- 1Division of Neurosurgery, Department of Neurology, University of São Paulo Medical School, São Paulo, Brazil
- 2Service of Interdisciplinary Neuromodulation, Instituto de Psiquiatria do Hospital das Clínicas da Faculdade de Medicina da Universidade de São Paulo, São Paulo, Brazil
- 3Instituto do Câncer do Estado de São Paulo, Hospital das Clínicas da Faculdade de Medicina da Universidade de São Paulo, São Paulo, Brazil
Introduction: The evaluation of brain plasticity can provide relevant information for the surgical planning of patients with brain tumors, especially when it comes to intrinsic lesions such as gliomas. Neuronavigated transcranial magnetic stimulation (nTMS) is a non-invasive tool capable of providing information about the functional map of the cerebral cortex. Although nTMS presents a good correlation with invasive intraoperative techniques, the measurement of plasticity still needs standardization. The present study evaluated objective and graphic parameters in the quantification and qualification of brain plasticity in adult patients with gliomas in the vicinity of the motor area.
Methods: This is a prospective observational study that included 35 patients with a radiological diagnosis of glioma who underwent standard surgical treatment. nTMS was performed with a focus on the motor area of the upper limbs in both the affected and healthy cerebral hemispheres in all patients to obtain data on motor thresholds (MT) and graphical evaluation by three-dimensional reconstruction and mathematical analysis of parameters related to the location and displacement of the motor centers of gravity (ΔL), dispersion (SDpc) and variability (VCpc) of the points where there was a positive motor response. Data were compared according to the ratios between the hemispheres of each patient and stratified according to the final pathology diagnosis.
Results: The final sample consisted of 14 patients with a radiological diagnosis of low-grade glioma (LGG), of which 11 were consistent with the final pathology diagnosis. The normalized interhemispheric ratios of ΔL, SDpc, VCpc, and MT were significantly relevant for the quantification of plasticity (p < 0.001). The graphic reconstruction allows the qualitative evaluation of this plasticity.
Conclusion: The nTMS was able to quantitatively and qualitatively demonstrate the occurrence of brain plasticity induced by an intrinsic brain tumor. The graphic evaluation allowed the observation of useful characteristics for the operative planning, while the mathematical analysis made it possible to quantify the magnitude of the plasticity.
Introduction
The relationship between morphological and functional neuroanatomy has been known for millennia (Duque-Parra, 2001) giving birth to maps of brain function (Sabbatini, 2004). During this time the observations of dynamic changes in this morpho-functional correlation has been described through concepts of neuroplasticity (Rosenzweig, 1996; Costandi, 2016). Several types of brain injuries can induce plasticity, such as vascular accidents, trauma, malformations, and neoplasms. While acute events offer a good perspective on areas directly related to primary functions, congenital malformations and progressive lesions allow an assessment of the intrinsic capacity of the neurodevelopmental brain to reorganize functions based on the absence or anomalies of anatomical structures (Herbet et al., 2016; Bourdillon et al., 2017; Mateos-Aparicio and Rodríguez-Moreno, 2019).
The evaluation of brain plasticity can provide useful information for treatment planning, as well as adjust prognostic expectations. In this sense, intracranial tumors, especially intrinsic lesions, due to their progressive nature, offer both a good field of study and a candidate to benefit from this information on neuroplasticity (Duffau, 2005; Desmurget et al., 2007; Fisicaro et al., 2016; Takakura et al., 2017).
The use of non-invasive methods for cortical mapping has captured increasing attention during the last decades with the navigated transcranial magnetic stimulation (nTMS) being one of the most used techniques, specially for its use on tumor resection planning and forecasting of eloquent involvement, since the good correlation of nTMS and direct cortical stimulation (Picht et al., 2016; Haddad et al., 2021; Sollmann et al., 2021). While the creation of cortical maps for surgical purposes is a consolidated matter, the use of objective parameters for quantifying the brain plasticity still needs further attention.
The aim of this study was to investigate possible measures that allows the evaluation of the magnitude of motor cortical plasticity induced by the presence of an intrinsic brain neoplasm in the vicinity of the motor cortex using nTMS.
Materials and methods
Setting
This prospective study recruited 35 adult patients with a single brain tumor with radiologic diagnosis of adult-type diffuse glioma in the vicinity of the pre-central gyrus, who underwent nTMS preoperative evaluations at a tertiary hospital in São Paulo, Brazil.
Preoperative clinical evaluation and brain tumor management
Muscle strength was assessed preoperatively, and the motor score was defined as upper extremities strengths of each hemibody according to Medical Research Council grade scale. Performance status was evaluated using Karnofsky Performance Status and Eastern Cooperative Oncology Group Scales (ECOG). The tumors were graded in low-grade glioma (LGG) and high-grade glioma (HGG) according to the presence of necrosis and contrast enhancement on the MRI.
