- 1Department of Clinical Psychology, The Affiliated Brain Hospital of Guangzhou Medical University, Guangzhou, China
- 2Department of Radiology, The Affiliated Brain Hospital of Guangzhou Medical University, Guangzhou, China
Objective: Childhood trauma is a strong predictor of major depressive disorder (MDD). Women are more likely to develop MDD than men. However, the neural basis of female MDD patients with childhood trauma remains unclear. We aimed to identify the specific brain regions that are associated with female MDD patients with childhood trauma.
Methods: We recruited 16 female MDD patients with childhood trauma, 16 female MDD patients without childhood trauma, and 20 age- and education level-matched healthy controls. All participants underwent resting-state functional magnetic resonance imaging (MRI). Regional brain activity was evaluated as the amplitude of low-frequency fluctuation (ALFF). Furthermore, functional connectivity (FC) analyses were performed on areas with altered ALFF to explore alterations in FC patterns.
Results: There was increased ALFF in the left middle frontal gyrus (MFG) and the right postcentral gyrus (PoCG) in MDD with childhood trauma compared with MDD without childhood trauma. The areas with significant ALFF discrepancies were selected as seeds for the FC analyses. There was increased FC between the left MFG and the bilateral putamen gyrus. Moreover, ALFF values were correlated with childhood trauma severity.
Conclusion: Our findings revealed abnormal intrinsic brain activity and FC patterns in female MDD patients with childhood trauma, which provides new possibilities for exploring the pathophysiology of this disorder in women.
Introduction
Major depressive disorder (MDD) is a serious mental disorder that affects mood, interest, and cognitive function (Otte et al., 2016). It has enduring impacts throughout life (Kessler et al., 2007) and heavy economic and social burdens (Murray et al., 2012; Ferrari et al., 2013). Women are approximately twice as likely to experience MDD as men (Seedat et al., 2009). Thus, being a woman is a risk factor for developing MDD (Otte et al., 2016). Researchers have speculated that the sex differences in MDD development might relate to variations in susceptibility (both physical and psychological) as well as environmental factors that work at both the micro and macro levels (Kuehner, 2017). However, the neural mechanisms underlying female MDD patients remain unclear.
Childhood trauma is a common psychological stressor and includes experiences of abuse and neglect (Bernstein et al., 2003). Multiple studies have reported that childhood trauma can predict psychiatric disorders such as bipolar disorder, anxiety, substance use disorder, post-traumatic stress disorder, and MDD (Baldwin et al., 2019; Hailes et al., 2019; McKay et al., 2021). There are sex differences in childhood trauma. Compared with men, the impact of childhood trauma is even more profound in women. Women also have more complex patterns of childhood trauma (Haahr-Pedersen et al., 2020), and the female sex also plays a synergistic role with childhood trauma in certain mental disorders (e.g., anxiety and depressive episodes) (Whitaker et al., 2021). Previous studies of childhood trauma have focused on neuroendocrinology (Silva et al., 2021; Tan et al., 2021), neuroinflammation (Andersen, 2022), and neuroimaging (Tozzi et al., 2020; Ma et al., 2021) to analyze the intrinsic biological mechanisms of MDD. However, trauma-related brain dysfunction is not fully understood. In particular, brain neuroimaging studies of women with childhood trauma experience remain severely lacking. It is, therefore, important to investigate the pathophysiology and etiology of MDD in women who have experienced childhood trauma.
A growing body of evidence indicates that the amplitude of the low-frequency fluctuation (ALFF) method can be used to capture local brain activity and identify various physiological conditions in the brain (Yang et al., 2007; Yan et al., 2009). Previous studies have detected ALFF alterations in MDD with childhood trauma, including in the left insula, right dorsal anterior cingulate cortex, bilateral amygdala, and left orbital/cerebellum (Du et al., 2016; Wu et al., 2020). Moreover, functional connectivity (FC) methods have been developed to measure both the temporal correlations (Du et al., 2016; Wu et al., 2020) and the coordination of brain activity (Biswal et al., 1995; Noble et al., 2019) among multiple brain regions. Yu et al. (2019) reported that childhood trauma across different dimensions of symptoms is associated with abnormal network architecture in patients with MDD. A combination of ALFF and FC has been recommended to investigate abnormal intrinsic brain function in patients with MDD (Hu et al., 2019; Ebneabbasi et al., 2021; Yan et al., 2022). However, ALFF and FC alterations in female MDD patients with childhood trauma have not yet been investigated. To address this gap, we used ALFF and FC methods to explore brain function and FC patterns in female MDD patients with childhood trauma. The aim of this study was to provide new insights into the underlying neurobiological mechanisms of the disease.
Materials and methods
Participants
Individual mentalization in early adulthood is not yet fully matured, and individuals in early adulthood are more vulnerable to childhood traumatic experiences (Sonu et al., 2019; Hamlat et al., 2021). Interestingly, the scholar found that early adulthood is the peak period of MDD onset (Kessler et al., 2007). However, mental illness in early adulthood did not raise major attention and age has not been well controlled in previous studies. Thus, we only included female participants in early adulthood (18–35) in this research.
A total of 52 early adulthood women were recruited. The diagnosis of MDD was made by professional psychiatrists referring to the Diagnostic and Statistical Manual of Mental Disorders–Fourth Edition (DSM-V) criteria. The 17-item Hamilton Depression Scale (HAMD) (Zimmerman et al., 2013) was used to measure depression severity. The Childhood Trauma Questionnaire (CTQ) was employed to evaluate the negative impact of childhood trauma. The CTQ can be divided into 5 subscales, including emotional abuse (EA), emotional neglect (EN), sexual abuse (SA), physical abuse (PA), and physical neglect (PN). The cutoff points for the CTQ subscale are as follows: (i) EA ≥ 13, (ii) EN ≥ 15, (iii) SA ≥ 8, (iv) PA ≥ 10, and (v) PN ≥ 10 (Xie et al., 2018; Georgieva et al., 2021). Childhood trauma history was considered to exist in participants scoring over the subscale threshold (moderate–severe). The HAMD and CTQ were only used for the assessment of patients with MDD.
According to the above criterion, participants were divided into MDD with the childhood trauma group (n = 16), MDD without the childhood trauma group (n = 16), and the healthy control group (n = 20). Subjects with MDD were recruited from the outpatient clinics of the Affiliated Brain Hospital of Guangzhou Medical University. We recruited healthy participants from the local community with matching age and education levels. In this study, the exclusion criteria are as follows: (i) any other physical and mental illness except for MDD; (ii) history of seizures, head trauma, or unconsciousness; (iii) received electroconvulsive therapy within the past 6 months, recently taken contraceptives, and taken psychiatric drugs before; (iv) substance dependence; (v) pregnant, lactating, or menstruating women; and (vi) any contraindications to magnetic resonance imaging (MRI). All participants were fully informed and written informed consent was obtained before enrollment. This study was approved by the Ethics Committee of the Affiliated Brain Hospital of Guangzhou Medical University.
Magnetic resonance imaging data acquisition
The MRI data were obtained on a 3.0T MRI system (Philips, Best, The Netherlands) in the Affiliated Brain Hospital of Guangzhou Medical University. Tampons were used to reduce noise, while foam pads were used to restrain head movement. During the scan, subjects were asked to remain still and close their eyes, but not fall asleep and think. The parameters of the echo plane imaging (EPI) sequence were as follows: repetition time (TR) = 2,000 ms, echo time (ET) = 30 ms, flip angle = 90°, field of view (FOV) = 220 × 220 mm2, slices = 33, thickness = 4 mm, inter-slice gap = 0.6 mm, and matrix = 64 × 64. Meanwhile, the parameters of the T1-weighted sagittal images were as follows: TR = 8.2 ms, ET = 3.7 ms, flip angle = 7°, thickness = 1 mm, and matrix = 256 × 256. To strictly control the effect of head movement, we excluded one subject whose head translation was greater than 1.5 mm.
