- 1Department of Radiology, Heping Hospital Affiliated to Changzhi Medical College, Changzhi, China
- 2Shanxi Key Laboratory of Artificial Intelligence Assisted Diagnosis and Treatment for Mental Disorder, First Hospital of Shanxi Medical University, Taiyuan, China
- 3Department of Psychiatry, First Hospital/First Clinical Medical College of Shanxi Medical University, Taiyuan, China
- 4Department of Medical Imaging, Changzhi Medical College, Changzhi, China
- 5Department of Mental Health, Shanxi Medical University, Taiyuan, China
Numerous neuroimaging studies have demonstrated that diverse brain structural plasticity could occur in a human brain during a depressive episode. However, there is a lack of knowledge regarding the underlying mechanisms of mild-to-moderate depression (MMD), especially the changes of brain structural characteristics after treatment with the Shuganjieyu capsule (SG), a kind of traditional Chinese medicine that has been recommended for the specialized treatment of MMD. In this study, we investigated the structural brain plasticity in MMD that have been undergoing 8 weeks of SG treatment compared with age- and sex-matched healthy controls (HCs) and assessed the relationship between these brain structural alternations and clinical symptoms in MMD. At the baseline, we found that: (1) fractional anisotropy (FA) values in patients with MMD were found to be significantly increased in the regions of anterior limb of internal capsule (ALIC) [MNI coordinates: Peak (x/y/z) = 102, 126, 77; MMD FApeak (Mean ± SD) = 0.621 ± 0.043; HCs FApeak (Mean ± SD) = 0.524 ± 0.052; MMD > HCs, t = 9.625, p < 0.001] and posterior limb of internal capsule (PLIC) [MNI coordinates: Peak (x/y/z) = 109, 117, 87; MMD FApeak (Mean ± SD) = 0.694 ± 0.042; HCs FApeak (Mean ± SD) = 0.581 ± 0.041; MMD > HCs, t = 12.90, p < 0.001], and FA values were significantly positively correlated with HAMD scores in patients with MMD. (2) Patients with MMD showed smaller gray matter volume (GMV) of the dorsolateral prefrontal cortex (DLPFC), frontal cortex, occipital cortex, and precuneus, and the GMV of DLPFC was negatively correlated with HAMD scores. After SG treatment, we found that (1) the HAMD scores decreased; (2) FA values were significantly decreased in the regions of the ALIC and PLIC compared to those at baseline and TBSS revealed no significant differences in FA values between patients with MMD and HCs. (3) The structural characteristics of DLPFC in patients with MMD obtained at the 8th week were improved, e.g., no significant differences in GMV of DLPFC between the two groups. Taken together, our results provided neuroimaging evidence suggesting that SG is an effective treatment for patients with MMD. Moreover, alterations of GMV after 8 weeks of SG treatment indicated a potential modulation mechanism in brain structural plasticity within the DLPFC in patients with MMD.
Introduction
Depression is a notably prevalent mental disorder, characterized by depressed mood, loss of interest, and increased fatigue, affecting an estimated 264 million people worldwide (Disease et al., 2018). Globally, the prevalence of depression is ~4.4%, and it has become the main factor contributing to the global disease burden. There are more than 95 million depressive patients in China, with a lifetime prevalence of 6.9% and a 12-month prevalence of 3.6% (Huang et al., 2019). Depending on the severity of the symptoms, depression can be categorized as mild, moderate, or severe. Mild-to-moderate depression (MMD) involves fewer than five symptoms of depression and is more common than severe depression in general medical settings (Liu et al., 2020; Meng et al., 2021).
With the development of neuroimaging technology, a growing number of researchers have begun to use magnetic resonance imaging (MRI) to study the structural and functional features of the brain in patients with depression. Structural imaging techniques are useful tools for detecting neurobiological alterations associated with depression. Surface-based morphometry based on anatomical T1-weighted MRI provides better alignment of cortical landmarks over the whole brain using vertex-by-vertex analysis to explore possible changes in the gray matter (GM) (Mills and Tamnes, 2014). Tract-based spatial statistics (TBSS) based on diffusion tensor imaging (DTI) implements voxel-wise statistical analysis of fractional anisotropy (FA) data to examine changes in the microstructure of white matter (WM) bundle fibers (Zhang H. et al., 2012). Structural neuroimaging studies have shown that various structural abnormalities can occur in the human brain during depressive episodes. Researchers have shown that depression is associated with general volume reductions in several brain regions, such as the frontal cortex, mainly the prefrontal cortex (PFC), orbitofrontal cortex (OFC), amygdala, thalamus, insula, and hippocampus (Kronenberg et al., 2009; Ancelin et al., 2019). Koolschijn et al. found that major depressive disorder (MDD) is characterized by a reduction in volume in several frontal regions, including the OFC and anterior cingulate cortex (ACC), which is attributed to dysfunctions in stress regulation and emotional processing (Koolschijn et al., 2009). In addition, the number of MDD was positively correlated with the degree of hippocampal volume reduction, suggesting that repeated depressive episodes may lead to a further reduction in hippocampal volume (Eker and Gonul, 2010). In another study, Bremner et al. found that compared with healthy controls (HCs), the left hippocampal volumes of patients with MDD were smaller (Bremner et al., 2002). Furthermore, other researchers found that the volumes of other brain regions, such as the frontal lobe, caudate, amygdala, and temporal lobe, were not significantly different between patients with MDD and HCs (Koolschijn et al., 2009).
For the visualization of brain networks, WM tract imaging technology has been widely used and FA is the most common variable used in DTI studies (Catani, 2006). Furthermore, FA abnormalities in the fiber tracts of individuals with MDD may indicate microstructural changes underlying the pathophysiology of the disorder. For example, Liao et al. performed a meta-analysis to identify areas of MDD WM change. They found that FA values were reduced in the WM tracts connecting the PFC to different brain cortices (temporal, occipital, and frontal lobes) and subcortical regions (amygdala and hippocampus), demonstrating the presence of structural network alterations in MDD (Liao et al., 2013). Furthermore, these researchers have used DTI technology to reveal that patients characterized with impaired WM integrity of fiber tracts that contribute to emotional regulation, including the superior longitudinal fasciculus, uncinate fasciculus, corpus callosum, anterior corona radiata, cingulum, and inferior fronto-occipital fasciculus (Kieseppa et al., 2010; Cole et al., 2012; Jiang et al., 2017; Vilgis et al., 2017).
Antidepressant medication is a first-line treatment for severe MDD (Cleare et al., 2015), and has been shown to ameliorate functional impairment, with changes in neural activation and brain structure (Dichter et al., 2015; Klauser et al., 2015). In recent years, imaging studies on the effects of different mechanism drugs on brain structure in patients with MDD have increased rapidly (Zeng et al., 2012; Groman et al., 2013; Yuen et al., 2014; Dusi et al., 2015; Fu et al., 2015; Liu et al., 2017). These studies have proved that whether the drug mechanism is the same or not, antidepressants can improve depression by changing brain structure, and the changes in the volume of different brain regions are closely related to the neuroplasticity of antidepressants. On the other hand, the brain areas improved by antidepressants with different mechanisms may also be different, which may be closely related to the different distribution sites of the brain-related neurotransmitters, and also have a great relationship with the occupancy of their neurotransmitter serum transporters.
Although previous studies have investigated structural abnormalities in patients with MDD, the underlying mechanisms of MMD, especially the changes in brain structural characteristics after drug treatment, remain unclear. Furthermore, MMD is the initial stage of a depressive disorder and is more common than severe depression in the general medical environment. Exploring the structural features of MMD provides a basis for the mechanism of MDD pathogenesis and may lead to optimized treatment for patients in clinical practice.
Shugan Jieyu Capsule (SG) is a Chinese herbal medicine mainly composed of Eleutherococcus senticosus (also known as Acanthopanax senticosus) and Hypericum perforatum (also known as St. John's wort) (~5:6). Both E. senticosus and H. perforatum have been proven to be effective in the treatment of depression and impaired cognition (Sithisarn et al., 2013; Ben-Eliezer and Yechiam, 2016). Furthermore, SG has been licensed and widely prescribed for the treatment of depression in China since 2008. The effect of SG in ameliorating depressive symptoms is similar to that of escitalopram, but owing to its fewer adverse reactions in relieving the symptoms of depression, it has been recommended for the specialized treatment of MMD (Zhang et al., 2014).
Previous studies have revealed that GM and WM in certain brain regions of patients with MDD are abnormal, and these brain regions are usually involved in individual emotion processing. The initial stage of depressive disorder is MMD, and it mainly presents in the form of emotional problems, which is a common clinical symptom. Therefore, we hypothesized that modified volumes and integrity of the GM and WM in certain brain regions could occur in patients with MMD, and these changes may be associated with clinical variables. Furthermore, depressive symptoms could be reduced after SG treatment, and the efficacy of SG in MMD might be reflected in the associated changes in the structural differences in brain regions. To test our hypothesis, we combined psychometrics and structural MRI techniques to investigate brain structural alterations in 47 patients with MMD following SG treatment compared with 43 age- and sex-matched HCs.
