001), and ICU admission (OR, 2.285; 95% CI [1.070, 4.881]; P = 0.033) were independent risk factors for cognitive impairment after 3 months of mild-to-moderate TBI (Table 1). In addition, age (OR, 1.035; 95% CI [1.003, 1.068]; P = 0.031), GCS scores (OR, 0.777; 95% CI [0.637, 0.948]; P = 0.013), diabetes mellitus (OR, 4.443; 95% CI [1.259, 15.681]; P = 0.020), tSAH (OR, 2.449; 95% CI [1.046, 5.735]; P = 0.039), and surgical treatment (OR, 2.473; 95% CI [1.031, 5.932]; P = 0.042) were independent risk factors for cognitive impairment after 12 months of mild-to-moderate TBI (Table 2).
Two nomograms to predict cognitive impairment after 3 and 12 months of mild-to-moderate TBI
Two nomograms were then created based on risk factors identified using the multivariable logistic regression analysis to predict cognitive impairment after 3 and 12 months of mild-to-moderate TBI (Figures 1, 2). The total score was the sum of the points for each covariate in the nomogram and corresponded to the predicted probability of the outcome of interest. The AUCs of the nomogram to predict cognitive impairment after 3 and 12 months of mild-to-moderate TBI were 0.852 (95% CI [0.810, 0.895]) and 0.817 (95% CI [0.762, 0.873]), respectively, suggesting a good discriminative ability of the two nomograms (Figures 3, 4). The C-statistics of a nomogram to predict cognitive impairment after 3 months of mild-to-moderate TBI was 0.834 by internal validation using bootstrapping with 1,000 iterations. The C-statistics of a nomogram to predict cognitive impairment after 12 months of mild-to-moderate TBI was 0.799 after bootstrapping. The actual and predicted probabilities of cognitive impairment on the Y-axis and X-axis were plotted, respectively. The calibration curves showed an acceptable goodness-of-fit of the nomograms (Figures 5, 6).
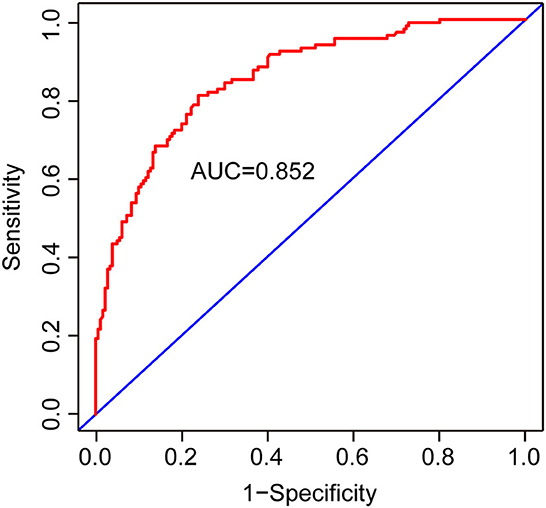
Figure 3. ROC curves to validate the discrimination of the nomogram predicting cognitive impairment 3 months after mild-to-moderate TBI.
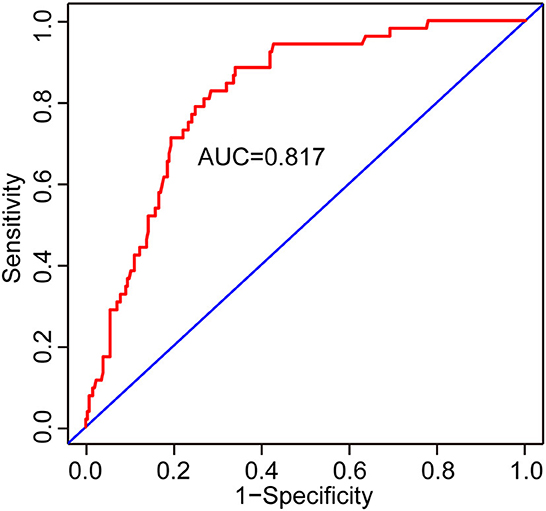
Figure 4. ROC curves to validate the discrimination of the nomogram predicting cognitive impairment at 12 months after mild-to-moderate TBI.
