- 1Department of Psychiatry and National Clinical Research Center for Mental Disorders, The Second Xiangya Hospital of Central South University, Changsha, China
- 2Department of Radiology, Tianjin Medical University General Hospital, Tianjin, China
- 3Department of Radiology, The Second Xiangya Hospital of Central South University, Changsha, China
- 4Department of Psychiatry, Qiqihar Medical University, Qiqihar, China
- 5Department of Psychiatry, The Third People’s Hospital of Foshan, Foshan, China
Objective: Gastrointestinal (GI) symptoms are prominent in major depressive disorder (MDD) and bring patients lots of complaints and troubles. We aimed to explore whether there were some distinctive brain image alterations in MDD with GI symptoms, which could be used to distinguish MDD with GI symptoms from those without GI symptoms and healthy controls (HCs).
Methods: A total of 35 outpatients with GI symptoms, 17 outpatients without GI symptoms, and 28 HCs were recruited. All the participants were scanned by a resting-state functional magnetic resonance imaging. Imaging data were analyzed with the global functional connectivity (GFC) and support vector machine methods.
Results: MDD with GI symptoms showed decreased GFC in the left superior medial prefrontal cortex (MPFC) compared with MDD without GI symptoms. Compared with HCs, MDD with GI symptoms showed decreased GFC in the bilateral middle temporal pole (MTP) and left posterior cingulate cortex/precuneus (PCC/Pcu), and increased GFC in the right insula and bilateral thalamus. SVM analysis showed that an accuracy was 78.85% in differentiating MDD with GI symptoms from MDD without GI symptoms by using the GFC of the left superior MPFC. A combination of GFC of the left PCC/Pcu and bilateral MTP exhibited the highest accuracy (87.30%) in differentiating patients with MDD with GI symptoms from HCs.
Conclusion: MDD with GI symptoms showed abnormal GFC in multiple networks, including the default mode network and cortico-limbic mood-regulating circuit. Using abnormal GFC might work well to discriminate MDD with GI symptoms from MDD without GI symptoms and HCs.
Introduction
In addition to the main psychological symptoms (such as persistently depressed mood state), major depressive disorder (MDD) often appears with medically unexplained somatic symptoms (Jain, 2009). Among these, gastrointestinal (GI) symptoms are prominent which bring patients with MDD a lot of complaints and troubles. A literature review (Lépine and Briley, 2004) supported that pain led to depression which in turn increased sensitivity to pain, which indicated that there might be bidirectional influences between MDD and GI symptoms (Karling et al., 2007). Besides, patients with somatic symptoms seemed to undergo more difficult and less effective treatment than patients without somatic symptoms. Some previous studies have shown that somatic symptoms were associated with exacerbation of clinical symptoms, low remission rates (Novick et al., 2013), and poor prognoses (Bekhuis et al., 2016) in patients with MDD. Nevertheless, in outpatient services, general physicians experienced hardly to recognize mental disorders such as MDD, and the chief complaint of somatic symptoms added a more difficult identification (Jain, 2009), which was often referred as to “masked depression” (Verster and Gagiano, 1995). Consequently, patients with MDD with GI symptoms underwent chronic lack of proper diagnoses and effective treatments (Kirmayer et al., 1993; García-Campayo et al., 2008). The economic burden increased because of more healthcare resources utilization during episodes of MDD (Painchault et al., 2014). It would result in an increasing medical cost of MDD and additional waste of medical resources (García-Campayo et al., 2008) when they constantly sought medical help. In the context of a global scarcity of psychiatrists and other mental health resources (Smith, 2014) increased consumption of medical resources caused by the failure to get a correct diagnosis undoubtedly undermines the rational allocation of medical resources. Thus, effective and proper identifications of MDD with GI symptoms are of great significance for the prognosis of MDD and the rational medical resource allocation.