Methods and materials
Participants
Forty-seven right-handed patients with MMD (17 men; mean age 26.12 ± 5.06 years) and 43 age- and sex-matched HCs (13 men; mean age 26.27 ± 5.69 years) participated in the study. All patients satisfied the following inclusion criteria: (1) Diagnostic and Statistical Manual of Mental Disorders-Fourth Edition (DSM-IV) criteria for MDD diagnosis with an actual mild-to-moderate episode; (2) score 7 < HAMD-24 scores < 24; (3) 18 years ≤ age ≤ 50 years; (4) right-handed (Edinburgh Handedness Inventory, EHI) (Oldfield, 1971); (5) no antidepressant treatment in the past 2 months; and (6) no comorbid Axis-I diagnosis, which comprises all psychological diagnostic categories except intellectual disability and personality disorder. It is worth noting that older patients have an increased risk of vascular disease, which may affect brain structure. To mitigate the influence of vascular factors on brain structure (Kendler et al., 2009), the participants in this study were adults under the age of 50. In fact, considering the vascular factors, we actively encouraged younger patients to participate in this study. Exclusion criteria included a history of neurological diseases, past or current history of alcoholism, elevated risk of suicide, and contraindications of MRI scans (e.g., implanted metal objects and claustrophobia). Forty-three age- and sex-matched, right-handed HCs were recruited from the community nearby and were accessed via the Structured Clinical Interview for DSM-IV, non-patient edition (SCID-I/NP) to exclude the diagnosis of MMD, as well as other axis-I psychiatric disorders. In addition to the above, the other criteria also included: (1) HAMD-24 scores <7 and (2) no family history of mental illness. (3) There were no psychological or psychopathological symptoms. The exclusion criteria for the HCs were identical to those for MMD. The participants signed an informed consent form to participate in this study. This study was approved by the Ethics Committee of Shanxi Medical University.
Clinical assessments
For patients with MMD, clinical assessments included age at illness onset and the severity of illness. Depressive symptoms and anxiety symptoms were independently assessed based on HAMD-24 and HAMA-14 by two research psychiatrists. The clinical assessment of HCs included the HAMD and HAMA. The HAMD was established in 1960 and adopts a 5-level rating scale from 0 to 4 points. The total score ranges from 0 to 78, and the depression level can be divided as follows: <8, no depression; 8–24, MMD; and > 24, severe depression. The HAMA was established in 1959 and adopts a 5-level rating scale from 0 to 4 points. The total score ranges from 0 to 56, and the anxiety levels can be divided as follows: <7, no anxiety; 7–14, possible anxiety; 15–21, anxiety; 21–29, obvious anxiety; and >29, severe anxiety. Both scales were confirmed to have good reliability.
Medication treatment
After the pre-tests, patients included were instructed to take a Chinese herbal medicine named SG, which is mainly made up of Acanthopanax and H. perforatum, licensed and widely prescribed for depression in China since 2008. Patients with MMD received SG treatment for 8 weeks after the baseline MRI scans. According to the recommended medication dose, patients with MMD took 1.44 g of SG per day, including 0.72 g in the morning and 0.72 g in the evening for 8 weeks. During treatment, all participants were followed up weekly via telephone interviews to maintain good adherence. After treatment, all the participants completed the HAMD and HAMA assessments.
MRI acquisition
MR images were acquired using a 3.0-T Siemens Skyra MRI scanner (Siemens Medical, Erlangen, Germany) at the Shanxi Provincial People's Hospital. A magnetization-prepared rapid gradient echo sequence was used to acquire high-resolution T1-weighted anatomical images (repetition time = 2,530 ms, echo time = 2.01 ms, inversion time = 900 ms, flip angle = 9°, resolution matrix = 256 × 256, slices = 192, thickness = 1 mm, voxel size = 1.0 × 1.0 × 1.0 mm3, and duration = 7 min). The diffusion data for each subject were obtained using a diffusion-weighted, single shot, spin-echo, echo planar imaging (EPI) sequence (TR/TE = 10,200/91 ms, matrix = 96 × 96, FOV = 192 × 192 mm, voxel size = 2.0 × 2.0 × 2.0 mm3, 70 axial slices, 2.0 mm slice thickness, b-value 1 = 0 s/mm2, and b-value 2 = 1,500 s/mm2) in 64 directions. Resting-state fMRI data were acquired using an EPI sequence (TR/TE, 2620/30 ms; flip angle: 90°, FOV 192 ×192 mm; matrix, 64 ×64; slice thickness, 3 mm; slices, 47; 220 volumes).
Voxel-based morphology analysis
The GM volume was examined using VBM from the FSLVBM. All T1-weighted images were first extracted from the brain and then segmented into GM, WM, or cerebrospinal fluid. A GM template was generated by registering and averaging all GM images. The GM image for each participant was then registered to the template using a non-linear transformation. A voxel-wise permutation test was used to identify significant group differences between patients with MMD and HCs to a distribution generated from 5,000 permutations of the data for each voxel of the template, using a sigma filter of 3 mm for smoothing. P < 0.05 (FWE, using the TFCE method) was considered statistically significant.
Tract-based spatial statistics
We analyzed WM properties using voxel-wise TBSS from the FMRIB Software Library (FSL) (https://fsl.fmrib.ox.ac.uk/fsl/fslwiki/TBSS) (Smith et al., 2006). This method identified a core WM “skeleton” that was anatomically equivalent across participants. First, diffusion data were corrected using Eddy correction (eddy_correct) and brain-extracted using BET in FSL (Smith et al., 2002). The FA images were created by fitting a tensor model using DTI fit, and then FA data from all participants were aligned into a common standard space using the non-linear registration tool, FNIRT. Next, the mean FA image was thinned to create a mean FA skeleton representing the centers of all tracts common to the group. The FA images from individual subjects were then projected onto this skeleton and the resulting data were fed into voxel-wise cross-subject statistics. The significant regions were thickened for visualization using the TBSS fill script in the FSL. The Johns Hopkins University ICBM-DTI-81 WM labels atlas was used to locate anatomical structures in the MNI152 spaces. Independent-sample t-tests were conducted to examine FA differences between the two groups using the non-parametric permutation tool Randomize in FSL.
Statistical analysis
For the demographic data, group differences in age and education level were evaluated using independent sample t-tests, and sex differences were assessed using Pearson's chi-square test. The difference between HAMA and HAMD scores of patients before and after treatment was tested by paired sample t-test. Pearson's correlation analyses were performed to assess the relationship between structural deficits and depressive symptoms in the MDD group.
Furthermore, to assess the joint influence of structural deficits on depression, a multiple linear regression analysis based on a forward stepwise selection procedure was applied. In this analysis, depression symptom severity (overall HAMD scores) was used as the dependent variable, and structural features (GM volume and FA values) were used as explanatory variables. The forward stepwise selection procedure started by including the explanatory variable that could mostly and significantly explain the dependent variable (p < 0.05) and adjusted by repeatedly adding other explanatory variables (if any) that could significantly improve the fit of the model (p < 0.05), until none could improve the model. Before multiple linear regression analysis, all variables were checked for deviation from normality using the Kolmogorov-Smirnov test, and no significant deviation from normality was observed for any variable (p > 0.05).
Results
Demographic and clinical characteristics
Demographic (i.e., age, sex, and years of education) and clinical variables in patients with MMD and HCs are summarized in Table 1. Although sex, age, and years of education were not significantly different between the two groups, the clinical variables, as quantified by 24-item Hamilton Depression Rating Scale (HAMD) (t = 16.060, p < 0.001; Table 1 and Figure 1A) and 14-item Hamilton Anxiety Rating Scale (HAMA) (t = 12.220, p < 0.001; Table 1 and Figure 1A), were significantly larger in patients with MMD than in HCs. Patients with MMD received SG treatment for 8 weeks, and the symptoms were significantly reduced from baseline to the end of SG treatment. Specifically, 37 patients had HAMD scores <7, and 10 patients had HAMD scores between 7 and 14. At the same time, 32 patients had HAMA scores <7, and 15 patients had HAMA scores between 7 and 14. In addition, the mean HAMD and HAMA scores were <7 in patients with MMD after 8 weeks of SG treatment (Table 2 and Figure 1B). Therefore, it can be considered that after 8 weeks of SG-treatment, the condition of all patients has been effectively alleviated.
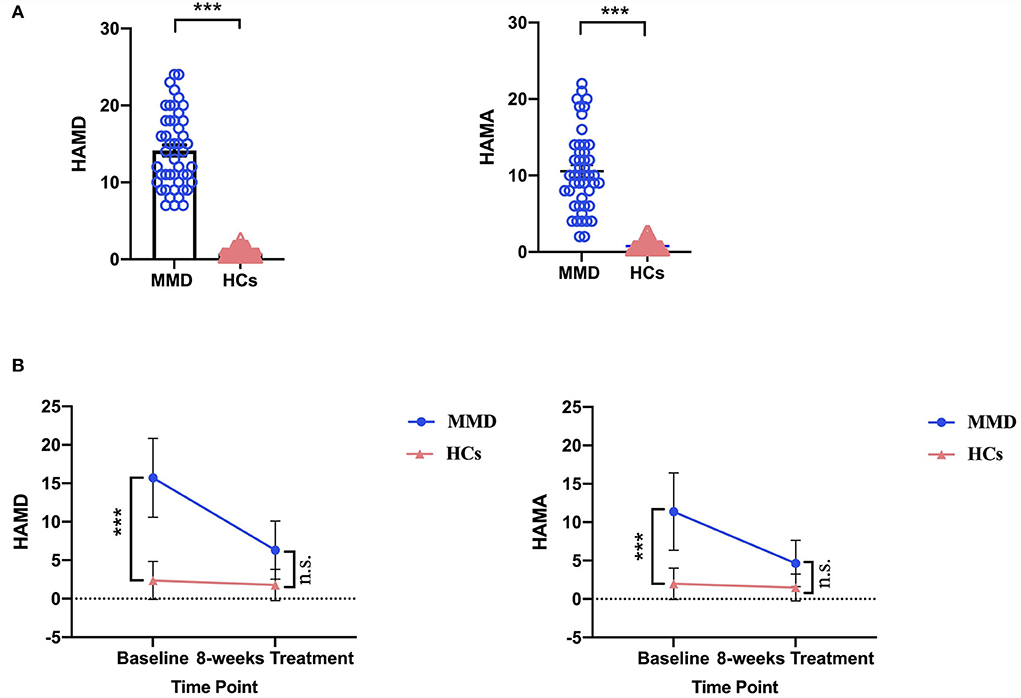
Figure 1. Comparison of HAMD and HAMA scores between MMD patients and HCs. (A) At the baseline, MMD patients exhibited significantly larger HAMD and HAMA scores than in HCs. The error bars indicate standard error. (B) After 8 weeks SG-treatment, the HAMD and HAMA scores of MMD patients decreased significantly, and there were no significant differences in HAMD and HAMA scores of MMD patients compared HCs. HAMD, hamilton depression; HAMA, hamilton anxiety; MMD, mild-to-moderate depression; HCs, healthy controls. ***, p < 0.001; n.s., no significance.