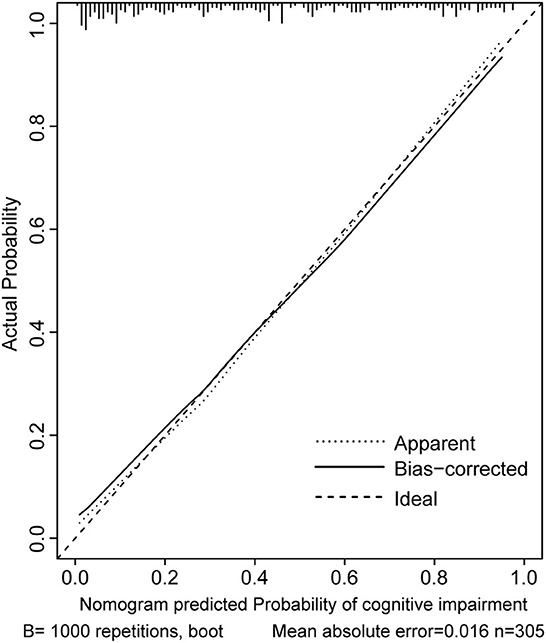
Figure 5. Calibration curves of the nomogram for predicting cognitive impairment at 3 months after mild-to-moderate TBI.
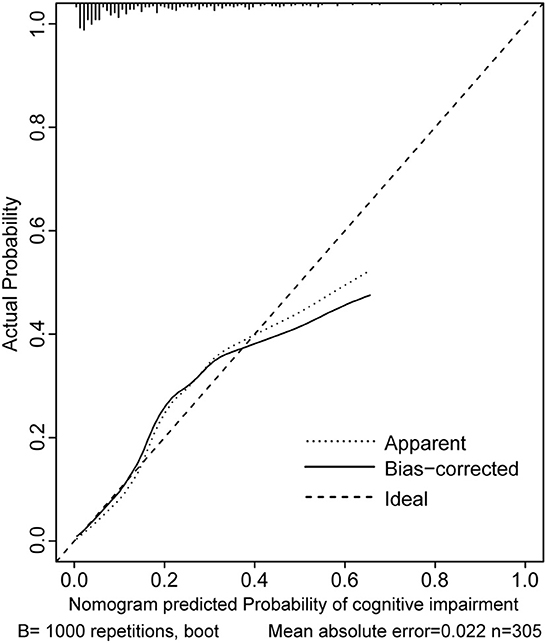
Figure 6. Calibration curves of the nomogram for predicting cognitive impairment at 12 months after mild-to-moderate TBI.
Discussion
Cognitive impairment is a common complication following mild-to-moderate TBI, which may last for a long term. de Boussard et al. (2005) have reported that 26% of mild TBI patients develop cognitive impairment 3 months later. Consistently, Skandsen et al. (2010) have illustrated that 43% of moderate TBI patients have cognitive impairment at 3 months post-TBI. An observational study in Malaysia has shown that 19.2% of mild TBI patients and 39.3% of moderate TBI patients presented cognitive impairment after 3 months of injury (Othman et al., 2022). In the present study, the incidences of cognitive impairment after 3 months of mild and moderate TBI were 28.9% and 60.9%, respectively. Both higher incidences, compared to those previously reported, may be attributed to the differences in age, cause of injury, and injury site. Few previous studies have focused on cognitive impairment 1 year after mild-to-moderate TBI. Schneider et al. (2022) have reported that 10.1% of mild TBI patients have cognitive impairment even after 1 year of injury. Similarly, our data revealed that the incidence of cognitive impairment after 12 months of mild-to-moderate TBI was 17.0%, i.e., 8.4% in mild and 31.3% in moderate TBI patients.
We later created two nomograms to predict cognitive impairment in mild-to-moderate TBI patients after 3 and 12 months of TBI, based on independent risk factors identified using the multivariable logistic regression analysis. Through a retrospective analysis of the clinical data of 305 mild-to-moderate TBI patients, we found that older age, low GCS score, hyperlipidemia, temporal lobe contusion, tSAH, and ICU admission were independent risk factors for cognitive impairment after 3 months of mild-to-moderate TBI, while >12 years of education and VER were protective factors. In addition, older age, low GCS score, diabetes mellitus, tSAH, and surgical treatment were independent risk factors for cognitive impairment after 12 months of mild-to-moderate TBI.