Magnetic resonance imaging data preprocessing
The fMRI data were conducted by a Data Processing Assistant for Resting-State fMRI Advanced Edition V4.5 (DPARSFA)1 (Yan et al., 2016). In addition to the first 10 volumes being removed, all the images were corrected for temporal differences and head motion. We excluded the participants whose image translation movement was more than 1.5 mm or rotational movement was more than 1.5°. The T1-weighted image was co-registered with the average functional image after motion correction. Then, the images were normalized to the Montreal Neurological Institute template and resampled to a spatial resolution of 3 × 3 × 3 mm3. Subsequently, the functional images were smoothed with a Gaussian kernel (full-width half-maximum = 4 mm). In addition, in order to remove the effects of nuisance covariates, we regressed headmotor parameters, white matter signals, and CSF signals. Finally, the time series for each voxel was subjected to linear trend reduction and temporal filtering (0.01–0.08 Hz) to reduce low-frequency drift and high-frequency noise (Biswal et al., 1995; Lowe et al., 1998).
Analysis of amplitude of low-frequency fluctuation
We applied DPASF4.5 to compute ALFF and FC. Briefly, we converted the frequency domain power spectrum of the whole-brain signal with the fast Fourier transform. In addition, based on the power spectrum between 0.01 and 0.08 Hz, we calculated the ALFF. Finally, to minimize variability in general whole-brain ALFF levels between participants, the ALFF value was standardized to the Z-value (zALFF).
Analysis of functional connectivity
Subsequently, a seed-based interregional FC analysis was conducted. Seeds were chosen from brain regions correlated with childhood trauma in between-group ALFF discrepancy. FC analysis was calculated after a time series of the seed area average was extracted. The seed area and the rest of the brain were then correlated voxel-by-voxel. Finally, in order to enhance the normality of the correlation coefficient, we performed a Fisher’s r to z transformation.
Statistical analysis
Statistical analyses were calculated using Statistical Package for the Social Sciences, version 19.0 (SPSS, Inc., Chicago, United States). Group differences in demographic and clinical data were assessed by one-way analysis of covariance (ANCOVA) or two sample t-tests. In this study, significant differences were defined as p < 0.05.
To identify the significance of the brain district that had altered ALFF and FC values, a voxel-based ANCOVA was conducted using education and age as covariates. The significance was set with a cluster-level corrected threshold of p < 0.05 (cluster-forming threshold at voxel level p < 0.001 using the AlphaSim method) (Forman et al., 1995; Poline et al., 1997). Then, to examine group differences in mean ALFF and FC values, two sample t-tests were conducted on three groups identified after ANCOVA. Multiple comparison correction was employed with the Bonferroni method, and the significance level was determined at p < 0.05/2 = 0.025 (voxel-wise concordance analysis resulting in two significant clusters). In addition, partial correlation analyses were performed to discover the contact of voxel-wise concordance with CTO score in all subjects with MDD [p < 0.05/12 = 0.0041 with Bonferroni correction of 12 being due to two clusters and 6 scales (i.e., CTO scale and its 5 subscales)]. Finally, we further explored the association of brain dysfunction with childhood trauma in female patients with MDD by multiple linear regression with CTQ total scores and the characteristic values of brain region (ALFF and FC). Age and education were considered control variables in partial correlation and multiple linear regression.
Results
Demographic and clinical measures
As shown in Table 1, no significant difference was found in age and education level among the three groups (all p < 0.05). Significant differences were detected in CTQ total score and its subscale scores (e.g., EA, EN, SA, and PN) between MDD with the childhood trauma group and MDD without the childhood trauma group.
Assessed by one-way analysis of covariance plus post hoc comparisons of amplitude of low-frequency fluctuation
Significant ALFF alterations were found in the left middle frontal gyrus (MFG) (57 voxels) and the right postcentral gyrus (PoCG) (63 voxels) between the three groups (Figure 1 and Table 2). MDD with the childhood trauma group revealed increased ALFF values in the left MFG compared to MDD without the childhood trauma group and the HC group (Figure 2 and Table 3). Moreover, decreased ALFF was detected in the right PoCG in MMD patient groups relative to the HC group. Nonetheless, no significant ALFF difference was discovered among MDD with and without childhood trauma groups (Figure 2 and Table 3).
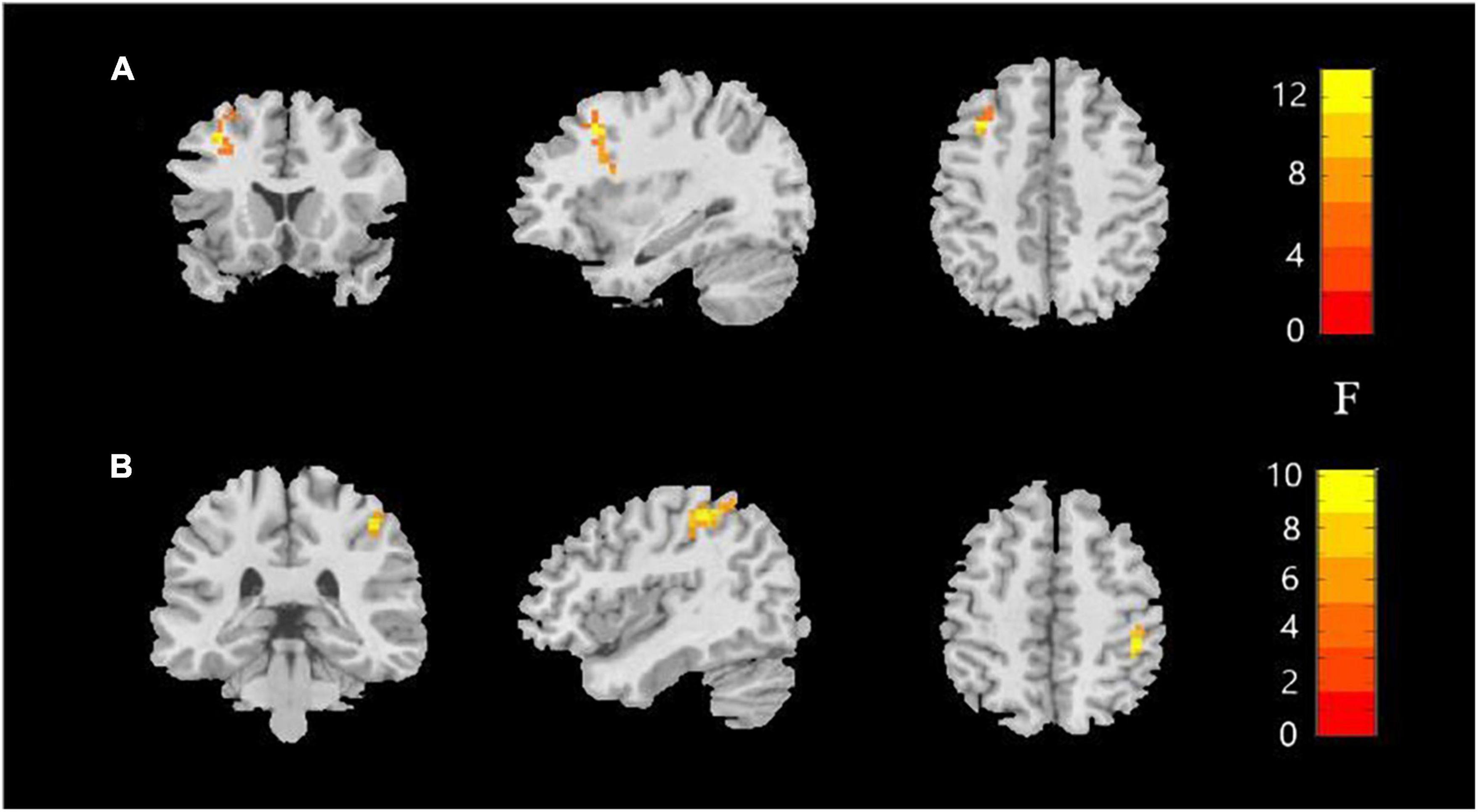
Figure 1. Amplitude of low-frequency fluctuations (ALFF) value among the MDD with childhood trauma, MDD without childhood trauma, and HC groups. One-way ANCOVA with age and education as covariates was performed to compare ALFF maps in the experimental groups. Left middle frontal gyrus (A) and right postcentral gyrus (B) showed the most significant differences according to ALFF analysis (AlphaSim-corrected p < 0.05).