However, the mechanism of GI symptoms in MDD remains unclear. Brain imaging techniques were greatly applied to research the brain image abnormalities in many mental disorders, by using different methods such as gray matter volume (GMV), network homogeneity (NH), functional connectivity (FC), and regional homogeneity (ReHo). Brain structural and functional aberrations were found in patients with MDD with GI symptoms based on literature reviews, such as altered GMV and ReHo (Yan et al., 2019; Liu et al., 2020). But, there is a lack of research into connections between spatially distant brain regions. Current evidence indicated that MDD exhibited abnormal resting-state FC in discrete brain networks, especially in the default-mode network (DMN) and cortico-limbic mood-regulating circuit (MRC) (prefrontal-amygdalar-pallidostriatal-mediothalamic MRC) (Wang et al., 2012). The DMN was regarded as a critical role in gastric sensations (Skrobisz et al., 2020) and functional abnormalities were reported in digestive diseases (Fan et al., 2019). Thus, it was curious to see whether FC of these areas was similarly altered in patients with MDD with GI symptoms. To avoid bias of the pre-selection of seeds in the seed-based regions of interest (ROI) methods and face the controversial issue of the number of independent components in the independent component analysis (ICA) approach (Kelly et al., 2012), a model-free voxel-wised global functional connectivity (GFC) method was applied in the present study with a less biased way (Cui et al., 2018; Ding et al., 2019; Pan et al., 2019).
Taken together, we aimed to explore the GFC differences across patients with MDD with GI symptoms, patients with MDD without GI symptoms and healthy controls (HCs) in the present study. We hypothesized that patients with MDD with GI symptoms would show abnormal GFC in multiple networks, especially in the DMN or cortico-limbic MRC. And it was expected that the altered GFC would correlate with clinical characteristics of patients with MDD with GI symptoms. In addition, the support vector machine (SVM) method was used to detect whether GFC values in these abnormal brain regions might be potential image markers to distinguish patients with MDD with GI symptoms from those without GI symptoms and HCs.
Materials and Methods
Participants
A total of 35 outpatients with one or more GI symptoms (GI symptoms group) and 17 outpatients without any GI symptoms (non-GI symptoms group) were recruited. GI symptoms mainly included medically unexplained nausea, vomiting, gastralgia (stomachache), gastric distention, constipation, diarrhea, heartburn, acid reflux. All of them were 18–55 years old outpatients from the Second Xiangya Hospital of Central South University, China. They received their final diagnoses independently by two psychiatrists according to the DSM-5 criteria. Inclusion criteria were: (1) first time of major depressive episode; (2) the 17-item Hamilton Rating Scale for Depression (HRSD-17) (Hamilton, 1967) total scores ≥ 17; (3) with no relative history of medication and physical therapy (such as repetitive transcranial magnetic stimulation, electroconvulsive therapy); (4) with no confirmed digestive tract diseases. The severity of MDD was evaluated by using the HRSD-17. The HRSD-17 can be divided into the following five types of factors: (1) anxiety/somatization (six items of psychic anxiety, somatic anxiety, gastrointestinal symptoms, hypochondriasis, insight, and general symptoms); (2) weight loss (one item); (3) cognitive disturbances (three items of self-guilt, suicide, and agitation); (4) retardation symptoms (four items of depression, work and interests, retardation, and sexual symptoms); (5) sleep disturbances (three items of difficulty falling asleep, superficial sleep, and early awakening).
A total of 28 HCs were recruited from the community, demographically matched (on age, gender, and education) with the patients. Anyone with a suspicious or explicit history of mental disorders running in the family would be excluded, same as any history of digestive tract diseases, neurological diseases, substance abuse, or psychotic symptoms (such as hallucination and delusion, etc.).
All the participants were right-handed and Han Chinese. Exclusion criteria for all the participants are as follows: (1) other mental disorders meeting DSM-5 criteria; (2) with any history of neurological diseases, severe physical illnesses, and substance abuse; (3) be pregnant; (4) with structural abnormalities of the brain; (5) and with any contraindications to MRI scanning.
The study was approved by the Medical Research Ethics Committee of the Second Xiangya Hospital of Central South University, China. It was conducted in accordance with the Helsinki Declaration. Each participant has submitted a written informed consent before enrollment.
Imaging Acquisition and Data Preprocessing
All the participants underwent a 3.0 T Siemens scanner (Germany) resting-state MRI scan in the Second Xiangya Hospital of Central South University. Initial brain image data were obtained using the echo planar imaging (EPI) sequence. The specific parameters were as follows: repetition time/echo time (TR/TE) 2,000/30 ms, 30 slices, 64 × 64 matrix, 90°flip angle, 24 cm field of view, 4 mm slice thickness, 0.4 mm gap, and 250 volumes lasting for 500 s.
