- Center for the Study of Learning, Department of Pediatrics, Georgetown University Medical Center, Washington, DC, United States
Studies of reading intervention in dyslexia have shown changes in performance and in brain function. However, there is little consistency in the location of brain regions associated with successful reading gains in children, most likely due to variability/limitations in methodologies (study design, participant criteria, and neuroimaging procedures). Ultimately for the results to be meaningful, the intervention has to be successful, be assessed against a control, use rigorous statistics, and take biological variables (sex) into consideration. Using a randomized, crossover design, 31 children with dyslexia were assigned to a phonological- and orthographic-based tutoring period as well as a within-subjects control period to examine: (1) intervention-induced changes in behavior (reading performance) and in brain activity (during reading); and (2) behavioral and brain activity pre-intervention data that predicted intervention-induced gains in reading performance. We found gains in reading ability following the intervention, but not following the control period, with no effect of participants’ sex. However, there were no changes in brain activity following the intervention (regardless of sex), suggesting that individual brain changes are too variable to be captured at the group level. Reading gains were not predicted by pre-intervention behavioral data, but were predicted by pre-intervention brain activity in bilateral supramarginal/angular gyri. Notably, some of this prediction was only found in females. Our results highlight the limitations of brain imaging in detecting the neural correlates of reading intervention in this age group, while providing further evidence for its utility in assessing eventual success of intervention, especially if sex is taken into consideration.
Introduction
Developmental dyslexia is a common learning disability, affecting approximately between 5 and 13% of the general United States population (Katusic et al., 2001). It is defined by difficulties in word recognition and word decoding, that are incongruent with other cognitive skills, classroom experience, and motivation to learn how to read (Lyon et al., 2003). The word decoding problems (sounding out of novel words) are believed to be due to difficulties with understanding how sounds in speech are isolated, manipulated, and recalled (phonological coding or phonological awareness; Scarborough and Brady, 2002) and other difficulties with representing the speech stream (Peterson and Pennington, 2012), therefore impeding their mapping onto their corresponding graphemes during reading. Further, word form recognition is also impaired in dyslexia, not only as a consequence of poor phonological coding skills, but also because of difficulties in establishing a “sight word vocabulary” through memorization of the visual (orthographic) word forms (Badian, 1995, 2001). Brain imaging studies have revealed hypoactivation in brain regions associated with phonological mapping in temporo-parietal cortex (TPC) and in regions associated with visual word form recognition in the occipito-temporal cortex (OTC) (Pugh et al., 2001; Sandak et al., 2004; Eden et al., 2015). The role of these areas in reading acquisition and in dyslexia continue to be an active area of discussion (Richlan, 2012).
When it comes to addressing the reading difficulties of children with dyslexia, explicit instructions in phonological coding (Alexander and Slinger-Constant, 2004), letter-speech sound training (Brem et al., 2010; Kyle et al., 2013) and orthographic facilitation (Baron et al., 2018) are often key elements in the interventions administered. Ideally, such tutoring occurs in small groups (or one-on-one) with an emphasis on early intervention (Wanzek and Vaughn, 2007). Understanding the kinds of reading interventions that work and how they work, is of continued interest. Investigations into the neural correlates of reading intervention in dyslexia have shown widespread increases in brain activity in children (Aylward et al., 2003; Temple et al., 2003; Shaywitz et al., 2004; Richards et al., 2006; Meyler et al., 2008; Odegard et al., 2008; Gebauer et al., 2012) and adults (Eden et al., 2004). A narrative review by Barquero et al. (2014) describes these to include left and right hemisphere inferior, middle, and superior frontal gyri, superior and middle temporal gyri, occipital cortices, inferior parietal lobule, post central gyrus, and insulae (Barquero et al., 2014). Notably it has been suggested that gains in reading in dyslexia are associated with increases in left-hemisphere regions typically involved in reading, while other regions, such as right frontal cortex (Temple et al., 2003; Richards et al., 2006; Meyler et al., 2008), perhaps serve in a compensatory role (Shaywitz et al., 2004; Hoeft et al., 2007; Barquero et al., 2014). However, a meta-analysis of intervention neuroimaging research demonstrates a lack of convergence across studies for many of these implicated brain regions (Barquero et al., 2014). The strongest results from this meta-analysis of eight studies (173 participants) were left thalamus (three studies contributing), right insula/inferior frontal gyrus (four studies contributing), and left inferior frontal gyrus (three studies contributing). Right posterior cingulate and left middle occipital gyrus were also identified (though with only two contributing studies). Notably, other left-hemisphere regions typically involved in reading, that is left OTC and PTC, were not found to change. A more recent meta-analysis of changes in brain activation following reading intervention of eight studies (151 participants) and differing from the Barquero meta-analysis by two out of eight studies, found no results (Perdue et al., 2022).
There are also methodological limitations that question the validity of prior findings on changes in brain activity following reading intervention in dyslexia. As a whole, the imaging thresholds used are far less stringent than those used today. Of the studies in children and adolescents included in the Barquero and Perdue meta-analyses, only four used any correction of cluster size in their whole brain analysis (Aylward et al., 2003; Gebauer et al., 2012; Heim et al., 2015; Partanen et al., 2019), and voxel level thresholds vary considerably. Another concern is the variability in reported behavioral gains associated with the interventions. Not all studies report on changes in single word reading ability (though most report comprehension level data), and only two have examined whether these gains persist in the long-term (Shaywitz et al., 2004; Meyler et al., 2008). Importantly, while many studies of dyslexia include a control group, none include a within-subject control intervention to assess specificity of these changes, a design that is considered best practice in clinical research.
In addition to examining the brain bases for reading disability and successful reading intervention, neuroimaging has also been used in a small number of studies to examine whether brain function can be used to “predict” later reading outcome in typically reading children as well as children with dyslexia; and these studies have been done either with or without conducting a formal intervention. For example, in typically developing children both left visual word system (fusiform gyrus) event-related potentials and functional magnetic resonance imaging (fMRI) signal attained during a lexical decision task in kindergarten (prior to learning to read) were found to be predictive of how well children (who participated in a speech-sound association training) could read in second grade (Bach et al., 2013). Specific to dyslexia, a study in children and adolescents with dyslexia found that right inferior frontal gyrus activation during a written word rhyming task predicted single word reading measures 2.5 years later (no intervention was provided) (Hoeft et al., 2011). Two studies have examined reading intervention in children with dyslexia and tested whether gains in reading following the intervention were predicted by pre-intervention brain activity. One study found gains in untimed pseudoword reading were predicted by pre-intervention activity during a phonological processing task in left inferior frontal gyrus, and gains in timed word reading were predicted by activity in left and right inferior frontal gyri (Farris et al., 2016). Another study found gains in basic reading were predicted by pre-intervention functional connectivity between middle temporal gyrus and left inferior parietal lobule during a lexical decision task (Aboud et al., 2018). Like studies investigating changes in brain activity with intervention, the use of cluster-level correction for the whole-brain analysis is mixed, with only the last two of the above mentioned studies using cluster level correction (Farris et al., 2016; Aboud et al., 2018).
There have been recent calls to pay more attention to sex as a biological variable in all research (Cahill, 2006, 2012) and especially in research of language processing and dyslexia (Ramus et al., 2018; Krafnick and Evans, 2019). Sex has played a role in language research, where converging evidence suggest sex-specific differences in language acquisition and development (Martin and Hoover, 1987; Bornstein et al., 2000; Dionne et al., 2003), as well as sex-specific organization of the brain for language (Shaywitz et al., 1995; Jaeger et al., 1998; Kansaku and Kitazawa, 2001; Burman et al., 2008). For example, males have been shown to have more left-lateralized activation of perisylvian brain regions during language tasks, whereas females activate bilateral perisylvian brain regions (Shaywitz et al., 1995; Jaeger et al., 1998).
Specific to dyslexia, prevalence differs amongst boys and girls with odds ratios ranging from 1.39 to 3.19 in favor of higher prevalence in boys (Rutter et al., 2004; Quinn and Wagner, 2015), even when controlling for ascertainment bias (Liederman et al., 2005; Quinn and Wagner, 2015). Neuroimaging studies of dyslexia have on average recruited more male subjects, as reflected in 65% male participants contributing to the meta-analysis in children, and 95% to the meta-analysis in adults reported by Richlan et al. (2011), and 59% of subjects in a meta-analysis of neuroimaging studies of reading intervention (Barquero et al., 2014). Most importantly, evidence of sex-specific differences in dyslexia from studies of gray matter volume (Evans et al., 2014) and cortical thickness (Altarelli et al., 2013; Clark et al., 2014) suggest that the brain bases of dyslexia may not be the same in males and females, with females, but not males, showing differences in anatomy in brain regions associated with early sensory processing (Altarelli et al., 2013; Clark et al., 2014; Evans et al., 2014). However, sex has not been accounted for in investigations into the brain-based correlates of successful reading intervention in dyslexia. Sex-specific differences in dyslexia prior to an intervention could lead to sex-specific differences in the neural correlates of successful intervention. Critical to this study, failure to identify any antagonist interactions for sex could result in failure to register significant (sex-specific) changes following intervention. That is, if sex-specific changes are in opposite directions, the changes during intervention could appear small or non-existent. Lastly, if the behavioral response to reading intervention is the same for males and females, it does not mean that the neural substrates underlying that change in performance is the same for both sexes (Cahill, 2006).
In the present study we report behavioral data for reading and reading-related skills as well as fMRI data during a word processing task in 31 children with dyslexia. These data were acquired in all children prior to and following (i) an intensive intervention focused on promoting reading through phonological and orthographic skills, and (ii) an intensive intervention focused on promoting math (active control) or, instead, a null period (developmental control), using a randomized, crossover design. Our study of dyslexia allowed us to ask: (1) What are the brain activation changes that follow a successful reading intervention, and are these changes specific to the reading intervention? (2) Can brain activity during reading indicate whether children will subsequently reap benefits from the reading intervention? And (3) Are these findings affected by sex? Together these findings should advance our understanding of the location and specificity of the neural correlates that underlie successful reading intervention in males and females with dyslexia, as well as whether brain activity signals a readiness to benefit from such a reading intervention.