Table 2. Group differences in amplitude of low-frequency fluctuations in MDD with childhood trauma, MDD without childhood trauma and HC.
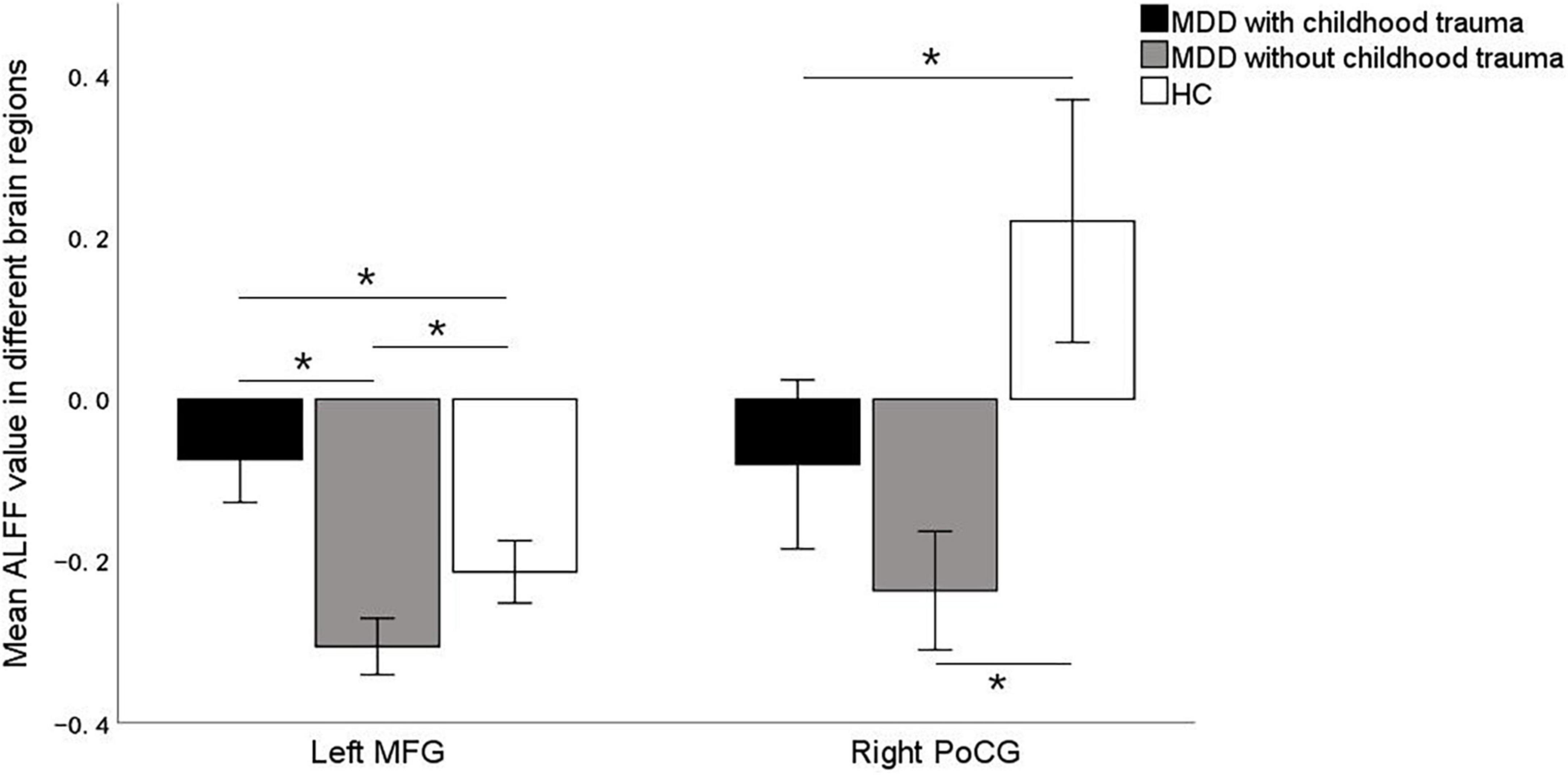
Figure 2. Post hoc two samplet—tests were used to determine the between groups differences in ALFF value in left middle frontal gyrus and right postcentral gyrus showing significant differences in ALFF maps in the previous ANOVA. *Bonferroni correction with P < 0.025 was set for significance. MFG, middle frontal gyrus; PoCG, postcentral gyrus.
Functional connectivity analyses
In this study, the brain areas that showed group differences in the ALFF analysis were selected as seed (i.e., left MFG) in the FC analysis. Significant FC difference in the left MFG–bilateral putamen was observed between groups (Figure 3 and Table 4). Compared to the HC group, MDD groups showed increased FC between left MFG and bilateral putamen (Figure 4 and Table 5). However, no observable discrepancy was found between MDD with and without childhood trauma (Figure 4 and Table 5).