Age, GCS score, and tSAH were all closely linked with cognitive impairment at 3 and 12 months after mild-to-moderate TBI. Leukoaraiosis and poor neuroplasticity in elderly patients can increase the risk of cognitive impairment after TBI (Nguyen et al., 2019). Both aging and TBI cause the loss of brain volume and a decline of white matter integrity, and their additive effect, notably, prolongs the negative influence of cognitive impairment on elderly patients with TBI (Farbota et al., 2012; Arenth et al., 2014; Kim et al., 2021). The hippocampus is a region responsible for cognitive function, especially memory function. Biological functions of the hippocampus can be largely impaired by subarachnoid hemorrhage, the subsequent middle cerebral artery spasm can reduce blood supply, block synaptic neurotransmission, and damage plasticity (Tariq et al., 2010; Regnier-Golanov et al., 2022). Neuroinflammation and oxidative stress in hippocampal neurons secondary to subarachnoid hemorrhage also contribute to cognitive impairment (Han et al., 2014; Hu et al., 2021). In patients with aneurysmal subarachnoid hemorrhage, abnormal changes in the microstructure of the white matter result in cognitive impairment 3 months after the onset (Reijmer et al., 2018).
Cognitive outcomes vary a lot after contusion and hemorrhage in different brain regions. Martin et al. (2017) have demonstrated that the volume of frontal lobe contusion is not linked with cognitive outcomes, while a larger volume of temporal lobe contusion predicts a worse cognitive function after 6 months of injury. A close correlation is identified between the hemorrhage site and the incidence of dementia within 6 months of cerebral hemorrhage rather than delayed dementia after 6 months (Biffi et al., 2016). Our results showed that mild-to-moderate TBI patients with temporal lobe contusions had a higher risk of cognitive impairment at 3 months post-TBI, but cognitive impairment at 12 months was not significantly correlated with the site of contusion.
Hypertension, diabetes mellitus, and hyperlipidemia have not been the focus of research concerning cognitive impairment following TBI. Our data demonstrated that hyperlipidemia and diabetes mellitus were independent risk factors of cognitive impairment 3 and 12 months after mild-to-moderate TBI, respectively. The risk of cognitive impairment in patients with diabetes mellitus is 1.5–2 times higher than that in patients without diabetes mellitus (Cukierman et al., 2005). Lachmann et al. (2018) have found that diabetes mellitus is linked with an increased risk of postoperative cognitive impairment rather than hypertension. The negative influence of diabetes mellitus on cognitive function can be attributed to hippocampal atrophy and cerebral microvascular damage (van Elderen et al., 2010; Hayashi et al., 2011; Vuletic et al., 2013). Hyperlipidemia increases the incidence of carotid intimal thickening, and meanwhile, TBI may accelerate the process of atherosclerosis (Wang et al., 2018). A synergic effect of hyperlipidemia and TBI causes carotid atherosclerosis, and the subsequent cerebrovascular insufficiency or cerebral microinfarct poses a long-term impact on cognitive function.
Mild-to-moderate TBI patients who were admitted to the ICU were more likely to have cognitive impairment 3 months after TBI than those without an ICU admission, which may be linked with ICU-acquired delirium. A much higher incidence of delirium is detected in patients admitted to the ICU, which in TBI patients, can be as high as 60% (Wilson et al., 2023). Delirium is one of the important causes of long-term cognitive decline (Goldberg et al., 2020).
