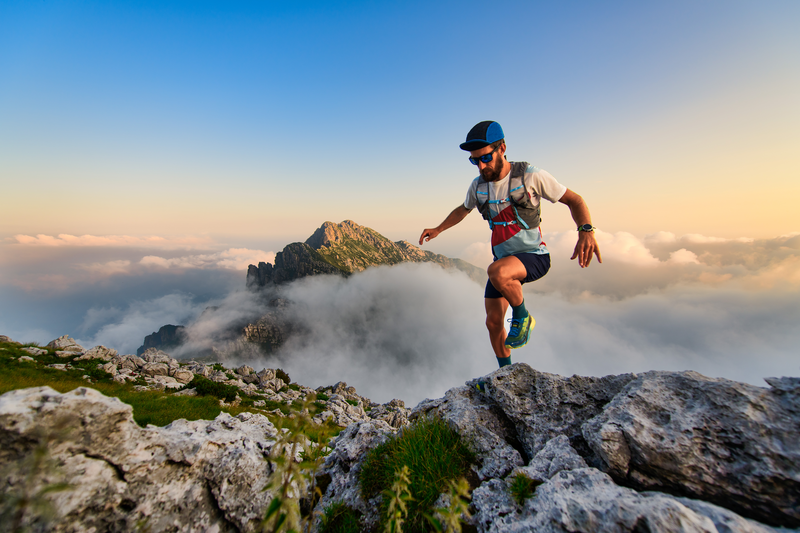
95% of researchers rate our articles as excellent or good
Learn more about the work of our research integrity team to safeguard the quality of each article we publish.
Find out more
SYSTEMATIC REVIEW article
Front. Neurosci. , 12 May 2023
Sec. Neuroprosthetics
Volume 17 - 2023 | https://doi.org/10.3389/fnins.2023.1145751
Background: Intra- or inter-muscular (EMG-EMG) coherence is a simple and non-invasive method for estimating central nervous system control during human standing tasks. Although this research area has developed, no systematic literature review has been conducted.
Objectives: We aimed to map the current literature on EMG-EMG coherence during various standing tasks to identify the research gaps and summarize previous studies comparing EMG-EMG coherence between healthy young and elderly adults.
Methods: Electronic databases (PubMed, Cochrane Library, and CINAHL) were searched for articles published from inception to December 2021. We incorporated studies that analyzed EMG-EMG coherence of the postural muscles in various standing tasks.
Results: Finally, 25 articles fulfilled the inclusion criteria and involved 509 participants. Most participants were healthy young adults, while only one study included participants with medical conditions. There was some evidence that EMG-EMG coherence could identify differences in standing control between healthy young and elderly adults, although the methodology was highly heterogeneous.
Conclusion: The present review indicates that EMG-EMG coherence may help elucidate changes in standing control with age. In future studies, this method should be used in participants with central nervous system disorders to understand better the characteristics of standing balance disabilities.
Bipedal standing is a posture exclusive to humans, and maintaining the standing balance is essential for daily living. However, the standing posture in humans is characterized by a narrow base of support and a high center of mass, making the posture biomechanically unstable. Further, functional decline with age increases postural sway (Masui et al., 2005; Roman-Liu, 2018). In addition, patients with neurological and musculoskeletal disorders have poor balance control (Tyson and Connell, 2009; de Lima et al., 2021) and are at high risk of falling (Weerdesteyn et al., 2008; Moutzouri et al., 2017). Therefore, to prevent falls, the neurophysiological mechanisms of standing balance control in healthy young adults and elderly adults, and individuals with the disease should be clarified.
Given the activities of daily living, the standing balance in various standing postures and sensory perturbations should be controlled. Because difficulty in standing varies with the size of the base of support, the flexible support of the central nervous system (CNS) in standing postures is often assumed (Watanabe et al., 2018a; Nandi et al., 2019). Furthermore, the difficulty in channeling the correct standing balance and the related neuromuscular activities varies depending on the available sensory inputs (Carver et al., 2006; Jeka et al., 2010). For example, more significant postural sway and specific neuromuscular activity while standing with eyes closed than with eyes open (Danna-Dos-Santos et al., 2015; Walker et al., 2020) has been reported. In addition, elderly adults (Nojima et al., 2018; Fujio and Takeuchi, 2021) and patients with stroke (de Haart et al., 2004) have a greater loss of standing balance in response to sensory disturbances than healthy young adults. Therefore, the mechanism of CNS controls of the postural muscles when the base of support and sensory information change should be investigated to elucidate human standing control.
Recently, intra- or inter-muscular (EMG-EMG) coherence was developed to investigate the control of the CNS during human standing tasks. The coherence analysis quantifies the strength of the common oscillation between two signals. Thus, EMG-EMG coherence quantifies the common oscillatory inputs to the motor neurons in the frequency band (Farmer et al., 1993), and can be conducted simply by measuring a pair of electromyography signals non-invasively. EMG-EMG coherence is analyzed from the auto-spectra of EMG signal x and y (Pxx and Pyy), and the cross-spectra (Pxy) in each frequency (f) with the Fourier transform. The coherence is estimated using the following equation.