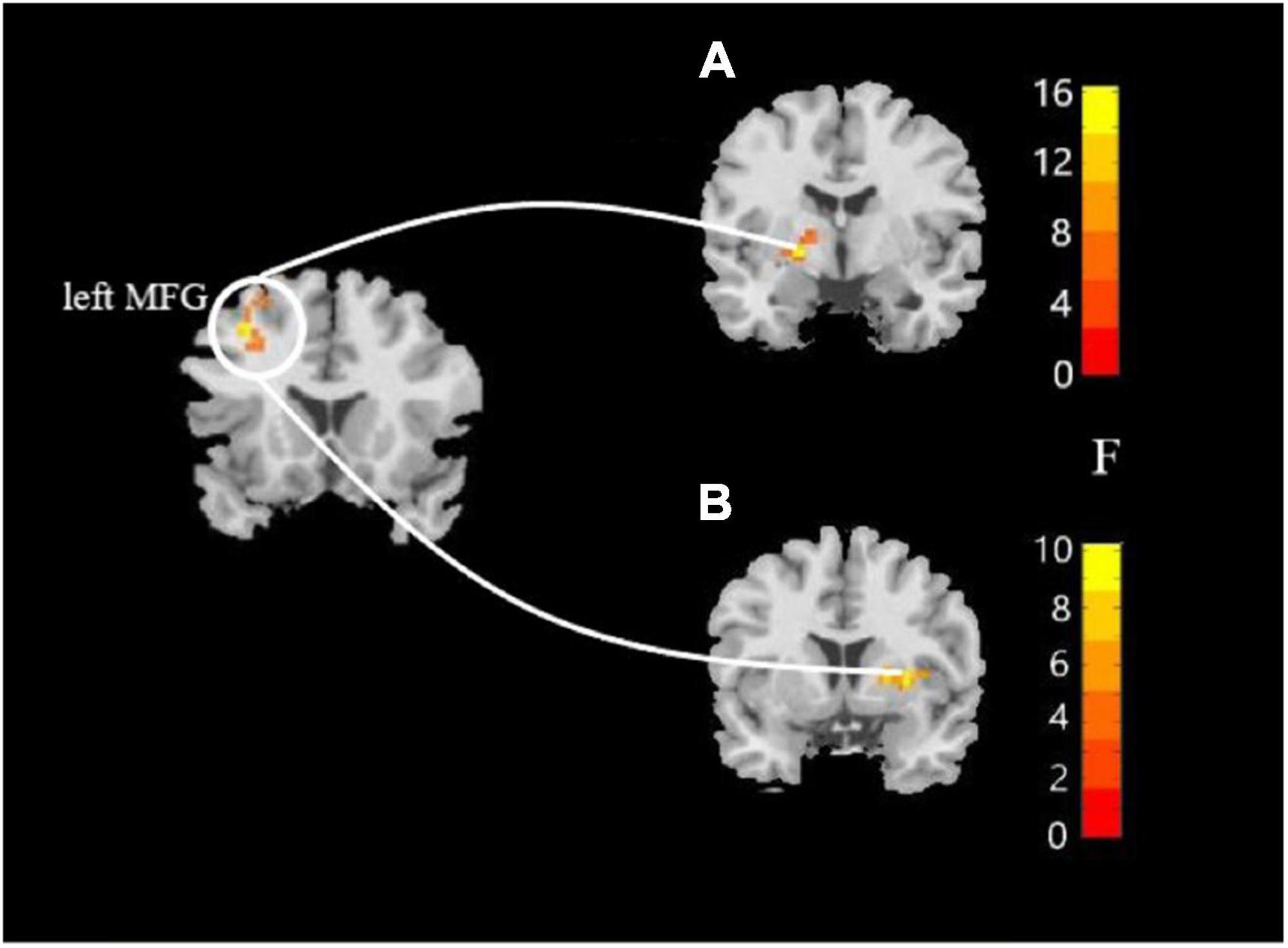
Figure 3. Resting-state functional connectivity analyses among MDD with childhood trauma, MDD without childhood trauma, and HC groups. One-way ANCOVA with age and as covariates was performed to compare functional connectivity maps in all the three groups and identified significant differences between left middle frontal gyrus and bilateral putamen (A, left putamen gyrus; B, right putamen gyrus) (AlphaSim-corrected p < 0.05).

Table 4. FC differences between left middle frontal gyrus seed and left putamen gyrus and right putamen gyrus in MDD with childhood trauma, MDD without childhood trauma, and HC.
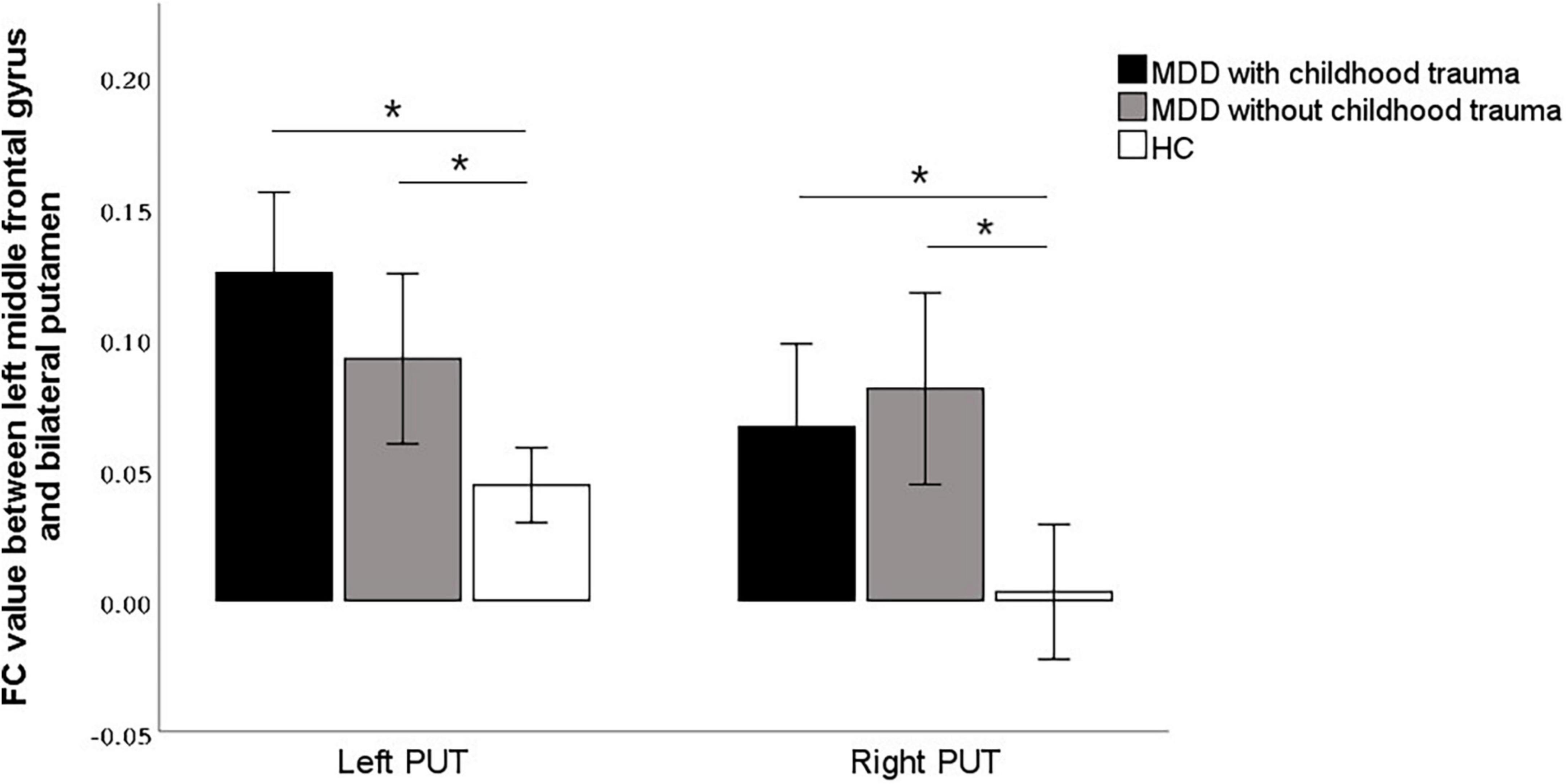
Figure 4. Post hoc analyses revealed significant increased functional connectivity of left PUT to left middle frontal gyrus in MDD with childhood trauma compared to both MDD without childhood trauma and HC group. The functional connectivity of right PUT to left middle frontal gyrus in the MMD patient groups were significantly higher than that in the HC group, but there was no significant difference between those with and without childhood trauma. *Bonferroni correction with P < 0.025 was set for significance.