Data preprocessing was finished by using DPABI in Matlab (Yan et al., 2016). The first 10 fMRI images data were discarded to avoid the influence of potential unstable factors, such as unstable initial MRI signals and adaptation time of subjects. Then, slice timing and head motion corrections were performed on the images. The maximum displacement on the x, y, or z axis was 2 mm and the maximum angular rotation was 2°. After that, the corrected images were spatially normalized to the MNI space with 3 mm × 3 mm × 3 mm. Next, the fMRI data were filtered by temporal band-pass (0.01–0.08 Hz) and linearly detrended. Some suspicious pseudo covariates were removed, such as signals from the center region of white matter and region of interest (ROI) based on ventricular seeds, the 24-head motion parameters obtained by the rigid body correction. The global signal was not removed in the present study (Hahamy et al., 2014).
Global Functional Connectivity Analysis
The specific analysis process was consistent with our previous studies (Cui et al., 2018; Ding et al., 2019; Pan et al., 2019). For each participant, voxel-wise GFC (FC between a given voxel and all the other voxels) was calculated within a gray matter mask in Matlab. The gray matter mask was generated in SPM8 at a threshold of probability > 0.2 (Liu et al., 2015). Pearson correlation coefficients (r) between time series of all pairs of voxels were calculated and then converted to z values using the Fisher r-to-z to transformation (Wang et al., 2015). The GFC of a voxel was the mean coefficient of this given voxel with all the other voxels. By composing the GFC of all voxels, the GFC maps were generated.
Statistical Analyses
Analysis of variance (ANOVA) was used to compare demographic data (age, years of education) and clinical data (HRSD-17 scores, and five factor scores of HRSD-17) across the three groups. A chi-square test was performed to describe gender distribution and differences in illness duration between the two patient groups were compared by using a two-sample t-test. P < 0.05 was considered statistically significant.
The frame-wise displacement (FD) value was calculated for each participant (Power et al., 2012). The group differences were identified by performing an analysis of covariance (ANCOVA) in individual whole-brain GFC maps across the three groups, followed by post hoc t-tests. Age, gender, years of education, and FD were applied as covariates. The results were false discovery rate (FDR) corrected at p < 0.05.
Correlation Analyses
We extracted the mean z values from brain regions with abnormal GFC. The correlations between abnormal GFC values and HRSD-17 scores as well as the five factor scores were assessed by the Pearson’s correlation analysis with a threshold of the Benjamini–Hochberg corrected p < 0.05.
Classification Analyses
Using the SVM method, the LIBSVM software package1 was conducted in MATLAB to verify the feasibility of using GFC values of abnormal brain regions to distinguish MDD with GI symptoms from those without GI symptoms and HCs. The approach of “leave-one-out” was applied in the study.
Results
Demographic and Clinical Characteristics
No group differences were observed in age, years of education, and gender distribution across the three groups. The two patient groups did not differ in illness durations. The HRSD-17 total and factor scores (excepting weight loss) of the two patient groups were all higher than those of HCs. The GI group showed significantly higher weight loss scores than both non-GI group and HCs, whereas these two groups showed no significant difference in weight loss scores. Besides, the HRSD-17 total scores, factor scores of anxiety/somatization, weight loss, and sleep disturbances of the GI group were higher than those of the non-GI group (Table 1).
Global Functional Connectivity Differences Across Groups
As shown in Figure 1, the three groups showed significant differences mainly in the frontal, temporal, insular, and limbic areas.