We further found that >12 years of education and VER were identified as protective factors for cognitive impairment at 3 months after mild-to-moderate TBI, which, however, did not influence cognitive function at 12 months. A high level of education provides a strong cognitive reserve to cope with TBI-induced physical and psychological challenges (Almeida-Meza et al., 2022). Cognitive impairment following TBI is found to be associated with the level of apolipoprotein E ε4 (ApoE-ε4), which reduces brain metabolism in the medial temporal and prefrontal lobe of TBI patients (Hellstrøm et al., 2022). Interestingly, a high level of education is able to reverse the negative influence of ApoE-ε4 on brain metabolism (Arenaza-Urquijo et al., 2015). VER is an emerging concept of rehabilitation. Currently, clinical data supporting the role of VER in TBI are controversial. In a retrospective cohort study involving acute stroke patients in Japan, VER is validated to reduce the disability rate of stroke (Matsui et al., 2010). However, a multi-center randomized controlled trial (RCT) illustrates that VER does not significantly improve the quality of life and communication skills of stroke patients compared with conventional nursing care (Cumming et al., 2019; Godecke et al., 2021). In the present study, VER protected cognitive function in mild-to-moderate TBI patients possibly due to selection biases resulting from differences in comorbidities of other injuries, duration of disease stabilization, and the willingness to cooperate with rehabilitation exercises. Rigorous-designed RCTs are needed in future to analyze the clinical benefits of VER for mild-to-moderate TBI patients.
We finally created two nomograms to predict risk factors for cognitive impairment after 3 and 12 months of mild-to-moderate TBI, in which conventional demographic, clinical, and radiological data were incorporated. Both were accurate to predict cognitive impairment after TBI, thus making it possible to design an individualized and timely therapeutic strategy to prevent cognitive dysfunction. There are several limitations in the present study. First of all, we excluded TBI patients with severe combined injuries, which limited the application of the prediction models. Second, we did not retrospectively analyze electroencephalogram (EEG) data and laboratory testing data in TBI patients, which may influence the discriminative ability of the nomograms. Third, it was a single-center retrospective study that lacked external validation. Our findings should be further validated in multi-center clinical studies.
Conclusion
Age, GCS score, >12 years of education, hyperlipidemia, temporal lobe contusion, tSAH, VER, and ICU admission are independent risk factors for cognitive impairment after 3 months of TBI; meanwhile, age, the GCS scores, diabetes mellitus, tSAH, and surgical treatment are independent risk factors of cognitive impairment after 12 months of TBI. Two nomograms, based on both groups of factors, respectively, showed strong discriminative abilities for cognitive impairment, which may be used to assist clinical management of cognitive impairment following TBI.
Data availability statement
The original contributions presented in the study are included in the article/supplementary material, further inquiries can be directed to the corresponding authors.
Ethics statement
The studies involving human participants were reviewed and approved by Ethics Committee of the First Affiliated Hospital of Nanjing Medical University. The patients/participants provided their written informed consent to participate in this study.
Author contributions
XiW, XH, and XiaW conceived and designed the study. XiW, XG, XL, and YS collected the dates. BH and XH analyzed the results and wrote the manuscript. YS and LL reviewed and edited the manuscript. All authors contributed to the article and approved the submitted version.
Funding
This study was supported by grants from the Jiangsu Province Capability Improvement Project through Science, Technology and Education (grant number: ZDXK202225) and the National Natural Science Foundation of China (grant number: 81171147).
Conflict of interest
The authors declare that the research was conducted in the absence of any commercial or financial relationships that could be construed as a potential conflict of interest.
Publisher's note
All claims expressed in this article are solely those of the authors and do not necessarily represent those of their affiliated organizations, or those of the publisher, the editors and the reviewers. Any product that may be evaluated in this article, or claim that may be made by its manufacturer, is not guaranteed or endorsed by the publisher.