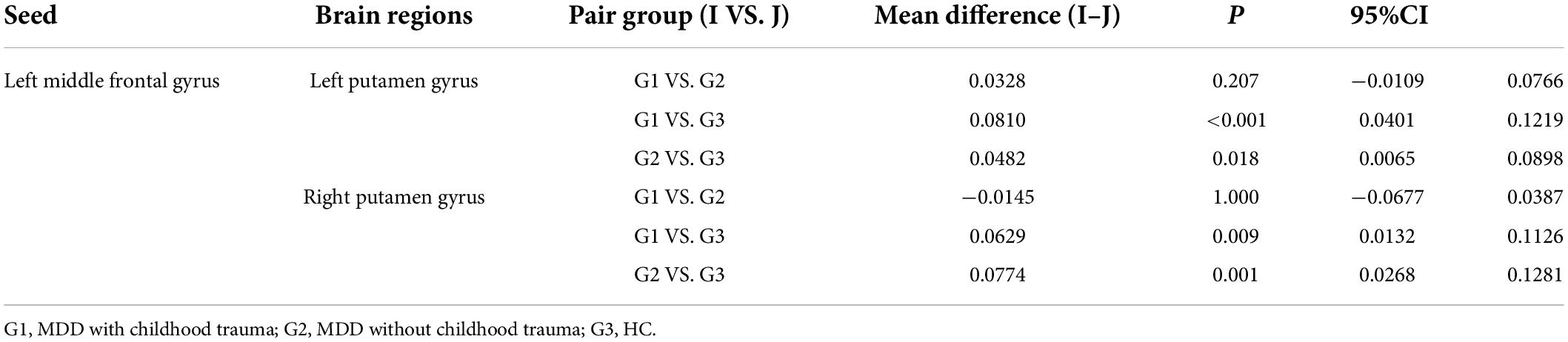
Table 5. Multiple comparisons of functional connectivity between left middle frontal gyrus seed and left putamen gyrus and right putamen gyrus.
Partial correlation analyses and multiple linear regressions analyses
Partial correlation analysis confirmed the positive correlation between ALFF in the left MFG and EN scores (r = 0.465 p = 0.001) and CTQ total score (r = 0.458, p = 0.001), respectively (Figure 5 and Table 6). We did not find a significant correlation between FC and CTQ total scores or its subscale scores. Regression analyses further showed the correlation of ALFF value in the left MFG on childhood trauma (F = 2.476, p < 0.05) (Table 7).
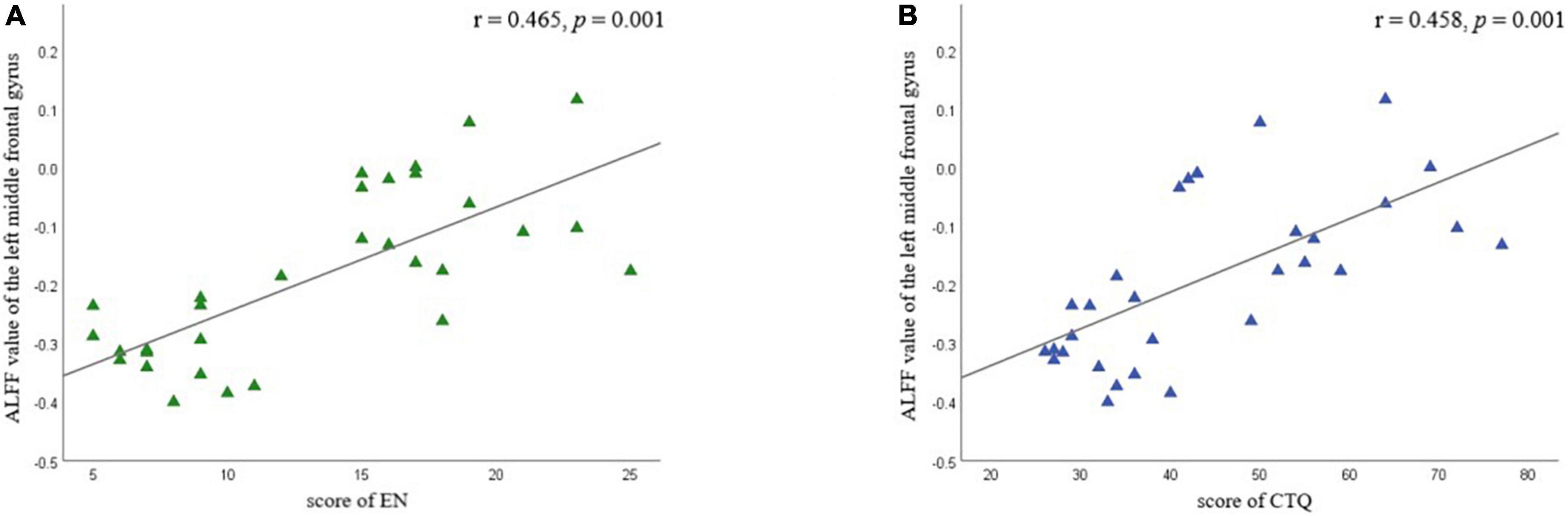
Figure 5. Partial correlation analyses between amplitude of low-frequency fluctuation value (ALFF) in left middle frontal gyrus and childhood trauma scores in different subscale were performed by combining all of MDD participants as a whole. Age and education were considered as control variables. (A) Shows the correlation between EN scores and ALFF value of the left middle frontal gyrus; (B) shows the correlation between CTQ total scores and ALFF value of the left middle frontal gyrus. EA, emotional neglect.
Discussion
In this study, we focused on ALFF and FC alterations in female MDD patients with childhood trauma. There was increased ALFF in the left MFG and right PoCG in MDD with childhood trauma compared with MDD without childhood trauma. The brain regions with significant ALFF discrepancies were selected as seeds for the FC analyses. There was increased FC in the left MFG and bilateral putamen gyrus. Moreover, we confirmed an association between altered ALFF and childhood trauma history. Together, our findings indicate the presence of abnormal intrinsic brain activity and FC patterns in female MDD patients with childhood trauma. The results of our research also offer important insights into the neurobiological mechanisms of MDD and childhood trauma.
An interesting finding in this study was that, in the left MFG, ALFF was higher in the MDD group with childhood trauma than in the group without childhood trauma. In addition, ALFF was positively correlated with CTQ scores and EN. Previous studies have reported that left MFG activation is associated with working memory (Zhang et al., 2003), the processing of social information and social perception (Vollm et al., 2006), memory retrieval (Tulving et al., 1994), and emotion regulation (Ochsner and Gross, 2005; Bermpohl et al., 2006). This area is also associated with rumination (Wang et al., 2018), which in turn increases an individual’s risk of MDD (Abela and Hankin, 2011). For example, Shors et al. (2017) reported that interventions targeting rumination generally reduce MDD incidence in women. Furthermore, O’Mahen et al. (2015) revealed that EN and abuse in childhood are associated with depression, with rumination partially mediating this effect. To some extent, our findings support this conjecture. Local brain activity may be affected by previous childhood trauma, especially EN, which in turn affects cognitive processing patterns such as rumination. Notably, Tulving et al. (1994) reported that the left MFG is related to memory retrieval. Thus, abnormal left MFG activation in individuals with childhood trauma may repeatedly trigger traumatic memories and exacerbate rumination. This may be the underlying cause of heightened depressive symptoms in MDD patients with childhood trauma. Overall, our results suggest that abnormal left MFG function might indicate the impact of childhood trauma in young adult women with MDD.
