- 1Instituto de Ciências Biológicas, Universidade Federal do Pará, Belém, Brazil
- 2Department of Integrative Biology, Michigan State University, East Lansing, MI, United States
- 3Department of Biological Sciences, Louisiana State University, Baton Rouge, LA, United States
- 4Instituto Tecnologico Vale, Belém, Brazil
The development of the vertebrate eye is a complex process orchestrated by several conserved transcriptional and signaling regulators. Aside from partial or complete loss, examples of exceptional modifications to this intricate organ are scarce. The unique eye of the four-eyed fish Anableps anableps is composed of duplicated corneas and pupils, as well as specialized retina regions associated with simultaneous aerial and aquatic vision. In a previous transcriptomic study of the A. anableps developing eye we identified expression of twenty non-visual and eleven visual opsin genes. Here, we surveyed the expression territories of three non-visual melanopsins genes (opn4×1, opn4×2, opn4m3), one teleost multiple tissue opsin (tmt1b) and two visual opsins (lws and rh2-1) in dorsal and ventral retinas. Our data showed that asymmetry of non-visual opsin expression is only established after birth. During embryonic development, while inside pregnant females, the expression of opn4×1, opn4×2, and tmt1b spans the whole retina. In juvenile fish (post birth), the expression of opn4×1, opn4×2, opn4m3, and tmt1b genes becomes restricted to the ventral retina, which receives aerial light. Raising juvenile fish in clear water instead of the murky waters found in its natural habitat is sufficient to change gene expression territories of opn4×1, opn4×2, opn4m3, tmt1b, and rh2-1, demonstrating that different lighting conditions can shift opsin expression and potentially contribute to changes in spectral sensitivity in the four eyed fish.
Introduction
Change in environmental light is an important driving force for the diversity of visual systems in vertebrates. Aquatic animals occupy a great range of visual environments and have evolved adaptations to increase sensitivity to the availability of light (Collin, 2009). Evolutionary and developmental adaptations to the visual system have ensured success in avoiding predation, establishing feeding behavior and finding sexual partners (Jeffery, 2020; Thomas et al., 2020). For example, cichlid fishes show a versatile visual system with a complex sensory plasticity that regulates reproductive, feeding, and social behavior (Butler et al., 2019; Maruska and Butler, 2021). Substantial changes to the general structure of the vertebrate eye are rare, and include total or partial loss of the eyes, as seen in cavefishes, and the specialized retinas of the deep-water fish (Warrant and Locket, 2004; Biagioni et al., 2016; Lunghi et al., 2019).
Attempt in eye duplication is a rare event observed in only a few fish lineages such as Dialommus fuscus and Dialommus macrocephalus, also known as “Rockskipper,” these fish are terrestrial predators and have a vertically split cornea that allows them to remain upright with one cornea in and the other out of the water. In addition, Bathylychnops exilis, inhabits depths between 600 and 3,000 ft, and has an auxiliary globe in addition to the main eyeball directed toward the ocean floor (Schwab et al., 2001). Species from the genus Anableps are also a remarkable example of extreme adaptation of the visual system. With a partially duplicated eye, simultaneous aerial and aquatic vision, and a single optic nerve, Anableps consists in a unique model to understand the genetic bases of eye duplication (Figure 1A; Schwab et al., 2001; Owens et al., 2012; Perez et al., 2017).
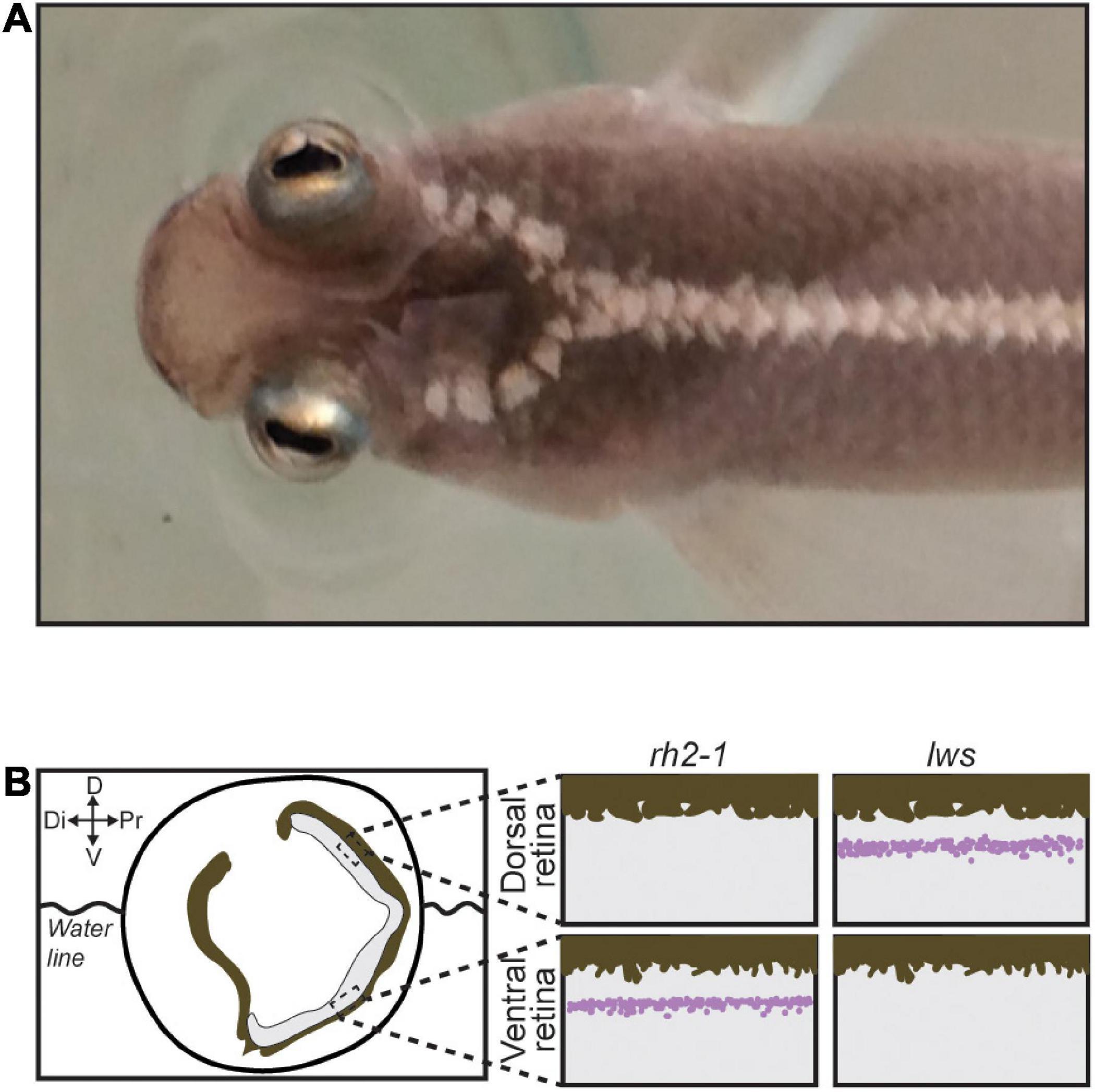
Figure 1. The Anableps anableps. (A) Anableps anableps female specimen, dorsal close-up view of the A. anableps head and part of the body. (B) Schematic of the A. anableps eye showing the water line and asymmetric gene expression of lws and rh2-1 genes in the dorsal and ventral retinas, respectively. Dorsal (D), Di (Distal), V (Ventral), and Pr (Proximal).