References
Almeida-Meza, P., Richards, M., and Cadar, D. (2022). Moderating role of cognitive reserve markers between childhood cognition and cognitive aging: evidence from the 1946 British Birth Cohort. Neurology 99, e1239–e1250. doi: 10.1212/wnl.0000000000200928
Arenaza-Urquijo, E. M., Gonneaud, J., Fouquet, M., Perrotin, A., Mézenge, F., Landeau, B., et al. (2015). Interaction between years of education and APOE ε4 status on frontal and temporal metabolism. Neurology 85, 1392–1399. doi: 10.1212/wnl.0000000000002034
Arenth, P. M., Russell, K. C., Scanlon, J. M., Kessler, L. J., and Ricker, J. H. (2014). Corpus callosum integrity and neuropsychological performance after traumatic brain injury: a diffusion tensor imaging study. J. Head Trauma Rehabil. 29, E1–e10. doi: 10.1097/HTR.0b013e318289ede5
Biffi, A., Bailey, D., Anderson, C. D., Ayres, A. M., Gurol, E. M., Greenberg, S. M., et al. (2016). Risk factors associated with early vs. delayed dementia after intracerebral hemorrhage. JAMA Neurol 73, 969–976. doi: 10.1001/jamaneurol.2016.0955
Cukierman, T., Gerstein, H. C., and Williamson, J. D. (2005). Cognitive decline and dementia in diabetes–systematic overview of prospective observational studies. Diabetologia 48, 2460–2469. doi: 10.1007/s00125-005-0023-4
Cumming, T. B., Churilov, L., Collier, J., Donnan, G., Ellery, F., Dewey, H., et al. (2019). Early mobilization and quality of life after stroke: findings from AVERT. Neurology 93, e717–e728. doi: 10.1212/wnl.0000000000007937
de Boussard Lundin, C. N., Karlstedt, A., Edman, D., Bartfai, G. A., and Borg, J. (2005). S100 and cognitive impairment after mild traumatic brain injury. J Rehabil Med 37, 53-57. doi: 10.1080/16501970410015587
Draper, K., and Ponsford, J. (2008). Cognitive functioning 10 years following traumatic brain injury and rehabilitation. Neuropsychology 22, 618–625. doi: 10.1037/0894-4105.22.5.618
Farbota, K. D., Sodhi, A., Bendlin, B. B., McLaren, D. G., Xu, G., Rowley, H. A., et al. (2012). Longitudinal volumetric changes following traumatic brain injury: a tensor-based morphometry study. J Int Neuropsychol Soc 18, 1006–1018. doi: 10.1017/s1355617712000835
Godecke, E., Armstrong, E., Rai, T., Ciccone, N., Rose, M. L., Middleton, S., et al. (2021). A randomized control trial of intensive aphasia therapy after acute stroke: the very early rehabilitation for SpEech (VERSE) study. Int. J. Stroke 16, 556–572. doi: 10.1177/1747493020961926
Goldberg, T. E., Chen, C., Wang, Y., Jung, E., Swanson, A., Ing, C., et al. (2020). Association of delirium with long-term cognitive decline: a meta-analysis. JAMA Neurol. 77, 1373–1381. doi: 10.1001/jamaneurol.2020.2273
Han, S. M., Wan, H., Kudo, G., Foltz, W. D., Vines, D. C., Green, D. E., et al. (2014). Molecular alterations in the hippocampus after experimental subarachnoid hemorrhage. J. Cereb. Blood Flow Metab. 34, 108–117. doi: 10.1038/jcbfm.2013.170
Hayashi, K., Kurioka, S., Yamaguchi, T., Morita, M., Kanazawa, I., Takase, H., et al. (2011). (2011). Association of cognitive dysfunction with hippocampal atrophy in elderly Japanese people with type 2 diabetes. Diabetes Res. Clin. Pract. 94, 180–185. doi: 10.1016/j.diabres.07002
Hellstrøm, T., Andelic, N., Holthe, Ø., Helseth, E., Server, A., Eiklid, K., et al. (2022). APOE-ε4 is associated with reduced verbal memory performance and higher emotional, cognitive, and everyday executive function symptoms 2 months after mild traumatic brain injury. Front. Neurol. 13, 735206. doi: 10.3389/fneur.2022.735206
Hu, X., Yan, J., Huang, L., Araujo, C., Peng, J., Gao, L., et al. (2021). INT-777 attenuates NLRP3-ASC inflammasome-mediated neuroinflammation via TGR5/cAMP/PKA signaling pathway after subarachnoid hemorrhage in rats. Brain Behav. Immun. 91, 587-600. doi: 10.1016/j.bbi.09016