All patients underwent standard surgical treatment and final histopathological data was recorded and stratified by OMS grading and LGG and HGG.
nTMS evaluation
During the week prior to the surgery participants underwent nTMS using structural MRI and infrared-based navigation system (Brainsight TMS 2.4.6, Canada) for guidance and registration of the stimulated points. The simulation was performed by applying single and paired pulses on the primary motor area (M1) corresponding to the cortical representation of hands and a radius of 5 cm around it, both on healthy and ill cerebral hemispheres, using a figure-of-eight stimulation coil (Magventure Tonica Elektronik, Farum, Denmark). Target points were identified when the hand movement was present using 65% TMS intensity. Muscle output due to cortical stimulation was evaluated using surface integrated electromyogram (EMG) electrodes attached to the muscles of hands (first dorsal interosseous) for determination of Resting Motor Threshold (RMT) as described previously in the literature (Rothwell et al., 1999; Groppa et al., 2012; Rossini et al., 2015). For the mapping registration of the response, considering the exploration of non M1 areas, single pulses were applied at 120% intensity of RMT and motor evoked potentials (MEP) were considered positive when any muscle twitch was present (all/none response). Then it was recorded if more than three activations out of five stimuli responses on the same point on a similar fashion of that performed during awake surgery (Morshed et al., 2021). All evaluations were performed by the same examiner.
Data evaluation and graphic representation
Four parameters were determined for the evaluation of the plastic effects. All graphic and the extraction of the coordinates were executed using the Brainsight software. Firstly, a reference point was set on the posterior margin of the precentral gyrus, aligned with the “omega” representing M1 on both the affected and healthy hemispheres, it was set manually by the average visual marking of principal examiner and the referred surgeon of the case in order to compensate for tumoral distortion, the setting of the point was defined as the apex of the precentral “omega” on the border of the central sulcus, when the distortion did not allow for visual identification of the precentral gyrus margin, a standardized stereotactic MNI map was used. Then, the Euclidian center of gravity was calculated for the positive motor points on both hemispheres, creating a center of gravity (CG) point. The first parameter, ΔL was the distance between the reference point and CG. The second parameter was the dispersion (SDpc) from all positive points regarding CG.
- SDpc: Dispersion (standard deviation) of all positive stimulated points.
- ΔPC: distance from positive stimulated point to center of gravity in each orthogonal plane.
- n: total of positive stimulated points.
- Xn; Yn; Zn: each orthogonal x,y,z axis reference for each positive stimulated point.
The third parameter was the variability of the same points (VCpc).
- VCpc: Variability (Variation Coefficient) of distances from each positive stimulated point to the center of gravity.
- SDpc: Dispersion (standard deviation) of all positive stimulated points.
- ΔPC: distance from stimulated point to center of gravity in each orthogonal plane.
- n: total of positive stimulated points.
- Xn; Yn; Zn: each orthogonal x,y,z axis reference for each positive stimulated point.
Finally, the RMT was defined as the fourth parameter. CG, ΔL, SDpc, and VCpc are graphically represented on Figure 1.
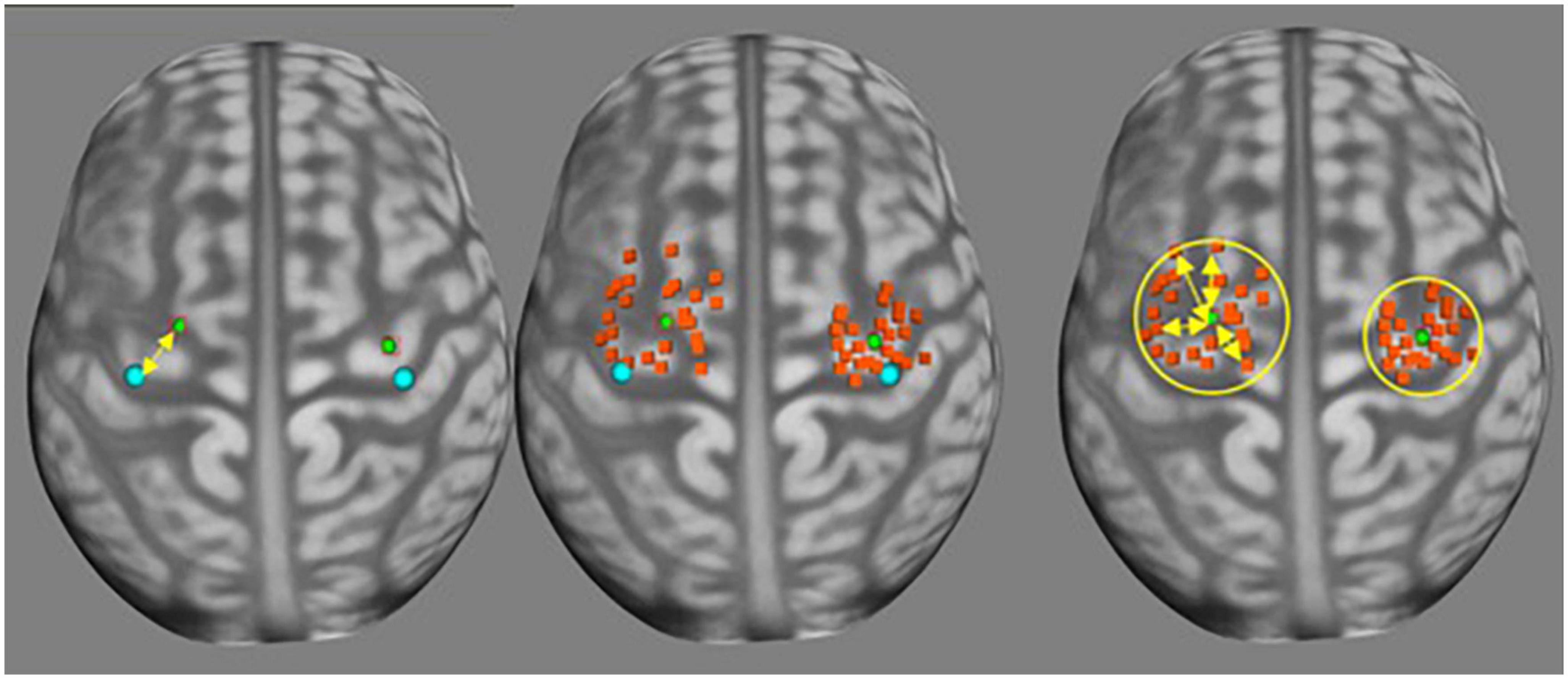