Table 2. Comparison of depression variables between patients with MMD after 8 weeks SG-treatment and HCs after 8 weeks (mean ± SD).
Tract-based spatial statistics
At the baseline, FA values in patients with MMD were significantly increased in the regions of the anterior limb of the internal capsule (ALIC) and posterior limb of the internal capsule (PLIC) compared to those in HCs (Figure 2).
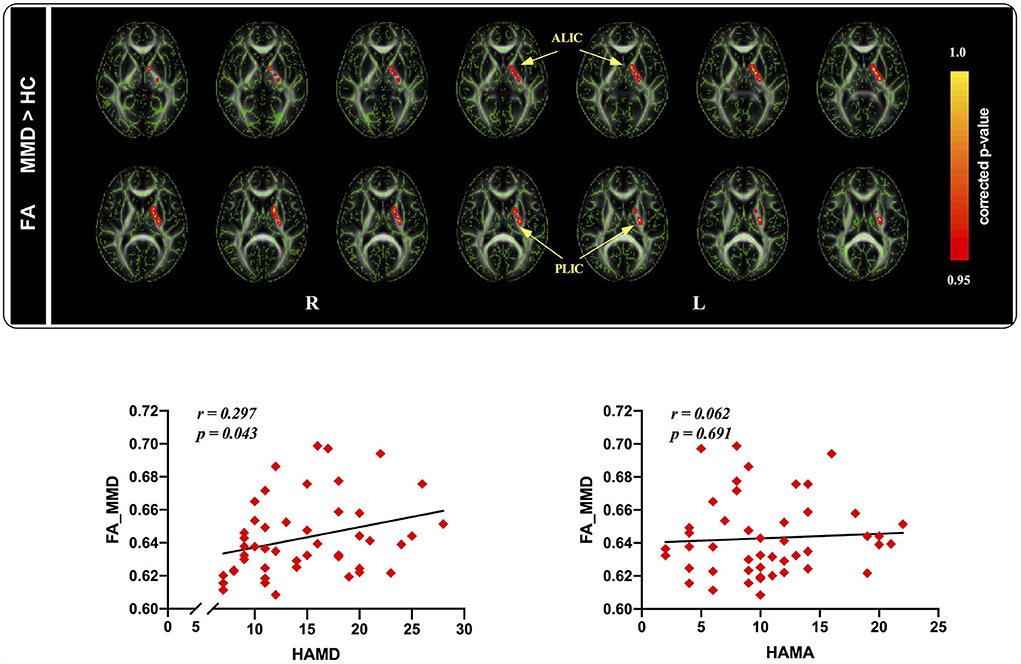
Figure 2. Tract-based spatial statistics maps are shown the FA differences between the two groups and the correlation between FA and HAMD as well as HAMA ratings in MMD patients. (Top): Red represents regions with significantly increased FA in MMD patients, compared with HCs. (Bottom): FA values were significantly correlated with HAMD ratings, whereas not related to HAMA ratings in MDD patients. FA, fractional anisotropy; HAMD, hamilton depression; HAMA, hamilton anxiety; MMD, mild-to-moderate depression; HCs, healthy controls.
After 8 weeks of SG treatment, FA values were significantly decreased in the regions of the ALIC (MNI coordinates: Peak(x/y/z) = 102, 126, 77; t = 3.720, p < 0.001; Table 3) and PLIC (MNI coordinates: Peak(x/y/z) = 109, 117, 87; t = 18.880, p < 0.001; Table 3) compared to those at baseline. TBSS revealed no significant differences in FA values between patients with MMD and HCs.
To further understand the association between FA values and clinical variables in patients with MMD, we conducted correlation analyses between FA values and depression and anxiety scores in the patient group. The results indicated that FA values were significantly positively correlated with HAMD scores (r = 0.297, p = 0.043; Figure 2), but not with HAMA scores (r = 0.062, p = 0.691; Figure 2) in patients with MMD.
Gray matter volume
At baseline, after controlling for the effects of age, sex, and total brain size, we observed that the GM volume of the dorsolateral prefrontal cortex (DLPFC), frontal cortex, occipital cortex, and precuneus was significantly smaller in patients with MMD than in HCs [p < 0.05, threshold-free cluster enhancement (TFCE) corrected; Figure 3].
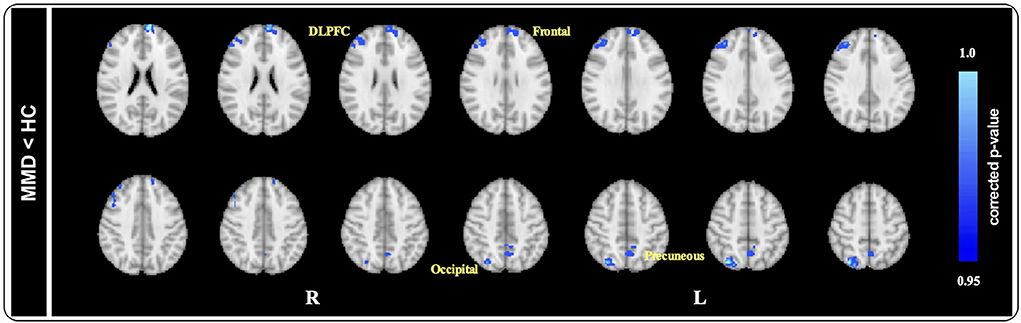
Figure 3. Voxel-based morphology comparison between MMD and HCs. After controlling the effects of age and gender by adding them as covariates, ACOVA showed the gray matter volume of the DLPFC, frontal cortex, occipital cortex, and precuneus were significantly smaller in MMD patients than in HCs (p < 0.05, TFCE corrected). DLPFC, dorsolateral prefrontal cortex; HAMD, hamilton depression; MMD, mild-to-moderate depression; HCs, healthy controls.
After 8 weeks of SG treatment, GM volume was significantly increased in the regions of the Frontal gyrus (MNI coordinates: Peak(x/y/z) = 50, 94, 43; t = 4.402, p < 0.001; Table 4), DLPFC (MNI coordinates: Peak(x/y/z) = 28, 84, 50; t = 7.416, p < 0.001; Table 4), precuneus (MNI coordinates: Peak(x/y/z) = 46, 33, 57; t = 5.766, p < 0.001; Table 4), and Frontal gyrus (MNI coordinates: Peak(x/y/z) = 29,83,26; t = 12.650, p < 0.001; Table 4) compared to those at baseline. There were no significant differences in GM volume between patients with MMD and HCs.
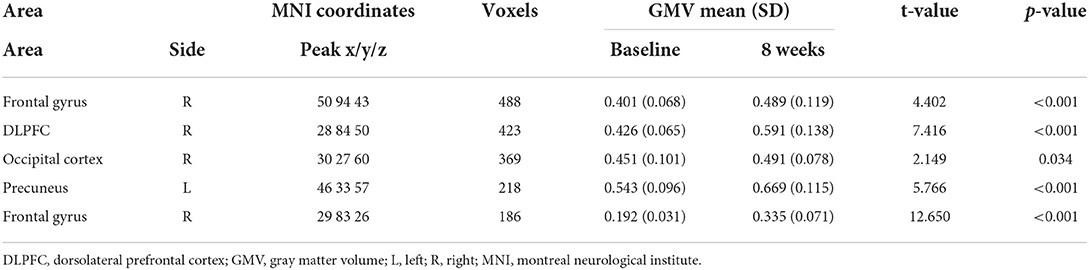
Table 4. Main regions showing gray matter volumes alterations in patients with MMD (clusters >50 voxels).
To understand the effects of depression on structural GM volume, correlation analyses were conducted to determine the relationship between GM volume abnormalities and depression scores. We extracted GM volume in the DLPFC and precuneus, correlation analyses indicating that the GM volume of DLPFC was negatively correlated with HAMD scores (r = 0.300, p = 0.041, Figure 4), whereas the GM volume of precuneus was not correlated with HAMD scores (r = 0.074, p = 0.621; Figure 4) in patients with MMD.