We also revealed that patients with MDD were at a higher risk for dysfunction in the right PoCG; however, there were no significant differences between patients with and without childhood trauma. Numerous studies have noted that the PoCG is mainly involved in the processing of some sensory information (Phillips et al., 2003), cognitive activities (Wager and Smith, 2003), and emotional processing (Luo et al., 2022). Tadayonnejad et al. (2015) reported that regional properties of neural activity in the PoCG are associated with depression severity. Moreover, neuroimaging studies have demonstrated structural and functional changes in the precentral and postcentral gyri of patients with MDD (Guo et al., 2011; Wang et al., 2012). Abnormal brain function in the PoCG may thus be a unique neurobiological feature of MDD; our results support this idea. Together, these findings provide theoretical support for further research into the relationship between the PoCG and MDD.
In this study, we investigated FC patterns in female MDD patients with childhood trauma. We measured the FC of each cluster vs. the rest of the brain using altered ALFF clusters with clinical correlations as the ROIs. The FC between the left MFG and bilateral putamen was observably increased in the MDD group compared with the HC group. The putamen is associated with motor control and learning (Luo et al., 2020), is one of the core regions for emotion production and processing (Wager et al., 2003), and plays an important role in cognitive and executive functions (Peters et al., 2016). Su et al. (2018) reported that a decrease in glucose metabolism in the putamen of patients with MDD impaired FC to key centers, such as the inferior and middle frontal gyri. Although the results of our study differed from those of predecessors, the discrepancies may be caused by differences in sample size, research subjects, or other reasons. Abnormal connectivity between the MFG and the putamen appears to be an important characteristic of MDD. However, our study revealed that the FC between the left MFG and bilateral putamen had no observable discrepancy in the MDD with the childhood trauma group compared with the MDD without the childhood trauma group. Jeong et al. (2021) found that trauma exposure may be related to structural alterations in the MFG and putamen. Thus, trauma exposure may also be an important factor underlying structural abnormalities of the MFG and putamen, but we need further research to find out if there are also functional abnormalities in this brain region. Furthermore, both trauma exposure and MDD appear to be associated with these two cognitively related regions. Thus, perhaps the main crux of depression with childhood trauma is changing in cognition; this may have a certain guiding significance for the clinical treatment of MDD and will be a major direction of our future research. Collectively, our findings provide an important base for investigating the neuropathological mechanisms of MDD as well as those of childhood trauma.
Our study has certain limitations. First, it remains unclear whether self-reported trauma history reflects authentic experiences during childhood and early adolescence. To minimize information bias, we, therefore, conducted in-depth interviews to confirm adverse childhood experiences. In addition, the study age was set at early adulthood (18–35 years of age) to minimize any differences in the duration of childhood trauma. However, this study did not further subdivide the types of childhood trauma. This is also the direction of future research to further analyze the effects of childhood trauma on the brain in terms of different dimensions, intensities, and durations, for example. Second, this study used a cross-sectional approach with small sample size and lacked any comparisons with the male population. The current results should, therefore, be interpreted with caution. Future studies will expand the sample size to further validate the results and add a male control group to investigate whether the identified brain regions are unique to women.
Conclusion
Overall, after controlling age-related confounding factors as much as possible, our study found that left MFG abnormality and left MFG–putamen dysfunction may be unique neural mechanisms in female MDD patients with childhood trauma. Our findings provide a basis for future research into the relationship between childhood trauma and MDD.
Data availability statement
The raw data supporting the conclusions of this article will be made available by the authors, without undue reservation.
Ethics statement
The studies involving human participants were reviewed and approved by The Affiliated Brain Hospital of Guangzhou Medical University. The patients/participants provided their written informed consent to participate in this study.
Author contributions
HP, HW, and JC designed the experiments. YL, ZW, XL, JY, HY, HN, and YD performed the clinical data collection and assessment. JC and QL performed the neuroimaging data analysis and wrote the draft. All authors discussed the results and reviewed the manuscript.
Funding
The Guangdong Natural Science Foundation, China (2015A030313800 to HP), supported the design of the study. The Guangzhou Municipal Key Discipline in Medicine for Guangzhou Brain Hospital (GBH2014-ZD04 to HP) supported the data collection for the study.
Conflict of interest
The authors declare that the research was conducted in the absence of any commercial or financial relationships that could be construed as a potential conflict of interest.
Publisher’s note
All claims expressed in this article are solely those of the authors and do not necessarily represent those of their affiliated organizations, or those of the publisher, the editors and the reviewers. Any product that may be evaluated in this article, or claim that may be made by its manufacturer, is not guaranteed or endorsed by the publisher.
Footnotes
References
Abela, J. R., and Hankin, B. L. (2011). Rumination as a vulnerability factor to depression during the transition from early to middle adolescence: A multiwave longitudinal study. J. Abnorm. Psychol. 120, 259–271. doi: 10.1037/a0022796
Andersen, S. L. (2022). Neuroinflammation, Early-Life Adversity, and Brain Development. Harv. Rev. Psychiatry 30, 24–39. doi: 10.1097/HRP.0000000000000325
Baldwin, J. R., Reuben, A., Newbury, J. B., and Danese, A. (2019). Agreement Between Prospective and Retrospective Measures of Childhood Maltreatment: A Systematic Review and Meta-analysis. JAMA Psychiatry 76, 584–593. doi: 10.1001/jamapsychiatry.2019.0097
Bermpohl, F., Pascual-Leone, A., Amedi, A., Merabet, L. B., Fregni, F., Gaab, N., et al. (2006). Attentional modulation of emotional stimulus processing: An fMRI study using emotional expectancy. Hum. Brain Mapp. 27, 662–677. doi: 10.1002/hbm.20209