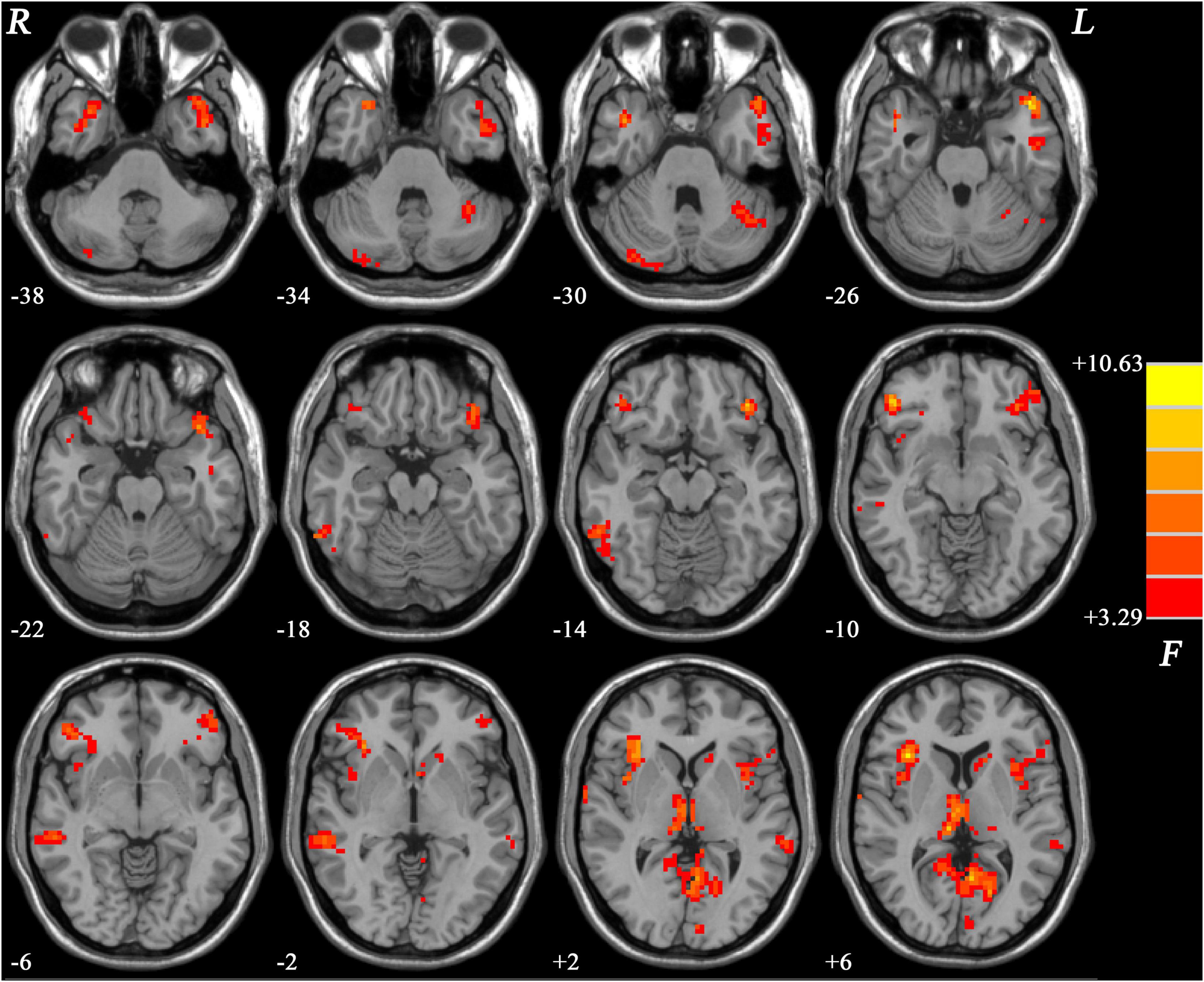
Figure 1. Brain regions showing group differences of GFC values across the three groups. Color bar indicates F values from ANCOVA (age, gender, years of education and framewise displacement as covariates). GFC, global functional connectivity; ANCOVA, analysis of covariance.
Major Depressive Disorder With Gastrointestinal Symptoms Versus Major Depressive Disorder Without Gastrointestinal Symptoms
Patients with MDD with GI symptoms showed lower GFC in the left superior medial prefrontal cortex (MPFC) than that in patients with MDD without GI symptoms (Table 2 and Figure 2). No higher GFC was observed in the group comparisons.