Coherence takes the range 0–1, with greater values reflecting higher common inputs to the two motor neuron pools. Therefore, EMG-EMG coherence can provide insight into how the CNS may synchronize and regulate motor control among a combination of a number of muscles. Also, the coherence in each frequency band is generated by various brain areas and circuits (spinal, subcortical, or cortical). Thus, EMG-EMG coherence can provide an estimate of the neural circuits and the neural origin contributing to motor tasks. Delta-band coherence reflects synchronous oscillations in motor unit firing (Lowery et al., 2007) and is thought to reflect subcortical activity (Boonstra and Breakspear, 2012). Alpha-band coherence is associated with multiple factors (Grosse et al., 2002) that reflect subcortical inputs and may also have some cortical contributions (Boonstra and Breakspear, 2012; Obata et al., 2014). In addition, beta-band coherence is strongly related to corticospinal drive (Conway et al., 1995; Farmer, 1998; Grosse et al., 2002). In standing tasks, EMG-EMG coherence is significant in bilateral homologous and unilateral plantar flexor pairs in the delta-band and some alpha frequencies (Mochizuki et al., 2006; Boonstra et al., 2009; Obata et al., 2014; Watanabe et al., 2018a,b,c). Delta-to-alpha band coherence between antagonistic muscles is increased with increasing standing difficulty (Nandi et al., 2019; Nojima et al., 2020), such as with unilateral standing. In addition, beta-band coherence between synergistic muscles is increased in relatively complex standing tasks (Watanabe et al., 2018a,b; Nandi et al., 2019; Nojima et al., 2020). This suggests that cortical activity is increased to maintain standing balance. Thus, EMG-EMG coherence in standing tasks comprise various muscle pairs and frequency bands, and therefore the analytical methods used in previous studies should be integrated to provide a direction for future research.
Several studies reported that elderly adults have greater EMG-EMG coherence in the delta-to-alpha band in bipedal standing compared to young adults (Obata et al., 2014; Walker et al., 2020) and that they are less likely to change the beta coherence in response to challenging standing task (Watanabe et al., 2018b). Thus, EMG-EMG coherence could provide insight into the neuromuscular control associated with age-related deficits of standing balance. Furthermore, patients with CNS disorder decreased beta-band coherence on the paretic muscle pairs during gait, which was associated with clinical scales (Nielsen et al., 2008; Kitatani et al., 2016). Therefore, EMG-EMG coherence especially in the beta-band can also serve as an indicator of impaired motor control mechanisms in CNS disorders with lesions in the corticospinal tract. However, it is unclear how much evidence exists for EMG-EMG coherence during standing tasks in individuals with CNS lesions.
Studies investigating EMG-EMG coherence in human standing tasks started in the 2000's and have been validated in various individuals and tasks. EMG-EMG coherence analysis may reveal differences in standing controls between different individuals and tasks, but there is no consensus on this point of view. Given that this is a relatively new approach to identifying voluntary control of human standing, the purpose of this systematic scoping review was to map the current literature pertaining to the analysis of EMG-EMG coherence during various standing tasks in individuals and to identify the gaps in the research. In addition, several studies have accumulated showing that EMG-EMG coherence during standing tasks differs between young and elderly adults. These studies could contribute to revealing the neurophysiological mechanisms of age-related decline in standing balance; however, no literature has systematically integrated the available evidence. Therefore, this scoping review focused on summarizing previous studies evaluating the difference in EMG-EMG coherence between healthy young and elderly adults.
This systematic scoping review of the literature was conducted according to the Preferred Reporting Items for Systematic reviews and Meta-Analyses extension for Scoping Reviews (PRISMA-ScR) check-list (Tricco et al., 2018) (Supplementary material 1).
The inclusion criteria were studies evaluating EMG-EMG coherence during voluntary control of various standing tasks in humans. There was no restriction on the publication year, and only English papers published till the search date were considered. The exclusion criteria for this study were as follows: (1) coherence analysis other than EMG-EMG, such as EEG-EMG, COP-EMG, etc., (2) coherence analysis of “involuntary” phenomena including tremors, spasms, and response to external stimuli, (3) coherence analysis during voluntary contractions that are not standing tasks, (4) conference abstracts, (5) studied in animals and in vitro studies. This review included studies that met the aforementioned criteria 1-3 and also included EMG-EMG coherence analysis during voluntary standing tasks.
We searched PubMed, Cochrane Library, and CINAHL for full-text articles. The end date of the study period was December 31, 2021. References were also searched manually by reviewing the reference lists of the included studies.
A Participant/Concept/Context (PCC) model was used to build the criteria for the search of electronic databases. The PCC model comprised the Participant: “limited to humans,” Concept: “EMG-EMG coherence, intra-/inter-muscular coherence, standing tasks,” and Context: “both laboratory and clinical settings.” We combined the search terms [(coherence) OR (coherent)] AND [(electromyo*) OR (emg)] AND [(postur*) OR (stand*) OR (stance) OR (balance)] (Supplementary material 2) and searched in all fields. The search was conducted by one author (E.Y.), and the consistency of the search results was determined by another researcher (Y.H.).
The eligibility of the included studies was determined independently by two authors (E.Y. and Y.H.), first by reading the title and abstract for screening and then by reading the full text. During the screening process of titles and abstracts, we excluded studies that did not explicitly mention the inclusion of standing tasks. However, for studies that did not clearly specify EMG-EMG coherence analysis in the title and abstract screening process, we made full-text eligibility determinations instead of excluding them during the initial screening phase, assuming that such analysis was reported as a sub-outcome. In cases of disagreement between the two authors, the opinion of a third researcher (I.N.) was sought.