Morphological innovations to the visual system require molecular changes in photoreception regulation. The distribution of photoreceptor cells and the composition of rods and cones play an important role in spectral sensitivity in teleost fish (Okano et al., 1994; Collin, 2009; Sato et al., 2018).
Membrane proteins known as visual opsins are present in retina cells and act as light sensors. Coupled with chromophores, these proteins mediate light detection and the initiation of visual processing in the photoreceptor cells (Wald, 1968; Yokoyama, 2000). Conversely, non-visual opsins, although expressed in the inner retina among other tissues in the vertebrate body, do not participate directly in image formation and instead respond to light by activating a series of non-visual sensory responses. In fish, these proteins are present in the pineal complex, epidermal photoreceptors and in the retina nuclear layers and play an important role in light detection, neural development and control of the circadian rhythm (Okano et al., 1994; Kojima and Fukada, 1999; Van Gelder, 2001; Peirson et al., 2009).
The melanopsins are the most widely studied non-visual opsins (Peirson et al., 2009; Diaz et al., 2016; Van Gelder and Buhr, 2016; Guido et al., 2020). In zebrafish (Danio rerio), expression of opn4×1a, opn4×1b, and opn4m3 are detected in horizontal, bipolar and amacrine cells while a small subgroup of retinal ganglion cells expresses opn4×1 and opn4m3. The expression of opn4m3 was also detected in the zebrafish retinal pigment epithelial (RPE) (Davies et al., 2011). In the retina cells of salmon fish (Salmo salar), five melanopsin genes were observed: opn4×1a and opn4×1b, opn4m1a1, opn4m1a2, and opn4m2 (Sandbakken et al., 2012). The non-visual opsin multiple tissue of teleosts (tmt) is expressed in the eyes, brain, liver, kidneys, and heart of fugu (Fugu rubripes) and zebrafish and in the brain and eyes of medaka (Oryzias latipes) (Moutsaki et al., 2003; Fischer et al., 2013). Previous studies have demonstrated that tmt1 and tmt2 opsins act as a blue light-sensitive Gi/Go-coupled receptors but exhibited spectral properties and photo-convertibility of the active state different from each other (Diaz et al., 2016; Guido et al., 2020). While non-visual opsins are expressed in the retina cells of many fish species, the exact role of these proteins and their physiological functions remain to be elucidated (Diaz et al., 2016; Guido et al., 2020).
Asymmetric gene expression of opsin genes is another way of modulating visual sensitivity, and this mechanism plays an important role in the diversification of visual pigments in fish, leading to changes in spectral sensitivity (Hofmann and Carleton, 2009; Hauser and Chang, 2017). In addition, modulation of spectral sensitivity could also occur as part of a short-term plastic response to environmental changes, as observed in the eyes of the four eyed fish Anableps anableps.
Anableps are livebearers; female fish give birth to fully developed progeny, similarly to mammals. Previous work from our group has shown that the establishment of asymmetric expression of visual opsins (rh2-1 and lws) occurs prior to birth and is independent of photic input, suggesting that it is genetically programmed (Perez et al., 2017). Here, we show that opn4×1, opn4×2, and tmt1b are expressed symmetrically in the entire retina of the developing eye of the A. anableps during larval stages. In the juvenile fish, tmt1b, opn4×1, opn4×2, and opn4m3 shift the expression domain and become restricted to the ventral retina. A. anableps inhabit murky waters of the Amazon River and its tributaries (Supplementary Video 1). However, when animals were maintained in aquaria with clear water, we observed loss of opn4×2 expression in the retina, and a shift in opn4×1, opn4m3, and tmt1b expression domains, from being restricted to the ventral retina, to being expanded dorsally and occupying the entire retina, recapitulating the expression pattern of the developing eye during larval stages.
Materials and methods
Sample collection
Specimens of A. anableps were collected by seine net in two locations: Abaetetuba, Pará, Brazil (1°42′24.9″S 48°53′46.4″W) and Bragança, Pará, Brazil (0°53′27.6″S 46°39′21.9″W). Animals were euthanized using MS-222 (Sigma-Aldrich Corp., Milwaukee, WI, USA) followed by decapitation.
Histological analysis
Eyes of wild-caught A. anableps juvenile (up to 15 cm long) and larvae were collected and flash frozen in Tissue-Tek® O.C.T TM embedding medium (Sakura Finetek USA Inc., Torrance, CA, USA). Sagittal cryosections (20 μm) of the eyes were obtained using CM1850 UV cryostat (Leica Biosystems Nussloch GmbH, Nussloch, BW, Germany) and collected on ColorFrost Plus microscope slides (Thermo Fisher Scientific, Pittsburgh, PA, USA), fixed in 3% paraformaldehyde (Sigma-Aldrich Inc., St. Louis, MO, USA) and stored at −80°C for further use.
Lighting environment conditions
Eight juvenile fish were kept in a 75 Gallon glass aquarium with UV filter and standard fluorescent light (40 watts); fish were kept in dechlorinated tap water at 28°C with aeration and biological and mechanical filtration and fed daily with fish ration. Fish were exposed to a day and night cycle of 12 h for 30 days followed by euthanasia, according to Fuller and Claricoates (Fuller and Claricoates, 2011; Supplementary Video 2).
Sagittal sections (20 μm) were obtained using CM1850 UV cryostat (Leica Biosystems Nussloch GmbH, Nussloch, BW, Germany), placed on ColorFrost Plus microscope slides (Thermo Fisher Scientific, Pittsburgh, PA, USA) and stored at −80°C for further use. Eight wild-caught juvenile fish were used as control for these experiments.