Figure 1. Demonstration of ΔL, SDpc, and VCpc over a standard tridimensional brain reconstruction. The blue dots are the reference points, green dots are CG points. The yellow arrow in the first panel represents ΔL. Orange points in the second and third panels are positive motor points. In the third panel, the yellow circle depicts an approximation of SDpc and the yellow arrows are the distances used for obtaining VCpc.
All values were compared by the ratio between the ill and healthy hemispheres. In order to access the magnitude of the difference, all ratios were fraction adjusted to values greater than 1.0. This mathematical maneuver allowed the comparison of the “amount” of change, regardless of the qualitative direction or characteristics of plasticity, i.e.: a ratio of 1.2 represents a difference of 20% among the ill and healthy hemispheres but does not imply it was an increase or decrease on the affected hemisphere. For the qualitative analysis, a graphic representation was used, and categorical factors regarding the affected hemisphere were defined. Closing and Distancing for ΔL; Dispersion and Concentration for SDpc; Homogeneous and Heterogeneous for VCpc; Increased or Decreased for RMT. Additional direction information–anterior, posterior, medial, lateral–was obtained by this analysis.
Due to the absence of data on most of the studied ratio parameters in healthy population, the reference for the expected normal ratios was obtained by the calculation of the normalized ratio of the average of all left healthy over the average of all right healthy hemispheres studied.
Ethical standard
This study was approved by Ethics and Research Committee of University of Sao Paulo Medical School and all individuals have signed an informed consent.
Statistical analysis
We compared the patients’ ratios with the control set using the Mann–Whitney U test. The Kruskal–Wallis test was performed to compare the studied variables regarding multiple categorical characteristics such as motor grading and performance status. Comparisons among the subgroups of patients according to brain tumor histopathology diagnosis [LGG vs. HGG and World Health Organization (WHO) grade I, II and III gliomas vs. glioblastomas (GBMs)] were performed using the Mann–Whitney test. Continuous variable normality was verified using Kolmogorov–Smirnov test. The analyses were performed using the Statistical Package for Social Sciences, version 28.0 (IBM Statistics, Armonk, NY, USA). The data were considered significant when p was < 0.05.
Results
Thirty-five patients were evaluated with complete nTMS and histopathological results. General characteristics of the patients are presented in Table 1. There were 23 male and 12 female patients with a mean age of 48.2 ± 15.9. The median KPS was 90 (ranging from 60 to 100) and the ECOG was 0 (range 3-0). The presence of motor deficit was observed in 15 patients (42.9%) with strengths 3 and 4, and none of the patients were complete or functionally hemiplegic. As both initial and ongoing symptom 17 patients presented seizures (48.6%), while 77% were in use of some antiepileptic drug. The preoperative assessment of the MRI indicated 23 patients with low-grade gliomas, while the histopathological results demonstrated 15 low-grade gliomas, all the eight patients with a final higher grade than expected presented a grade III tumor. Astrocytoma was the most common tumor type (15 patients, 42.9%), followed by glioblastoma (11 patients, 31.4%). All patients were naïve regarding not only brain tumor treatment but also any brain injuries, surgeries or intracranial treatment.
We observed that the adjusted ratios of ΔL, SDpc, VCpc, and RMT were significantly different from the expected normality. Suggesting a good capacity to identify neuroplastic changes, detailed in Table 2.