Materials and Methods
Participants
Thirty-one dyslexic children (14 female; age average 9.6 and range 7.4–12.6 years) were recruited from a private school specializing in students with learning disabilities. School records were used to identify children who had a score of less than or equal to 92 on the Woodcock–Johnson Test of Achievement III Letter-Word Identification (W-J WID) and/or Word Attack (W-J WA) (Woodcock et al., 2001), and a documented diagnosis of dyslexia. In order to be included in the study, children had to score at least 80 on Verbal, Performance, and Full IQ on the Wechsler Abbreviated Scale of Intelligence (WASI) (Wechsler, 1999). All children were in good health and free of other developmental disabilities, neurological and psychiatric disorders or any disease affecting brain function, except for ADHD (children taking medication for ADHD had to refrain taking it prior to the scans). Other exclusion criteria included contraindications to MRI scanning such as metallic implants or severe claustrophobia. fMRI data for some of these children using the same reading task have been published previously in a comparison with typically reading children (Olulade et al., 2015).
Behavioral Tests
All subjects received a battery of psychoeducational tests to evaluate intelligence quotient (IQ), reading, and skills that are related to reading. Except for IQ, the entire testing battery was administered at all three visits (prior to and following interventions). The WASI (Wechsler, 1999) was used to measure IQ. The Woodcock–Johnson Test of Achievement III was used to assess reading ability: Word Identification (W-J WID) subtest for single real word reading, Word Attack (W-J WA) subtest for single pseudoword reading, and Passage Comprehension (W-J PC) for understanding of written text (Woodcock et al., 2001). In addition, we measured skills that play a role in acquiring reading and are typically impaired in dyslexia: the Lindamood Auditory Conceptualization Test (LAC) for phonemic awareness (Lindamood and Lindamood, 1971), the Rapid Automatized Naming test (RAN L&N and C&O) for naming fluency of letters/numbers and colors/objects (Denckla and Rudel, 1976a,b), the Digit Span test for working memory (Wechsler, 1999), and the Symbol Imagery (SI) test for visual imagery (memory for letters and orthographic patterns) (Bell, 1997). These measures were used to gauge improvement in reading and reading-related skills, which were expected to increase following the reading intervention but not following the math intervention. To also assess changes in mathematical performance, we used the Calculation subtest for computational ability, the Math Fluency subtest for timed arithmetic, and the Applied Problems subtest of the Woodcock–Johnson Test of Achievement III (Woodcock et al., 2001) for mathematical word problems. All scores reported are standard scores (Population Mean = 100, SD = 15). Researchers acquiring these data were blind to each child’s group assignment.
Study Design
The children were randomly assigned to one of three groups. Each of the three groups received the same reading intervention (3 h a day, for a total of 90 h). For Group 1 (n = 10) this 6-week reading intervention was followed by a 6-week math intervention, and for Group 2 (n = 9) it was preceded by a math intervention (math intervention was also 3 h a day, for a total of 90 h). As such, these 19 children received the intervention of interest (reading) and an active control intervention (math), with the order counterbalanced (a 10th child originally assigned to Group 2 left the study after it had begun). Group 3 (n = 12) received the same reading intervention followed by a 6-week null period (no intervention) to provide a developmental control period (Krafnick et al., 2011). As such, we would be able to weigh any benefits resulting from the reading intervention against the possibility of a Hawthorn effect and/or a placebo effect (by comparison to the active control math intervention). The latter effects could result from participating in a study that involves intensive work on the part of the participants as well as strong encouragement by others for their efforts. Further, both the reading intervention and the active control math intervention could be assessed relative to no intervention (null period) to be able to assess changes relative to the normal developmental changes that would occur during this time span. Three behavioral testing/scanning sessions were scheduled eight weeks apart (one prior to any intervention/control period and another after each intervention/control period; see Figure 1). One-way ANOVAs showed that randomization to group was successful in keeping the groups similar in age, IQ, reading, and reading-related skills prior to intervention (Table 1). As such, age and IQ were not included in the analyses looking at gains in performance measures following the interventions. Further, a Chi-square test revealed no significant difference in sex amongst the groups (Table 1). Most subjects (26 of the original 31) returned for behavioral testing 1 year later, allowing us to gauge long-term outcome of the intervention.
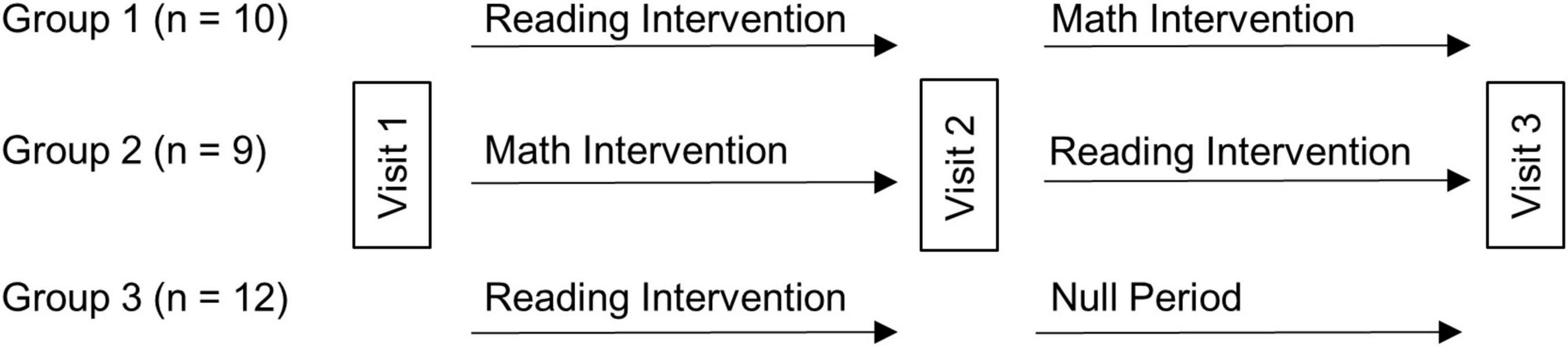
Figure 1. Intervention study design. Thirty-one children with dyslexia were randomly assigned to one of three intervention arms. All groups received the reading intervention, and either a math intervention (active control, Groups 1 and 2) or null period (developmental control, Group 3). Each visit consisted of acquisition of behavioral and imaging data, with 6-week intervention periods between Visits 1 and 2, and Visits 2 and 3.
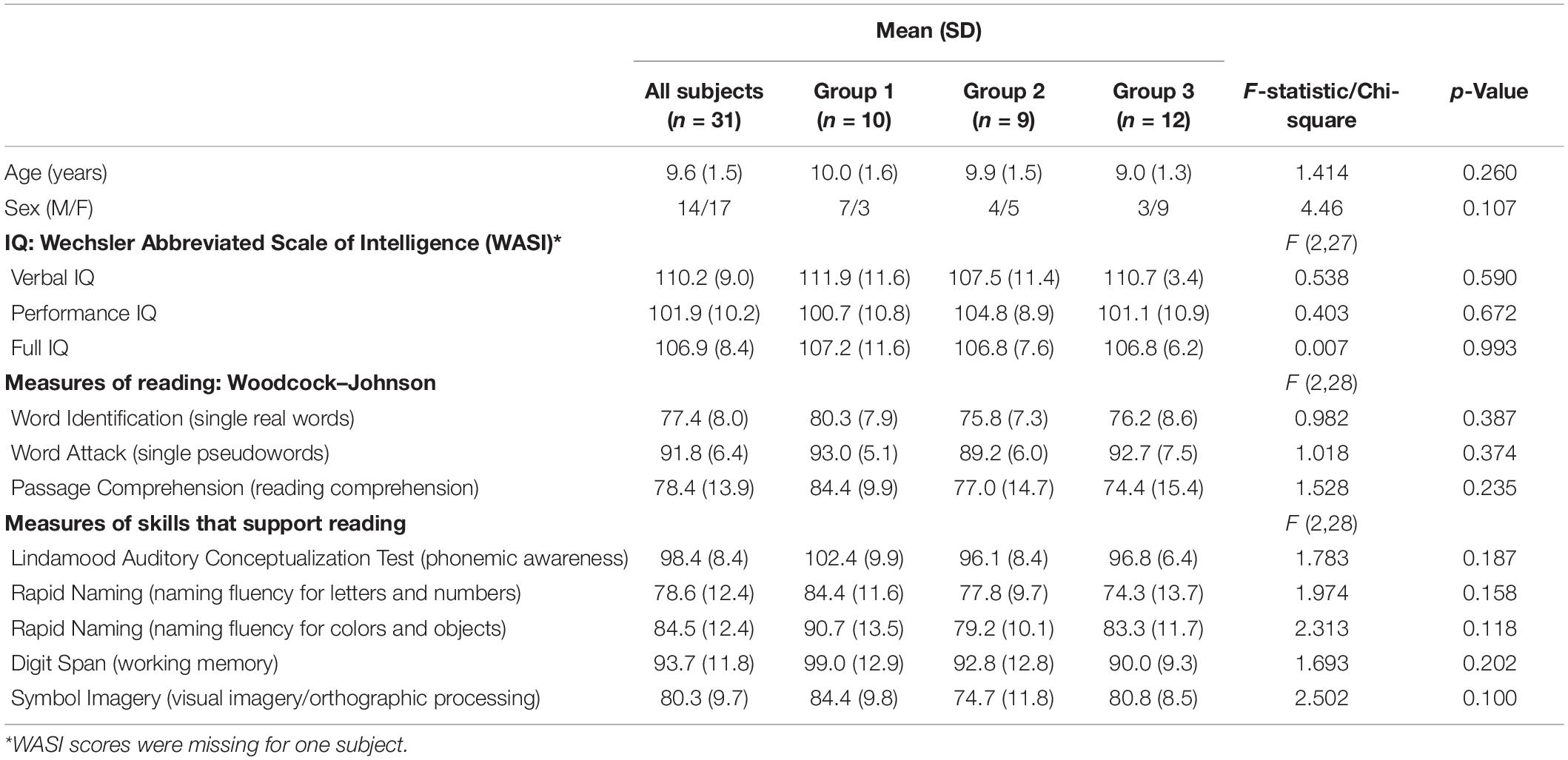
Table 1. Behavioral profile prior to intervention (Visit 1) for entire group and by intervention group.