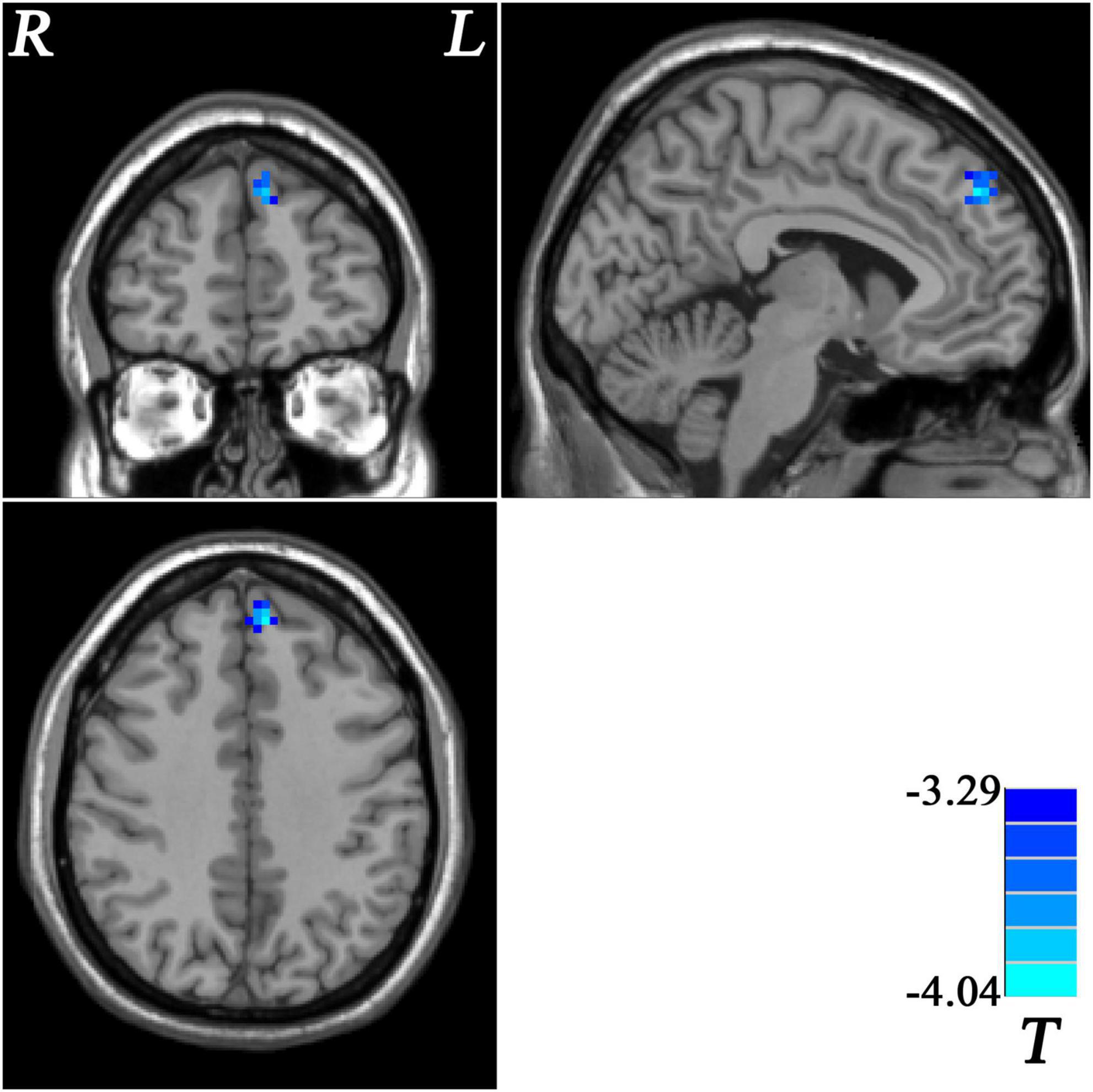
Figure 2. Statistical map depicts lower GFC of MDD patients with GI symptoms compared with MDD patients without GI symptoms. Blue denotes lower GFC. Color bar indicates T values from two-sample t-test. MDD, major depressive disorder; GFC, global functional connectivity; GI, gastrointestinal symptoms.
Major Depressive Disorder With Gastrointestinal Symptoms Versus Healthy Controls
Patients with MDD with GI symptoms showed decreased GFC in the bilateral middle temporal pole (MTP) and left posterior cingulate cortex/precuneus (PCC/Pcu), and increased GFC in the right insula and bilateral thalamus, compared with HCs (Table 2 and Figure 3).