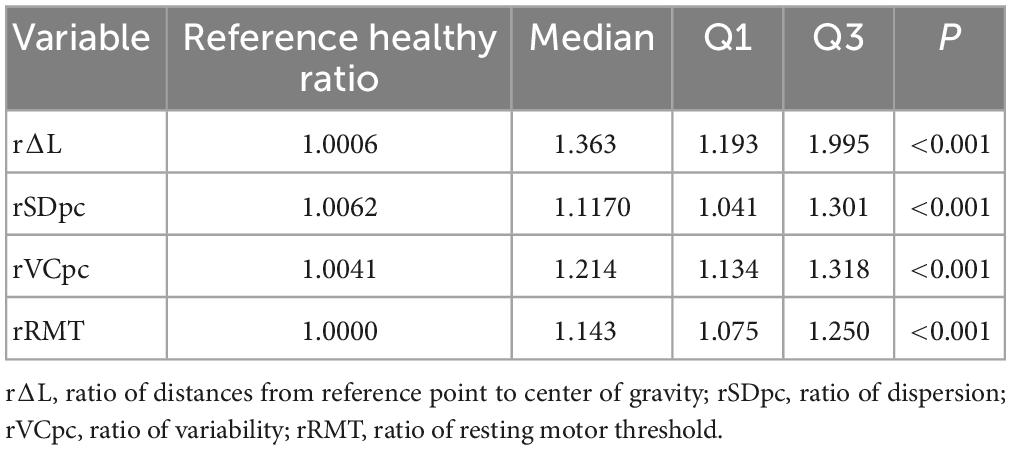
Table 2. Evaluation of significance and distribution of rΔL, rSDpc, rVCpc, and rRMT related to the control set.
Lower values of rΔL and higher values of rRMT were related to the presence of hemiparesis (p = 0.036 and p = 0.028, respectively), and the rRMT was also related to the grading of motor strength (p = 0.019) as shown in the Figures 2, 3 The performance status, and more importantly the ECOG value related directly to the rRMT (p = 0.028, Figure 4). There were no significant difference on rRMT regarding the occurrence of seizures, use of antiepileptic drugs.
Comparing the tumor grading, no statistically significant difference was found between LGG and HGG regarding any of the ratios parameters. We observed rRMT values were significantly higher in grade IV tumors compared to grades I, II and III (p = 0.025, Figure 5). A ROC analysis estimation determined an AUC of 0.737 with a threshold value of 1.169 rRMT value as cutoff for grade IV HGG. The analysis of independent factors to evaluate hemiparesis demonstrated a milder tendency of higher rRMT values in high grade gliomas, but with no statistical significance (grade p = 0.167/hemiparesis p = 0.388, Figure 6).
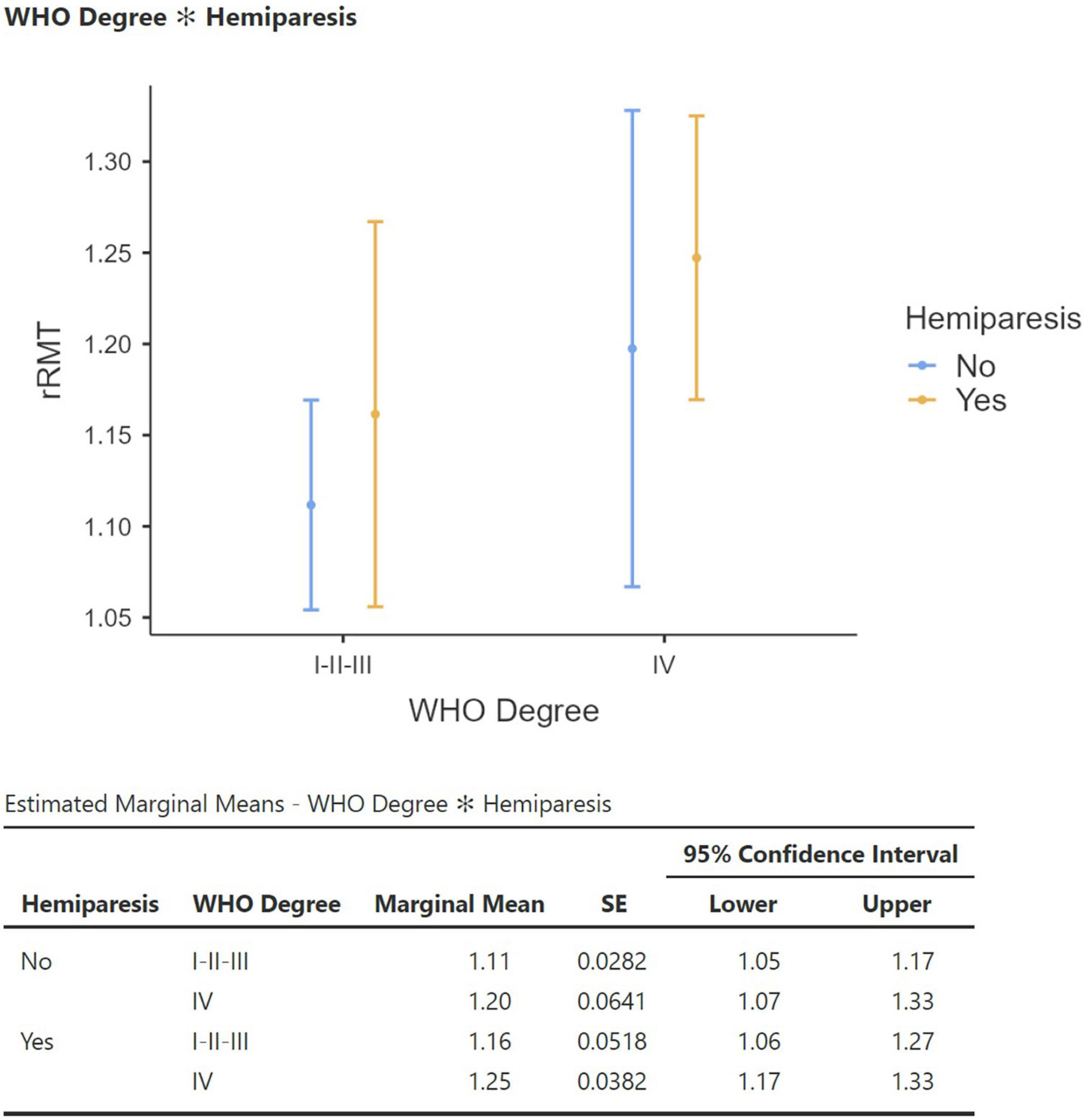
Figure 6. Estimated marginal means graph of linear regression of rRMT, tumor grading, and hemiparesis.
There was no relation observed to ratios of ΔL, SDpc, VCpc, and RMT to the location of the tumor, when studied both absolute–frontal lobe and parietal lobe–and relative–anterior, posterior, lateral or medial to the reference point. Also there was no pattern regarding the location or grading of the tumor and the qualitative plastic changes. On an individual case level, the graphic representation allowed the surgeon to access the direction of the motor points displacement, as demonstrated with representative cases in Figure 7.
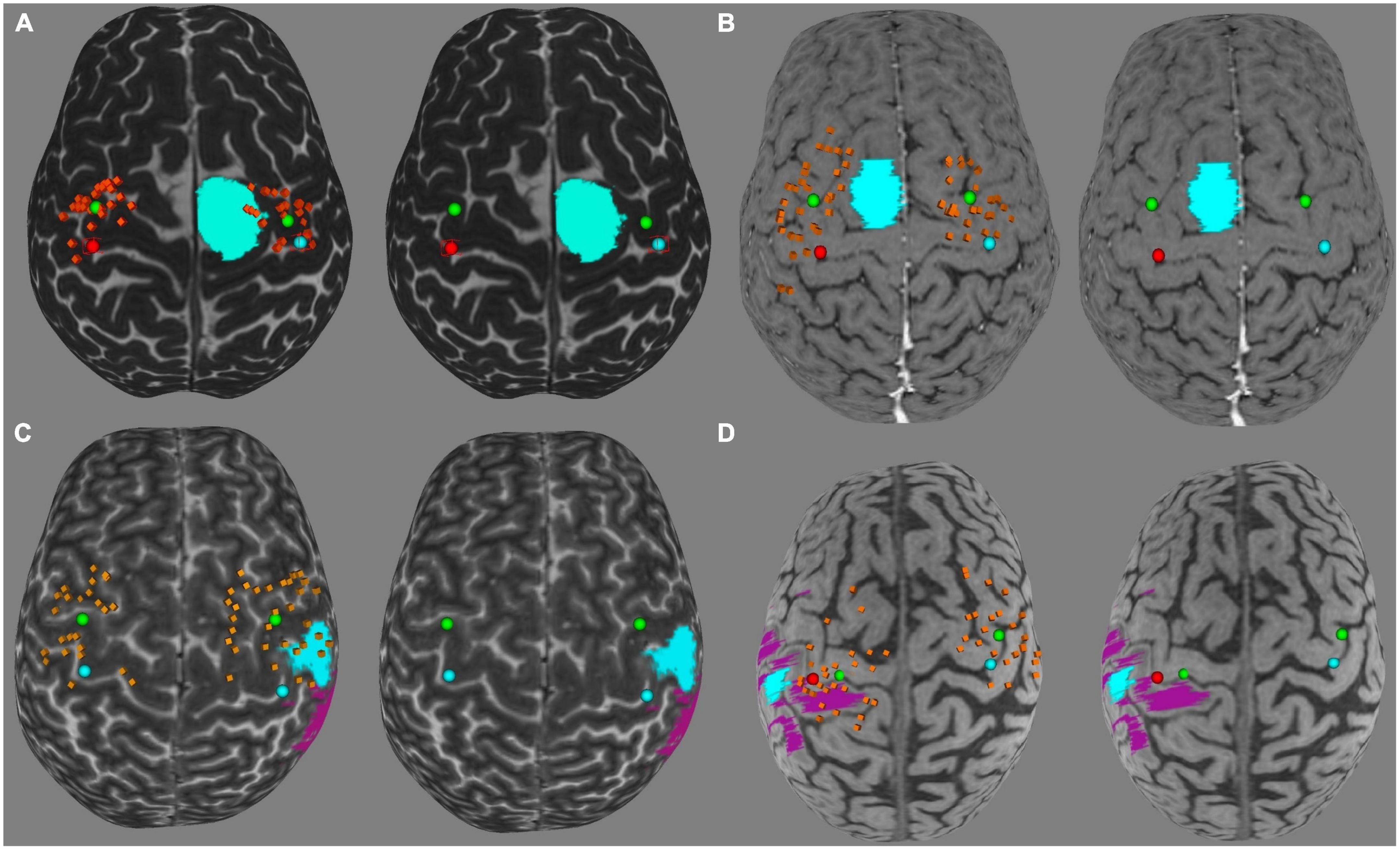