A data-charting form was jointly developed by the reviewers to determine the variables to be extracted. We used a table as a charting form because it was most frequently reported in previous scoping reviews (Miake-Lye et al., 2016). Data charting was performed by one author (E.Y.), and the research team verified whether the charted summaries were consistent with those of the included articles. The data charting included citations (authors and years), participants (number of participants and participant characteristics), study design (cross-sectional or longitudinal), standing tasks, methods of coherence analysis (muscular pair and frequency bands), and principal findings.
Since the purpose of this review was to clarify the differences in EMG-EMG coherence between healthy young and elderly adults during standing tasks, we summarized the results of studies that compared these groups. The studies included in this summary were those that were identified in the participant section during the data charting phase as included both young (20–40 years) and elderly or older (>60 years) groups. We listed the results of each study rather than synthesized the results, because each study involved different muscle pairs and frequency bands for the coherence analysis.
We obtained 272 articles from the database search and 15 articles from additional records. After removing duplicate articles (n = 15), the titles and abstracts of 272 publications were screened (Figure 1). Of these, 47 articles were assessed for eligibility by full-text screening, and 22 articles were excluded based on the following exclusion criteria: (1) coherence analysis other than EMG-EMG (n = 6), (2) coherence analysis of involuntary phenomena including tremors, spasms, and response to external stimuli (n = 9), (3) coherence analysis during voluntary contractions that are not standing tasks (n = 5), and (4) conference abstracts (n = 2).
Figure 1. Study results. A flowchart of the selection process based on inclusion and exclusion criteria.
The characteristics of included studies are listed in Table 1. Of the 25 included studies, 21 were cross-sectional and only four were longitudinal studies. In these cross-sectional studies, the research purpose was mainly to compare EMG-EMG coherence among different participant groups and/or different standing tasks. Three longitudinal studies examined EMG-EMG coherence before and immediately after a single session of a fatigue protocol (Watanabe et al., 2018c) and alcohol intake (Boonstra et al., 2008, 2009), and the other longitudinal study investigated coherence before and after 14 weeks of resistance training (Walker et al., 2020).
The 25 included studies involved 509 participants, including 315 healthy young adults, 159 healthy elderly adults, 21 healthy children, and 14 patients with idiopathic Parkinson's disease and Pisa syndrome. Sixteen studies (Mochizuki et al., 2006; Boonstra et al., 2008, 2009, 2016; Saffer et al., 2008; Danna-Dos-Santos et al., 2014, 2015; von Tscharner et al., 2014; Mohr et al., 2015; García-Massó et al., 2016; Noé et al., 2017; Tanabe et al., 2017; Watanabe et al., 2018c; Nandi et al., 2019; Kenville et al., 2020; Hug et al., 2021) involved a single group of healthy young adults, of which, one study involved a group of non-professional classical ballet dancers (Tanabe et al., 2017). Only one study (Nojima et al., 2020) involved a single group of healthy elderly adults. Six studies (Degani et al., 2017, 2020; Watanabe et al., 2018a,b; Walker et al., 2020; Minamisawa et al., 2021) analyzed two groups of healthy young and elderly adults, and one study (Obata et al., 2014) assessed three groups of healthy young adults, elderly adults, and children. Only one study (Formaggio et al., 2019) recruited participants with physical disabilities and Parkinson's disease with Pisa syndrome. While several studies investigated healthy young and elderly adults, the results for patients in clinical settings were revealed only to a limited extent.
Various standing tasks were performed in previous studies (Table 1). Most studies (Mochizuki et al., 2006; Boonstra et al., 2008, 2016; Saffer et al., 2008; Obata et al., 2014; Danna-Dos-Santos et al., 2015; García-Massó et al., 2016; Noé et al., 2017; Watanabe et al., 2018a,b,c; Formaggio et al., 2019; Nandi et al., 2019; Degani et al., 2020; Nojima et al., 2020; Walker et al., 2020; Minamisawa et al., 2021) reported common tasks as natural bipedal standing. In addition, several studies implemented unipedal (García-Massó et al., 2016; Watanabe et al., 2018a; Nandi et al., 2019; Nojima et al., 2020) or bipedal standing with eyes closed (Saffer et al., 2008; Obata et al., 2014; Danna-Dos-Santos et al., 2015; Noé et al., 2017; Degani et al., 2020; Walker et al., 2020), and compared with natural bipedal standing. Other standing tasks included shifting the center of pressure within the base of support (Boonstra et al., 2009; Watanabe et al., 2018b), dual tasks (Boonstra et al., 2016), standing at a height (Boonstra et al., 2016), squatting (Mohr et al., 2015; Kenville et al., 2020), and heel raises (von Tscharner et al., 2014; Hug et al., 2021). EMG-EMG coherence increased as the base of support narrowed and the difficulty in standing increased (Watanabe et al., 2018a; Nandi et al., 2019; Nojima et al., 2020). These studies reported that beta-band coherence, in particular, varied with the complexity of the standing tasks. Regarding comparisons with and without visual information, no clear consensus can be reached. Several studies (Boonstra et al., 2008; Obata et al., 2014; Walker et al., 2020) reported increased coherence during eye-closed conditions than during natural standing, but a few studies found no change (Saffer et al., 2008) or the opposite effect (Danna-Dos-Santos et al., 2015; Degani et al., 2020).