Reading and Math Interventions
All 31 children received the reading intervention Seeing Stars® (Bell, 1997) purchased by us from Lindamood-Bell Learning Processes® and delivered by their employees at the children’s school in small groups. This intervention uses a “multisensory approach” to promote integration of internal visual and phonological representations of letters and letter strings. The imagery portion increases in difficulty starting with single letter imagery and increasing through two and three syllable words. A tactile/motor portion involves finger tracing of visualized letters, and a language production portion involves aloud verbalization of letter and syllable sounds while they are finger traced in the air. The use of imagery/visualization in this reading intervention is based on several studies involving the use of imagery in reading, including self-report of imagery during reading (Long et al., 1989), imagery in semantic retrieval (Kosslyn, 1976) and the use of imagery to improve processing and comprehension (Linden and Wittrock, 1981; Sadoski, 1983).
The math intervention was On Cloud Nine® (Bell and Tuley, 1997) and was delivered by the same Lindamood-Bell Learning Processes® staff at the school. It utilizes a multisensory approach focusing on imagery, tracing and verbalization, similar to the reading intervention, but with a focus on numbers and number lines instead of letters and syllables, thus serving as a suitable active control for the reading intervention.
Behavioral Analyses
To test for performance changes brought about by the interventions, we conducted 2 × 2 repeated measures ANOVAs with Time Point (pre- versus post intervention) as a within-subjects factor and sex (male versus female) as a between-subjects factor (Time Point × Sex). Each analysis was specific to a reading or reading-related measure and a particular intervention, e.g., children’s pre- and post-reading intervention data for a given measure were included as “Time Points” to examine changes during the reading intervention. We refer to Time Points here as opposed to Visits in the description of the intervention design above (Figure 1) because of the counterbalanced design. For example, some participants’ pre-reading intervention visit was Visit 1, whereas others it was Visit 2; to investigate changes following the reading intervention, we use data from each subject’s pre- and post-reading intervention Time Points. We employed a Holm–Bonferroni correction for multiple comparisons (Holm, 1979). These pre- versus post intervention comparisons are similar to those presented in previous reading intervention studies (that did not include a control period) and are presented here for the purpose of comparison with behavioral gains following intervention in those studies.
However, to test whether any such gains in reading during the reading intervention are significantly greater than any gains during the control period, we conducted a 2 × 2 repeated measures ANOVA using intervention (reading intervention period versus math intervention/null period) and performance measure (change in reading ability on W-J WID versus change in math ability on Calculation standard score) as within-subjects factors and tested for an interaction.
To test if performance measures predicted reading gains, the three reading and the five reading-related measures at Visit 1 (prior to any intervention), age, IQ, and sex were entered into a single multiple regression with change in reading ability on W-J WID standard score as the dependent variable.
Behavioral analyses and visualization were carried out in SPSS (IBM SPSS Statistics 22), jamovi (version 2.5.5), and Microsoft Excel.
Functional Magnetic Resonance Imaging Data Acquisition and Preprocessing
During acquisition of fMRI data, subjects performed an implicit reading task (Price et al., 1996). The children saw single real words (Word) or false font strings (False Font) and responded with a button press in their right hand if the Word or False Fonts contained a “tall” letter or character (e.g., “alarm” contains the tall letter “l”) and a button press in their left hand if it did not (e.g., sauce has no tall letters). This task has been used previously in our studies of reading and reading disability (Turkeltaub et al., 2003, 2004; Olulade et al., 2013, 2015; Evans et al., 2016). Blocks of Word and blocks of False Font stimuli alternated (twice each) and were separated by blocks of Fixation. Blocks of Words and False Font contained 10 trials each, lasting 42 s, and Fixation blocks lasted 18 s each (with additional Fixation scans at the beginning and end of the run, resulting in a total scan time of four minutes, twenty-seven seconds). Each child underwent two scan acquisitions (two runs yielding 28 whole-head echo planar imaging (EPI) volumes for each condition, Word, False Font and Fixation) at three different times over the study (one at each visit as described above, see Figure 1).
Functional magnetic resonance imaging fMRI data was acquired using an EPI sequence using a 3 Tesla Siemens Trio whole-body MRI system [TE = 30 ms, TR = 3 s, 64 × 64 matrix, 192 mm FOV, 50 axial slices, 2.8 mm slice thickness (0.2 mm interslice gap) yielding 3 mm cubic voxels, flip angle 90°]. A high resolution, 3D T1-weighted MPRAGE image obtained at the outset of the study (Visit 1, prior to any intervention) on the same Siemens Trio whole-body MRI system was used to aid in anatomical localization of the fMRI data.
Pre-processing for functional analysis began by segmenting the subjects’ MPRAGE images and normalizing to a standard template brain (Montreal Neurological Institute, MNI). For all functional runs, the first five scans were removed, and the remaining scans were corrected for head motion by realigning to the mean image, co-registered to the subjects MPRAGE, normalized using the same parameters for the MPRAGE image and finally smoothed using a 6 mm × 6 mm × 5.8 mm Gaussian kernel. For each subject’s first level analysis, both runs were included, and contrasts were generated for the Word versus False Font condition, Word versus Fixation condition and False Font versus Fixation condition. Motion parameters and global mean signal were included as regressors of no interest to account for subject movement and global signal variation during each run.
Functional Magnetic Resonance Imaging Group Level Analyses
All analyses were carried out in SPM (Statistical Parametric Mapping, Wellcome Trust Centre for Neuroimaging, London, United Kingdom). All group analyses (differences in activation pre- versus post the intervention, and activation to predict intervention-induced changes in reading performance) were performed on Words > False Font contrasts at an uncorrected height threshold of p < 0.001, and an extent threshold of p < 0.05 family wise error (FWE) corrected. For the analysis on activation to predict intervention-induced reading gains, the MarsBaR toolbox (Brett et al., 2002) was used to extract the signal from clusters identified in the analyses (described below), to display the mean percent signal change. Again, sex was included as a between-subjects factor in the intervention Time Point comparisons (same as in the behavioral analyses).
Results
Behavioral Measures Change Following the Reading Intervention
To evaluate the impact of the reading intervention, the 2 × 2 repeated measures ANOVAs conducted on the standard scores of the three measures of reading and the five measures of reading-related skills, as well as the three math skills, immediately prior to and following the reading intervention (Time Point as within-subjects factor and Sex as between-subjects factor) found six of the eight reading/reading-related measures showed a significant main effect of Time Point and increased scores following the reading intervention (Table 2): real word reading (W-J WID), pseudoword reading (W-J WA), reading comprehension (W-J PC), phonemic awareness (LAC), naming fluency of letters/numbers (RAN L&N), and visual imagery (SI). One of the math measures (W-J Math Fluency) showed a significant main effect of Time Point, decreasing after the reading intervention. After Holm–Bonferroni correction (Holm, 1979), all three reading measures (real word reading, pseudoword reading, and passage comprehension), two of the five reading-related measures (phonemic awareness and visual imagery), and none of the math measures remained significant. As such the children made gains on a range of measures of reading, as well as the skills targeted by the intervention and known to promote reading acquisition (see Figure 2). There were no significant interactions for Time-Point × Sex.
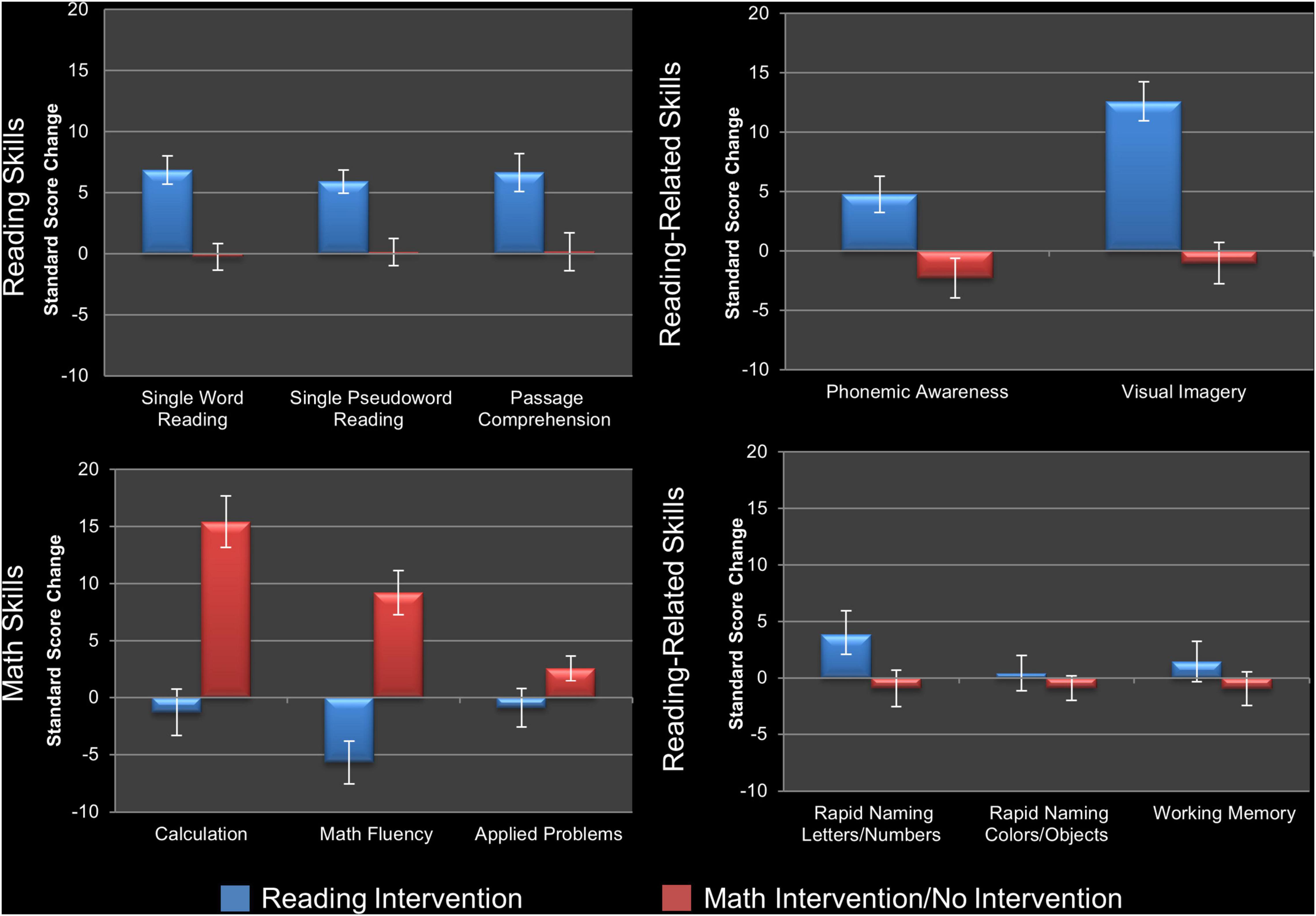
Figure 2. Changes in behavioral measures following intervention. Visualization of standard score changes in measures of reading skills (top left), phonological and orthographic reading-related skills (top right), other reading-related skills (bottom right), and math skills (bottom left) for the analyses reported in Table 2. Reading skills: single real word reading (Word Identification), single pseudoword reading (Word Attack), and reading comprehension (Passage Comprehension). Phonological and orthographic reading-related skills: phonemic awareness (Lindamood Auditory Conceptualization Test), and visual imagery/orthographic processing (Symbol Imagery). Other reading-related skills: naming fluency of letters/numbers and colors/objects (Rapid Automatized Naming test, and working memory (Digit Span). Math skills: computational ability (Calculation), timed arithmetic (Math Fluency), and mathematical word problems (Applied Problems). Error bars show standard error for the average change in standard score. For statistical tests see text.