Figure 7. Graphic representation of four patients (A–D) mapping. The red and blue dots are the reference points, green dots are CG points, orange points are positive motor points. Light blue areas are cortical tumor delimitation and purple areas represents edema. Example of qualitative analysis on the tumor hemisphere compared to the healthy hemisphere: (A) closing, Homogeneous; (B) lateral, dispersion, Heterogeneous; (C) distancing, Heterogeneous; (D) posterior, medial, dispersion.
Discussion
This study sums to the current knowledge of brain plasticity in the context of brain tumors involving the primary motor cortex by evaluating graphic cortical maps representations already reported, as well as describing adjusted quantitative parameters as rRMT and, introducing ratio values of ΔL, SDpc, VCpc as possible markers of brain plasticity. The use of the normalized ratios was to our knowledge a novelty, and despite masking the qualitative evaluation of the absolute parameters, allows for a better interpatient comparison, mitigating previous dissonances in literature, such as reports of both higher and lower RMT ratios in the similar groups of glioma patients (Lavrador et al., 2020; Gomes dos Santos et al., 2021).
Regarding the dislocation of the CG, Conway et al. (2017) described that tumors located anterior to the precentral gyrus tend to generate displacements toward the tumor, while other authors saw the same effect but for tumors located in the parietal lobe (Takahashi et al., 2013; Bulubas et al., 2018), in both cases, the amount of displacement was not directly associated with the motor status. Our study focused on sheer difference among both sides, demonstrating that smaller rΔL were associated the presence of hemiparesis, the mechanism underlying these observations are still unclear, but two main hypothesis were be addressed during the evaluation of these patients: the first one is that despite the direction of the CG displacement, higher rΔL might represent slower and more important changes in the cortical representation, making the preservation of the motor strength possible, similarly to what is observed during long term rehabilitation (Desmurget et al., 2007). The second hypothesis considers that pathways of white matter tracts might also be affected and the migration of these pathways might recruit more sparse and farther cortical neurons (Duffau, 2022).