Coherence analyses were performed in intra-muscular (von Tscharner et al., 2014; Hug et al., 2021), bilateral homonymous muscle pairs (Mochizuki et al., 2006; Boonstra et al., 2008, 2009, 2016; Obata et al., 2014; Watanabe et al., 2018b,c; Kenville et al., 2020; Minamisawa et al., 2021), unilateral plantar flexor pairs (Mochizuki et al., 2006; Saffer et al., 2008; Obata et al., 2014; Noé et al., 2017; Watanabe et al., 2018a,b,c; Nandi et al., 2019; Nojima et al., 2020; Walker et al., 2020; Hug et al., 2021; Minamisawa et al., 2021), unilateral knee extensor pairs (Mohr et al., 2015; Boonstra et al., 2016; Tanabe et al., 2017), and unilateral antagonistic muscle pairs (Saffer et al., 2008; Boonstra et al., 2016; García-Massó et al., 2016; Noé et al., 2017; Tanabe et al., 2017; Nandi et al., 2019; Degani et al., 2020; Nojima et al., 2020; Walker et al., 2020). Several studies (Danna-Dos-Santos et al., 2015; García-Massó et al., 2016; Degani et al., 2020) investigated muscle pairs across joints according to the components of muscle synergy (M-mode). There was no standard set of muscle pairs for analysis in the standing tasks, and the variability between the studies was high (Table 2). The delta-band was the most frequent frequency band of interest for coherence analysis. Many studies quantified coherence by classifying it into delta, alpha, beta, and gamma bands. However, the specific values for classifying the delta, alpha, and beta bands were researcher-dependent and differed among the studies. Most studies selected 0–5 Hz for the coherence analysis of the delta-band, and about half of the studies selected 15–35 Hz for the beta-band (Figure 2).
Figure 2. Numerical values separating the delta, alpha, and beta bands applied in the included studies.
Obata et al. (2014) reported that a significant coherence of 0–4 Hz was found for both groups in all muscle pairs (bilateral and unilateral plantar flexors), while a significant coherence of 8–12 Hz was found only for the elderly group in bilateral muscle pairs. Furthermore, coherence of 0–4 and 8–12 Hz were higher in the elderly group, and in the closed-eye condition.
Watanabe et al. (2018a) also found that during bipedal standing, the delta-band coherence of the plantar flexor pairs was greater in elderly adults than in young adults. Similarly, the delta-band coherence for the plantar flexor pair was greater in unipedal than in bipedal standing for both age groups and in the elderly than in young adults. In addition, the beta-band coherence for this pair was greater than the other pairs in unipedal standing for elderly adults, which implies that elderly adults have selectively increased corticospinal drive to these muscles to cope with postural sway. Another study (Watanabe et al., 2018b) allotted quiet standing and standing tasks in which the center of pressure was maintained in front of the base of support. When the center of pressure was moved forward, the beta-band coherence of the unilateral plantar flexor pairs increased in young adults, whereas no such change was observed in elderly adults.
Minamisawa et al. (2021) reported that during bipedal standing, significant coherence below 10 Hz was observed in bilateral and unilateral plantar flexor pairs in the elderly group, whereas significant coherence was below 4 Hz in the young group. They also found that the correlation coefficient between the center of mass acceleration and coherence level was low in young adults and moderate in elderly adults. These results suggest that EMG-EMG coherence is related to increased postural sway during standing with age.
Walker et al. (2020) examined changes in coherence after 14 weeks of resistance training in open- and closed-eye standing positions. Before the training, apparent increasing of the medial gastrocnemius and tibialis anterior coherence levels was observed in closed-eye standing tasks compared to the open-eye standing tasks in young adults, which was not observed in elderly adults. In addition, strength training did not alter the significant coherence increase between open- and closed-eyes standing conditions in either muscle pair. The only training-induced change in coherence magnitude was observed in the soleus and tibialis anterior pair for the beta-band during the closed-eye standing task, which was lower in young adults after training.
Degani et al. (2017) reported EMG-EMG coherence in the pairs of synergistic muscle groups. They found that young adults presented with significant coherence of 0–5 Hz in the soleus and biceps femoris pair, whereas elderly adults presented with significant coherence of 0–10 Hz. In addition, elderly adults exhibited significantly greater coherence of 0–10 Hz in the soleus and elector spinae and biceps femoris and elector spinae pairs than young adults. These findings were consistent with the results of previous studies (Obata et al., 2014; Watanabe et al., 2018a,b). Another study (Degani et al., 2020) investigated EMG-EMG coherence during open- and closed-eyes bipedal standing with the M-mode. They found that young adults exhibited a significantly decreased coherence of 1–10 Hz in the anterior and posterior muscle pairs with eyes closed, while no such change was observed in elderly adults. This result was different from those of studies (Obata et al., 2014; Walker et al., 2020) that investigated the same standing tasks.
This scoping review was conducted to map the literature on EMG-EMG coherence during standing tasks in humans to identify preliminary findings and areas requiring further research. We identified that most studies were cross-sectional, and that more than half of the participants were healthy young adults. While various studies included healthy elderly adults for comparison with young adults, only one study has included participants with preexisting medical conditions. Patients with CNS disorders exhibited EMG-EMG coherence characteristics different from those of healthy participants in motor tasks other than standing tasks, and coherence was associated with severity of the disorder (Nielsen et al., 2008; Fisher et al., 2012; Kitatani et al., 2016). EMG-EMG coherence analysis could be easy to perform and helps to quantify neuromuscular activity during motor tasks non-invasively. Further research should be conducted on standing tasks better to understand the characteristics of the disability of standing balance.