Bernstein, D. P., Stein, J. A., Newcomb, M. D., Walker, E., Pogge, D., Ahluvalia, T., et al. (2003). Development and validation of a brief screening version of the Childhood Trauma Questionnaire. Child Abuse Negl. 27, 169–190. doi: 10.1016/s0145-2134(02)00541-0
Biswal, B., Yetkin, F. Z., Haughton, V. M., and Hyde, J. S. (1995). Functional connectivity in the motor cortex of resting human brain using echo-planar MRI. Magn. Reson. Med. 34, 537–541. doi: 10.1002/mrm.1910340409
Du, L., Wang, J., Meng, B., Yong, N., Yang, X., Huang, Q., et al. (2016). Early life stress affects limited regional brain activity in depression. Sci. Rep. 6:25338. doi: 10.1038/srep25338
Ebneabbasi, A., Mahdipour, M., Nejati, V., Li, M., Liebe, T., Colic, L., et al. (2021). Emotion processing and regulation in major depressive disorder: A 7T resting-state fMRI study. Hum. Brain Mapp. 42, 797–810. doi: 10.1002/hbm.25263
Ferrari, A. J., Charlson, F. J., Norman, R. E., Patten, S. B., Freedman, G., Murray, C. J., et al. (2013). Burden of depressive disorders by country, sex, age, and year: Findings from the global burden of disease study 2010. PLoS Med. 10:e1001547. doi: 10.1371/journal.pmed.1001547
Forman, S. D., Cohen, J. D., Fitzgerald, M., Eddy, W. F., Mintun, M. A., and Noll, D. C. (1995). Improved assessment of significant activation in functional magnetic resonance imaging (fMRI): Use of a cluster-size threshold. Magn. Reson. Med. 33, 636–647. doi: 10.1002/mrm.1910330508
Georgieva, S., Tomas, J. M., and Navarro-Perez, J. J. (2021). Systematic review and critical appraisal of Childhood Trauma Questionnaire - Short Form (CTQ-SF). Child Abuse Negl. 120:105223. doi: 10.1016/j.chiabu.2021.105223
Guo, W. B., Liu, F., Xue, Z. M., Yu, Y., Ma, C. Q., Tan, C. L., et al. (2011). Abnormal neural activities in first-episode, treatment-naive, short-illness-duration, and treatment-response patients with major depressive disorder: A resting-state fMRI study. J. Affect. Disord. 135, 326–331. doi: 10.1016/j.jad.2011.06.048
Haahr-Pedersen, I., Perera, C., Hyland, P., Vallieres, F., Murphy, D., Hansen, M., et al. (2020). Females have more complex patterns of childhood adversity: Implications for mental, social, and emotional outcomes in adulthood. Eur. J. Psychotraumatol. 11:1708618. doi: 10.1080/20008198.2019.1708618
Hailes, H. P., Yu, R., Danese, A., and Fazel, S. (2019). Long-term outcomes of childhood sexual abuse: An umbrella review. Lancet Psychiatry 6, 830–839. doi: 10.1016/S2215-0366(19)30286-X
Hamlat, E. J., Prather, A. A., Horvath, S., Belsky, J., and Epel, E. S. (2021). Early life adversity, pubertal timing, and epigenetic age acceleration in adulthood. Dev. Psychobiol. 63, 890–902. doi: 10.1002/dev.22085
Hu, L., Xiao, M., Ai, M., Wang, W., Chen, J., Tan, Z., et al. (2019). Disruption of resting-state functional connectivity of right posterior insula in adolescents and young adults with major depressive disorder. J. Affect. Disord. 257, 23–30. doi: 10.1016/j.jad.2019.06.057
Jeong, H. J., Durham, E. L., Moore, T. M., Dupont, R. M., McDowell, M., Cardenas-Iniguez, C., et al. (2021). The association between latent trauma and brain structure in children. Transl. Psychiatry 11:240. doi: 10.1038/s41398-021-01357-z
Kessler, R. C., Angermeyer, M., Anthony, J. C., De Graaf, R., Demyttenaere, K., Gasquet, I., et al. (2007). Lifetime prevalence and age-of-onset distributions of mental disorders in the World Health Organization’s World Mental Health Survey Initiative. World Psychiatry 6, 168–176.
Kuehner, C. (2017). Why is depression more common among women than among men? Lancet Psychiatry 4, 146–158. doi: 10.1016/S2215-0366(16)30263-2
Lowe, M. J., Mock, B. J., and Sorenson, J. A. (1998). Functional connectivity in single and multislice echoplanar imaging using resting-state fluctuations. Neuroimage 7, 119–132. doi: 10.1006/nimg.1997.0315
Luo, Q., Chen, J., Li, Y., Wu, Z., Lin, X., Yao, J., et al. (2022). Altered regional brain activity and functional connectivity patterns in major depressive disorder: A function of childhood trauma or diagnosis? J. Psychiatry Res. 147, 237–247. doi: 10.1016/j.jpsychires.2022.01.038
Luo, X., Guo, X., Tan, Y., Zhang, Y., Garcia-Milian, R., Wang, Z., et al. (2020). KTN1 variants and risk for attention deficit hyperactivity disorder. Am. J. Med. Genet. B Neuropsychiatr. Genet. 183, 234–244. doi: 10.1002/ajmg.b.32782
Ma, M., Zhang, X., Zhang, Y., Su, Y., Yan, H., Tan, H., et al. (2021). Childhood Maltreatment Was Correlated With the Decreased Cortical Function in Depressed Patients Under Social Stress in a Working Memory Task: A Pilot Study. Front. Psychiatry 12:671574. doi: 10.3389/fpsyt.2021.671574
McKay, M. T., Cannon, M., Chambers, D., Conroy, R. M., Coughlan, H., Dodd, P., et al. (2021). Childhood trauma and adult mental disorder: A systematic review and meta-analysis of longitudinal cohort studies. Acta Psychiatr. Scand. 143, 189–205. doi: 10.1111/acps.13268
Murray, C. J., Vos, T., Lozano, R., Naghavi, M., Flaxman, A. D., Michaud, C., et al. (2012). Disability-adjusted life years (DALYs) for 291 diseases and injuries in 21 regions, 1990-2010: A systematic analysis for the Global Burden of Disease Study 2010. Lancet 380, 2197–2223. doi: 10.1016/S0140-6736(12)61689-4
Noble, S., Scheinost, D., and Constable, R. T. (2019). A decade of test-retest reliability of functional connectivity: A systematic review and meta-analysis. Neuroimage 203:116157. doi: 10.1016/j.neuroimage.2019.116157
Ochsner, K. N., and Gross, J. J. (2005). The cognitive control of emotion. Trends Cogn. Sci. 9, 242–249. doi: 10.1016/j.tics.2005.03.010
O’Mahen, H. A., Karl, A., Moberly, N., and Fedock, G. (2015). The association between childhood maltreatment and emotion regulation: Two different mechanisms contributing to depression? J. Affect. Disord. 174, 287–295. doi: 10.1016/j.jad.2014.11.028
Otte, C., Gold, S. M., Penninx, B. W., Pariante, C. M., Etkin, A., Fava, M., et al. (2016). Major depressive disorder. Nat. Rev. Dis. Primers 2:16065. doi: 10.1038/nrdp.2016.65
Peters, S. K., Dunlop, K., and Downar, J. (2016). Cortico-Striatal-Thalamic Loop Circuits of the Salience Network: A Central Pathway in Psychiatric Disease and Treatment. Front. Syst. Neurosci. 10:104. doi: 10.3389/fnsys.2016.00104
Phillips, M. L., Drevets, W. C., Rauch, S. L., and Lane, R. (2003). Neurobiology of emotion perception II: Implications for major psychiatric disorders. Biol. Psychiatry 54, 515–528. doi: 10.1016/s0006-3223(03)00171-9
Poline, J. B., Worsley, K. J., Evans, A. C., and Friston, K. J. (1997). Combining spatial extent and peak intensity to test for activations in functional imaging. Neuroimage 5, 83–96. doi: 10.1006/nimg.1996.0248
Seedat, S., Scott, K. M., Angermeyer, M. C., Berglund, P., Bromet, E. J., Brugha, T. S., et al. (2009). Cross-national associations between gender and mental disorders in the World Health Organization World Mental Health Surveys. Arch Gen. Psychiatry 66, 785–795. doi: 10.1001/archgenpsychiatry.2009.36