When the same analyses were conducted following the control periods (math and no intervention) none of the reading or reading-related measures changed (Table 2), demonstrating the specificity of the above-described effects of the reading intervention. To ensure this result was not because one type of control period (e.g., math intervention) had effects which were canceled out or diluted by opposite effects of the other control period (e.g., no intervention developmental control), a one-way ANOVA for Group on changes following the reading intervention was conducted for these two specific arms of the study and showed no significant differences (Table 3). While there were no gains made in reading in the groups receiving the math intervention, this intervention resulted in significant gains on measures of mathematics. Specifically, there were significant main effects of Time Point for the measures of mathematic computational ability (W-J Calculation), timed arithmetic (W-J Math Fluency), and mathematical word problems (W-J Applied Problems), with all measures increasing over this time period. The first two results remain significant after Holm–Bonferroni correction (Table 2), demonstrating that the math intervention was successful and specific in bringing about gains in the domain of math (see Figure 2). There were no significant interactions for Time-Point × Sex during the math intervention.
While these analyses allow for direct comparison with previous studies that did not include control periods in their experimental design, we ultimately wanted to test whether gains in reading were statistically greater during the reading intervention period compared to the control periods. For this we conducted a 2 × 2 repeated measures ANOVA for intervention (reading intervention versus math intervention/null period) × measure (change in reading ability on W-J WID versus change in math ability on Calculation standard score) and specifically examined the interaction. The interaction was significant [F(1,30) = 18.52, p < 0.001], and the post hoc test of reading change during the reading intervention versus reading change during the math intervention/null period was significant [t(30) = 3.81, p = 0.004). During the reading intervention the average reading (W-J WID) standard score change was 6.84, while during the math intervention/null period it was −0.29. Similarly, for the average math (Calculation) standard score change during the math intervention/null period there was an average increase of 7.84, while during the reading intervention scores decreased by −0.26.
Lastly, turning to the follow-up visit, there were no significant differences on the standardized reading measures between the time the children completed the reading intervention and 1 year later (12.4 months on average; assessed in 26 of the original 31 participants; Digit Span was not assessed) indicating that the children maintained the same level of performance they had reached at the end of the intervention. Specifically, as the raw scores on average increased, the standardized measures revealed no significant changes (p > 0.05) for any of the eight measures listed above.
Behavioral Measures as Predictors of Intervention-Induced Gains in Reading
We next investigated whether our behavioral measures of reading and reading-related skills prior to the intervention were predictive of the reading gains made in single real word reading (W-J WID) by the completion of the reading intervention. That is, in the whole group (n = 31), each of the eight reading or reading-related measures at Visit 1, along with age, Full IQ, and sex were entered into a multiple regression with change in single real word reading (W-J WID difference before and after the reading intervention) standard score as the dependent variable. The model was not significant [F(11,18) = 0.523, p = 0.863], and none of the variables contributed to predicting single word reading score change (all p-values > 0.05). There was evidence of collinearity among several of the reading and reading-related measures (VIF > 2.5). Removing these specific variables (pseudoword reading, passage comprehension, SI, and age) showed no improvement [F(7,22) = 0.788, p = 0.605) and again none of the variables contributed to predicting single real word reading score change (all p-values > 0.05).
Brain Activation Changes Following the Reading Intervention
A 2 × 2 ANOVA (Time Point × Sex) to examine changes in brain activity following the reading intervention, and whether sex played a role, yielded no significant results for main effect of Time Point, or for Time Point × Sex interaction (height threshold of p < 0.001, uncorrected, extent threshold p < 0.05 FWE corrected).
As there were no significant findings in this first analysis examining changes in activation following the reading intervention, there no longer was a need to assess these pre-post differences in comparison to the control periods (math intervention/null).
Brain Activation as Predictors of Intervention-Induced Gains in Reading
A simple regression analysis of brain activity during reading task at Visit 1 with change in single real word reading (W-J WID difference before and after the reading intervention), revealed two clusters, one in left and the other in right supramarginal/angular gyri (BA 39/40) (height threshold of p < 0.001 uncorrected, extent threshold of p < 0.05 FWE, corrected) as depicted in Figure 3 (see Table 4). To examine if this predictive relationship was specific to the reading intervention, the signal in these two clusters (activity during reading task at Visit 1) was submitted for correlations with change in single real word reading standard scores during the math intervention (as above, W-J WID standard score differences prior to and immediately after intervention), but neither cluster was significant, showing that the predictive powers in this region were specific to outcomes following the reading intervention.
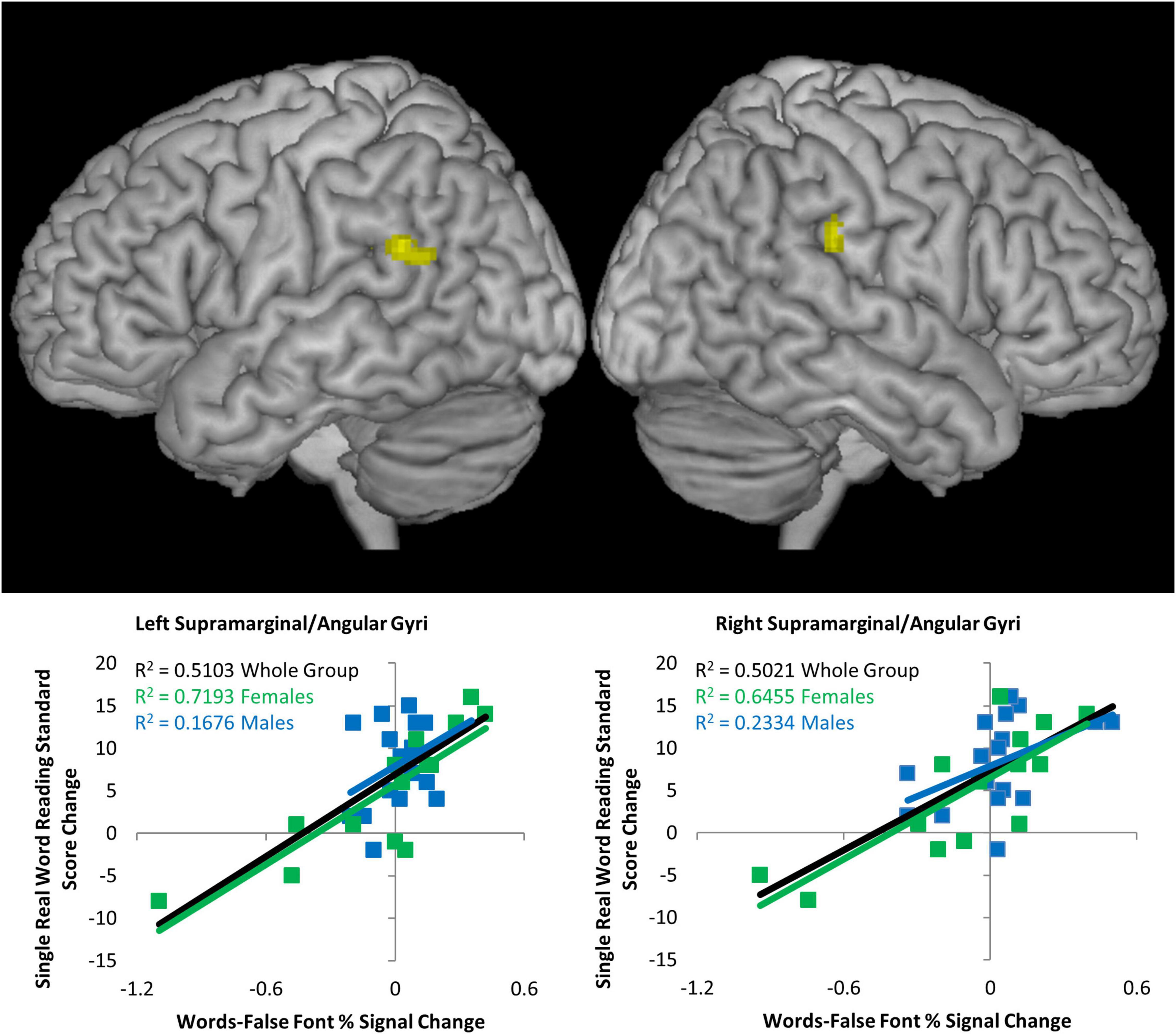
Figure 3. Brain activation predictors of reading gain. Simple regression of Words > False Font activation prior to the intervention versus change in single real word reading (Word Identification) standard score following the reading intervention (p < 0.001 uncorrected height threshold, FWE corrected extent threshold p < 0.05). Top: lateral whole brain views of the whole group relationship between left and right supramarginal/angular gyrus activation and change in score. Bottom: signal extracted from each cluster with scatterplots for the whole group (black), females only (green), and males only (blue). R2 values show strong relationships for both brain regions for females, while in males on the right hemisphere cluster was (barely) significant.