Half of the previous studies included in this review analyzed natural bipedal standing, though other standing tasks were also analyzed. Most studies compared EMG-EMG coherence in multiple standing tasks and many employed standing tasks with varying sizes of the base of support and sensory perturbation. These studies reported different muscle pairs, frequency bands, and magnitudes in EMG-EMG coherence among standing tasks, suggesting that human standing flexibly modulates neuromuscular activity according to task characteristics. Interestingly, although healthy young adults showed significant changes in EMG-EMG coherence in several standing conditions, such as unilateral standing (Watanabe et al., 2018a), forward leaning (Watanabe et al., 2018b), and sensory perturbation (Degani et al., 2020; Walker et al., 2020), these changes were limited in healthy elderly adults. These showed the importance of including multiple standing tasks and analyzing the changes in coherence between standing tasks in this research area. Thus, more challenging tasks could be analyzed in addition to bipedal standing to elucidate the neural mechanisms associated with standing disturbance.
Many muscle pairs and frequency bands were investigated using the coherence analytical method, resulting in a high degree of heterogeneity among the studies. The most frequently assessed muscle pairs were the unilateral and bilateral plantar flexor pairs. In contrast, the muscle pairs that produce significant coherence vary with the difficulty and characteristics of the standing task. Thus, the muscle pairs to be investigated should be determined by the characteristics of the standing task. During quiet standing, numerous studies have shown significant coherence between bilateral and unilateral plantar flexor muscles (Table 2), making these muscle pairs the primary focus in this field of research. Furthermore, it is recommended to analyze antagonistic muscle pairs (i.e., unilateral tibialis anterior and plantar flexors) as co-contraction between agonist-antagonist muscles is likely to occur in challenging or fearful standing tasks, or in groups at high risk of fall (Boonstra et al., 2016; Nandi et al., 2019; Nojima et al., 2020). Regarding the frequency band in coherence analysis, significant coherence was observed primarily in the delta band, regardless of the muscle pair. The delta frequency band is thought to reflect the synchronous activity of the motor neuron pool (Lowery et al., 2007). In addition, several studies reported significant beta-band coherence in some standing tasks. Beta-band coherence is thought to reflect activity in the corticospinal tract (Conway et al., 1995; Farmer, 1998; Grosse et al., 2002), suggesting control from the cortex during standing tasks. Since significant beta-band coherence was reported in standing tasks that are characterized by a narrow base of support (i.e., unipedal standing) (Watanabe et al., 2018a; Nandi et al., 2019; Nojima et al., 2020) and forward leaning (Watanabe et al., 2018b), cortical control of standing may increase as the difficulty of the standing task increases. Patients with CNS disorders that damage the corticospinal tracts may not be able to control complex standing tasks, possibly because of the lack of beta-band coherence. This point of view must be further verified. Many studies have divided the EMG-EMG coherence into the delta, alpha, beta bands and quantified it in each band. However, the present review revealed that the specific values for classifying the delta, alpha, and beta bands were researcher-dependent and differed among the studies. Most studies selected 0–5 Hz for the coherence analysis of the delta-band, and about half of the studies selected 15–35 Hz for the beta-band. The authors would recommend selecting these bandwidths in the delta and beta coherence analysis in order to reduce heterogeneity and accumulate results in this research area. In contrast, no specific recommendations could be established for the alpha-band. This is probably related to the fact that coherence in these bands occurs multifactorial and is less likely to be as significant as in the delta-band coherence. A consistent method to set the parameters of coherence analysis for integrating the results in this research area should be determined in the future.
This scoping review did not synthesize the results, summarized each study to determine the heterogeneity. Despite these limitations, each study reported consistent trends between healthy young and elderly adults. For example, in natural bipedal standing, elderly adults reported higher coherence levels in some muscle pairs and significant coherence for broader frequency bands than young adults (Obata et al., 2014; Degani et al., 2017, 2020; Watanabe et al., 2018a,b; Walker et al., 2020; Minamisawa et al., 2021). This phenomenon could occur because elderly adults have more significant postural sway and require more descending neural input in the motor neuron pools, even in natural bipedal standing. Furthermore, EMG-EMG coherence analysis indicates neural oscillations from different circuits in each frequency hand, which implies that older adults may have a wider range of neural activity during standing control compared to younger adults. Conversely, higher coherence levels indicate greater common input to the two motor neuron pools, but this does not necessarily indicate a greater range of neural activity. Instead, coherence analysis provides information about the degree of coordination between different neural circuits in each frequency band, which may be related to the level of corticospinal activity. Although the main origin of alpha-band coherence is proposed to be the reticulospinal pathway (Obata et al., 2014), the corticospinal pathway may also contribute to coherence in this frequency band, given that corticomuscular coherence at approximately 10 Hz has been reported in previous study (Conway et al., 1995). Consequently, it is plausible that elderly adults may improve postural sway by increasing corticospinal tract activity during quiet standing. Thus, postural sway was reported to be associated with coherence levels in elderly adults (Watanabe et al., 2018a; Minamisawa et al., 2021). Previous study (Nojima et al., 2020) reported that increased beta-band coherence in elderly adults was associated with smaller muscle mass in lower leg. This suggests that the elderly adults may compensate for the loss of muscle mass by increasing activity in the corticospinal tract during standing tasks. It was also shown that elderly adults were less likely to experience changes in EMG-EMG coherence in response to changes in standing difficulty and characteristics (Watanabe et al., 2018b; Walker et al., 2020). This corresponds to the fact that elderly adults exhibit inadequate standing control in a narrow base of support or a disturbance of sensory information (Fujio and Takeuchi, 2021). In addition, elderly adults require higher descending neural input, even in natural standing, and may not have the sufficient reserve capacity to augment or change their descending neural input with the change in task characteristics. Thus, the flexibility of EMG-EMG coherence between standing tasks may reflect the ability of elderly adults to control standing balance and can be used as a biomarker to predict falls in future studies.