Riboprobe synthesis
To produce riboprobes for in situ hybridization for the genes opn4m3, opn4×1, opn4×2, and tmt1b, we adapted a PCR-based technique for the preparation of riboprobe templates (David and Wedlich, 2001). First, vectors containing target gene sequences were synthesized in pBlueScript II SK(+) by BioCat GmbH (Heidelberg, Germany). Next, these vectors were used as a template on a PCR reaction. The T7 promoter sequence was included at the 5′ end of each gene-specific forward primer, whereas the SP6 promoter sequence was added to the 5′ end of each gene-specific reverse primer. The resulting amplicons for each target gene were subsequently used as template for sense (T7) or antisense (SP6) riboprobe synthesis. Primers (with promoter sequences underlined) were: opn4m3-Forward: 5′ TAATACGACTCACTATAGGCTCGTCTGAG GTGGTTTTAGG 3 and reverse: 5′ ATTTAGGTGACAC TATAGTGTAGGAGCAGGGTGGAGAAG 3′, opn4×1-Forward: 5′TAATACGACTCACTATAGCAAAGAAGGCAAC GATGTAGTGA 3′ and reverse: 5′ ATTTAGGTGACACTA TAGACACGGATTCTATCGGCATGT 3′, opn4×2-Forward: 5′ TAATACGACTCACTATAGCCATTCCTTGTAGAGGCAGTT GATA 3′ and reverse: 5′ ATTTAGGTGACACTATAGGCGACT TCCTCATGGCTTTC3′, tmt1b-Forward: 5′ TAATACGA CTCACTATAGCAGTGGTCGTCCGAAAATGG 3′ and reverse: 5′ ATTTAGGTGACACTATAGGCGGTTCAAAGGG ATTACTGT 3′.
To produce riboprobes for the visual opsins lws and rh2-1, we used as templates vectors previously described (Perez et al., 2017). The pCRII-TOPO vector (Thermo Fisher Scientific, Pittsburgh, PA, USA) containing the target gene sequence was used as a template in a PCR reaction with M13 forward (5′ TAAAACGACGGCCAG-3′) and M13 reverse (5′-CAGGAAACAGCTATGAC 3′) primers. The resulting amplicon contained the sequence of the gene of interest flanked by an upstream T7 promoter and a downstream SP6 promoter. Sense and antisense probes were produced using T7 and SP6 RNA polymerases, respectively. Riboprobe reaction was performed using DIG-labeling mix (Roche Diagnostics Deutschland GmbH, Mannheim, BW., GER.) and according to manufacturer’s protocol (mMESSAGE mMACHINE Transcription kits, Ambion, Carlsbad, CA, USA).
In situ hybridization
In situ hybridization was performed according to previously established protocol (Lovell et al., 2013; Carleton et al., 2014; De Lima et al., 2015). Cryosections from heads A. anableps specimens were acetylated for 10 min in a solution of 0.33% acetic anhydride in DEPC-treated water, rinsed with 2× SSPE buffer (0.02 M EDTA and 2.98 M NaCl in 0.2 M phosphate buffer) and dehydrated in 70, 95, and 100% RNase-free ethanol. Each section was then hybridized with a solution (32 μL) containing 50% formamide, 2× SSPE, 1 μg/μl BSA, 1 μg/μL poly(A) (Sigma-Aldrich Corp., Milwaukee, WI, USA) in DEPC-treated water, and 1 μL of the DIG-labeled riboprobe. Slides were cover slipped, sealed by immersion in mineral oil, and incubated overnight at 65°C. Next, sections were rinsed in chloroform, de-cover slipped in 2× SSPE, and incubated serially in 2× SSPE/50% formamide, and in 0.1× SSPE at 65°C. Sections were then blocked for 30 min at RT in blocking buffer with 1% skim milk and incubated overnight with an alkaline phosphatase-conjugated anti-DIG antibody (Roche Diagnostics Deutschland GmbH, Mannheim, BW, Germany) Slides were washed twice for 15 min in TMN (100 mM Tris, pH 9.5; 150 mM NaCl, 0.05 M MgCl2), and incubated in a detection solution containing the alkaline phosphatase substrates Nitro-Blue Tetrazolium Chloride (NBT) (Roche Diagnostics Deutschland GmbH, Mannheim, BW, Germany). and 5-Bromo-4-Chloro-3-Indolyl-phosphate p-Toluidine Salt (BCIP) (Roche Diagnostics Deutschland GmbH, Mannheim, BW, Germany) Slides were washed overnight, fixed in 4% paraformaldehyde (dissolved in PBS) and cover slipped with Cytoseal (Thermo Fisher Scientific, Pittsburgh, PA, USA). Slides were imaged using a Nikon SMZ1500 microscope and processed on NIS-Elements D 4.10.01 program (Nikon Instruments Inc., Melville, NY, USA).
Results
Non-visual opsins are expressed symmetrically in the developing eye of Anableps anableps prior to birth
Our previous work identified twenty non-visual and eleven visual opsins expressed during the development of the eye in the four-eyed fish (Beaudry et al., 2017; Perez et al., 2017). Here, in situ hybridization was performed to examine the expression domains of three types of melanopsin subfamily of genes, opn4×1, opn4×2, opn4m3, and one type of teleost multiple tissue opsin, tmt1b, in the developing retina of pre-birth larvae. Our results showed that opn4×1, opn4×2, and tmt1b are expressed uniformly in the outer nuclear layer (ONL), inner nuclear layer (INL), and ganglion cell layer (GCL) in both dorsal and ventral regions of the retina (Figures 2A–D,G,H). The expression of opn4m3 was not detected by in situ hybridization at stage 5 (Figures 2E,F).