This relationship between brain activity during reading task at Time Point 1 and change in reading score was examined for females and males separately. In females only, the relationships were very strong in both the left hemisphere (r = 0.848, p = 1.3 × 10–4) and right hemisphere (r = 0.803, p = 5.4 × 10–4). In males only, the relationships was not significant in the left hemisphere (r = 0.409, p = 0.103) and barely significant in the right hemisphere (r = 0.483, p = 0.049) as visualized by scatterplots in Figure 3.
Discussion
In the present study we studied children with dyslexia: (1) to test for intervention-induced changes in reading performance and in brain activity during reading; and (2) to determine if behavioral measures or brain activity prior to the reading intervention predicted intervention-induced gains in reading. We used a cross-over design allowing us to directly compare reading intervention outcomes with a control period (within-subject control). Overall, the children made strong gains in reading performance (single real word reading, single pseudoword decoding and reading comprehension) as well as the two skills trained during the intervention (phonological and orthographic processing). These gains were specific to the reading intervention as the control math intervention resulted in gains on math but not reading measures, with an ANOVA showing a clear dissociation of the effects of the reading intervention period versus the math/null control period on reading performance versus math performance. However, there were no significant changes in brain activity following the reading intervention. On the other hand, while behavioral measures prior to the onset of the intervention did not predict reading gains made during the reading intervention, brain activation during reading prior to the reading intervention did predict reading gains made during the reading intervention (in left and right supramarginal/angular gyri). Interestingly, while sex was not a significant factor in any of the analyses up until this point, this predictive relationship between pre-intervention brain activity and reading gains following the reading intervention was significant in female subjects, whereas males showed no significant relationship in the left hemisphere and barely in the right hemisphere. These results show that brain activity does not shed light on the neural bases of a successful and enduring reading intervention, but unlike measures of behavior, it identifies regions that signal a level of brain activity that indicates eventual treatment success; and this predictive signal is manifest strongly in females, but not males.
Behavioral Measures Change Following Reading Intervention
Our study showed performance gains in reading and reading-related skills following the reading intervention. Notably gains occurred on all three measures of reading, namely single real word reading, pseudoword decoding, as well as reading comprehension, the latter ultimately being the raison d’être for reading. No such reading gains occurred in the same children following their control period where some were engaged in a math intervention (active control) with others receiving no intervention at all (null period for developmental control). While one might have expected small carryover effects from the reading intervention into the control periods (due to the within-subject, cross-over design), we did not see gains in reading during the math intervention control period or the null period developmental control. Importantly, an ANOVA confirmed that gains in single word reading performance following the reading intervention was significantly different from any changes in single word reading following the math intervention. Both interventions required the participants’ attention; and they both involved the tutor motivating the child to learn. As such, we can be assured that the reading gains can unequivocally be attributed to the information learned during the reading intervention and were not due to domain-general effects such as attention, or the result of a Hawthorn, or placebo effect. Lastly, these behavioral gains were independent of sex, demonstrating no sex-specific effects on intervention-induced gains in reading.
Overall, our results are similar to those in a prior study of adults with dyslexia (Eden et al., 2004), which used a similar tutoring approach and resulted in measurable gains in single real and pseudoword word reading (but not reading comprehension) as well as in the skills that were trained by the intervention (phonemic awareness and visual imagery). However, children with reading disability in a recent study (Christodoulou et al., 2017; Romeo et al., 2017) did not make gains on these (Word Identification subtest and Word Attack) or other measures of reading, even though the same intervention was used as the one employed here. This underscores the challenges of dyslexia and the fact that not all intervention studies result in a favorable outcome.
Turning to the gains in reading-related measures, it is no surprise that we found gains in those skills trained by the reading intervention, namely, phonemic awareness and visual imagery, demonstrating task-specific training. However, these gains were accompanied by gains in reading, indicating that these improvements in skills that support reading generalized and transferred to reading. Other skills known to support reading acquisition were studied: naming fluency and digit span, which together with phonemic awareness have been described as a set of interrelated phonological processing skills that are impaired in dyslexia due to a core phonological deficit (Wagner and Torgesen, 1987; Stanovich, 1988). As such our results shed light on the fact that these three skills are interrelated yet separate, with gains in one not necessarily accompanied by an equal gain in another. It is also possible that some of these other skills are not as pliable. For example, while some have advocated that working memory can be improved through training (Spencer-Smith and Klingberg, 2015), the strength and generalizability of these gains has been debated (Shipstead et al., 2012; Spencer-Smith and Klingberg, 2015; Nutley and Söderqvist, 2017). However, most likely changes in naming fluency and digit span did not occur because they were not targeted by the intervention. Nevertheless, it is interesting that gains can be made in reading without advancing these two skills.
None of these gains in reading, phonemic awareness or visual imagery were influenced by sex (male versus female). So, while there are behavioral (Wolf and Gow, 1986; Voyer et al., 1995; Weiss et al., 2003; Bornstein et al., 2004) and brain imaging studies (Clements et al., 2006; Sato, 2020; however, see Wallentin, 2009) showing sex-specific effects for language and spatial processing skills, in children with reading disability one sex does not have an advantage over the other when it comes to training the understanding of the sound structure of spoken language and how it maps to print, or the ability to visualize words in one’s mind.
Benefits of the intervention appeared to have longevity, as the standard scores were maintained a year later (as a function of their raw scores increasing), indicating that the students’ progress since the intervention ended was of a magnitude that is consistent with all children in their age group based on this normed testing instrument.
No Brain Activation Changes Following Reading Intervention
There has been much interest in brain changes following skill acquisition and training. It is known that brain function during object processing in adults who are literate is dramatically different from that of illiterate adults, speaking to the adaptations that occur in the brain as a consequence of learning to read (Dehaene et al., 2010). Successful treatment of dyslexia, it would seem, should be accompanied by changes in brain function. Not only did we observe changes in brain function in adults with dyslexia undergoing a similar intervention (Eden et al., 2004), but prior studies in children have shown increases in activation during letter or word stimuli following reading interventions (Aylward et al., 2003; Temple et al., 2003; Shaywitz et al., 2004). However, as already noted in the Introduction, findings from individual intervention studies are variable. While one meta-analysis of brain imaging studies of reading interventions identified five regions of overlap, specifically in left thalamus, right insula/inferior frontal gyrus, left inferior frontal gyrus, right posterior cingulate, and left middle occipital gyrus, some foci in the meta-analysis were found in only two of the eight studies included. The authors themselves expressed caution in their interpretation of these results because of the variability in the methodologies used in these studies (Barquero et al., 2014). A more recent meta-analysis found no such convergence of results anywhere in the brain (Perdue et al., 2022) and pointed out significant limitations in the existing studies. It is worth noting that ours is the first study to use a within-subject control period to assess activation changes in dyslexia. We also applied a more stringent threshold than previous studies, recognizing that all older studies were accustomed to less stringent practices. Taking all of these factors into consideration our absence of a finding should perhaps not be all that surprising.
However, the question arises whether using a more targeted approach than the whole-brain analysis would have yielded a finding. As such we also conducted a post hoc region-of-interest (ROI) analysis. The details of this analysis were not described in section “Materials and Methods” because they followed later, but the approach is similar to that used in other studies (e.g., Brem et al., 2010) and in our prior work (Olulade et al., 2015), and is described in the Supplementary Material with results presented in Supplementary Figure 1. The results yielded no significant changes in specific regions of the fusiform gyrus (home of the visual word form area), even though this very region has shown to be altered by learning to read (Dehaene et al., 2010).
Our interpretation of these results is that there are several possible mechanisms at work, which cannot be differentiated in the current study and could also account for prior variability in the published results. One possibility is that even though gains were made in reading performance, the brain has remained unchanged, such that improved behavior occurs despite persisting functional aberrations. Based on prior, varied findings, we think it is more likely, however, that it has changed, but we are not able to measure these changes because they are too variable to be captured in a group analysis. Such variability would reflect the fact that reading intervention promotes functional changes, but that they occur in different brain regions for different individuals. A likely reason for this would be that if brain regions typically involved in reading do not change following the intervention and instead other brain areas compensate, this compensation may fall to different regions in different individuals. A subset of individuals may be mobilizing traditional reading networks, however, they represent enough of a minority that they are not captured in the group results. In addition to these mechanisms, there will always be some children who did not have brain changes because they did not make significant gains in reading. In this context, however, it is worth noting that a post hoc analysis correlating change in reading with change with activity did not yield any findings either. Considering that profiles of dyslexia can be unique, and prior studies on differences in brain anatomy and function in dyslexia have not entirely converged, it is not unreasonable to expect that changes following remediation could show similar variability. In fact, children who have struggled with reading will have received reading instruction or intervention of varying quality, and these prior experiences (which are very difficult to control for) will also be reflected in this variability. It is also possible that any of these sources of variability is reduced in adults, where lower plasticity in adulthood constrains intervention-induced changes to a limited set of brain regions, a possibility that merits further investigations by studying children and adults with dyslexia in the same study. It is also possible that heterogeneity amongst children can be reduced by identifying children at risk for dyslexia (based on a family history of dyslexia) an approach that is helpful in the quest to determine the etiology of dyslexia (Lohvansuu et al., 2021).
In Females, Brain Activity but Not Behavioral Measures, Predict Gains in Reading Following Intervention
Behavioral measures were unsuccessful at predicting later reading outcome, yet brain activity was. While prior behavioral studies, which demonstrated phonological skills to predict word level reading skills (Hatcher and Hulme, 1999; Torgesen et al., 1999; Catts et al., 2001) set expectations that we would find skills like single real and single pseudoword reading and phonemic awareness to be indicative of later reading gains, we did not. This aligns with a prior report by Hoeft et al. (2011), who found none of 17 reading measures to predict changes in single real word reading over 2.5 years, yet brain activation in right inferior frontal gyrus during a written word rhyming task predicted change in single word reading over the same time period. The current study found that activity in inferior parietal cortex in the left and right hemisphere predicted post-reading intervention gains in single word reading. However, closer examination showed that this effect was driven by the females in the group. Females had strong predictive relationships between activity during reading in the left and right supramarginal/angular gyri and later reading gains, while there was no result for males in the left hemisphere and the effect in the right supramarginal/angular gyrus barely meet significance.