The present review suggests that EMG-EMG coherence may help elucidate changes in standing control with age. Elderly adults have greater EMG-EMG coherence during natural bipedal standing than young adults, and significant coherence is observed over a broader frequency band. This could suggest that elderly adults require more descending neural input in bipedal standing and some cortical control of postural muscles. This analysis may contribute to understanding the mechanisms of balance disorders, not only age-related balance deficits, but also in individuals with CNS disorders, and should be investigated in a wider population. In addition, a consensus in the method of coherence analysis is required.
The original contributions presented in the study are included in the article/Supplementary material, further inquiries can be directed to the corresponding author.
IN conceived the review paper idea and guided the literature and drafting of the manuscript. EY and YH conducted the literature search. EY prepared the manuscript for this review. All authors contributed to the article and approved the submitted version.
This work was supported by JSPS KAKENHI Grant Numbers 20K09448, 20K20657, and 19K22804.
We would like to thank Editage (www.editage.com) for English language editing.
The authors declare that the research was conducted in the absence of any commercial or financial relationships that could be construed as a potential conflict of interest.
All claims expressed in this article are solely those of the authors and do not necessarily represent those of their affiliated organizations, or those of the publisher, the editors and the reviewers. Any product that may be evaluated in this article, or claim that may be made by its manufacturer, is not guaranteed or endorsed by the publisher.
The Supplementary Material for this article can be found online at: https://www.frontiersin.org/articles/10.3389/fnins.2023.1145751/full#supplementary-material
Boonstra, T. W., and Breakspear, M. (2012). Neural mechanisms of intermuscular coherence: Implications for the rectification of surface electromyography. J. Neurophysiol. 107, 796–807. doi: 10.1152/jn.00066.2011
Boonstra, T. W., Daffertshofer, A., Roerdink, M., Flipse, I., Groenewoud, K., and Beek, P. J. (2009). Bilateral motor unit synchronization of leg muscles during a simple dynamic balance task. Eur. J. Neurosci. 29, 613–622. doi: 10.1111/j.1460-9568.2008.06584.x
Boonstra, T. W., Danna-Dos-Santos, A., Xie, H.-B., Roerdink, M., Stins, J. F., and Breakspear, M. (2016). Muscle networks: connectivity analysis of EMG activity during postural control. Sci. Rep. 5, 17830. doi: 10.1038/srep17830
Boonstra, T. W., Roerdink, M., Daffertshofer, A., van Vugt, B., van Werven, G., and Beek, P. J. (2008). Low-alcohol doses reduce common 10- to 15-Hz input to bilateral leg muscles during quiet standing. J. Neurophysiol. 100, 2158–2164. doi: 10.1152/jn.90474.2008
Carver, S., Kiemel, T., and Jeka, J. J. (2006). Modeling the dynamics of sensory reweighting. Biol. Cybern. 95, 123–134. doi: 10.1007/s00422-006-0069-5
Conway, B. A., Halliday, D. M., Farmer, S. F., Shahani, U., Maas, P., Weir, A. I., et al. (1995). Synchronization between motor cortex and spinal motoneuronal pool during the performance of a maintained motor task in man. J. Physiol. 489, 917–924. doi: 10.1113/jphysiol.1995.sp021104
Danna-Dos-Santos, A., Boonstra, T. W., Degani, A. M., Cardoso, V. S., Magalhaes, A. T., Mochizuki, L., et al. (2014). Multi-muscle control during bipedal stance: an EMG–EMG analysis approach. Exp. Brain Res. 232, 75–87. doi: 10.1007/s00221-013-3721-z
Danna-Dos-Santos, A., Degani, A. M., Boonstra, T. W., Mochizuki, L., Harney, A. M., Schmeckpeper, M. M., et al. (2015). The influence of visual information on multi-muscle control during quiet stance: a spectral analysis approach. Exp. Brain Res. 233, 657–669. doi: 10.1007/s00221-014-4145-0
de Haart, M., Geurts, A. C., Huidekoper, S. C., Fasotti, L., and van Limbeek, J. (2004). Recovery of standing balance in postacute stroke patients: a rehabilitation cohort study. Arch. Phys. Med. Rehabil. 85, 886–895. doi: 10.1016/j.apmr.2003.05.012
de Lima, F., Melo, G., Fernandes, D. A., Santos, G. M., and Rosa Neto, F. (2021). Effects of total knee arthroplasty for primary knee osteoarthritis on postural balance: a systematic review. Gait Posture 89, 139–160. doi: 10.1016/j.gaitpost.2021.04.042
Degani, A. M., Leonard, C. T., and Danna-dos-Santos, A. (2017). The use of intermuscular coherence analysis as a novel approach to detect age-related changes on postural muscle synergy. Neurosci. Lett. 656, 108–113. doi: 10.1016/j.neulet.2017.07.032
Degani, A. M., Leonard, C. T., and Danna-dos-Santos, A. (2020). The effects of aging on the distribution and strength of correlated neural inputs to postural muscles during unperturbed bipedal stance. Exp. Brain Res. 238, 1537–1553. doi: 10.1007/s00221-020-05837-4