Injury, G. B. D. T. B., and Spinal Cord Injury, C. (2019). Global, regional, and national burden of traumatic brain injury and spinal cord injury, 1990-2016: a systematic analysis for the Global Burden of Disease Study 2016. Lancet Neurol. 18, 56–87. doi: 10.1016/S1474-4422(18)30415-0
Jenkins, P. O., Simoni, D. e., Bourke, S., Fleminger, N. J., Scott, J., Towey, G. D.J., et al. (2019). Stratifying drug treatment of cognitive impairments after traumatic brain injury using neuroimaging. Brain 142, 2367–2379. doi: 10.1093/brain/awz149
Kim, E., Seo, H. G., Lee, H. H., Lee, S. H., Choi, S. H., Yoo, R. E., et al. (2021). Reduced brainstem volume after mild traumatic brain injury. Am. J. Phys. Med. Rehabil. 100, 473–482. doi: 10.1097/phm.0000000000001580
Lachmann, G., Feinkohl, I., Borchers, F., Ottens, T. H., Nathoe, H. M., Sauer, A. M., et al. (2018). Diabetes, but not hypertension and obesity, is associated with postoperative cognitive dysfunction. Dement. Geriatr. Cogn. Disord. 46, 193–206. doi: 10.1159/000492962
Martin, R. M., Wright, M. J., Lutkenhoff, E. S., Ellingson, B. M., Van Horn, J. D., Tubi, M., et al. (2017). Traumatic hemorrhagic brain injury: impact of location and resorption on cognitive outcome. J. Neurosurg. 126, 796–804. doi: 10.3171/2016.3.jns151781
Martinez-Molina, N., Siponkoski, S. T., and Sarkamo, T. (2022). Cognitive efficacy and neural mechanisms of music-based neurological rehabilitation for traumatic brain injury. Ann. N. Y. Acad. Sci. 1515, 20–32. doi: 10.1111/nyas.14800
Matsui, H., Hashimoto, H., Horiguchi, H., Yasunaga, H., and Matsuda, S. (2010). An exploration of the association between very early rehabilitation and outcome for the patients with acute ischaemic stroke in Japan: a nationwide retrospective cohort survey. BMC Health Serv. Res. 10, 213. doi: 10.1186/1472-6963-10-213
McHugh, T., Laforce, R. Jr., Gallagher, P., Quinn, S., Diggle, P., and Buchanan, L. (2006). Natural history of the long-term cognitive, affective, and physical sequelae of mild traumatic brain injury. Brain Cogn. 60, 209–211.
Miotto, E. C., Cinalli, F. Z., Serrao, V. T., Benute, G. G., Lucia, M. C., Scaff, M., et al. (2010). Cognitive deficits in patients with mild to moderate traumatic brain injury. Arq. Neuropsiquiatr. 68, 862–868. doi: 10.1590/s0004-282x2010000600006
Nasreddine, Z. S., Phillips, N. A., Bédirian, V., Charbonneau, S., Whitehead, V., Collin, I., et al. (2005). The montreal cognitive assessment, MoCA: a brief screening tool for mild cognitive impairment. J. Am. Geriatr. Soc. 53, 695–699. doi: 10.1111/j.1532-5415.2005.53221.x
Neville, I. S., Hayashi, C. Y., El Hajj, S. A., Zaninotto, A. L., Sabino, J. P., Sousa, L. M. Jr., et al. (2015). Repetitive transcranial magnetic stimulation (rTMS) for the cognitive rehabilitation of traumatic brain injury (TBI) victims: study protocol for a randomized controlled trial. Trials 16, 440. doi: 10.1186/s13063-015-0944-2
Nguyen, L., Murphy, K., and Andrews, G. (2019). Cognitive and neural plasticity in old age: a systematic review of evidence from executive functions cognitive training. Ageing Res. Rev. 53, 100912. doi: 10.1016/j.arr.2019.100912
Othman, A., Idris, Z., Rosman, A. K., Abdullah, J. M., Ghan, I. A., Zakaria, A. Z., et al. (2022). Cognitive impairment and neuropsychiatry manifestation following mild and moderate traumatic brain injury at 3 months and 6 months. Malays. J. Med. Sci. 29, 48–58. doi: 10.21315/mjms295.6
Panwar, N., Purohit, D., Deo Sinha, V., and Joshi, M. (2019). Evaluation of extent and pattern of neurocognitive functions in mild and moderate traumatic brain injury patients by using Montreal Cognitive Assessment (MoCA) score as a screening tool: an observational study from India. Asian J. Psychiatr. 41, 60–65. doi: 10.1016/j.ajp.08007