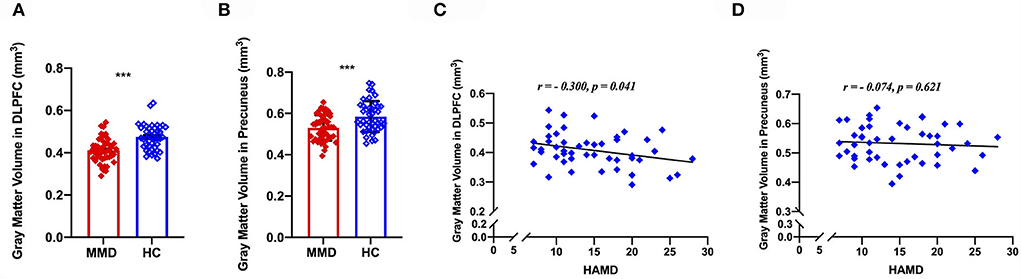
Figure 4. Voxel-based morphology comparison between MMD patients and HCs, and correlations between the reduction volumes and depression symptoms. The gray matter volume of DLPFC was significantly smaller in MMD patients than in HCs (A), meanwhile, the gray matter volume of precuneus was significantly smaller in MMD patients than in HCs (B). Scatter plots indicating that the gray matter volume of DLPFC was negatively correlated with HAMD scores in MMD patients (C), whereas the gray matter volume of precuneus was not correlated with HAMD scores in MMD patients (D). DLPFC, dorsolateral prefrontal cortex; MMD, mild-to-moderate depression; HCs, healthy control. ***, p < 0.001.
Comparison of mild depression and moderate depression
It is worth noting that researchers have previously found that MDD patients with different severity have different neuroimaging changes in the default mode network (DMN) and the execution control network (ECN) (He et al., 2022). Thus, we divided patients with MMD into mild (N = 24) and moderate (N = 23) depression groups, and compared the GM volume and FA values of the two groups at baseline and 8 weeks of SG-treatment, respectively. At the whole brain level, there was no significant difference in structural characteristics between the two groups. In addition, we compared whether there were differences between mild and moderate depression groups in brain regions (FA: ALIC and PLIC; GMV: DLPFC, frontal cortex, occipital cortex, and precuneus) with structural differences between MMD and HCs. We found that there was no significant difference in those brain regions characteristics between the two groups at baseline (FA: t = 1.601, p > 0.05; DLPFC: t = 1.582, p > 0.05; frontal cortex: t = 1.032, p > 0.05; occipital cortex: t = 1.374, p > 0.05; precuneus: t = 0.403, p > 0.05) and 8 weeks of SG-treatment (FA: t = 0.442, p > 0.05; DLPFC: t = 0.997, p > 0.05; frontal cortex: t = 0.785, p > 0.05; occipital cortex: t = 0.893, p > 0.05; precuneus: t = 0.305, p > 0.05).
Correlation between clinical symptoms and FA value alterations, as well as GM volume alterations
Alterations of GM volume in the DLPFC was found to be positively correlated with HAMD score changes in patients with MMD after 8 weeks of SG treatment (Figure 5; r = 0.335, p = 0.048). And GMV in other three brain regions was not related to the HAMD scores changes: frontal cortex (r = 0.127, p = 0.942); occipital cortex (r = 0.250, p = 0.147); precuneus (r = 0.275, p = 0.109).
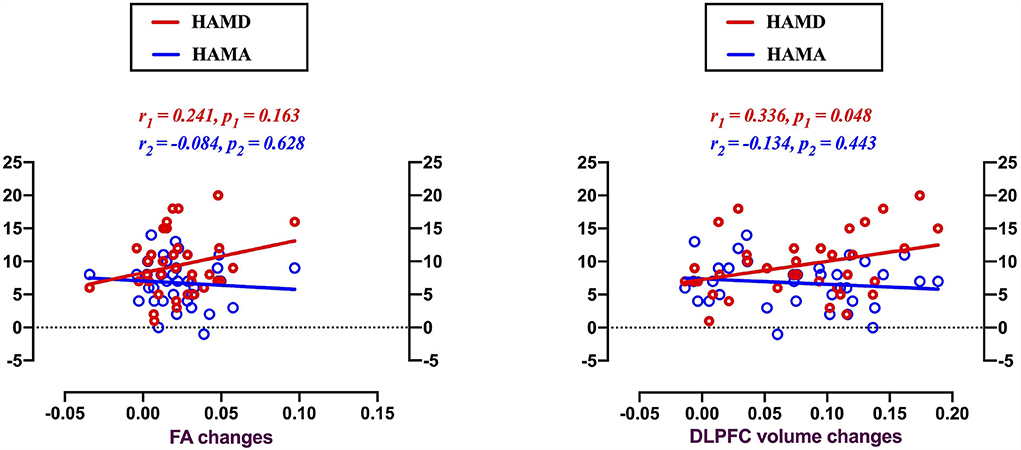
Figure 5. Voxel-based morphology comparison between MMD patients and HCs, and correlations between the reduction volumes and depression symptoms. Scatter plots indicating gray matter volume alterations in the DLPFC were found positively correlated with the HAMD score changes in MMD patients after 8 weeks SG-treatment (r = 0.336, p = 0.048). There were no significantly correlations between clinical symptom changes and FA value alterations.
There were no significant correlations between clinical symptoms and FA value alterations.
Discussion
The initial stage of a depressive disorder is MMD, and it is more common than severe depression in the general medical environment. The underlying mechanisms of MMD, especially the characteristics of brain structure, are poorly understood. In this study, we investigated brain structural alterations in patients with MMD compared with age- and sex-matched HCs and assessed the relationship between these brain structural alterations and clinical symptoms in patients with MMD. Our findings can be summarized as follows: at baseline, (1) FA values in patients with MMD were found to be significantly increased in the ALIC and PLIC, and FA values were significantly positively correlated with HAMD scores in patients with MMD; (2) patients with MMD showed smaller GM volume of the DLPFC, frontal cortex, occipital cortex, and precuneus than HCs, and the GM volume of the DLPFC was negatively correlated with HAMD scores. After SG treatment, we found that: (1) the HAMD scores decreased; (2) FA values were significantly decreased in the regions of the ALIC and PLIC compared to those at baseline and TBSS revealed no significant differences in FA values between patients with MMD and HCs; (3) the structural characteristics of the DLPFC in patients with MMD obtained at the 8th week were improved; for example, no significant differences were observed in GM volume of the DLPFC between the two groups. Our results provide neuroimaging evidence showing that SG is an effective treatment for patients with MMD. Moreover, alterations in GM volume after 8 weeks of SG treatment indicated a potential modulation mechanism in brain structural plasticity within the DLPFC in patients with MMD.
Deficits of white matter microstructure and its relationship with clinical characteristics in MMD
Structural properties were obtained using voxel-wise TBSS. The most commonly used parameter in DTI research is FA, which reflects the structural integrity and geometry of axonal fibers (Gulani and Sundgren, 2006). Greater FA may reflect greater myelination of WM fibers, an increased number of myelinated fibers, smaller axonal diameter, or reduced neural branches within the MRI voxel (Boero et al., 1999; Beaulieu, 2002). In this study, the pattern in patients with MMD was different from that in HCs; increased FA values were observed in the ALIC and PLIC in MMD.
The ALIC in human and non-human primates carries ascending and descending fibers from the OFC and ACC. The OFC and ACC are associated with several psychiatric disorders, including obsessive-compulsive disorder, MDD, posttraumatic stress disorder, and addiction (Mayberg et al., 1997; Volkow et al., 2011; Milad and Quirk, 2012; Haber and Heilbronner, 2013). Importantly, diseases linked to the OFC and ACC show abnormal volume, FA, and diffusivity in the internal capsule, and some studies have demonstrated these abnormalities, specifically in the ALIC (Lang et al., 2006; Wobrock et al., 2008; Zou et al., 2008; de Souza Duran et al., 2009; Jia et al., 2010; Togao et al., 2010; Upadhyay et al., 2010; Nakamae et al., 2011; Zhu et al., 2011; Koch et al., 2014; Kuan et al., 2015). In addition, the ALIC is a WM bundle between the head of the caudate nucleus and the lenticular nucleus, which plays an important role in motivation, decision-making, and evaluation of the saliency of emotional and reward stimuli (Henderson et al., 2013). Consistent with our findings, Hyett et al. found that FA was distinctly increased in the right anterior internal capsule in patients with melancholia compared to controls (Hyett et al., 2018), and Coloigner et al. reported patterns of increased FA in the splenium of the corpus callosum and PLIC in patients with depression (Coloigner et al., 2019). In addition, researchers also found consistent results in other mental diseases, such as schizophrenia, obsessive-compulsive disorder, and bipolar depression; that is, the FA values of ALIC and PLIC in patients were increased compared with those in HCs (Versace et al., 2010; Lochner et al., 2012; Alba-Ferrara and de Erausquin, 2013). In patients with depression, a higher FA in the ALIC and PLIC was associated with more severe overall clinical symptoms, which is consistent with previous studies that have shown a strong association between WM disruptions in these regions and the symptoms of depression. Since FA values were significantly positively correlated with HAMD scores in patients with MMD after 8 weeks of SG treatment, TBSS revealed that there were no significant differences in FA values between patients with MMD and HCs. Similarly, behavioral data showed no significant difference in HAMD scores between patients with MMD and HCs. The Chinese herbal medicine SG is mainly composed of Acanthopanax and H. perforatum. Acanthopanax, commonly known as Ciwujia or Siberian ginseng, is a traditional Chinese medicine that ameliorates cognitive dysfunction induced by cholinergic blockade (Li et al., 2016). H. perforatum, also known as St. John's wort, has been used as an antidepressant agent and cognitive enhancer in rodents (Avila et al., 2018). H. perforatum has been shown to induce positive effects on both mood and short-term verbal memory (Yechiam et al., 2019). The main drug components of SG have good therapeutic effects on the clinical symptoms of MMD. Therefore, after 8 weeks of SG treatment, the clinical symptoms of patients with MMD were reduced, and there was no significant difference compared with HCs, while the FA positively correlated with clinical symptoms was also reduced, and there was no significant difference compared with HCs.