Farmer, S. F. (1998). Rhythmicity, synchronization and binding in human and primate motor systems. J. Physiol. 509, 3–14. doi: 10.1111/j.1469-7793.1998.003bo.x
Farmer, S. F., Bremner, F. D., Halliday, D. M., Rosenberg, J. R., and Stephens, J. A. (1993). The frequency content of common synaptic inputs to motoneurones studied during voluntary isometric contraction in man. J. Physiol. 470, 127–155. doi: 10.1113/jphysiol.1993.sp019851
Fisher, K. M., Zaaimi, B., Williams, T. L., Baker, S. N., and Baker, M. R. (2012). Beta-band intermuscular coherence: a novel biomarker of upper motor neuron dysfunction in motor neuron disease. Brain 135, 2849–2864. doi: 10.1093/brain/aws150
Formaggio, E., Masiero, S., Volpe, D., Demertzis, E., Gallo, L., and Del Felice, A. (2019). Lack of inter-muscular coherence of axial muscles in Pisa syndrome. Neurol. Sci. 40, 1465–1468. doi: 10.1007/s10072-019-03821-7
Fujio, K., and Takeuchi, Y. (2021). Discrimination of standing postures between young and elderly people based on center of pressure. Sci. Rep. 11, 195. doi: 10.1038/s41598-020-80717-z
García-Massó, X., Pellicer-Chenoll, M., Gonzalez, L. M., and Toca-Herrera, J. L. (2016). The difficulty of the postural control task affects multi-muscle control during quiet standing. Exp. Brain Res. 234, 1977–1986. doi: 10.1007/s00221-016-4602-z
Grosse, P., Cassidy, M. J., and Brown, P. (2002). EEG–EMG, MEG–EMG and EMG–EMG frequency analysis: physiological principles and clinical applications. Clin. Neurophysiol. 113, 1523–1531. doi: 10.1016/S1388-2457(02)00223-7
Hug, F., Del Vecchio, A., Avrillon, S., Farina, D., and Tucker, K. (2021). Muscles from the same muscle group do not necessarily share common drive: evidence from the human triceps surae. J. Appl. Physiol. 130, 342–354. doi: 10.1152/japplphysiol.00635.2020
Jeka, J. J., Allison, L. K., and Kiemel, T. (2010). The dynamics of visual reweighting in healthy and fall-prone older adults. J. Mot. Behav. 42, 197–208. doi: 10.1080/00222895.2010.481693
Kenville, R., Maudrich, T., Vidaurre, C., Maudrich, D., Villringer, A., Ragert, P., et al. (2020). Intermuscular coherence between homologous muscles during dynamic and static movement periods of bipedal squatting. J. Neurophysiol. 124, 1045–1055. doi: 10.1152/jn.00231.2020
Kitatani, R., Ohata, K., Aga, Y., Mashima, Y., Hashiguchi, Y., Wakida, M., et al. (2016). Descending neural drives to ankle muscles during gait and their relationships with clinical functions in patients after stroke. Clin. Neurophysiol. 127, 1512–1520. doi: 10.1016/j.clinph.2015.10.043
Lowery, M. M., Myers, L. J., and Erim, Z. (2007). Coherence between motor unit discharges in response to shared neural inputs. J. Neurosci. Methods 163, 384–391. doi: 10.1016/j.jneumeth.2007.03.011
Masui, T., Hasegawa, Y., Matsuyama, Y., Sakano, S., Kawasaki, M., and Suzuki, S. (2005). Gender differences in platform measures of balance in rural community-dwelling elders. Arch. Gerontol. Geriatr. 41, 201–209. doi: 10.1016/j.archger.2005.02.003
Miake-Lye, I. M., Hempel, S., Shanman, R., and Shekelle, P. G. (2016). What is an evidence map? a systematic review of published evidence maps and their definitions, methods, and products. Syst. Rev. 5, 28. doi: 10.1186/s13643-016-0204-x
Minamisawa, T., Chiba, N., and Suzuki, E. (2021). Intra- and intermuscular coherence and body acceleration control in older adults during bipedal stance. Geriatrics 6, 114. doi: 10.3390/geriatrics6040114
Mochizuki, G., Semmler, J. G., Ivanova, T. D., and Garland, S. J. (2006). Low-frequency common modulation of soleus motor unit discharge is enhanced during postural control in humans. Exp. Brain Res. 175, 584–595. doi: 10.1007/s00221-006-0575-7
Mohr, M., Nann, M., von Tscharner, V., Eskofier, B., and Nigg, B. M. (2015). Task-dependent intermuscular motor unit synchronization between medial and lateral vastii muscles during dynamic and isometric squats. PLoS ONE 10, e0142048. doi: 10.1371/journal.pone.0142048
Moutzouri, M., Gleeson, N., Billis, E., Tsepis, E., Panoutsopoulou, I., and Gliatis, J. (2017). The effect of total knee arthroplasty on patients' balance and incidence of falls: a systematic review. Knee Surg. Sports Traumatol. Arthrosc. 25, 3439–3451. doi: 10.1007/s00167-016-4355-z
Nandi, T., Hortobágyi, T., van Keeken, H. G., Salem, G. J., and Lamoth, C. J. C. (2019). Standing task difficulty related increase in agonist-agonist and agonist-antagonist common inputs are driven by corticospinal and subcortical inputs respectively. Sci. Rep. 9, 2439. doi: 10.1038/s41598-019-39197-z
Nielsen, J. B., Brittain, J.-S., Halliday, D. M., Marchand-Pauvert, V., Mazevet, D., and Conway, B. A. (2008). Reduction of common motoneuronal drive on the affected side during walking in hemiplegic stroke patients. Clin. Neurophysiol. 119, 2813–2818. doi: 10.1016/j.clinph.2008.07.283