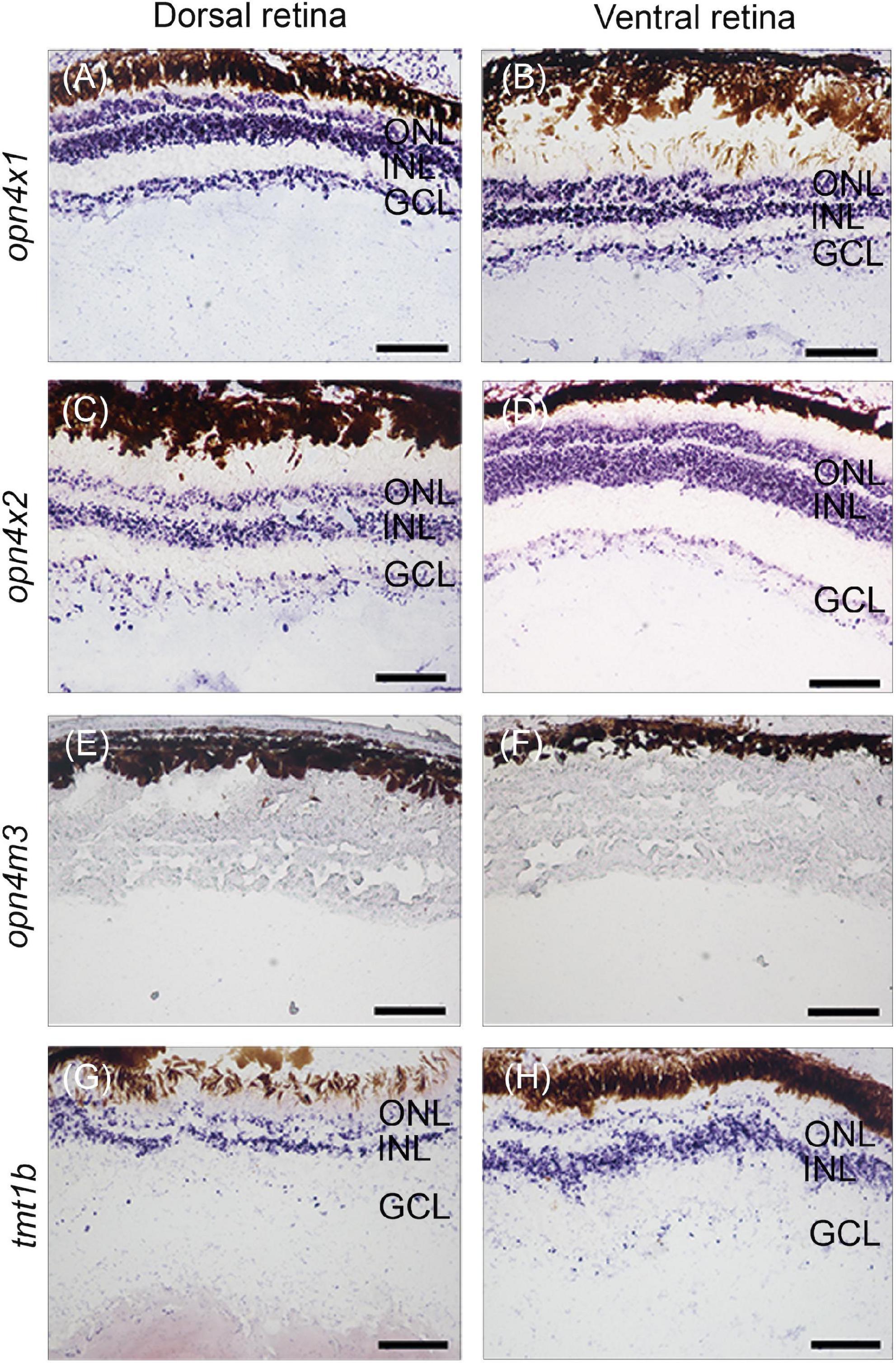
Figure 2. Non-visual opsin gene expression in the larval retina of Anableps anableps. In situ hybridizations for opn4×1 (A,B), opn4×2 (C,D), opn4m3 (E,F), and tmt1b (G, H) were performed in eyes of larvae collected before birth. Sections of the dorsal retina are on the left, and ventral retina to the right. ONL, outer nuclear layer; INL, inner nuclear layer; GCL, ganglion cell layer. Scale bars: 0.05 mm.
Expression domains of visual and non-visual opsins change upon different lighting conditions in juvenile Anableps anableps
We have previously shown that in the A. anableps, asymmetric expression of lws and rh2.1 in the dorsal and ventral regions of the retina, respectively, is established prior to birth in photoreceptor cells (Perez et al., 2017). Here, we wanted to investigate the plasticity of visual and non-visual opsins in the retina upon changes in environmental lighting. To this end, juvenile fish were maintained in clear water for 30 days and surveyed for visual and non-visual opsin gene expression using RNA in situ hybridization.
In wild-caught juvenile A. anableps found in murky river water, the expression of opn4×1 (Figures 3A,B) and opn4×2 (Figures 3E,F) is restricted to the ONL and INL of the ventral retina while opn4m3 and tmt1b are expressed in the INL, ONL and GCL of the ventral retina (Figures 3I,J,M,N). We next used a 30-day light-induced cycle and assayed opsin gene expression. Our results showed that expression of opn4×1 and tmt1b, restricted to the ONL and INL in the ventral retina in wild-caught fish, expands to the ONL, INL and GCL in the dorsal retina (Figures 3C,D,O,P); opn4×2 expression is lost in the entire retina (Figures 3G,H) while opn4m3 expression becomes sparse in the ONL, INL and GCL of the ventral retina (Figures 3K,L).
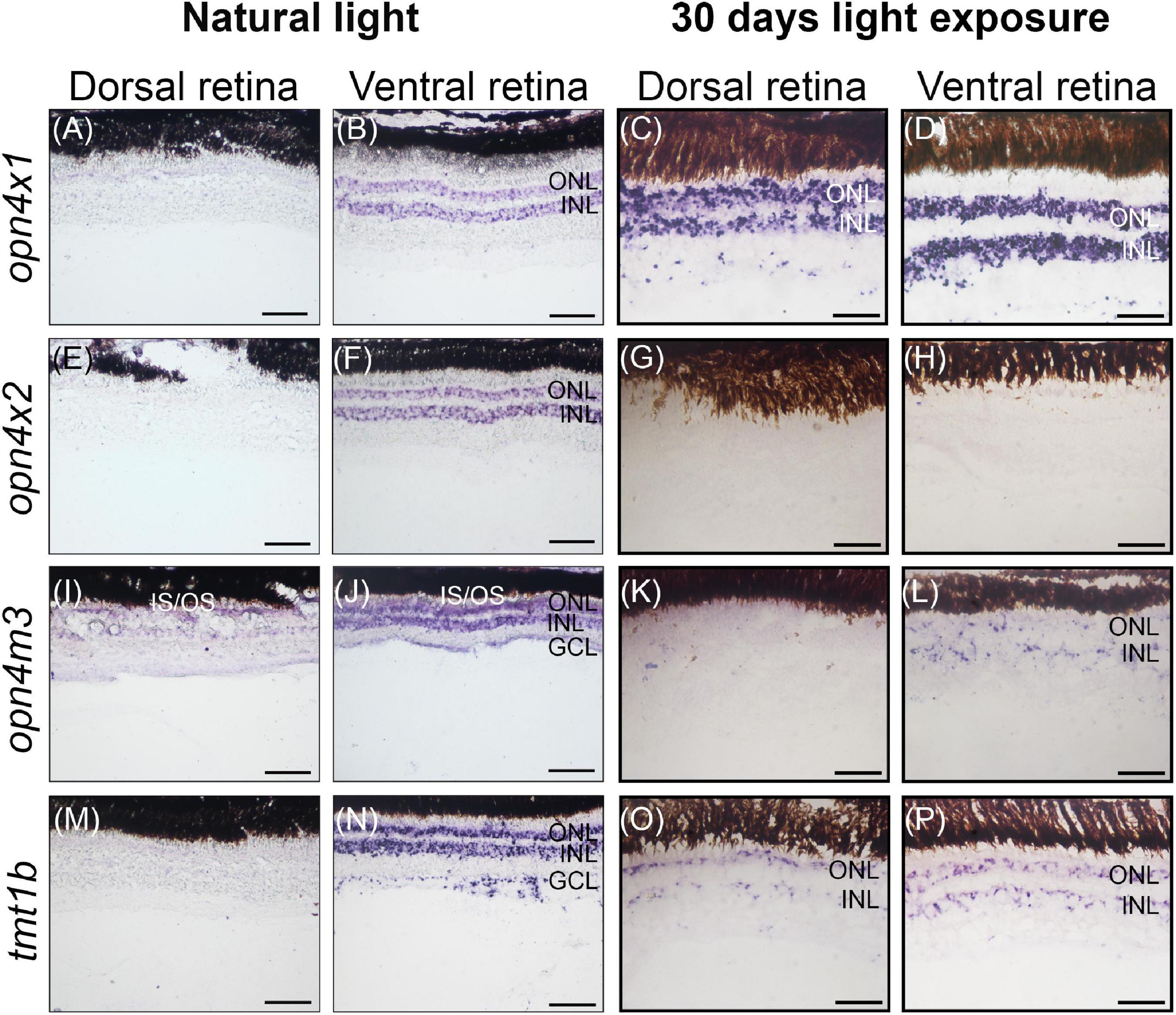
Figure 3. Non-visual opsin gene expression in the juvenile retina of Anableps anableps. In situ hybridizations of opn4×1 (A–D), opn4×2 (E–H), opn4m3 (I–L), and tmt1b (M–P) were performed in A. anableps’ eyes from wild-caught juvenile fish under natural light conditions (A,B,E,F,I,J,M,N) and juveniles that were kept for 30 days in light exposure from above and below the water (C,D,G,H,K,L,O,P). The panel shows sections of the dorsal retina and ventral retina. ONL, outer nuclear layer; INL, inner nuclear layer; GCL, ganglion cell layer; IS, photoreceptor inner segments; OS, photoreceptor outer segments. Scale bars: 0.05 mm.