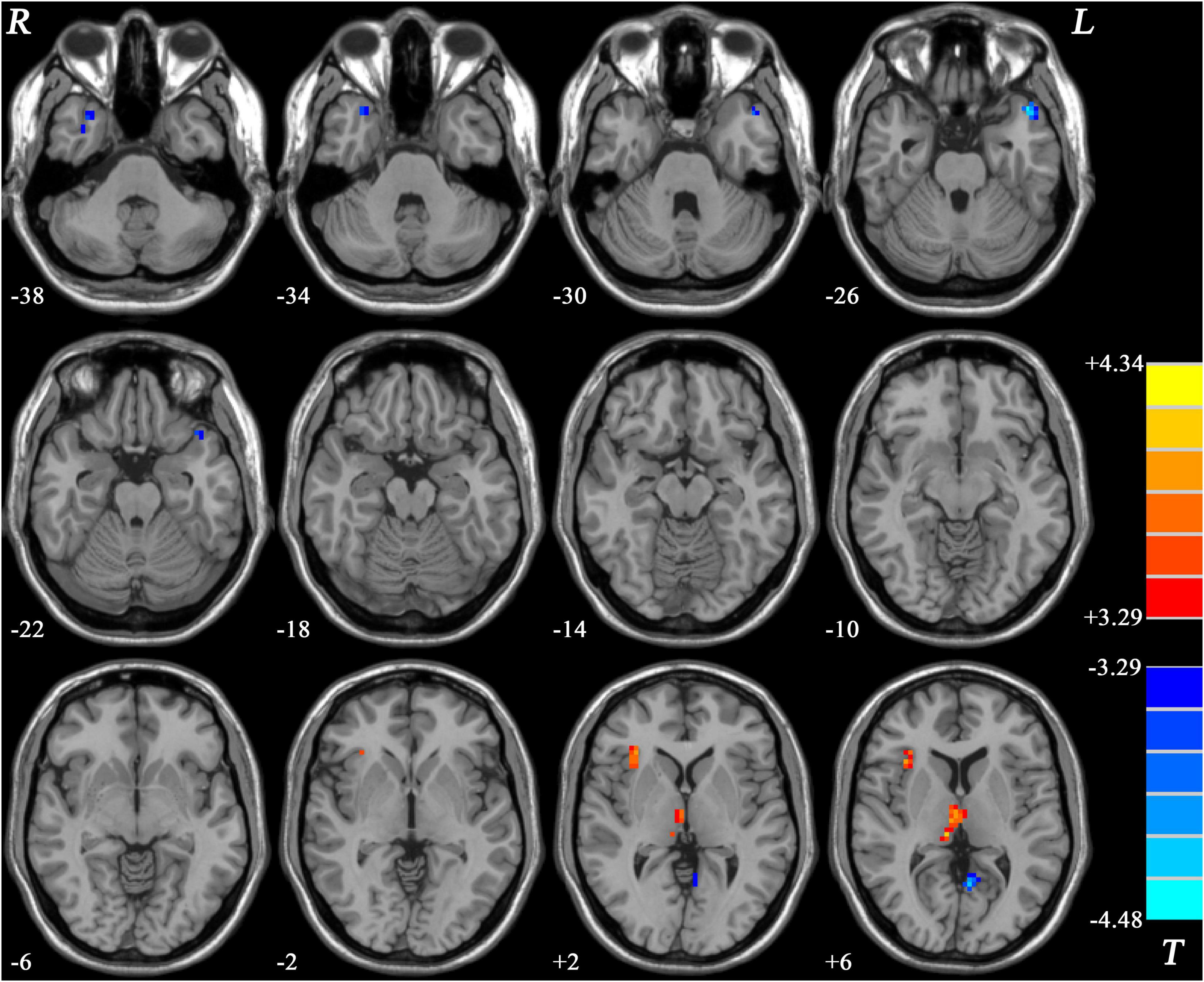
Figure 3. Statistical map depicts higher and lower GFC of MDD patients with GI symptoms compared with healthy controls. Blue denotes lower GFC and red denotes higher GFC. Color bar indicates T values from two-sample t-test. MDD, major depressive disorder; GFC, global functional connectivity; GI, gastrointestinal symptoms.
Major Depressive Disorder Without Gastrointestinal Symptoms Versus Healthy Controls
Compared with HCs, patients with MDD without GI symptoms showed decreased GFC in the left PCC/Pcu and increased GFC in the right inferior frontal gyrus (IFG) (Table 2 and Figure 4).