Shors, T. J., Millon, E. M., Chang, H. Y., Olson, R. L., and Alderman, B. L. (2017). Do sex differences in rumination explain sex differences in depression? J. Neurosci. Res. 95, 711–718. doi: 10.1002/jnr.23976
Silva, R. C., Maffioletti, E., Gennarelli, M., Baune, B. T., and Minelli, A. (2021). Biological correlates of early life stressful events in major depressive disorder. Psychoneuroendocrinology 125:105103. doi: 10.1016/j.psyneuen.2020.105103
Sonu, S., Post, S., and Feinglass, J. (2019). Adverse childhood experiences and the onset of chronic disease in young adulthood. Prev. Med. 123, 163–170. doi: 10.1016/j.ypmed.2019.03.032
Su, H., Zuo, C., Zhang, H., Jiao, F., Zhang, B., Tang, W., et al. (2018). Regional cerebral metabolism alterations affect resting-state functional connectivity in major depressive disorder. Quant. Imaging Med. Surg. 8, 910–924. doi: 10.21037/qims.2018.10.05
Tadayonnejad, R., Yang, S., Kumar, A., and Ajilore, O. (2015). Clinical, cognitive, and functional connectivity correlations of resting-state intrinsic brain activity alterations in unmedicated depression. J. Affect. Disord. 172, 241–250. doi: 10.1016/j.jad.2014.10.017
Tan, X., Zhang, L., Wang, D., Guan, S., Lu, P., Xu, X., et al. (2021). Influence of early life stress on depression: From the perspective of neuroendocrine to the participation of gut microbiota. Aging 13, 25588–25601. doi: 10.18632/aging.203746
Tozzi, L., Garczarek, L., Janowitz, D., Stein, D. J., Wittfeld, K., Dobrowolny, H., et al. (2020). Interactive impact of childhood maltreatment, depression, and age on cortical brain structure: Mega-analytic findings from a large multi-site cohort. Psychol. Med. 50, 1020–1031. doi: 10.1017/S003329171900093X
Tulving, E., Kapur, S., Markowitsch, H. J., Craik, F. I., Habib, R., and Houle, S. (1994). Neuroanatomical correlates of retrieval in episodic memory: Auditory sentence recognition. Proc. Natl. Acad. Sci. U.S.A. 91, 2012–2015. doi: 10.1073/pnas.91.6.2012
Vollm, B. A., Taylor, A. N., Richardson, P., Corcoran, R., Stirling, J., McKie, S., et al. (2006). Neuronal correlates of theory of mind and empathy: A functional magnetic resonance imaging study in a nonverbal task. Neuroimage 29, 90–98. doi: 10.1016/j.neuroimage.2005.07.022
Wager, T. D., and Smith, E. E. (2003). Neuroimaging studies of working memory: A meta-analysis. Cogn. Affect. Behav. Neurosci. 3, 255–274. doi: 10.3758/cabn.3.4.255
Wager, T. D., Phan, K. L., Liberzon, I., and Taylor, S. F. (2003). Valence, gender, and lateralization of functional brain anatomy in emotion: A meta-analysis of findings from neuroimaging. Neuroimage 19, 513–531. doi: 10.1016/s1053-8119(03)00078-8
Wang, L., Dai, W., Su, Y., Wang, G., Tan, Y., Jin, Z., et al. (2012). Amplitude of low-frequency oscillations in first-episode, treatment-naive patients with major depressive disorder: A resting-state functional MRI study. PLoS One 7:e48658. doi: 10.1371/journal.pone.0048658
Wang, Y., Zhu, W., Xiao, M., Zhang, Q., Zhao, Y., Zhang, H., et al. (2018). Hostile Attribution Bias Mediates the Relationship Between Structural Variations in the Left Middle Frontal Gyrus and Trait Angry Rumination. Front. Psychol. 9:526. doi: 10.3389/fpsyg.2018.00526
Whitaker, R. C., Dearth-Wesley, T., Herman, A. N., Block, A. E., Holderness, M. H., Waring, N. A., et al. (2021). The interaction of adverse childhood experiences and gender as risk factors for depression and anxiety disorders in US adults: A cross-sectional study. BMC Public Health 21:2078. doi: 10.1186/s12889-021-12058-z
Wu, Z., Luo, Q., Wu, H., Wu, Z., Zheng, Y., Yang, Y., et al. (2020). Amplitude of Low-Frequency Oscillations in Major Depressive Disorder With Childhood Trauma. Front. Psychiatry 11:596337. doi: 10.3389/fpsyt.2020.596337
Xie, P., Wu, K., Zheng, Y., Guo, Y., Yang, Y., He, J., et al. (2018). Prevalence of childhood trauma and correlations between childhood trauma, suicidal ideation, and social support in patients with depression, bipolar disorder, and schizophrenia in southern China. J. Affect. Disord. 228, 41–48. doi: 10.1016/j.jad.2017.11.011
Yan, C. G., Wang, X. D., Zuo, X. N., and Zang, Y. F. (2016). DPABI: Data Processing & Analysis for (Resting-State) Brain Imaging. Neuroinformatics 14, 339–351. doi: 10.1007/s12021-016-9299-4
Yan, C., Liu, D., He, Y., Zou, Q., Zhu, C., Zuo, X., et al. (2009). Spontaneous brain activity in the default mode network is sensitive to different resting-state conditions with limited cognitive load. PLoS One 4:e5743. doi: 10.1371/journal.pone.0005743
Yan, R., Huang, Y., Shi, J., Zou, H., Wang, X., Xia, Y., et al. (2022). Alterations of regional spontaneous neuronal activity and corresponding brain circuits related to non-suicidal self-injury in young adults with major depressive disorder. J. Affect. Disord. 305, 8–18. doi: 10.1016/j.jad.2022.02.040
Yang, H., Long, X. Y., Yang, Y., Yan, H., Zhu, C. Z., Zhou, X. P., et al. (2007). Amplitude of low frequency fluctuation within visual areas revealed by resting-state functional MRI. Neuroimage 36, 144–152.
Yu, M., Linn, K. A., Shinohara, R. T., Oathes, D. J., Cook, P. A., Duprat, R., et al. (2019). Childhood trauma history is linked to abnormal brain connectivity in major depression. Proc. Natl. Acad. Sci. U.S.A. 116, 8582–8590. doi: 10.1073/pnas.1900801116
Zhang, J. X., Leung, H. C., and Johnson, M. K. (2003). Frontal activations associated with accessing and evaluating information in working memory: An fMRI study. Neuroimage 20, 1531–1539. doi: 10.1016/j.neuroimage.2003.07.016
Keywords: childhood trauma, amplitude of low-frequency fluctuation, functional connectivity, middle frontal gyrus, postcentral gyrus, putamen
Citation: Chen J, Luo Q, Li Y, Wu Z, Lin X, Yao J, Yu H, Nie H, Du Y, Peng H and Wu H (2022) Intrinsic brain abnormalities in female major depressive disorder patients with childhood trauma: A resting-state functional magnetic resonance imaging study. Front. Neurosci. 16:930997. doi: 10.3389/fnins.2022.930997
Received: 28 April 2022; Accepted: 11 July 2022;
Published: 09 August 2022.
Edited by:
Zaixu Cui, Chinese Institute for Brain Research, Beijing (CIBR), ChinaReviewed by:
Xinyu Liang, Fudan University, ChinaSuyu Zhong, Beijing University of Posts and Telecommunications (BUPT), China
Copyright © 2022 Chen, Luo, Li, Wu, Lin, Yao, Yu, Nie, Du, Peng and Wu. This is an open-access article distributed under the terms of the Creative Commons Attribution License (CC BY). The use, distribution or reproduction in other forums is permitted, provided the original author(s) and the copyright owner(s) are credited and that the original publication in this journal is cited, in accordance with accepted academic practice. No use, distribution or reproduction is permitted which does not comply with these terms.
*Correspondence: Hongjun Peng, cGVuZ2RvY3RvcjJAMTYzLmNvbQ==; Huawang Wu, SHVhd2FuZ3d1QDEyNi5jb20=
†These authors have contributed equally to this work