We next assayed the lws and rh2-1 visual opsins, which show expression domains restricted to the dorsal and ventral retinas, respectively (Perez et al., 2017). Upon light-induced exposure, in situ hybridization showed that lws expression remained in the photoreceptor layer of the dorsal retina, however, rh2-1 expression expanded to the photoreceptor layer of ventral and dorsal retina territories (Figures 1B, 4A–D for reference).
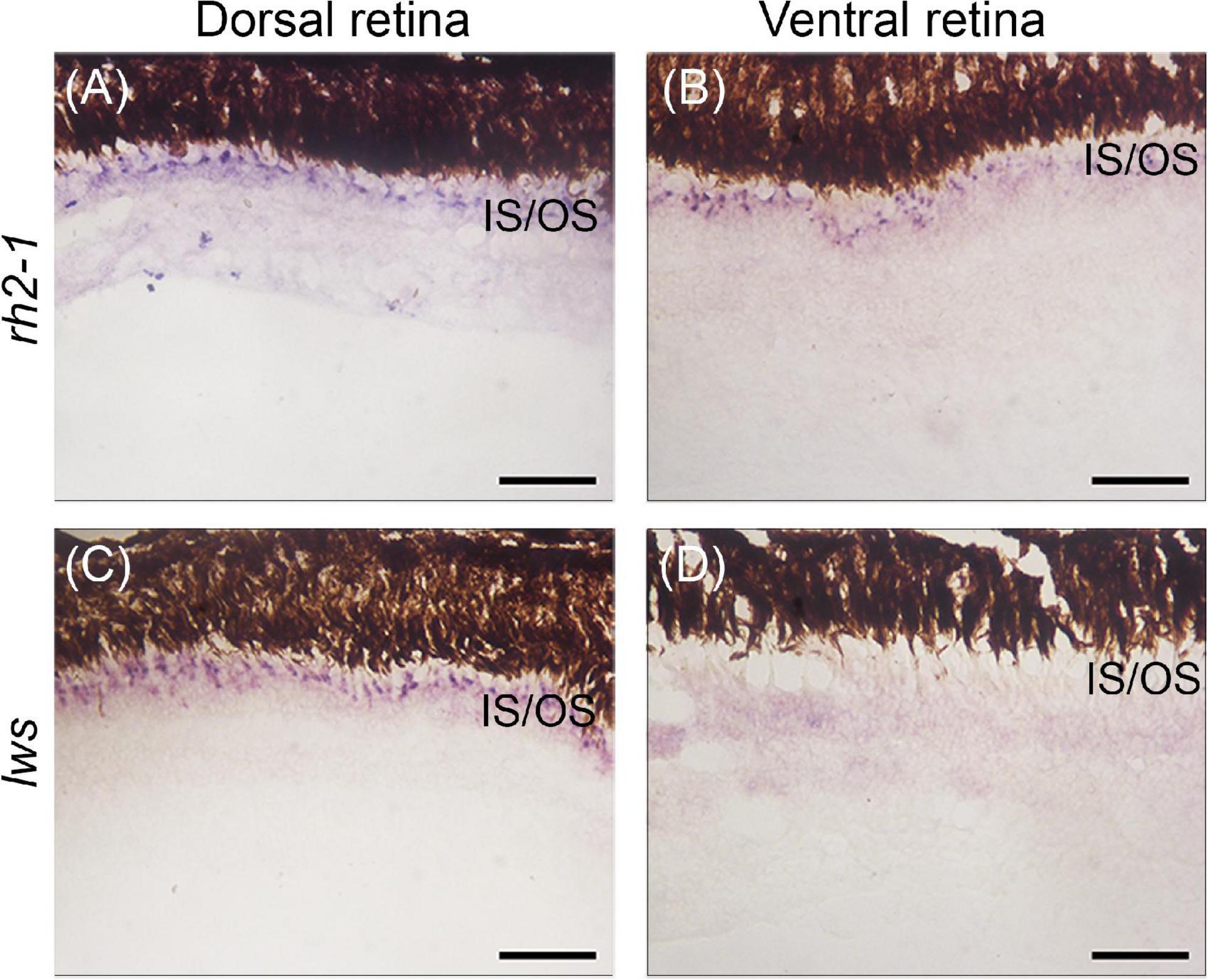
Figure 4. Gene expression of visual opsins in the retina of juvenile A. anableps under light stimuli for 30 days. In situ hybridizations of rh2-1 (A,B) and lws (C,D) in dorsal and ventral retinas from juveniles of A. anableps. Scale bars: 0.05 mm.