The capabilities of nTMS mapping regarding brain glioma surgery and it’s impacts on prognosis have been thoroughly demonstrated during the last years (Picht et al., 2016; Haddad et al., 2021; Schiavao et al., 2022). Our observation that the rRMT relates to the performance status reinforces that the preoperative mapping might someday be integrated to a broader prognostic tool. The grade IV tumor was also associated with higher rRMT, and while the possibility of tumor grade prediction by nTMS has been previously described (Lavrador et al., 2020; Neville et al., 2021), we propose an initial take on cutoff values that might be useful as an aid to the imaging methods, specifically for differentiating WHO grade IV tumors. The occurrence of hemiparesis alone was an important confounding factor, but even though there was still a tendency for higher rRMT values for grade IV tumors. Additionally, even though the remaining parameters were not significantly different among tumor grades, further studies with larger samples might also provide information for the clinically impactful differentiation of grade II and III tumors.
A limitation of our study is that the MEP and the motor twitch were only considered for hands and upper limbs, which might hinder the full evaluation of the motor system related gliomas. Another aspect that may be considered a limitation to our method was the use of the motor twitch alone for the creation of the map, while it allows for a broader evaluation of non-primary areas and was compensated by intrinsic control with the healthy hemisphere, a markedly high MEP might dislocate the CG in different patterns, the use of a MEP amplitude pondered CG might prove useful in future studies. Finally, despite the consecutive selection of patients within our service the non-evaluation of patients who were not candidates for surgery and the absence of patients with more severe motor deficits reflect a possible selection bias. The nature of a single evaluation precludes us from the evaluation of possible correlations of the plasticity parameters studied and the outcome of the treatment.