Deficits of GM volume and its relationship with clinical characteristics in MMD
After taking sex, age, and baseline volume as covariates, brain GM volume of the DLPFC, frontal cortex, occipital cortex, and precuneus were significantly smaller in patients with MMD than in HCs. Consistent with our findings, several previous studies have reported differences in GM volume between patients with depression and HCs. For example, Lyoo et al. found evidence of decreased GM volume in the anterior cingulate gyrus, left medial frontal gyrus, and right precentral gyrus (Lyoo et al., 2004). Most studies have identified decreased GM volume in a wide range of brain regions, including the pre-supplementary motor area, parietal-temporal regions, frontal cortex, temporal cortex, cingulate cortex, insular cortex, parahippocampal gyrus, hippocampus, cerebellum, and OFC (Tang et al., 2007; Cheng et al., 2010; Lai et al., 2010; Zou et al., 2010; Peng et al., 2011; Ma et al., 2012; Wang et al., 2012; Zhang X. et al., 2012; Kong et al., 2014). Neuroimaging studies have produced interesting findings on the structural effects of antidepressant treatment in the prefrontal areas. The medial frontal gyrus and DLPFC volumes predict remission after antidepressant treatment (Chen et al., 2007; Yucel et al., 2009; Lorenzetti et al., 2010). Furthermore, effective treatment with fluoxetine and sertraline results in enlargement of the middle frontal gyrus, DLPFC, and OFC (Smith et al., 2013; Kong et al., 2014). Accordingly, remission has been shown to correlate with more preserved volumes of the anterior cingulate gyrus, dorsomedial prefrontal gyrus, and DLPFC over time (Frodl et al., 2008; Gunning et al., 2009). Furthermore, correlation analyses indicated that the GM volume of the DLPFC was negatively correlated with HAMD scores. Depression is characterized by dysfunction in cognitive, emotional, and behavioral processes related to emotion processing and regulation (Joormann and Gotlib, 2010). Thus, the study of neurobiologically informed models of depression focuses on brain regions underlying normative functioning of these processes (Drevets et al., 2008), including examining structural differences in these regions between patients with depression and healthy. The DLPFC is a large and functionally heterogeneous brain region (Glasser et al., 2016) and is involved in emotional and cognitive processing, and is one of the key players in the pathophysiology of mood disorders (Matsuo et al., 2019). Meta-analyses of MR images from patients with MDD have shown that compared with healthy subjects, patients with MDD have decreased GM volumes in the right DLPFC (Bora et al., 2012). In one another previous meta-analysis study, patients with MDD were shown to have reduced activation in the DLPFC (Fitzgerald et al., 2008). Besides, excitatory repetitive transcranial magnetic stimulation (rTMS) over the left DLPFC (Gu and Chang, 2017) or anodal left/cathodal right DLPFC transcranial direct current stimulation (tDCS) (Valiengo et al., 2017) have been shown to reduce depressive symptoms in post-stroke depression patients. This provides evidence for the negative correlation between DLPFC and MDD symptoms from the perspective of non-pharmacological interventions. In fact, stimulation at the DLPFC is the FDA-approved antidepressant intervention (Lan et al., 2016), and is based on the observation that, in depression, this structure has impaired functional connectivity (Sheline et al., 2010; Liston et al., 2014) and that left prefrontal cortical strokes increase the risk of depression (Shimoda and Robinson, 1999). The above studies are in line with a common idea that the structural changes of DLPFC either predict the onset of depression or reflect the damage caused by repeated emotions in depression patients.
A potential modulation mechanism in brain structural plasticity within the DLPFC in MMD
Multiple linear regression analysis showed that, as a typical mental disorder, MMD also has serious brain structural deficits, such as reduced GM volume, in addition to its etiology. Previous studies on depression have reported consistent findings regarding the association between the left DLPFC and clinical outcomes. Several lines of evidence suggest a role of the DLPFC in the phenotypes, onset, duration, and treatment efficacy of depression (Kinou et al., 2013; Tsujii et al., 2016). For example, previous studies have shown that patients with MDD have a significantly decreased GM volume in the left DLPFC, which plays a key role in positive emotional judgment. The significantly decreased GM volume we observed in the DLPFC of depression was in agreement with the clinical symptoms of diminished interest or pleasure in almost all activities in depression (Qi et al., 2022), supporting the view that DLPFC plays a crucial role in the symptomatology of depression (Koenigs and Grafman, 2009). This view is further supported by functional MRI study, which indicates that DLPFC activity was decreased and the functional connection (FC) between the amygdala and the DLPFC was reduced in untreated depression patients performing cognitive function tests (Siegle et al., 2007), and that in depression patients there was a widespread reduction in FC between the left DLPFC and other brain areas (Liston et al., 2014). Our results also provide evidence that DLPFC is a key brain region in the pathogenesis and rehabilitation of depression. At baseline, after controlling for the effects of age, sex, and total brain size, we observed that the GMV of the DLPFC, frontal cortex, occipital cortex, and precuneus was significantly smaller in patients with MMD than in HCs. And there were no significant differences in GMV between patients with MMD and HCs after 8 weeks of SG treatment. This means that after treatment, the structure of the above four brain regions has changed, that is, GMV has been improved. Then, we made a correlation analysis between the alterations of GMV in these four brain regions and the HAMD score changes. The results showed that only the alteration of GMV in DLPFC was positively correlated with the HAMD score change. We consider that the possible reason for this result is that as one of the key brain regions in the pathogenesis and rehabilitation of depression, DLPFC is a key hub involved in cognitive control, working memory, and emotion regulation. This means that DLPFC may be more sensitive to SG treatment than the other three brain regions. And in line with previous reports, alterations in GM volume after 8 weeks of SG treatment indicated a potential modulation mechanism in brain structural plasticity within the DLPFC in patients with MMD. In addition, PFC abnormalities observed in patients with MDD or rodent models are partly corrected by antidepressants. An 8-week escitalopram treatment indicated reduced irregular high functional connectivity in the bilateral dorsal medial PFC in patients with MDD, and a 2-week administration of a selective serotonin reuptake inhibitor, fluvoxamine, restored dendritic length, and spine densities, but not cortical thickness after early life stress exposure (Lyttle et al., 2015; Wang et al., 2015). Overall, our data suggest that the degree of depression in MMD largely depends on the GM volume of the DLPFC in a given patient.
Limitations
First, the sample size of patients was limited and involved patients with varying severity and duration of disease, which limits the investigation of the detailed role of each mechanism in the development of MMD. Second, the causal relationship between pathophysiological factors and depression severity should be detected not only based on a large sample of patients but also using some effective experimental designs, for example, a longitudinal study to monitor the transition from MMD to major depression. Of course, such a study would be fraught with additional challenges based on time effects and the likely contaminating effects of medication or therapeutic interventions to address depression. Third, in addition to the severity of depression, other factors such as the duration of depression and the number of depressive episodes can regulate the brain structure. Unfortunately, we have not collected information on these factors. Finally, our study may have underestimated the extent of structural changes associated with depression. Insufficient sensitivity may have arisen because of the limited sample size. Thus, if there were mood-state-dependent changes in GM volume, we may not have detected them because of insufficient power.
Further prospects
Despite these limitations, this study provides further evidence that the PFC might play a key role in the regulation of depression in the pathophysiology of MMD. Future studies using a larger sample size or longitudinal studies are needed to replicate and generalize these results across patients with severe depression. More detailed insights into the structural and functional brain plasticity underlying MMD will shed light on the development of new and more targeted treatment options in clinical practice.
Data availability statement
The raw data supporting the conclusions of this article will be made available by the authors, without undue reservation.
Ethics statement
The studies involving human participants were reviewed and approved by the Ethics Committee of Shanxi Medical University. The patients/participants provided their written informed consent to participate in this study.
Author contributions
YL: data curation, formal analysis, and writing—original draft. JW: data curation and writing, reviewing, and editing the manuscript. XY: investigation and resources. HL: supervision and writing, reviewing, and editing the manuscript. All authors contributed to and approved the final manuscript.
Funding
This work was supported by the National Key Research and Development Program of China (2016YFC1307004), the National Natural Science Foundation of China (81971601, 82101610, and 81571319), the Wu Jieping Foundation (320.6750.18283), the Special Project for Guiding the Transformation of Scientific and Technological Achievements in Shanxi Province (201904D131020), and the Multidisciplinary Team for Cognitive Impairment of Shanxi Science and Technology Innovation Training Team (201705D131027).
Acknowledgments
We thank Shanxi Quan-Xin Science and Technology Co., Ltd. for the data processing and thank Dr. Zhongshi Jin for critically proofreading the manuscript.
Conflict of interest
The authors declare that the research was conducted in the absence of any commercial or financial relationships that could be construed as a potential conflict of interest.
Publisher's note
All claims expressed in this article are solely those of the authors and do not necessarily represent those of their affiliated organizations, or those of the publisher, the editors and the reviewers. Any product that may be evaluated in this article, or claim that may be made by its manufacturer, is not guaranteed or endorsed by the publisher.