Altogether, our results show that lws and rh2-1 visual opsin expression is established without environmental light stimuli, prior to birth. However, changes in light input can modulate the expression of rh2-1 and cause a shift the expression domain, from being restricted to the ventral retina, to being expanded to the entire retina. Non-visual opsins also show asymmetric expression in the nuclear layer of the retina in juvenile fish. Although established only after birth, changes in the environmental lighting caused a shift in the expression domains of opn4×1, opn4m3, and tmt1b, suggesting that both visual and non-visual opsins can be modulated by changes in the photic input.
Discussion
In this work, we surveyed the expression of three melanopsins opn4×1, opn4×2, opn4m3, and one tmt1b in the nuclear layers of the A. anableps retina. The spatial pattern observed pre-birth diverges from that seen in free-swimming juvenile individuals, which express opn4×1 and opn4×2 in the ONL and INL of the ventral retina while opn4m3 and tmt1b are expressed in the INL, ONL and GCL of the ventral retina.
In zebrafish, melanopsin genes are expressed in different regions of the retina and have been associated with the regulation of negative phototaxis (Matos-Cruz et al., 2011; Fernandes et al., 2012). In teleost fish, the expression of melanopsin in various types of neuronal cells can directly influence retinal function in a dependent and/or independent way from intrinsically photosensitive retinal ganglion cells (Matos-Cruz et al., 2011). In other vertebrates, including teleost fish, tmt expression is distributed across many tissues including internal organs where light can hardly penetrate, suggesting that tmt opsin may have both photosensitive and non-photosensitive functions (Fischer et al., 2013; Sakai et al., 2015). In our work, we speculate that light-induced changes in non-visual opsins expression could be due to the indirect regulation of visual opsins in the photoreceptor cells of the retina.
Previous studies from our group and others have shown asymmetric expression of lws and rh2.1 in the photoreceptor cells in the dorsal and ventral domains of the developing retina, respectively, with the establishment of asymmetric expression of lws and rh2.1 occurring before birth, suggesting that it is genetically programmed and independent of light stimuli (Owens et al., 2012; Perez et al., 2017). This asymmetry allows the partial duplicated eye of the A. anableps to optimize the absorption of different wavelengths coming simultaneously from the aerial and aquatic environment. In this work, we manipulated light input by changing the turbid water where the A. anableps is normally found and replacing it with clear water. We tested the plasticity of lws and rh2.1 in the photoreceptor cells in the retina of juvenile fish after a 30 day period and our results showed that, despite being established before light stimuli reach the retina (Perez et al., 2017), the expression of rh2.1 (normally expressed in the ventral retina) shifted and became uniformly expressed in the entire retina, while the expression of lws remained unchanged and restricted to the photoreceptor cells in the dorsal retina. While in captivity, animals were maintained in tanks with average temperature of 28°C, which closely mirrors that of their natural environment. The commercial diet provided for the animals in captivity, however, differs from their diet in natural settings. In the wild, the stomach content of A. anableps ranges from micro and macroalgae, insects, crustaceans, molluscs, fishes, to mud and detritus (Figueiredo et al., 2019). While we cannot rule out a potential feeding behavior of food content impact on opsin gene expression, we have no knowledge of a documented link between the former and the latter.
In zebrafish and guppies, the expression patterns of different subtypes of rh2 and lws vary according to the developmental stage and region of the retina (Takechi and Kawamura, 2005; Owens et al., 2012). Several studies have demonstrated that opsin expression is subject to phenotypic plasticity (Fuller et al., 2005; Shand et al., 2008; Veen et al., 2017). Several teleost species placed under altered light conditions, such as damsel and cardinal fish (Luehrmann et al., 2018), African cichlids (Wright et al., 2019), guppies (Kranz et al., 2018), and Senegalese sole (Frau et al., 2020) can alter the expression of opsin genes to adapt to the environmental changes. Here we show that shift in opsin expression happens in the dorsal and ventral domains of the retina in A. anableps within a 30-day period, our results do not exclude the possibility that the change in the expression pattern of opsin genes occurs in a shorter period. Here, we show that after a 30-day period of exposure to clear water, opsin expression changes in the dorsal and ventral retinas, given that, this shift happens in portions of the retina for both visual and non-visual opsin genes, our results suggest that the regulatory control of the expression of opsins in the eye of the A. anableps is complex and compartmentalized in the dorsal and ventral retinas.
Conclusion
Our study shows that the expression of visual opsins in the retina of A. anableps can be modulated by spectral and intensity changes in ambient light. These adjustments allow for a quick adaptation of the visual system to different water turbidity and environmental luminosity. Our findings indicate that the A. anableps exhibits great potential to modulate its vision according to the environment, allowing this organism to adjust its vision to different photic conditions. Furthermore, our findings suggest that the expression of non-visual opsins can also be altered upon changes in environmental light, suggesting a regulatory correlation between visual and non-visual opsin regulation of physiological functions responsive to light.
Data availability statement
The raw data supporting the conclusions of this article will be made available by the authors, without undue reservation.
Ethics statement
This study was approved by IBAMA/SISBIO under license number 47206-1 and the Ethics Committee for Animal Research at the Universidade Federal do Pará (protocol number 037-2015).
Author contributions
DS, MA, JL, LP, and PS designed the research. LP, MA, JL, and BM performed in situ hybridizations (ISH) of A. anableps larvae. RR and JS designed the riboprobes for ISH. DS and LP performed light-induced experiments and analysis. DS, LP, and PS wrote the manuscript with input from BM, MA, JL, RR, and JS. All authors contributed to the article and approved the submitted version.
Acknowledgments
We thank Jeferson Carneiro and Karen Pinto for help in fish collection. We also thank Iracilda Sampaio for logistical support at the collection site. We would also like to thank Igor Schneider for critical reading of the manuscript.
Conflict of interest
The authors declare that the research was conducted in the absence of any commercial or financial relationships that could be construed as a potential conflict of interest.
Publisher’s note
All claims expressed in this article are solely those of the authors and do not necessarily represent those of their affiliated organizations, or those of the publisher, the editors and the reviewers. Any product that may be evaluated in this article, or claim that may be made by its manufacturer, is not guaranteed or endorsed by the publisher.
Supplementary material
The Supplementary Material for this article can be found online at: https://www.frontiersin.org/articles/10.3389/fnins.2022.995469/full#supplementary-material
Supplementary Figure 1 | In situ hybridization showing the sense control riboprobes for the non-visualopsins: opn4×1, opn4×2, opn4m3, tmt1b.
Supplementary Figure 2 | In situ hybridization showing the sense control riboprobes for thevisual opsins lws and rh2-1.
Supplementary Video 1 | A group of five Anableps anableps specimens in the wild. Movie was recorded in the estuarine region of Bragança, Pará, Brazil (0°53′27.6″S 46°39′21.9″W).
Supplementary Video 2 | A fish tank with Anableps anableps specimens during the 30-day light induced experiments.