Conclusion
The nTMS was able to quantitatively and qualitatively demonstrate the occurrence of brain plasticity induced by an intrinsic brain tumor. The graphic evaluation allowed the observation of useful characteristics for the operative planning, while the mathematical analysis made it possible to quantify the magnitude of the plasticity.
Data availability statement
The raw data supporting the conclusions of this article will be made available by the authors, without undue reservation.
Ethics statement
The studies involving human participants were reviewed and approved by the Ethics and Research Committee of the University of São Paulo Medical School. The patients/participants provided their written informed consent to participate in this study. Written informed consent was obtained from the individual(s) for the publication of any potentially identifiable images or data included in this article.
Author contributions
CA, IN, CH, and WP: conception and design. IN and WP: patients recruitment. CA, CH, and AG: data collection. CA, IN, and WP: data analysis and interpretation. CA and IN: drafting the manuscript for important intellectual content. CH, AG, MT, and AB: text review. CA, IN, CH, AG, AB, MT, and WP: final approval. All authors contributed to the article and approved the submitted version.
Conflict of interest
The authors declare that the research was conducted in the absence of any commercial or financial relationships that could be construed as a potential conflict of interest.
Publisher’s note
All claims expressed in this article are solely those of the authors and do not necessarily represent those of their affiliated organizations, or those of the publisher, the editors and the reviewers. Any product that may be evaluated in this article, or claim that may be made by its manufacturer, is not guaranteed or endorsed by the publisher.
References
Bourdillon, P., Apra, C., Guénot, M., and Duffau, H. (2017). Similarities and differences in neuroplasticity mechanisms between brain gliomas and nonlesional epilepsy. Epilepsia 58, 2038–2047. doi: 10.1111/epi.13935
Bulubas, L., Sollmann, N., Tanigawa, N., Zimmer, C., Meyer, B., and Krieg, S. (2018). Reorganization of motor representations in patients with brain lesions: A navigated transcranial magnetic stimulation study. Brain Topogr. 31, 288–299. doi: 10.1007/s10548-017-0589-4
Conway, N., Wildschuetz, N., Moser, T., Bulubas, L., Sollmann, N., Tanigawa, N., et al. (2017). Cortical plasticity of motor-eloquent areas measured by navigated transcranial magnetic stimulation in patients with glioma. J. Neurosurg. 127, 981–991. doi: 10.3171/2016.9.JNS161595
Costandi, M. (2016). Neuroplasticity. Cambridge, MA: The MIT Press. doi: 10.7551/mitpress/10499.001.0001
Desmurget, M., Bonnetblanc, F., and Duffau, H. (2007). Contrasting acute and slow-growing lesions: A new door to brain plasticity. Brain 130, 898–914. doi: 10.1093/brain/awl300
Duffau, H. (2005). Lessons from brain mapping in surgery for low-grade glioma: Insights into associations between tumour and brain plasticity. Lancet Neurol. 4, 476–486. doi: 10.1016/S1474-4422(05)70140-X
Duffau, H. (2022). White matter tracts and diffuse lower-grade gliomas: The pivotal role of myelin plasticity in the tumor pathogenesis, infiltration patterns, functional consequences and therapeutic management. Front. Oncol. 12:855587. doi: 10.3389/fonc.2022.855587
Duque-Parra, J. (2001). Functional neuroanatomy: The first daughter of neuroscience and the mother of neural science. Anat. Rec. 265, 250–253. doi: 10.1002/ar.10037
Fisicaro, R., Jost, E., Shaw, K., Brennan, N., Peck, K., and Holodny, A. (2016). Cortical plasticity in the setting of brain tumors. Top. Magn. Reson. Imaging 25, 25–30. doi: 10.1097/RMR.0000000000000077
Gomes dos Santos, A., Hayashi, C., de Almeida, C., Paiva, W., de Andrade, D., Galhardoni, R., et al. (2021). Letter: Altered motor excitability in patients with diffuse gliomas involving motor eloquent areas: The impact of tumor grading. Neurosurgery 88, E302–E303. doi: 10.1093/neuros/nyaa512
Groppa, S., Oliviero, A., Eisen, A., Quartarone, A., Cohen, L., Mall, V., et al. (2012). A practical guide to diagnostic transcranial magnetic stimulation: Report of an IFCN committee. Clin. Neurophysiol. 123, 858–882. doi: 10.1016/j.clinph.2012.01.010
Haddad, A., Young, J., Berger, M., and Tarapore, P. (2021). Preoperative applications of navigated transcranial magnetic stimulation. Front. Neurol. 11:628903. doi: 10.3389/fneur.2020.628903