Left temporoparietal cortex represents the indirect route in the dual-route model for reading and is thought to subserve phoneme-grapheme mapping (Pugh et al., 2000, 2001; Coltheart et al., 2001; Jobard et al., 2003). It is thought to be especially important during the early process of learning to read (when new words need to be “sounded out”) and remains engaged into adulthood (Pugh et al., 2001; Turkeltaub et al., 2003; Sandak et al., 2004; Frost et al., 2009). In dyslexia however, this region is underactivated: The left inferior parietal region identified here maps precisely onto the location of less activity in those with dyslexia relative to typical readers identified by meta-analysis (Maisog et al., 2008). Greater engagement of left inferior parietal cortex while processing orthographic and phonological representations of words represent a sign of a brain that is ready to make greater gains in reading once targeted, structured and intense instructions are provided. Of the studies reviewed in the Introduction, one showed left inferior parietal lobule connectivity with middle temporal gyrus during a lexical decision task was predictive of basic reading skill gains following a reading intervention (Aboud et al., 2018). Our results are also consistent with two magnetoencephalography (MEG) studies showing activity in temporoparietal regions (and others) at baseline predicted reading fluency gains following intervention at a 1 year follow-up (Rezaie et al., 2011a,b). Specifically, signal in left and right middle and superior temporal gyri, left supramarginal and angular gyri, left ventral occipitotemporal regions, and right mesial temporal cortex were related to gains in reading fluency.
Why girls but not boys show a relationship where more engagement of left inferior parietal cortex during reading leads to reaping greater benefits from the intervention is not clear. Turning to the literature on the role of sex hormones on brain development, it has been shown that there is a negative correlation between fetal testosterone (in utero) and early childhood gray matter volume (8–11 years old) in right TPC, suggesting that the development of this region in males may be modulated by this sex hormone (Lombardo et al., 2012). This in turn may have an impact on brain function, possibly even in the contralateral hemisphere. Post-mortem studies in adults known to have had dyslexia during their lifetime revealed neuronal ectopias (attributed to developmental errors in neuronal migration), primarily in perisylvian regions (Galaburda et al., 1985) and primarily in males (Humphreys et al., 1990). It has been shown that estrogen treatment in women results in increased activation for verbal stimuli, and decreased activation for non-verbal stimuli in the left and right inferior parietal lobule during working memory tasks (Shaywitz et al., 1999). Based on these factors it has been suggested that males and females with dyslexia may have different etiological profiles due to their different hormonal environments (Krafnick and Evans, 2019).
However, it is important to note that the males and females did not differ in the gains they made following the reading intervention. While they may have reached these identical goals in different ways, it is not clear whether there are changes in brain activity following the intervention that are sex-dependent but if there are, we did not capture them. Yet in females, but not males, we were able to identify a left inferior parietal brain region that signals a level or readiness of brain function, promising that the introduction of an intervention will lead to a successful outcome. This suggests some separation of brain function in regions that bring about gains in reading, and regions that signal what may be a certain level of brain function that is required in order to harness the benefits of the intervention, but just in females. Future studies will need to disentangle the relationship between these and directly examine if this predictive relationship is under hormonal influence.
Conclusion
The results of this study suggest that while it is possible to see significant, specific and enduring gains in reading performance in children/adolescents with dyslexia following intensive treatment, individual variability may explain the fact that we did not observe any change in brain activity following the intervention. On the other hand, brain activity in left TPC predicted reading gains resulting from the intervention, while behavioral measures did not. Interestingly, the predictive powers of brain activity for reading outcome were attributed to the females but not males in our group, suggesting sexual dimorphism in the relationship between brain function during reading and the ability to reap benefits from intensive, structured reading intervention. As a whole, this work suggests there is considerable work to be done to understand brain changes related to reading intervention in order to determine what mechanisms are at work to promote these gains.
Data Availability Statement
The raw data supporting the conclusions of this article will be made available by the authors, without undue reservation.
Ethics Statement
The studies involving human participants were reviewed and approved by the Georgetown University (IRB). Written informed consent to participate in this study was provided by the participants’ legal guardian/next of kin.
Author Contributions
GE and DLF conceived and designed the study. GE and EN were involved in overall study logistics and data collection. AK performed the statistical analyses and together with GE drafted the manuscript. All authors contributed to the manuscript and approved the submission.
Funding
This work was supported by the Eunice Kennedy Shriver Institute for Child Health and Human Development (P50 HD040095 and HD081078).
Conflict of Interest
The authors declare that the research was conducted in the absence of any commercial or financial relationships that could be construed as a potential conflict of interest.
Publisher’s Note
All claims expressed in this article are solely those of the authors and do not necessarily represent those of their affiliated organizations, or those of the publisher, the editors and the reviewers. Any product that may be evaluated in this article, or claim that may be made by its manufacturer, is not guaranteed or endorsed by the publisher.
Acknowledgments
We would like to thank Ashley Wall, Emma Cole, Corinna Moore, Jenni Rosenberg, Iain DeWitt, Robert Twomey, and Alison Merikangas for aiding with data acquisition. We also thank our participants and their families for volunteering their time, the Jemicy School for their support and for allowing us to conduct the intervention at their school and the staff from Lindamood-Bell Learning Processes for administering the intervention as determined by the research protocol.
Supplementary Material
The Supplementary Material for this article can be found online at: https://www.frontiersin.org/articles/10.3389/fnins.2022.898661/full#supplementary-material
References
Aboud, K. S., Barquero, L. A., and Cutting, L. E. (2018). Prefrontal mediation of the reading network predicts intervention response in dyslexia. Cortex 101, 96–106. doi: 10.1016/j.cortex.2018.01.009
Alexander, A. W., and Slinger-Constant, A. M. (2004). Current status of treatments for dyslexia: critical review. J. Child Neurol. 19, 744–758. doi: 10.1177/08830738040190100401
Altarelli, I., Monzalvo, K., Iannuzzi, S., Fluss, J., Billard, C., Ramus, F., et al. (2013). A functionally guided approach to the morphometry of occipitotemporal regions in developmental dyslexia: evidence for differential effects in boys and girls. J. Neurosci. 33, 11296–11301. doi: 10.1523/JNEUROSCI.5854-12.2013
Aylward, E. H., Richards, T. L., Berninger, V. W., Nagy, W. E., Field, K. M., Grimme, A. C., et al. (2003). Instructional treatment associated with changes in brain activation in children with dyslexia. Neurology 61, 212–219. doi: 10.1212/01.wnl.0000068363.05974.64
Bach, S., Richardson, U., Brandeis, D., Martin, E., and Brem, S. (2013). Print-specific multimodal brain activation in kindergarten improves prediction of reading skills in second grade. Neuroimage 82, 605–615. doi: 10.1016/j.neuroimage.2013.05.062
Badian, N. A. (1995). Predicting reading ability over the long term: the changing roles of letter naming, phonological awareness and orthographic processing. Ann. Dyslexia 45, 79–96. doi: 10.1007/BF02648213
Badian, N. A. (2001). Phonological and orthographic processing: their roles in reading prediction. Ann. Dyslexia 51, 179–202.
Baron, L. S., Hogan, T. P., Alt, M., Gray, S., Cabbage, K. L., Green, S., et al. (2018). Children with dyslexia benefit from orthographic facilitation during spoken word learning. J. Speech Lang. Hear. Res. 61, 2002–2014. doi: 10.1044/2018_JSLHR-L-17-0336
Barquero, L. A., Davis, N., and Cutting, L. E. (2014). Neuroimaging of reading intervention: a systematic review and activation likelihood estimate meta-analysis. PLoS One 9:e83668. doi: 10.1371/journal.pone.0083668
Bell, N. (1997). Seeing Stars: Symbol Imagery for Phonemic Awareness, Sight Words, and Spelling. San Luis Obispo, CA: Gander Publishing.
Bell, N., and Tuley, K. (1997). On Cloud Nine: Visualizing and Verbalizing for Math. San Luis Obispo, CA: Gander Publishing.
Bornstein, M. H., Hahn, C.-S., and Haynes, O. M. (2004). Specific and general language performance across early childhood: stability and gender considerations. First Lang. 24, 267–304
Bornstein, M. H., Haynes, O. M., Painter, K. M., and Jenevro, J. L. (2000). Child language with mother and with stranger at home and in the laboratory: a methodological study. J. Child Lang. 27, 407–420. doi: 10.1017/s0305000900004165
Brem, S., Bach, S., Kucian, K., Guttorm, T. K., Martin, E., Lyytinen, H., et al. (2010). Brain sensitivity to print emerges when children learn letter-speech sound correspondences. PNAS 107, 7939–7944. doi: 10.1073/pnas.0904402107
Brett, M., Valabregue, R., and Poline, J. (2002). “Region of interest analysis using an SPM toolbox,” in Proceedingds of the 8th International Conference on Functional Mapping of the Human Brain, Sendai.
Burman, D. D., Bitan, T., and Booth, J. R. (2008). Sex differences in neural processing of language among children. Neuropsychologia 46, 1349–1362. doi: 10.1038/nmeth.2250.Digestion
Cahill, L. (2006). Why sex matters for neuroscience. Nat. Rev. Neurosci. 7, 477–484. doi: 10.1038/nrn1909
Cahill, L. (2012). A half-truth is a whole lie: on the necessity of investigating sex influences on the brain. Endocrinology 153, 2541–2543. doi: 10.1210/en.2011-2167
Catts, H. W., Fey, M. E., Zhang, X., and Tomblin, J. B. (2001). Estimating the risk of future reading difficulties in kindergarten children. Lang. Speech Hear. Serv. Sch. 32, 38–50. doi: 10.1044/0161-1461(2001/004)
Christodoulou, J. A., Cyr, A., Murtagh, J., Chang, P., Lin, J., Guarino, A. J., et al. (2017). Impact of intensive summer reading intervention for children with reading disabilities and difficulties in early elementary school. J. Learn. Disabil. 50, 115–127. doi: 10.1177/0022219415617163
Clark, K. A., Helland, T., Specht, K., Narr, K. L., Manis, F. R., Toga, A. W., et al. (2014). Neuroanatomical precursors of dyslexia identified from pre-reading through to age 11. Brain 137, 3136–3141. doi: 10.1093/brain/awu229
Clements, A. M., Rimrodt, S. L., Abel, J. R., Blankner, J. G., Mostofsky, S. H., Pekar, J. J., et al. (2006). Sex differences in cerebral laterality of language and visuospatial processing. Brain Lang. 98, 150–158. doi: 10.1016/j.bandl.2006.04.007
Coltheart, M., Rastle, K., Perry, C., Langdon, R., and Ziegler, J. (2001). DRC: a dual route cascaded model of visual word recognition and reading aloud. Psychol. Rev. 108, 204–256.