References
Beaudry, F. E. G., Iwanicki, T. W., Mariluz, B. R. Z., Darnet, S., Brinkmann, H., Schneider, P., et al. (2017). The non-visual opsins: Eighteen in the ancestor of vertebrates, astonishing increase in ray-finned fish, and loss in amniotes. J. Exp. Zool. Part B Mol. Dev. Evol. 328, 685–696. doi: 10.1002/jez.b.22773
Biagioni, L. M., Hunt, D. M., and Collin, S. P. (2016). Morphological Characterization and Topographic Analysis of Multiple Photoreceptor Types in the Retinae of Mesopelagic Hatchetfishes with Tubular Eyes. Front. Ecol. Evol. 4:25. doi: 10.3389/fevo.2016.00025
Butler, J. M., Whitlow, S. M., Rogers, L. S., Putland, R. L., Mensinger, A. F., and Maruska, K. P. (2019). Reproductive state-dependent plasticity in the visual system of an African cichlid fish. Horm. Behav. 114:104539. doi: 10.1016/j.yhbeh.2019.06.003
Carleton, J. B., Lovell, P. V., Mchugh, A., Marzulla, T., Horback, K. L., and Mello, C. V. (2014). An optimized protocol for high-throughput in situ hybridization of zebra finch brain. Cold Spring Harb. Protoc. 2014, 1249–1258. doi: 10.1101/pdb.prot084582
Collin, S. P. (2009). “Evolution of the Visual System in Fishes,” in Encyclopedia of Neuroscience, eds M. D. Binder, N. Hirokawa, and U. Windhorst (Berlin: Springer), 1459–1466. doi: 10.1007/978-3-540-29678-2_3178
David, R., and Wedlich, D. (2001). Pcr-based Rna probes: A quick and sensitive method to improve whole mount embryo in situ hybridizations. Biotechniques 30:74. doi: 10.2144/01304st02
Davies, W. I., Zheng, L., Hughes, S., Tamai, T. K., Turton, M., Halford, S., et al. (2011). Functional diversity of melanopsins and their global expression in the teleost retina. Cell. Mol. Life Sci. 68, 4115–4132. doi: 10.1007/s00018-011-0785-4
De Lima, J. L., Soares, F. A., Remedios, A. C., Thom, G., Wirthlin, M., Aleixo, A., et al. (2015). A putative Ra-like region in the brain of the scale-backed antbird, Willisornis poecilinotus (Furnariides, Suboscines, Passeriformes, Thamnophilidae). Genet. Mol. Biol. 38, 249–254. doi: 10.1590/S1415-475738320150010
Diaz, N. M., Morera, L. P., and Guido, M. E. (2016). Melanopsin and the Non-visual Photochemistry in the Inner Retina of Vertebrates. Photochem. Photobiol. 92, 29–44. doi: 10.1111/php.12545
Fernandes, A. M., Fero, K., Arrenberg, A. B., Bergeron, S. A., Driever, W., and Burgess, H. A. (2012). Deep brain photoreceptors control light-seeking behavior in zebrafish larvae. Curr. Biol. 22, 2042–2047. doi: 10.1016/j.cub.2012.08.016
Figueiredo, M. B., Nunes, J. L., Almeida, Z. S., Paz, A. C., Piorski, N. M., and Reis, M. R. (2019). Feeding ecology of Anableps Anableps (Actinopterygii: Cyprinodontiformes: Anablepidae) off the north-eastern coast of brazil. Acta Ichthyol. Piscat. 49, 213–219. doi: 10.3750/AIEP/02477
Fischer, R. M., Fontinha, B. M., Kirchmaier, S., Steger, J., Bloch, S., Inoue, D., et al. (2013). Co-expression of Val- and Tmt-opsins uncovers ancient photosensory interneurons and motorneurons in the vertebrate brain. PLoS Biol. 11:e1001585. doi: 10.1371/journal.pbio.1001585
Frau, S., Loentgen, G., Martin-Robles, A. J., and Munoz-Cueto, J. A. (2020). Ontogenetic expression rhythms of visual opsins in senegalese sole are modulated by photoperiod and light spectrum. J. Comp. Physiol. B 190, 185–204. doi: 10.1007/s00360-020-01264-7
Fuller, R. C., and Claricoates, K. M. (2011). Rapid light-induced shifts in opsin expression: Finding new opsins, discerning mechanisms of change, and implications for visual sensitivity. Mol. Ecol. 20, 3321–3335. doi: 10.1111/j.1365-294X.2011.05180.x
Fuller, R. C., Carleton, K. L., Fadool, J. M., Spady, T. C., and Travis, J. (2005). Genetic and environmental variation in the visual properties of bluefin killifish, Lucania goodei. J. Evol. Biol. 18, 516–523. doi: 10.1111/j.1420-9101.2005.00886.x
Guido, M. E., Marchese, N. A., Rios, M. N., Morera, L. P., Diaz, N. M., Garbarino-Pico, E., et al. (2020). Non-visual Opsins and Novel Photo-Detectors in the Vertebrate Inner Retina Mediate Light Responses Within the Blue Spectrum Region. Cell. Mol. Neurobiol. 42, 59–83. doi: 10.1007/s10571-020-00997-x
Hauser, F. E., and Chang, B. S. W. (2017). Insights into visual pigment adaptation and diversity from model ecological and evolutionary systems. Curr. Opin. Genet. Dev. 47, 110–120. doi: 10.1016/j.gde.2017.09.005
Hofmann, C. M., and Carleton, K. L. (2009). Gene duplication and differential gene expression play an important role in the diversification of visual pigments in fish. Integr. Comp. Biol. 49, 630–643. doi: 10.1093/icb/icp079
Jeffery, W. R. (2020). Astyanax surface and cave fish morphs. Evodevo 11:14. doi: 10.1186/s13227-020-00159-6
Kojima, D., and Fukada, Y. (1999). Non-visual photoreception by a variety of vertebrate opsins. Novartis Found Symp. 224, 265–279. doi: 10.1002/9780470515693.ch15
Kranz, A. M., Forgan, L. G., Cole, G. L., and Endler, J. A. (2018). Light environment change induces differential expression of guppy opsins in a multi-generational evolution experiment. Evolution 72, 1656–1676. doi: 10.1111/evo.13519
Lovell, P. V., Carleton, J. B., and Mello, C. V. (2013). Genomics analysis of potassium channel genes in songbirds reveals molecular specializations of brain circuits for the maintenance and production of learned vocalizations. BMC Genom. 14:470. doi: 10.1186/1471-2164-14-470
Luehrmann, M., Stieb, S. M., Carleton, K. L., Pietzker, A., Cheney, K. L., and Marshall, N. J. (2018). Short-term colour vision plasticity on the reef: Changes in opsin expression under varying light conditions differ between ecologically distinct fish species. J. Exp. Biol. 221:jeb175281. doi: 10.1242/jeb.175281