References
Alba-Ferrara, L., and de Erausquin, G. A. (2013). What does anisotropy measure? Insights from increased and decreased anisotropy in selective fiber tracts in schizophrenia. Front. Integr. Neurosci. 7, 9. doi: 10.3389/fnint.2013.00009
Ancelin, M. L., Carriere, I., Artero, S., Maller, J., Meslin, C., Ritchie, K., et al. (2019). Lifetime major depression and grey-matter volume. J. Psychiatry Neurosci. 44, 45–53. doi: 10.1503/jpn.180026
Avila, C., Whitten, D., and Evans, S. (2018). The safety of St John's wort (Hypericum perforatum) in pregnancy and lactation: a systematic review of rodent studies. Phytother. Res. 32, 1488–1500. doi: 10.1002/ptr.6099
Beaulieu, C. (2002). The basis of anisotropic water diffusion in the nervous system - a technical review. NMR Biomed. 15, 435–455. doi: 10.1002/nbm.782
Ben-Eliezer, D., and Yechiam, E. (2016). Hypericum perforatum as a cognitive enhancer in rodents: a meta-analysis. Sci. Rep. 6, 35700. doi: 10.1038/srep35700
Boero, J. A., Ascher, J., Arregui, A., Rovainen, C., and Woolse, A. T. (1999). Increased brain capillaries in chronic hypoxia. J. Appl. Physiol. 86, 1211–1219. doi: 10.1152/jappl.1999.86.4.1211
Bora, E., Fornito, A., Pantelis, C., and Yucel, M. (2012). Gray matter abnormalities in major depressive disorder: a meta-analysis of voxel based morphometry studies. J. Affect. Disord. 138, 9–18. doi: 10.1016/j.jad.2011.03.049
Bremner, J. D., Vythilingam, M., Vermetten, E., Nazeer, A., Adil, J., Khan, S., et al. (2002). Reduced volume of orbitofrontal cortex in major depression. Biol. Psychiatry 51, 273–279. doi: 10.1016/S0006-3223(01)01336-1
Catani, M. (2006). Diffusion tensor magnetic resonance imaging tractography in cognitive disorders. Curr. Opin. Neurol. 19, 599–606. doi: 10.1097/01.wco.0000247610.44106.3f
Chen, C. H., Ridler, K., Suckling, J., Williams, S., Fu, C. H., Merlo-Pich, E., et al. (2007). Brain imaging correlates of depressive symptom severity and predictors of symptom improvement after antidepressant treatment. Biol. Psychiatry 62, 407–414. doi: 10.1016/j.biopsych.2006.09.018
Cheng, Y. Q., Xu, J., Chai, P., Li, H. J., Luo, C. R., Yang, T., et al. (2010). Brain volume alteration and the correlations with the clinical characteristics in drug-naive first-episode MDD patients: a voxel-based morphometry study. Neurosci. Lett. 480, 30–34. doi: 10.1016/j.neulet.2010.05.075
Cleare, A., Pariante, C. M., Young, A. H., Anderson, I. M., Christmas, D., Cowen, P. J., et al. (2015). Evidence-based guidelines for treating depressive disorders with antidepressants: a revision of the 2008 british association for psychopharmacology guidelines. J. Psychopharmacol. 29, 459–525. doi: 10.1177/0269881115581093
Cole, J., Chaddock, C. A., Farmer, A. E., Aitchison, K. J., Simmons, A., McGuffin, P., et al. (2012). White matter abnormalities and illness severity in major depressive disorder. Br. J. Psychiatry 201, 33–39. doi: 10.1192/bjp.bp.111.100594
Coloigner, J., Batail, J. M., Commowick, O., Corouge, I., Robert, G., Barillot, C., et al. (2019). White matter abnormalities in depression: a categorical and phenotypic diffusion MRI study. Neuroimage Clin. 22, 101710. doi: 10.1016/j.nicl.2019.101710
de Souza Duran, F. L., Hoexter, M. Q., Valente, A. A. Jr, and Miguel, C. E. (2009). Association between symptom severity and internal capsule volume in obsessive-compulsive disorder. Neurosci. Lett. 452, 68–71. doi: 10.1016/j.neulet.2009.01.007
Dichter, G. S., Gibbs, D., and Smoski, J. M. (2015). A systematic review of relations between resting-state functional-MRI and treatment response in major depressive disorder. J. Affect. Disord. 172, 8–17. doi: 10.1016/j.jad.2014.09.028
Disease, G. B. D., Injury, I., and Prevalence, C. (2018). Global, regional, national incidence. prevalence, and years lived with disability for 354 diseases and injuries for 195 countries and territories, 1990-2017: a systematic analysis for the global burden of disease study 2017. Lancet 392, 1789–1858. doi: 10.1016/S0140-6736(18)32279-7
Drevets, W. C., Price, J. L., and Furey, L. M. (2008). Brain structural and functional abnormalities in mood disorders: implications for neurocircuitry models of depression. Brain Struct. Funct. 213, 93–118. doi: 10.1007/s00429-008-0189-x
Dusi, N., Barlati, S., Vita, A., and Brambilla, P. (2015). Brain structural effects of antidepressant treatment in major depression. Curr. Neuropharmacol. 13, 458–465. doi: 10.2174/1570159X1304150831121909
Eker, C., and Gonul, A. S. (2010). Volumetric MRI studies of the hippocampus in major depressive disorder: meanings of inconsistency and directions for future research. World J. Biol. Psychiatry 11, 19–35. doi: 10.3109/15622970902737998
Fitzgerald, P. B., Laird, A. R., Maller, J., and Daskalakis, J. Z. (2008). A meta-analytic study of changes in brain activation in depression. Hum. Brain Mapp. 29, 683–695. doi: 10.1002/hbm.20426
Frodl, T. S., Koutsouleris, N., Bottlender, R., Born, C., Jager, M., Scupin, I., et al. (2008). Depression-related variation in brain morphology over 3 years: effects of stress? Arch. Gen. Psychiatry 65, 1156–1165. doi: 10.1001/archpsyc.65.10.1156
Fu, C. H., Costafreda, S. G., Sankar, A., Adams, T. M., Rasenick, M. M., Liu, P., et al. (2015). Multimodal functional and structural neuroimaging investigation of major depressive disorder following treatment with duloxetine. BMC Psychiatry 15, 82. doi: 10.1186/s12888-015-0457-2
Glasser, M. F., Coalson, T. S., Robinson, E. C., Hacker, C. D., Harwell, J., Yacoub, E., et al. (2016). A multi-modal parcellation of human cerebral cortex. Nature 536, 171–178. doi: 10.1038/nature18933
Groman, S. M., Morales, A. M., Lee, B., London, E. D., and Jentsch, D. J. (2013). Methamphetamine-induced increases in putamen gray matter associate with inhibitory control. Psychopharmacology 229, 527–538. doi: 10.1007/s00213-013-3159-9
Gu, S. Y., and Chang, M. C. (2017). The effects of 10-Hz repetitive transcranial magnetic stimulation on depression in chronic stroke patients. Brain Stimul. 10, 270–274. doi: 10.1016/j.brs.2016.10.010
Gulani, V., and Sundgren, P. C. (2006). Diffusion tensor magnetic resonance imaging. J. Neuroophthalmol. 26, 51–60. doi: 10.1097/01.wno.0000205978.86281.3e
Gunning, F. M., Cheng, J., Murphy, C. F., Kanellopoulos, D., Acuna, J., Hoptman, M. J., et al. (2009). Anterior cingulate cortical volumes and treatment remission of geriatric depression. Int. J. Geriatr. Psychiatry 24, 829–836. doi: 10.1002/gps.2290
Haber, S. N., and Heilbronner, S. R. (2013). Translational research in OCD: circuitry and mechanisms. Neuropsychopharmacology 38, 252–253. doi: 10.1038/npp.2012.182
He, M., Cheng, Y., Chu, Z., Xu, J., Lu, Y., Shen, Z., et al. (2022). Disrupted default mode network and executive control network are associated with depression severity on structural network. Neuroreport 33, 227–235. doi: 10.1097/WNR.0000000000001773
Henderson, S. E., Johnson, A. R., Vallejo, A. I., Katz, L., Wong, E., and Gabbay, V. (2013). A preliminary study of white matter in adolescent depression: relationships with illness severity, anhedonia, and irritability. Front. Psychiatry 4, 152. doi: 10.3389/fpsyt.2013.00152
Huang, Y., Wang, Y., Wang, H., Liu, Z., Yu, X., Yan, J., et al. (2019). Prevalence of mental disorders in China: a cross-sectional epidemiological study. Lancet Psychiatry 6, 211–224. doi: 10.1016/S2215-0366(18)30511-X
Hyett, M. P., Perry, A., Breakspear, M., Wen, W., and Parker, B. G. (2018). White matter alterations in the internal capsule and psychomotor impairment in melancholic depression. PLoS ONE 13, e0195672. doi: 10.1371/journal.pone.0195672
Jia, Z., Huang, X., Wu, Q., Zhang, T., Lui, S., Zhang, J., et al. (2010). High-field magnetic resonance imaging of suicidality in patients with major depressive disorder. Am. J. Psychiatry 167, 1381–1390. doi: 10.1176/appi.ajp.2010.09101513
Jiang, J., Zhao, Y. J., Hu, X. Y., Du, M. Y., Chen, Z. Q., Wu, M., et al. (2017). Microstructural brain abnormalities in medication-free patients with major depressive disorder: a systematic review and meta-analysis of diffusion tensor imaging. J. Psychiatry Neurosci. 42, 150–163. doi: 10.1503/jpn.150341