Dehaene, S., Pegado, F., Braga, L. W., Ventura, P., Nunes Filho, G., Jobert, A., et al. (2010). How learning to read changes the cortical networks for vision and language. Science 330, 1359–1364. doi: 10.1126/science.1194140
Denckla, M. B., and Rudel, R. G. (1976b). Rapid “automatized” naming (RAN): dyslexia differentiated from other learning disabilities. Neuropsychologia 14, 471–479.
Denckla, M. B., and Rudel, R. G. (1976a). Naming of object-drawlings by dyslexic and other learning disabled children. Brain Lang. 3, 1–15.
Dionne, G., Dale, P. S., Boivin, M., and Plomin, R. (2003). Genetic evidence for bidirectional effects of early lexical and grammatical development. Child Dev. 74, 394–412. doi: 10.1111/1467-8624.7402005
Eden, G. F., Jones, K. M., Cappell, K., Gareau, L., Wood, F. B., Zeffiro, T. A., et al. (2004). Neural changes following remediation in adult developmental dyslexia. Neuron 44, 411–422. doi: 10.1016/j.neuron.2004.10.019
Eden, G. F., Olulade, O. A., Evans, T. M., Krafnick, A. J., and Alkire, D. R. (2015). “Developmental Dyslexia,” in Neurobiology of Language, eds G. Hickok and S. L. Small (Cambridge, MA: Academic Press), 815–826.
Evans, T. M., Flowers, D. L., Luetje, M. M., Napoliello, E. M., and Eden, G. F. (2016). Functional anatomy of arithmetic and word reading and its relationship to age. Neuroimage 143, 304–315. doi: 10.1016/j.neuroimage.2016.08.048
Evans, T. M., Flowers, D. L., Napoliello, E. M., and Eden, G. F. (2014). Sex-specific gray matter volume differences in females with developmental dyslexia. Brain Struct. Funct. 219, 1041–1054. doi: 10.1007/s00429-013-0552-4
Farris, E. A., Ring, J., Black, J., Lyon, G. R., and Odegard, T. N. (2016). Predicting growth in word level reading skills in children with developmental dyslexia using an object rhyming functional neuroimaging task. Dev. Neuropsychol. 41, 145–161. doi: 10.1080/87565641.2016.1158264
Frost, S. J., Landi, N., Mencl, W. E., Sandak, R., Fulbright, R. K., Tejada, E. T., et al. (2009). Phonological awareness predicts activation patterns for print and speech. Ann. Dyslexia 59, 78–97. doi: 10.1007/s11881-009-0024-y
Galaburda, A. M., Sherman, G. F., Rosen, G. D., Aboitiz, F., and Geschwind, N. (1985). Developmental dyslexia: four consecutive patients with cortical anomalies. Ann. Neurol. 18, 222–233. doi: 10.1002/ana.410180210
Gebauer, D., Fink, A., Kargl, R., Reishofer, G., Koschutnig, K., Purgstaller, C., et al. (2012). Differences in brain function and changes with intervention in children with poor spelling and reading abilities. PLoS One 7:e38201. doi: 10.1371/journal.pone.0038201
Hatcher, P. J., and Hulme, C. (1999). Phonemes, rhymes, and intelligence as predictors of children’s responsiveness to remedial reading instruction: evidence from a longitudinal intervention study. J. Exp. Child Psychol. 72, 130–153. doi: 10.1006/jecp.1998.2480
Heim, S., Pape-Neumann, J., van Ermingen-Marbach, M., Brinkhaus, M., and Grande, M. (2015). Shared vs. specific brain activation changes in dyslexia after training of phonology, attention, or reading. Brain Struct. Funct. 220, 2191–2207. doi: 10.1007/s00429-014-0784-y
Hoeft, F., McCandliss, B. D., Black, J. M., Gantman, A., Zakerani, N., Hulme, C., et al. (2011). Neural systems predicting long-term outcome in dyslexia. PNAS 108, 361–366. doi: 10.1073/pnas.1008950108
Hoeft, F., Ueno, T., Reiss, A. L., Meyler, A., Whitfield-Gabrieli, S., Glover, G. H., et al. (2007). Prediction of children’s reading skills using behavioral, functional, and structural neuroimaging measures. Behav. Neurosci. 121, 602–613. doi: 10.1037/0735-7044.121.3.602
Holm, S. (1979). Board of the foundation of the scandinavian journal of statistics a simple sequentially rejective multiple test procedure a simple sequentially rejective multiple test procedure. Scand. J. Statist. 6, 65–70.
Humphreys, P., Kaufmann, W. E., and Galaburda, A. M. (1990). Developmental dyslexia in women: neuropathological findings in three patients. Ann. Neurol. 28, 727–738. doi: 10.1002/ana.410280602
Jaeger, J. J., Lockwood, A. H., van Valin, R. D., Kemmerer, D. L., Murphy, B. W., and Wack, D. S. (1998). Sex differences in brain regions activated by grammatical and reading tasks. NeuroReport 9, 2803–2807. doi: 10.1097/00001756-199808240-00022
Jobard, G., Crivello, F., and Tzourio-Mazoyer, N. (2003). Evaluation of the dual route theory of reading: a metanalysis of 35 neuroimaging studies. Neuroimage 20, 693–712. doi: 10.1016/S1053-8119(03)00343-4
Kansaku, K., and Kitazawa, S. (2001). Imaging studies on sex differences in the lateralization of language. Neurosci. Res. 41, 333–337. doi: 10.1016/s0168-0102(01)00292-9
Katusic, S. K., Colligan, R. C., Barbaresi, W. J., Schaid, D. J., and Jacobsen, S. J. (2001). Incidence of reading disability in a population-based birth cohort, 1976-1982, Rochester, Minn. Mayo Clin. Proc. 76, 1081–1092. doi: 10.4065/76.11.1081
Kosslyn, S. M. (1976). Using imagery to retrieve semantic information : a developmental study. Child Dev. 47, 434–444.
Krafnick, A. J., Flowers, D. L., Napoliello, E. M., and Eden, G. F. (2011). Gray matter volume changes following reading intervention in dyslexic children. Neuroimage 57, 733–741.
Krafnick, A. J., and Evans, T. M. (2019). Neurobiological sex differences in developmental dyslexia. Front. Psychol. 9:2669. doi: 10.3389/fpsyg.2018.02669
Kyle, F., Kujala, J., Richardson, U., Lyytinen, H., and Goswami, U. (2013). Assessing the effectiveness of two theoretically motivated computerassisted reading interventions in the United Kingdom: GG Rime and GG Phoneme. Read. Res. Q. 48, 61–76. doi: 10.1002/rrq.038
Liederman, J., Kantrowitz, L., and Flannery, K. (2005). Male vulnerability to reading disability is not likely to be a myth: a call for new data. J. Learn. Disabil. 38, 109–129. doi: 10.1177/00222194050380020201
Lindamood, C., and Lindamood, P. (1971). Lindamood Auditory Conceptualization (LAC) Test. Austin, TX: Pro-Ed.
Linden, M., and Wittrock, M. C. (1981). The teaching of reading comprehension according to the model of generative learning. Read. Res. Q. 17, 44–57.
Lohvansuu, K., Torppa, M., Ahonen, T., Eklund, K., Hämäläinen, J. A., Leppänen, P. H. T., et al. (2021). Unveiling the mysteries of dyslexia—lessons learned from the prospective jyväskylä longitudinal study of dyslexia. Brain Sci. 11:427. doi: 10.3390/brainsci11040427
Lombardo, M. V., Ashwin, E., Auyeung, B., Chakrabarti, B., Taylor, K., Hackett, G., et al. (2012). Fetal testosterone influences sexually dimorphic gray matter in the human brain. J. Neurosci. 32, 674–680. doi: 10.1523/JNEUROSCI.4389-11.2012
Long, S. A., Winograd, P. N., and Bridge, C. A. (1989). The effects of reader and text characteristics on imagery reported during and after reading. Read. Res. Q. 24, 353–372.
Lyon, G. R., Shaywitz, S. E., and Shaywitz, B. A. (2003). Defining dyslexia, comorbidity, teachers’ knowledge of language and reading. A definition of dyslexia. Ann. Dyslexia 53, 1–14.