Pavlovic, D., Pekic, S., Stojanovic, M., and Popovic, V. (2019). Traumatic brain injury: neuropathological, neurocognitive and neurobehavioral sequelae. Pituitary 22, 270–282. doi: 10.1007/s11102-019-00957-9
Regnier-Golanov, A. S., Gulinello, M., Hernandez, M. S., Golanov, E. V., and Britz, G. W. (2022). Subarachnoid hemorrhage induces sub-acute and early chronic impairment in learning and memory in mice. Transl. Stroke Res. 13, 625–640. doi: 10.1007/s12975-022-00987-9
Reijmer, Y. D., van den Heerik, M. S., Heinen, R., Leemans, A., Hendrikse, J., Vis, d. e., et al. (2018). Microstructural white matter abnormalities and cognitive impairment after aneurysmal subarachnoid hemorrhage. Stroke 49, 2040–2045. doi: 10.1161/strokeaha.118.021622
Schneider, A. L. C., Huie, J. R., Boscardin, W. J., Nelson, L., Barber, J. K., Yaffe, K., et al. (2022). Cognitive outcome 1 year after mild traumatic brain injury: results from the TRACK-TBI study. Neurology 98, e1248–e1261. doi: 10.1212/wnl.0000000000200041
Skandsen, T., Finnanger, T. G., Andersson, S., Lydersen, S., Brunner, J. F., Vik, A., et al. (2010). (2010). Cognitive impairment 3 months after moderate and severe traumatic brain injury: a prospective follow-up study. Arch. Phys. Med. Rehabil. 91, 1904-1913. doi: 10.1016/j.apmr.0821
Tariq, A., Ai, J., Chen, G., Sabri, M., Jeon, H., Shang, X., et al. (2010). Loss of long-term potentiation in the hippocampus after experimental subarachnoid hemorrhage in rats. Neuroscience 165, 418-426. doi: 10.1016/j.neuroscience.10040
van Elderen, S. G., Roos, d. e., de Craen, A., Westendorp, A. J., Blauw, R. G., Jukema, G. J., et al. (2010). Progression of brain atrophy and cognitive decline in diabetes mellitus: a 3-year follow-up. Neurology 75, 997–1002. doi: 10.1212/WNL.0b013e3181f25f06
Vuletic, V., Drenjancevic, I., Rahelic, D., and Demarin, V. (2013). Effect of indomethacin on cerebrovascular reactivity in patients with type 2 diabetes mellitus. Diabetes Res. Clin. Pract. 101, 81–87. doi: 10.1016/j.diabres.04004
Wang, J., Su, E., Wang, H., Guo, C., Lawrence, D. A., Eitzman, D. T., et al. (2018). Traumatic brain injury leads to accelerated atherosclerosis in apolipoprotein E deficient mice. Sci. Rep. 8, 5639. doi: 10.1038/s41598-018-23959-2
Keywords: traumatic brain injury, cognitive impairment, predictive factor, nomogram, radiological parameters
Citation: Wang X, Hui X, Wang X, Huang B, Gan X, Liu X, Shen Z, Sun Y and Li L (2023) Utilization of clinical and radiological parameters to predict cognitive prognosis in patients with mild-to-moderate traumatic brain injury. Front. Neurosci. 17:1222541. doi: 10.3389/fnins.2023.1222541
Received: 14 May 2023; Accepted: 03 July 2023;
Published: 27 July 2023.
Edited by:
Guang-qing Xu, The First Affiliated Hospital of Sun Yat-sen University, ChinaReviewed by:
Lihua Sun, Shanghai Jiao Tong University, ChinaZong Zhuang, Nanjing Drum Tower Hospital, China
Wenhua Fan, Capital Medical University, China
Copyright © 2023 Wang, Hui, Wang, Huang, Gan, Liu, Shen, Sun and Li. This is an open-access article distributed under the terms of the Creative Commons Attribution License (CC BY). The use, distribution or reproduction in other forums is permitted, provided the original author(s) and the copyright owner(s) are credited and that the original publication in this journal is cited, in accordance with accepted academic practice. No use, distribution or reproduction is permitted which does not comply with these terms.
*Correspondence: Yi Sun, c3VueWlnb29kMjAyMUAxNjMuY29t; Lixin Li, bGlsaXhpbjJAaG90bWFpbC5jb20=
†These authors have contributed equally to this work and share first authorship