Joormann, J., and Gotlib, I. H. (2010). Emotion regulation in depression: relation to cognitive inhibition. Cogn. Emot. 24, 281–298. doi: 10.1080/02699930903407948
Kendler, K. S., Fiske, A., Gardner, C. O., and Gatz, M. (2009). Delineation of two genetic pathways to major depression. Biol. Psychiatry 65, 808–811. doi: 10.1016/j.biopsych.2008.11.015
Kieseppa, T., Eerola, M., Mantyla, R., Neuvonen, T., Poutanen, V. P., Luoma, K., et al. (2010). Major depressive disorder and white matter abnormalities: a diffusion tensor imaging study with tract-based spatial statistics. J. Affect. Disord. 120, 240–244. doi: 10.1016/j.jad.2009.04.023
Kinou, M., Takizawa, R., Marumo, K., Kawasaki, S., Kawakubo, Y., Fukuda, M., et al. (2013). Differential spatiotemporal characteristics of the prefrontal hemodynamic response and their association with functional impairment in schizophrenia and major depression. Schizophr. Res. 150, 459–467. doi: 10.1016/j.schres.2013.08.026
Klauser, P., Fornito, A., Lorenzetti, V., Davey, C. G., Dwyer, D. B., Allen, N. B., et al. (2015). Cortico-limbic network abnormalities in individuals with current and past major depressive disorder. J. Affect. Disord. 173, 45–52. doi: 10.1016/j.jad.2014.10.041
Koch, K., Reess, T. J., Rus, O. G., Zimmer, C., and Zaudig, M. (2014). Diffusion tensor imaging (DTI) studies in patients with obsessive-compulsive disorder (OCD): a review. J. Psychiatr. Res. 54, 26–35. doi: 10.1016/j.jpsychires.2014.03.006
Koenigs, M., and Grafman, J. (2009). The functional neuroanatomy of depression: distinct roles for ventromedial and dorsolateral prefrontal cortex. Behav. Brain Res. 201, 239–243. doi: 10.1016/j.bbr.2009.03.004
Kong, L., Wu, F., Tang, Y., Ren, L., Kong, D., Liu, Y., et al. (2014). Frontal-subcortical volumetric deficits in single episode, medication-naive depressed patients and the effects of 8 weeks fluoxetine treatment: a VBM-DARTEL study. PLoS ONE 9, e79055. doi: 10.1371/journal.pone.0079055
Koolschijn, P. C., van Haren, N. E., Lensvelt-Mulders, G. J., Hulshoff Pol, H. E., and Kahn, S. R. (2009). Brain volume abnormalities in major depressive disorder: a meta-analysis of magnetic resonance imaging studies. Hum. Brain Mapp. 30, 3719–3735. doi: 10.1002/hbm.20801
Kronenberg, G., Tebartz van Elst, L., Regen, F., Deuschle, M., Heuser, I., and Colla, M. (2009). Reduced amygdala volume in newly admitted psychiatric in-patients with unipolar major depression. J. Psychiatr. Res. 43, 1112–1117. doi: 10.1016/j.jpsychires.2009.03.007
Kuan, L., Li, Y., Lau, C., Feng, D., Bernard, A., Sunkin, S. M., et al. (2015). Neuroinformatics of the allen mouse brain connectivity atlas. Methods 73, 4–17. doi: 10.1016/j.ymeth.2014.12.013
Lai, C. H., Hsu, Y. Y., and Wu, T. Y. (2010). First episode drug-naive major depressive disorder with panic disorder: gray matter deficits in limbic and default network structures. Eur. Neuropsychopharmacol. 20, 676–682. doi: 10.1016/j.euroneuro.2010.06.002
Lan, M. J., Chhetry, B. T., Liston, C., Mann, J. J., and Dubin, M. (2016). Transcranial magnetic stimulation of left dorsolateral prefrontal cortex induces brain morphological changes in regions associated with a treatment resistant major depressive episode: an exploratory analysis. Brain Stimul. 9, 577–583. doi: 10.1016/j.brs.2016.02.011
Lang, D. J., Khorram, B., Goghari, V. M., Kopala, L. C., Vandorpe, R. A., Rui, Q., et al. (2006). Reduced anterior internal capsule and thalamic volumes in first-episode psychosis. Schizophr. Res. 87, 89–99. doi: 10.1016/j.schres.2006.05.002
Li, T., Ferns, K., Yan, Z. Q., Yin, S. Y., Kou, J. J., Li, D., et al. (2016). Acanthopanax senticosus: photochemistry and anticancer potential. Am. J. Chin. Med. 44, 1543–1558. doi: 10.1142/S0192415X16500865
Liao, Y., Huang, X., Wu, Q., Yang, C., Kuang, W., Du, M., et al. (2013). Is depression a disconnection syndrome? Meta-analysis of diffusion tensor imaging studies in patients with MDD. J. Psychiatry Neurosci. 38, 49–56. doi: 10.1503/jpn.110180
Liston, C., Chen, A. C., Zebley, B. D., Drysdale, A. T., Gordon, R., Leuchter, B., et al. (2014). Default mode network mechanisms of transcranial magnetic stimulation in depression. Biol. Psychiatry 76, 517–526. doi: 10.1016/j.biopsych.2014.01.023
Liu, J., Xu, X., Luo, Q., Luo, Y., Chen, Y., Lui, S., et al. (2017). Brain grey matter volume alterations associated with antidepressant response in major depressive disorder. Sci. Rep. 7, 10464. doi: 10.1038/s41598-017-10676-5
Liu, S., Zhao, W., Li, Y., Li, X., Li, J., Cao, H., et al. (2020). Improve cognition of depressive patients through the regulation of basal ganglia connectivity: combined medication using Shuganjieyu capsule. J. Psychiatr. Res. 123, 39–47. doi: 10.1016/j.jpsychires.2020.01.013
Lochner, C., Fouch,é, J.-P., du Plessis, S., Spottiswoode, B., Seedat, S., Fineberg, N., et al. (2012). Evidence for fractional anisotropy and mean diffusivity white matter abnormalities in the internal capsule and cingulum in patients with obsessive–compulsive disorder. J. Psychiatry Neurosci. 37, 193–199. doi: 10.1503/jpn.110059
Lorenzetti, V., Allen, N. B., Whittle, S., and Yucel, M. (2010). Amygdala volumes in a sample of current depressed and remitted depressed patients and healthy controls. J. Affect. Disord. 120, 112–119. doi: 10.1016/j.jad.2009.04.021
Lyoo, I. K., Kim, M. J., Stoll, A. L., Demopulos, C. M., Parow, A. M., Dager, S. R., et al. (2004). Frontal lobe gray matter density decreases in bipolar I disorder. Biol. Psychiatry 55, 648–651. doi: 10.1016/j.biopsych.2003.10.017
Lyttle, K., Ohmura, Y., Konno, K., Yoshida, T., Izumi, T., Watanabe, M., et al. (2015). Repeated fluvoxamine treatment recovers juvenile stress-induced morphological changes and depressive-like behavior in rats. Brain Res. 1616, 88–100. doi: 10.1016/j.brainres.2015.04.058
Ma, C., Ding, J., Li, J., Guo, W., Long, Z., Liu, F., et al. (2012). Resting-state functional connectivity bias of middle temporal gyrus and caudate with altered gray matter volume in major depression. PLoS ONE 7, e45263. doi: 10.1371/journal.pone.0045263
Matsuo, K., Harada, K., Fujita, Y., Okamoto, Y., Ota, M., Narita, H., et al. (2019). Distinctive neuroanatomical substrates for depression in bipolar disorder versus major depressive disorder. Cerebral Cortex 29, 202–214. doi: 10.1093/cercor/bhx319
Mayberg, H. S., Brannan, S. K., Mahurin, R. K., Jerabek, P. A., Brickman, J. S., Tekell, J. L., et al. (1997). Cingulate function in depression: a potential predictor of treatment response. Neuroreport 8, 1057–1061. doi: 10.1097/00001756-199703030-00048
Meng, Y., Li, H., Wang, J., Xu, Y., and Wang, B. (2021). Cognitive behavioral therapy for patients with mild to moderate depression: treatment effects and neural mechanisms. J. Psychiatr. Res. 136, 288–295. doi: 10.1016/j.jpsychires.2021.02.001
Milad, M. R., and Quirk, G. J. (2012). Fear extinction as a model for translational neuroscience: ten years of progress. Annu. Rev. Psychol. 63, 129–151. doi: 10.1146/annurev.psych.121208.131631
Mills, K. L., and Tamnes, C. K. (2014). Methods and considerations for longitudinal structural brain imaging analysis across development. Dev. Cogn. Neurosci. 9, 172–190. doi: 10.1016/j.dcn.2014.04.004
Nakamae, T., Narumoto, J., Sakai, Y., Nishida, S., Yamada, K., Nishimura, T., et al. (2011). Diffusion tensor imaging and tract-based spatial statistics in obsessive-compulsive disorder. J. Psychiatr. Res. 45, 687–690. doi: 10.1016/j.jpsychires.2010.09.016
Oldfield, R. C. (1971). The assessment and analysis of handedness: the Edinburgh inventory. Neuropsychologia 9, 97–113. doi: 10.1016/0028-3932(71)90067-4
Peng, J., Liu, J., Nie, B., Li, Y., Shan, B., Wang, G., et al. (2011). Cerebral and cerebellar gray matter reduction in first-episode patients with major depressive disorder: a voxel-based morphometry study. Eur. J. Radiol. 80, 395–399. doi: 10.1016/j.ejrad.2010.04.006
Qi, Y. J., Lu, Y. R., Shi, L. G., Demmers, J. A. A., Bezstarosti, K., Rijkers, E., et al. (2022). Distinct proteomic profiles in prefrontal subareas of elderly major depressive disorder and bipolar disorder patients. Transl. Psychiatry 12, 275. doi: 10.1038/s41398-022-02040-7