Maisog, J. M., Einbinder, E. R., Flowers, D. L., Turkeltaub, P. E., and Eden, G. F. (2008). A meta-analysis of functional neuroimaging studies of dyslexia. Ann. N. Y. Acad. Sci. 1145, 237–259. doi: 10.1196/annals.1416.024
Martin, D. J., and Hoover, H. D. (1987). Sex differences in educational achievement: a longitudinal study. J. Early Adolesc. 7, 65–83. doi: 10.1177/0272431687071007
Meyler, A., Keller, T. A., Cherkassky, V. L., Gabrieli, J. D. E., and Just, M. A. (2008). Modifying the brain activation of poor readers during sentence comprehension with extended remedial instruction: a longitudinal study of neuroplasticity. Neuropsychologia 46, 2580–2592. doi: 10.1016/j.neuropsychologia.2008.03.012
Nutley, S. B., and Söderqvist, S. (2017). How is working memory training likely to influence academic performance? Current evidence and methodological considerations. Front. Psychol. 8:69. doi: 10.3389/fpsyg.2017.00069
Odegard, T. N., Ring, J., Smith, S., Biggan, J., and Black, J. (2008). Differentiating the neural response to intervention in children with developmental dyslexia. Ann. Dyslexia 58, 1–14. doi: 10.1007/s11881-008-0014-5
Olulade, O. A., Flowers, D. L., Napoliello, E. M., and Eden, G. F. (2013). Developmental differences for word processing in the ventral stream. Brain Lang. 125, 134–145. doi: 10.1016/j.bandl.2012.04.003
Olulade, O. A., Flowers, D. L., Napoliello, E. M., and Eden, G. F. (2015). Dyslexic children lack word selectivity gradients in occipito-temporal and inferior frontal cortex. Neuroimage Clin. 7, 742–754. doi: 10.1016/j.nicl.2015.02.013
Partanen, M., Siegel, L., and Giaschi, D. (2019). Effect of reading intervention and task difficulty on orthographic and phonological reading systems in the brain. Neuropsychologia 130, 13–25. doi: 10.1016/j.neuropsychologia.2018.07.018
Perdue, M. V., Mahaffy, K., Vlahcevic, K., Wolfman, E., Erbeli, F., Richlan, F., et al. (2022). Reading intervention and neuroplasticity: a systematic review and meta-analysis of brain changes associated with reading intervention. Neurosci. Biobehav. Rev. 132, 465–494. doi: 10.1016/j.neubiorev.2021.11.011
Peterson, R. L., and Pennington, B. F. (2012). Developmental dyslexia. Lancet 379, 1997–2007. doi: 10.1016/S0140-6736(12)60198-6
Price, C. J., Wise, R. J., and Frackowiak, R. S. (1996). Demonstrating the implicit processing of visually presented words and pseudowords. Cereb. Cortex 6, 62–70. doi: 10.1093/cercor/6.1.62
Pugh, K. R., Mencl, W. E., Jenner, A. R., Katz, L., Frost, S. J., Lee, J. R., et al. (2000). Functional neuroimaging studies of reading and reading disability(developmental dyslexia). Ment. Retard. Dev. Disabil. Res. Rev. 213, 207–213. doi: 10.1002/1098-2779(2000)6:3<207::AID-MRDD8>3.0.CO;2-P
Pugh, K. R., Mencl, W. E., Jenner, A. R., Katz, L., Frost, S. J., Lee, J. R., et al. (2001). Neurobiological studies of reading and reading disability. J. Commun. Disord. 34, 479–492. doi: 10.1016/s0021-9924(01)00060-0
Quinn, J. M., and Wagner, R. K. (2015). Gender differences in reading impairment and in the identification of impaired readers: results from a large-scale study of at-risk readers. J. Learn. Disabil. 48, 433–445. doi: 10.1177/0022219413508323
Ramus, F., Altarelli, I., Jednoróg, K., Zhao, J., and Scotto di Covella, L. (2018). Neuroanatomy of developmental dyslexia: pitfalls and promise. Neurosci. Biobehav. Rev. 84, 434–452. doi: 10.1016/j.neubiorev.2017.08.001
Rezaie, R., Simos, P. G., Fletcher, J. M., Cirino, P. T., Vaughn, S., and Papanicolaou, A. C. (2011b). Temporo-parietal brain activity as a longitudinal predictor of response to educational interventions among middle school struggling readers. J. Int. Neuropsychol. Soc. 17, 875–885. doi: 10.1017/S1355617711000890
Rezaie, R., Simos, P. G., Fletcher, J. M., Cirino, P. T., Vaughn, S., and Papanicolaou, A. C. (2011a). Engagement of temporal lobe regions predicts response to educational interventions in adolescent struggling readers. Dev. Neuropsychol. 36, 869–888. doi: 10.1080/87565641.2011.606404
Richards, T. L., Aylward, E. H., Berninger, V. W., Field, K. M., Grimme, A. C., Richards, A. L., et al. (2006). Individual fMRI activation in orthographic mapping and morpheme mapping after orthographic or morphological spelling treatment in child dyslexics. J. Neurolinguist. 19, 56–86. doi: 10.1016/j.jneuroling.2005.07.003
Richlan, F. (2012). Developmental dyslexia: dysfunction of a left hemisphere reading network. Front. Hum. Neurosci. 6:120. doi: 10.3389/fnhum.2012.00120
Richlan, F., Kronbichler, M., and Wimmer, H. (2011). Meta-analyzing brain dysfunctions in dyslexic children and adults. Neuroimage 56, 1735–1742. doi: 10.1016/j.neuroimage.2011.02.040
Romeo, R. R., Christodoulou, J. A., Halverson, K. K., Murtagh, J., Cyr, A. B., Schimmel, C., et al. (2017). Socioeconomic status and reading disability: neuroanatomy and plasticity in response to intervention. Cereb. Cortex 28, 2297–2312. doi: 10.1093/cercor/bhx131
Rutter, M., Caspi, A., Fergusson, D., Horwood, L. J., Goodman, R., Maughan, B., et al. (2004). Sex differences in developmental reading disability: new findings from 4 epidemiological studies. J. Am. Med. Assoc. 291, 2007–2012. doi: 10.1001/jama.291.16.2007
Sadoski, M. (1983). An exploratory study of the relationships between reported imagery and the comprehension and recall of a story. Read. Res. Q. 19, 110–123.
Sandak, R., Mencl, W. E., Frost, S. J., and Pugh, K. R. (2004). The neurobiological basis of skilled and impaired reading: recent findings and new directions. Sci. Stud. Read. 8, 273–292.
Sato, M. (2020). The neurobiology of sex differences during language processing in healthy adults: a systematic review and a meta-analysis. Neuropsychologia 140:107404 doi: 10.1016/j.neuropsychologia.2020.107404
Scarborough, H. S., and Brady, S. A. (2002). Toward a common terminology for talking about speech and reading: a glossary of the “phon” words and some related terms. J. Lit. Res. 34, 299–336. doi: 10.1207/s15548430jlr3403
Shaywitz, B. A., Shaywitz, S. E., Blachman, B. A., Pugh, K. R., Fulbright, R. K., Skudlarski, P., et al. (2004). Development of left occipitotemporal systems for skilled reading in children after a phonologically- based intervention. Biol. Psychiatry 55, 926–933. doi: 10.1016/j.biopsych.2003.12.019
Shaywitz, B. A., Shaywitz, S. E., Pugh, K. R., Constable, R. T., Skudlarski, P., Fulbright, R. K., et al. (1995). Sex differences in the functional organization of the brain for language. Nature 373, 607–609.
Shaywitz, S. E., Shaywitz, B. A., Pugh, K. R., Fulbright, R. K., Skudlarski, P., Mencl, W. E., et al. (1999). Effect of estrogen on brain activation patterns in postmenopausal women during working memory tasks. JAMA 281, 1197–1202. doi: 10.1001/jama.281.13.1197
Shipstead, Z., Hicks, K. L., and Engle, R. W. (2012). Cogmed working memory training: Does the evidence support the claims? J. Appl. Res. Mem. Cogn. 1, 185–193. doi: 10.1016/j.jarmac.2012.06.003
Spencer-Smith, M., and Klingberg, T. (2015). Benefits of a working memory training program for inattention in daily life: a systematic review and meta-analysis. PLoS One 10:e0119522. doi: 10.1371/journal.pone.0119522
Stanovich, K. E. (1988). Explaining the differences between the dyslexic and the garden-variety poor reader: the phonological-core variable-difference model. J. Learn. Disabil. 21, 590–604. doi: 10.1177/002221948802101003
Temple, E., Deutsch, G. K., Poldrack, R. A., Miller, S. L., Tallal, P., Merzenich, M. M., et al. (2003). Neural deficits in children with dyslexia ameliorated by behavioral remediation: evidence from functional MRI. PNAS 100, 2860–2865. doi: 10.1073/pnas.0030098100
Torgesen, J. K., Wagner, R. K., Rashotte, C. A., Rose, E., Lindamood, P., Conway, C., et al. (1999). Preventing reading failure in young children with phonological processing disabilities: group and individual responses to instruction. J. Educ. Psychol. 91, 589–593.
Turkeltaub, P. E., Flowers, D. L., Verbalis, A., Miranda, M., Gareau, L., and Eden, G. F. (2004). The neural basis of hyperlexic reading: an fMRI case study. Neuron 41, 11–25. doi: 10.1016/s0896-6273(03)00803-1
Turkeltaub, P. E., Gareau, L., Flowers, D. L., Zeffiro, T. A., and Eden, G. F. (2003). Development of neural mechanisms for reading. Nat. Neurosci. 6, 767–773. doi: 10.1038/nn1065
Voyer, D., Voyer, S., Bryden, M. P., thank Ann Bigelow, W., Brooks, G. P., Bulman-Fleming, B., et al. (1995). Magnitude of sex differences in spatial abilities: a meta-analysis and consideration of critical variables. Psychol. Bull. 117, 250–270. doi: 10.1037/0033-2909.117.2.250
Wagner, R. K., and Torgesen, J. K. (1987). The nature of phonological processing and its causal role in the acquisition of reading skills. Psychol. Bull. 101, 192–212. doi: 10.1037//0033-2909.101.2.192
Wallentin, M. (2009). Putative sex differences in verbal abilities and language cortex: a critical review. Brain Lang. 108, 175–183.
Wanzek, J., and Vaughn, S. (2007). Research-based implications from extensive early reading interventions. Sch. Psychol. Rev. 36, 541–561.
Wechsler, D. (1999). Wechsler Abbreviated Scale of Intelligence. San Antonio: Psychological Corporation.
Weiss, E. M., Kemmler, G., Deisenhammer, E. A., Fleischhacker, W. W., and Delazer, M. (2003). Sex differences in cognitive functions. Pers. Individ. Differ. 35, 863–875.
Wolf, M., and Gow, D. (1986). A longitudinal investigation of gender differences in language and reading development. First Lang. 6, 81–110. doi: 10.1177/014272378600601701
Keywords: dyslexia, reading disability, intervention, fMRI, children
Citation: Krafnick AJ, Napoliello EM, Flowers DL and Eden GF (2022) The Role of Brain Activity in Characterizing Successful Reading Intervention in Children With Dyslexia. Front. Neurosci. 16:898661. doi: 10.3389/fnins.2022.898661
Received: 17 March 2022; Accepted: 23 May 2022;
Published: 13 June 2022.
Edited by:
Li-Hai Tan, Shenzhen Institute of Neuroscience, ChinaReviewed by:
Fan Cao, Sun Yat-sen University, ChinaHeikki Juhani Lyytinen, Niilo Mäki Institute, Finland
Copyright © 2022 Krafnick, Napoliello, Flowers and Eden. This is an open-access article distributed under the terms of the Creative Commons Attribution License (CC BY). The use, distribution or reproduction in other forums is permitted, provided the original author(s) and the copyright owner(s) are credited and that the original publication in this journal is cited, in accordance with accepted academic practice. No use, distribution or reproduction is permitted which does not comply with these terms.
*Correspondence: Guinevere F. Eden, ZWRlbmdAZ2VvcmdldG93bi5lZHU=
†Present address: Anthony J. Krafnick, Department of Psychology, Dominican University, River Forest, IL, United States