Lunghi, E., Zhao, Y., Sun, X., and Zhao, Y. (2019). Morphometrics of eight Chinese cavefish species. Sci. Data 6:233. doi: 10.1038/s41597-019-0257-5
Maruska, K. P., and Butler, J. M. (2021). Reproductive- and Social-State Plasticity of Multiple Sensory Systems in a Cichlid Fish. Integr. Comp. Biol. 61, 249–268. doi: 10.1093/icb/icab062
Matos-Cruz, V., Blasic, J., Nickle, B., Robinson, P. R., Hattar, S., and Halpern, M. E. (2011). Unexpected diversity and photoperiod dependence of the zebrafish melanopsin system. PLoS One 6:e25111. doi: 10.1371/journal.pone.0025111
Moutsaki, P., Whitmore, D., Bellingham, J., Sakamoto, K., David-Gray, Z. K., and Foster, R. G. (2003). Teleost multiple tissue (tmt) opsin: A candidate photopigment regulating the peripheral clocks of zebrafish? Brain Res. Mol. Brain Res. 112, 135–145. doi: 10.1016/S0169-328X(03)00059-7
Okano, T., Yoshizawa, T., and Fukada, Y. (1994). Pinopsin is a chicken pineal photoreceptive molecule. Nature 372, 94–97. doi: 10.1038/372094a0
Owens, G. L., Rennison, D. J., Allison, W. T., and Taylor, J. S. (2012). In the four-eyed fish (Anableps anableps), the regions of the retina exposed to aquatic and aerial light do not express the same set of opsin genes. Biol. Lett. 8, 86–89. doi: 10.1098/rsbl.2011.0582
Peirson, S. N., Halford, S., and Foster, R. G. (2009). The evolution of irradiance detection: Melanopsin and the non-visual opsins. Philos. Trans. R. Soc. Lond. B Biol. Sci. 364, 2849–2865. doi: 10.1098/rstb.2009.0050
Perez, L. N., Lorena, J., Costa, C. M., Araujo, M. S., Frota-Lima, G. N., Matos-Rodrigues, G. E., et al. (2017). Eye development in the four-eyed fish Anableps anableps: Cranial and retinal adaptations to simultaneous aerial and aquatic vision. Proc. Biol. Sci. 284:20170157. doi: 10.1098/rspb.2017.0157
Sakai, K., Yamashita, T., Imamoto, Y., and Shichida, Y. (2015). Diversity of Active States in Tmt Opsins. PLoS One 10:e0141238. doi: 10.1371/journal.pone.0141238
Sandbakken, M., Ebbesson, L., Stefansson, S., and Helvik, J. V. (2012). Isolation and characterization of melanopsin photoreceptors of Atlantic salmon (Salmo salar). J. Comp. Neurol. 520, 3727–3744. doi: 10.1002/cne.23125
Sato, K., Yamashita, T., Kojima, K., Sakai, K., Matsutani, Y., Yanagawa, M., et al. (2018). Pinopsin evolved as the ancestral dim-light visual opsin in vertebrates. Commun. Biol. 1:156. doi: 10.1038/s42003-018-0164-x
Schwab, I. R., Ho, V., Roth, A., Blankenship, T. N., and Fitzgerald, P. G. (2001). Evolutionary attempts at 4 eyes in vertebrates. Trans. Am. Ophthalmol. Soc. 99, 145–156.
Shand, J., Davies, W. L., Thomas, N., Balmer, L., Cowing, J. A., Pointer, M., et al. (2008). The influence of ontogeny and light environment on the expression of visual pigment opsins in the retina of the black bream, Acanthopagrus butcheri. J. Exp. Biol. 211, 1495–1503. doi: 10.1242/jeb.012047
Takechi, M., and Kawamura, S. (2005). Temporal and spatial changes in the expression pattern of multiple red and green subtype opsin genes during zebrafish development. J. Exp. Biol. 208, 1337–1345. doi: 10.1242/jeb.01532
Thomas, K. N., Gower, D. J., Bell, R. C., Fujita, M. K., Schott, R. K., and Streicher, J. W. (2020). Eye size and investment in frogs and toads correlate with adult habitat, activity pattern and breeding ecology. Proc. Biol. Sci. 287:20201393. doi: 10.1098/rspb.2020.1393
Van Gelder, R. N. (2001). Non-visual ocular photoreception. Ophthalmic Genet. 22, 195–205. doi: 10.1076/opge.22.4.195.2215
Van Gelder, R. N., and Buhr, E. D. (2016). Melanopsin: The Tale of the Tail. Neuron 90, 909–911. doi: 10.1016/j.neuron.2016.05.033
Veen, T., Brock, C., Rennison, D., and Bolnick, D. (2017). Plasticity contributes to a fine-scale depth gradient in sticklebacks’ visual system. Mol. Ecol. 26, 4339–4350. doi: 10.1111/mec.14193
Wald, G. (1968). The molecular basis of visual excitation. Nature 219, 800–807. doi: 10.1038/219800a0
Warrant, E. J., and Locket, N. A. (2004). Vision in the deep sea. Biol. Rev. Camb. Philos. Soc. 79, 671–712. doi: 10.1017/S1464793103006420
Wright, D. S., Meijer, R., Van Eijk, R., Vos, W., Seehausen, O., and Maan, M. E. (2019). Geographic variation in opsin expression does not align with opsin genotype in Lake Victoria cichlid populations. Ecol. Evol. 9, 8676–8689. doi: 10.1002/ece3.5411
Keywords: evolution, opsin, plasticity, retina, Anableps
Citation: Salgado D, Mariluz BR, Araujo M, Lorena J, Perez LN, Ribeiro RdL, Sousa JdF and Schneider PN (2022) Light-induced shifts in opsin gene expression in the four-eyed fish Anableps anableps. Front. Neurosci. 16:995469. doi: 10.3389/fnins.2022.995469
Received: 15 July 2022; Accepted: 08 September 2022;
Published: 29 September 2022.
Edited by:
Daniel Ortuño-Sahagún, University of Guadalajara, MexicoReviewed by:
Kanta Mizusawa, Kitasato University, JapanRyota Matsuo, Fukuoka Women’s University, Japan
Copyright © 2022 Salgado, Mariluz, Araujo, Lorena, Perez, Ribeiro, Sousa and Schneider. This is an open-access article distributed under the terms of the Creative Commons Attribution License (CC BY). The use, distribution or reproduction in other forums is permitted, provided the original author(s) and the copyright owner(s) are credited and that the original publication in this journal is cited, in accordance with accepted academic practice. No use, distribution or reproduction is permitted which does not comply with these terms.
*Correspondence: Patricia N. Schneider, cHNjaG5laWRlckBsc3UuZWR1