Sheline, Y. I., Price, J. L., Yan, Z., and Mintun, A. M. (2010). Resting-state functional MRI in depression unmasks increased connectivity between networks via the dorsal nexus. Proc. Natl. Acad. Sci. USA. 107, 11020–11025. doi: 10.1073/pnas.1000446107
Shimoda, K., and Robinson, R. G. (1999). The relationship between poststroke depression and lesion location in long-term follow-up. Biol. Psychiatry 45, 187–192. doi: 10.1016/S0006-3223(98)00178-4
Siegle, G. J., Thompson, W., Carter, C. S., Steinhauer, S. R., and Thase, E. M. (2007). Increased amygdala and decreased dorsolateral prefrontal BOLD responses in unipolar depression: related and independent features. Biol. Psychiatry 61, 198–209. doi: 10.1016/j.biopsych.2006.05.048
Sithisarn, P., Rojsanga, P., Jarikasem, S., Tanaka, K., and Matsumoto, K. (2013). Ameliorative effects of acanthopanax trifoliatus on cognitive and emotional deficits in olfactory bulbectomized mice: an animal model of depression and cognitive deficits. Evid. Based Complement. Alternat. Med. 2013, 701956. doi: 10.1155/2013/701956
Smith, R., Chen, K., Baxter, L., Fort, C., and Lane, D. R. (2013). Antidepressant effects of sertraline associated with volume increases in dorsolateral prefrontal cortex. J. Affect. Disord. 146, 414–419. doi: 10.1016/j.jad.2012.07.029
Smith, S. M., Jenkinson, M., Johansen-Berg, H., Rueckert, D., Nichols, T. E., Mackay, C. E., et al. (2006). Tract-based spatial statistics: voxelwise analysis of multi-subject diffusion data. Neuroimage 31, 1487–1505. doi: 10.1016/j.neuroimage.2006.02.024
Smith, S. M., Zhang, Y. Y., Jenkinson, M., Chen, J., Matthews, P. M., Federico, A., et al. (2002). Accurate, robust, and automated longitudinal and cross-sectional brain change analysis. Neuroimage 17, 479–489. doi: 10.1006/nimg.2002.1040
Tang, Y., Wang, F., Xie, G., Liu, J., Li, L., Su, L., et al. (2007). Reduced ventral anterior cingulate and amygdala volumes in medication-naive females with major depressive disorder: a voxel-based morphometric magnetic resonance imaging study. Psychiatry Res. 156, 83–86. doi: 10.1016/j.pscychresns.2007.03.005
Togao, O., Yoshiura, T., Nakao, T., Nabeyama, M., Sanematsu, H., Nakagawa, A., et al. (2010). Regional gray and white matter volume abnormalities in obsessive-compulsive disorder: a voxel-based morphometry study. Psychiatry Res. 184, 29–37. doi: 10.1016/j.pscychresns.2010.06.011
Tsujii, N., Mikawa, W., Tsujimoto, E., Akashi, H., Adachi, T., Kirime, E., et al. (2016). Relationship between prefrontal hemodynamic responses and quality of life differs between melancholia and non-melancholic depression. Psychiatry Res. Neuroimaging 253, 26–35. doi: 10.1016/j.pscychresns.2016.04.015
Upadhyay, J., Maleki, N., Potter, J., Elman, I., Rudrauf, D., Knudsen, J., et al. (2010). Alterations in brain structure and functional connectivity in prescription opioid-dependent patients. Brain 133, 2098–2114. doi: 10.1093/brain/awq138
Valiengo, L. C. Goulart, A. C. de Oliveira, J. F. Benseñor, I. M. Lotufo, P. A. Brunoni, R. A. (2017). Transcranial direct current stimulation for the treatment of post-stroke depression: results from a randomised, sham-controlled, double-blinded trial. J. Neurol. Neurosurg. Psychiatry 88, 170–175. doi: 10.1136/jnnp-2016-314075
Versace, A., Almeida, J. R., Quevedo, K., Thompson, W. K., Terwilliger, R. A., Hassel, S., et al. (2010). Right orbitofrontal corticolimbic and left corticocortical white matter connectivity differentiate bipolar and unipolar depression. Biol. Psychiatry 68, 560–567. doi: 10.1016/j.biopsych.2010.04.036
Vilgis, V., Vance, A., Cunnington, R., and Silk, J. T. (2017). White matter microstructure in boys with persistent depressive disorder. J. Affect. Disord. 221, 11–16. doi: 10.1016/j.jad.2017.06.020
Volkow, N. D., Wang, G. J., Fowler, J. S., Tomasi, D., and Telang, F. (2011). Addiction: beyond dopamine reward circuitry. Proc. Natl. Acad. Sci. USA. 108, 15037–15042. doi: 10.1073/pnas.1010654108
Wang, L., Dai, W., Su, Y., Wang, G., Tan, Y., Jin, Z., et al. (2012). Amplitude of low-frequency oscillations in first-episode, treatment-naive patients with major depressive disorder: a resting-state functional MRI study. PLoS ONE 7, e48658. doi: 10.1371/journal.pone.0048658
Wang, L., Xia, M., Li, K., Zeng, Y., Su, Y., Dai, W., et al. (2015). The effects of antidepressant treatment on resting-state functional brain networks in patients with major depressive disorder. Hum. Brain Mapp. 36, 768–778. doi: 10.1002/hbm.22663
Wobrock, T., Kamer, T., Roy, A., Vogeley, K., Schneider-Axmann, T., Wagner, M., et al. (2008). Reduction of the internal capsule in families affected with schizophrenia. Biol. Psychiatry 63, 65–71. doi: 10.1016/j.biopsych.2007.02.026
Yechiam, E., Ben-Eliezer, D., Ashby, N. J. S., and Bar-Shaked, M. (2019). The acute effect of Hypericum perforatum on short-term memory in healthy adults. Psychopharmacology 236, 613–623. doi: 10.1007/s00213-018-5088-0
Yucel, K., McKinnon, M., Chahal, R., Taylor, V., Macdonald, K., Joffe, R., et al. (2009). Increased subgenual prefrontal cortex size in remitted patients with major depressive disorder. Psychiatry Res. 173, 71–76. doi: 10.1016/j.pscychresns.2008.07.013
Yuen, G. S., Gunning, F. M., Woods, E., Klimstra, S. A., Hoptman, M. J., and Alexopoulos, S. G. (2014). Neuroanatomical correlates of apathy in late-life depression and antidepressant treatment response. J. Affect. Disord. 166, 179–186. doi: 10.1016/j.jad.2014.05.008
Zeng, L. L., Liu, L., Liu, Y., Shen, H., Li, Y., and Hu, D. (2012). Antidepressant treatment normalizes white matter volume in patients with major depression. PLoS ONE 7, e44248. doi: 10.1371/journal.pone.0044248
Zhang, H., Lin, J., Sun, Y., Huang, Y., Ye, H., Wang, X., et al. (2012). Compromised white matter microstructural integrity after mountain climbing: evidence from diffusion tensor imaging. High Alt. Med. Biol. 13, 118–125. doi: 10.1089/ham.2011.1073
Zhang, X., Kang, D., Zhang, L., and Peng, L. (2014). Shuganjieyu capsule for major depressive disorder (MDD) in adults: a systematic review. Aging Ment. Health 18, 941–953. doi: 10.1080/13607863.2014.899975
Zhang, X., Yao, S., Zhu, X., Wang, X., Zhu, X., and Zhong, M. (2012). Gray matter volume abnormalities in individuals with cognitive vulnerability to depression: a voxel-based morphometry study. J. Affect. Disord. 136, 443–452. doi: 10.1016/j.jad.2011.11.005
Zhu, X., Wang, X., Xiao, J., Zhong, M., Liao, J., and Yao, S. (2011). Altered white matter integrity in first-episode, treatment-naive young adults with major depressive disorder: a tract-based spatial statistics study. Brain Res. 1369, 223–229. doi: 10.1016/j.brainres.2010.10.104
Zou, K., Deng, W., Li, T., Zhang, B., Jiang, L., Huang, C., et al. (2010). Changes of brain morphometry in first-episode, drug-naive, non-late-life adult patients with major depression: an optimized voxel-based morphometry study. Biol. Psychiatry 67, 186–188. doi: 10.1016/j.biopsych.2009.09.014
Keywords: mild-to-moderate depression, fractional anisotropy, subcortical volume, dorsolateral prefrontal cortex, Shuganjieyu capsule
Citation: Li Y, Wang J, Yan X and Li H (2022) Combined fractional anisotropy and subcortical volumetric deficits in patients with mild-to-moderate depression: Evidence from the treatment of antidepressant traditional Chinese medicine. Front. Neurosci. 16:959960. doi: 10.3389/fnins.2022.959960
Received: 02 June 2022; Accepted: 29 July 2022;
Published: 23 August 2022.
Edited by:
L. Tugan Muftuler, Medical College of Wisconsin, United StatesReviewed by:
Zonglin Shen, The First Affiliated Hospital of Kunming Medical University, ChinaBurak Yulug, Alanya Alaaddin Keykubat University, Turkey
Copyright © 2022 Li, Wang, Yan and Li. This is an open-access article distributed under the terms of the Creative Commons Attribution License (CC BY). The use, distribution or reproduction in other forums is permitted, provided the original author(s) and the copyright owner(s) are credited and that the original publication in this journal is cited, in accordance with accepted academic practice. No use, distribution or reproduction is permitted which does not comply with these terms.
*Correspondence: Hong Li, 18734558363@163.com
†These authors have contributed equally to this work