Noé, F., García-Massó, X., and Paillard, T. (2017). Inter-joint coordination of posture on a seesaw device. J. Electromyogr. Kinesiol. 34, 72–79. doi: 10.1016/j.jelekin.2017.04.003
Nojima, I., Suwa, Y., Sugiura, H., Noguchi, T., Tanabe, S., Mima, T., et al. (2020). Smaller muscle mass is associated with increase in EMG–EMG coherence of the leg muscle during unipedal stance in elderly adults. Hum. Mov. Sci. 71, 102614. doi: 10.1016/j.humov.2020.102614
Nojima, I., Watanabe, T., Saito, K., Tanabe, S., and Kanazawa, H. (2018). Modulation of EMG-EMG coherence in a choice stepping task. Front. Hum. Neurosci. 12, 50. doi: 10.3389/fnhum.2018.00050
Obata, H., Abe, M. O., Masani, K., and Nakazawa, K. (2014). Modulation between bilateral legs and within unilateral muscle synergists of postural muscle activity changes with development and aging. Exp. Brain Res. 232, 1–11. doi: 10.1007/s00221-013-3702-2
Roman-Liu, D. (2018). Age-related changes in the range and velocity of postural sway. Arch. Gerontol. Geriatr. 77, 68–80. doi: 10.1016/j.archger.2018.04.007
Saffer, M., Kiemel, T., and Jeka, J. (2008). Coherence analysis of muscle activity during quiet stance. Exp. Brain Res. 185, 215–226. doi: 10.1007/s00221-007-1145-3
Tanabe, H., Fujii, K., and Kouzaki, M. (2017). Joint coordination and muscle activities of ballet dancers during tiptoe standing. Motor Control 21, 72–89. doi: 10.1123/mc.2015-0002
Tricco, A. C., Lillie, E., Zarin, W., O'Brien, K. K., Colquhoun, H., Levac, D., et al. (2018). PRISMA extension for scoping reviews (PRISMA-ScR): checklist and explanation. Ann. Intern. Med. 169, 467–473. doi: 10.7326/M18-0850
Tyson, S., and Connell, L. (2009). How to measure balance in clinical practice. a systematic review of the psychometrics and clinical utility of measures of balance activity for neurological conditions. Clin. Rehabil. 23, 824–840. doi: 10.1177/0269215509335018
von Tscharner, V., Maurer, C., and Nigg, B. M. (2014). Correlations and coherence of monopolar EMG-currents of the medial gastrocnemius muscle in proximal and distal compartments. Front. Physiol. 5, 223. doi: 10.3389/fphys.2014.00223
Walker, S., Piitulainen, H., Manlangit, T., Avela, J., and Baker, S. N. (2020). Older adults show elevated intermuscular coherence in eyes-open standing but only young adults increase coherence in response to closing the eyes. Exp. Physiol. 105, 1000–1011. doi: 10.1113/EP088468
Watanabe, T., Saito, K., Ishida, K., Tanabe, S., and Nojima, I. (2018a). Coordination of plantar flexor muscles during bipedal and unipedal stances in young and elderly adults. Exp. Brain Res. 236, 1229–1239. doi: 10.1007/s00221-018-5217-3
Watanabe, T., Saito, K., Ishida, K., Tanabe, S., and Nojima, I. (2018b). Age-related declines in the ability to modulate common input to bilateral and unilateral plantar flexors during forward postural lean. Front. Hum. Neurosci. 12, 254. doi: 10.3389/fnhum.2018.00254
Watanabe, T., Saito, K., Ishida, K., Tanabe, S., and Nojima, I. (2018c). Fatigue-induced decline in low-frequency common input to bilateral and unilateral plantar flexors during quiet standing. Neurosci. Lett. 686, 193–197. doi: 10.1016/j.neulet.2018.09.019
Keywords: EMG-EMG coherence, intra-muscular coherence, inter-muscular coherence, standing control, scoping review
Citation: Yamanaka E, Horiuchi Y and Nojima I (2023) EMG-EMG coherence during voluntary control of human standing tasks: a systematic scoping review. Front. Neurosci. 17:1145751. doi: 10.3389/fnins.2023.1145751
Received: 16 January 2023; Accepted: 24 April 2023;
Published: 12 May 2023.
Edited by:
Mesut Sahin, New Jersey Institute of Technology, United StatesReviewed by:
Akshay Sujatha Ravindran, University of Houston, United StatesCopyright © 2023 Yamanaka, Horiuchi and Nojima. This is an open-access article distributed under the terms of the Creative Commons Attribution License (CC BY). The use, distribution or reproduction in other forums is permitted, provided the original author(s) and the copyright owner(s) are credited and that the original publication in this journal is cited, in accordance with accepted academic practice. No use, distribution or reproduction is permitted which does not comply with these terms.
*Correspondence: Ippei Nojima, bm9qaW1hQHNoaW5zaHUtdS5hYy5qcA==
Disclaimer: All claims expressed in this article are solely those of the authors and do not necessarily represent those of their affiliated organizations, or those of the publisher, the editors and the reviewers. Any product that may be evaluated in this article or claim that may be made by its manufacturer is not guaranteed or endorsed by the publisher.
Research integrity at Frontiers
Learn more about the work of our research integrity team to safeguard the quality of each article we publish.