Herbet, G., Maheu, M., Costi, E., Lafargue, G., and Duffau, H. (2016). Mapping neuroplastic potential in brain-damaged patients. Brain 139, 829–844. doi: 10.1093/brain/awv394
Lavrador, J., Gioti, I., Hoppe, S., Jung, J., Patel, S., Gullan, R., et al. (2020). Altered motor excitability in patients with diffuse gliomas involving motor eloquent areas: The impact of tumor grading. Neurosurgery 88, 183–192. doi: 10.1093/neuros/nyaa354
Mateos-Aparicio, P., and Rodríguez-Moreno, A. (2019). The impact of studying brain plasticity. Front. Cell. Neurosci. 13:66. doi: 10.3389/fncel.2019.00066
Morshed, R., Young, J., Lee, A., and Hervey-Jumper, S. (2021). Functional mapping for glioma surgery, part 2. Neurosurg. Clin. N. Am. 32, 75–81. doi: 10.1016/j.nec.2020.09.001
Neville, I., Gomes dos Santos, A., Almeida, C., Hayashi, C., Solla, D., Galhardoni, R., et al. (2021). Evaluation of changes in preoperative cortical excitability by navigated transcranial magnetic stimulation in patients with brain tumor. Front. Neurol. 11:582262. doi: 10.3389/fneur.2020.582262
Picht, T., Frey, D., Thieme, S., Kliesch, S., and Vajkoczy, P. (2016). Presurgical navigated TMS motor cortex mapping improves outcome in glioblastoma surgery: A controlled observational study. J. Neurooncol. 126, 535–543. doi: 10.1007/s11060-015-1993-9
Rosenzweig, M. (1996). Aspects of the search for neural mechanisms of memory. Annu. Rev. Psychol. 47, 1–32. doi: 10.1146/annurev.psych.47.1.1
Rossini, P., Burke, D., Chen, R., Cohen, L., Daskalakis, Z., di Iorio, R., et al. (2015). Non-invasive electrical and magnetic stimulation of the brain, spinal cord, roots and peripheral nerves: Basic principles and procedures for routine clinical and research application. An updated report from an I.F.C.N. committee. Clin. Neurophysiol. 126, 1071–1107. doi: 10.1016/j.clinph.2015.02.001
Rothwell, J., Hallett, M., Berardelli, A., Eisen, A., Rossini, P., and Paulus, W. (1999). Magnetic stimulation: Motor evoked potentials. The international federation of clinical neurophysiology. Electroencephalogr. Clin. Neurophysiol. Suppl. 52, 97–103.
Sabbatini, R. (2004). The history of electrical stimulation of the brain. Brain and mind magazine. Available online at: http://www.cerebromente.org.br/n18/history/stimulation_i.htm (accessed July 10, 2018).
Schiavao, L., Neville Ribeiro, I., Yukie Hayashi, C., Gadelha Figueiredo, E., Russowsky Brunoni, A., Jacobsen Teixeira, M., et al. (2022). Assessing the capabilities of transcranial magnetic stimulation (TMS) to aid in the removal of brain tumors affecting the motor cortex: A systematic review. Neuropsychiatr. Dis. Treat. 18, 1219–1235. doi: 10.2147/NDT.S359855
Sollmann, N., Krieg, S., Säisänen, L., and Julkunen, P. (2021). Mapping of motor function with neuronavigated transcranial magnetic stimulation: A review on clinical application in brain tumors and methods for ensuring feasible accuracy. Brain Sci. 11:897. doi: 10.3390/brainsci11070897
Takahashi, S., Vajkoczy, P., and Picht, T. (2013). Navigated transcranial magnetic stimulation for mapping the motor cortex in patients with rolandic brain tumors. Neurosurg. Focus 34:E3. doi: 10.3171/2013.1.FOCUS133
Keywords: transcranial magnetic stimulation, glioma, neurosurgery, neuronavigation guided, brain neoplasms, neuronal plasticity (MeSH), cerebral cortex
Citation: Almeida CC, Neville IS, Hayashi CY, Gomes dos Santos A, Brunoni AR, Teixeira MJ and Paiva WS (2023) Quantification of tumor induced motor cortical plasticity using navigated transcranial magnetic stimulation in patients with adult-type diffuse gliomas. Front. Neurosci. 17:1143072. doi: 10.3389/fnins.2023.1143072
Received: 12 January 2023; Accepted: 27 February 2023;
Published: 15 March 2023.
Edited by:
Yen-Feng Lin, National Health Research Institutes, TaiwanReviewed by:
Sudhakar Tummala, The University of Texas MD Anderson Cancer Center, United StatesYvonne Dzierma, Saarland University Hospital, Germany
Copyright © 2023 Almeida, Neville, Hayashi, Gomes dos Santos, Brunoni, Teixeira and Paiva. This is an open-access article distributed under the terms of the Creative Commons Attribution License (CC BY). The use, distribution or reproduction in other forums is permitted, provided the original author(s) and the copyright owner(s) are credited and that the original publication in this journal is cited, in accordance with accepted academic practice. No use, distribution or reproduction is permitted which does not comply with these terms.
*Correspondence: Cesar Cimonari de Almeida, Y2VzYXIuY2E4N0BnbWFpbC5jb20=