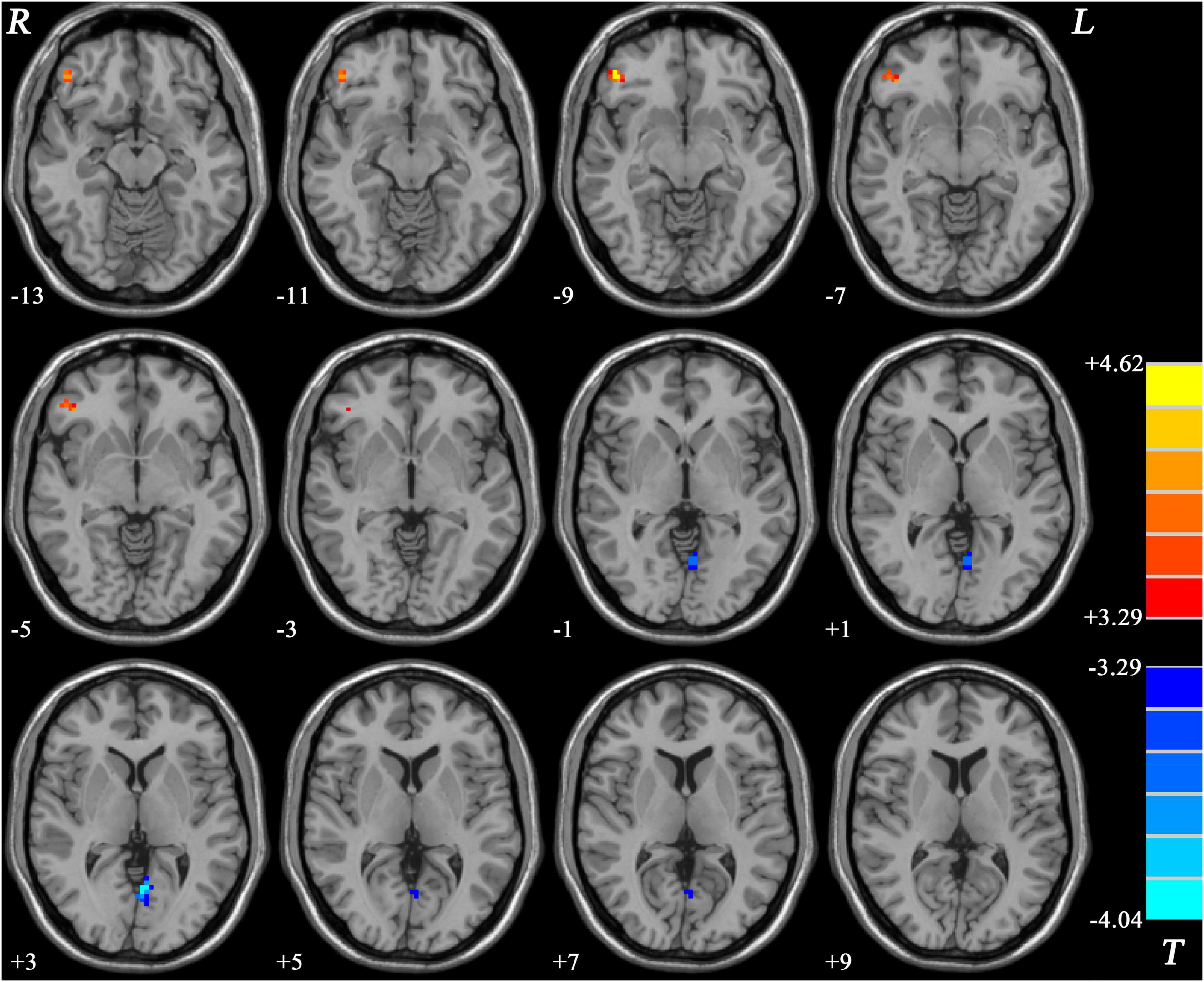
Figure 4. Statistical map depicts higher and lower GFC of MDD patients without GI symptoms compared with healthy controls. Blue denotes lower GFC and red denotes higher GFC. Color bar indicates T values from two-sample t-test. MDD, major depressive disorder; GFC, global functional connectivity; GI, gastrointestinal symptoms.
Classification Analyses
Major Depressive Disorder With and Without Gastrointestinal Symptoms
The results of SVM analysis showed that the accuracy, sensitivity, and specificity were 78.85% (41/52), 85.71% (30/35), and 64.71% (11/17) respectively, in differentiating patients with MDD with GI symptoms from patients with MDD without GI symptoms by using abnormal GFC values of the left superior MPFC (Figure 5).
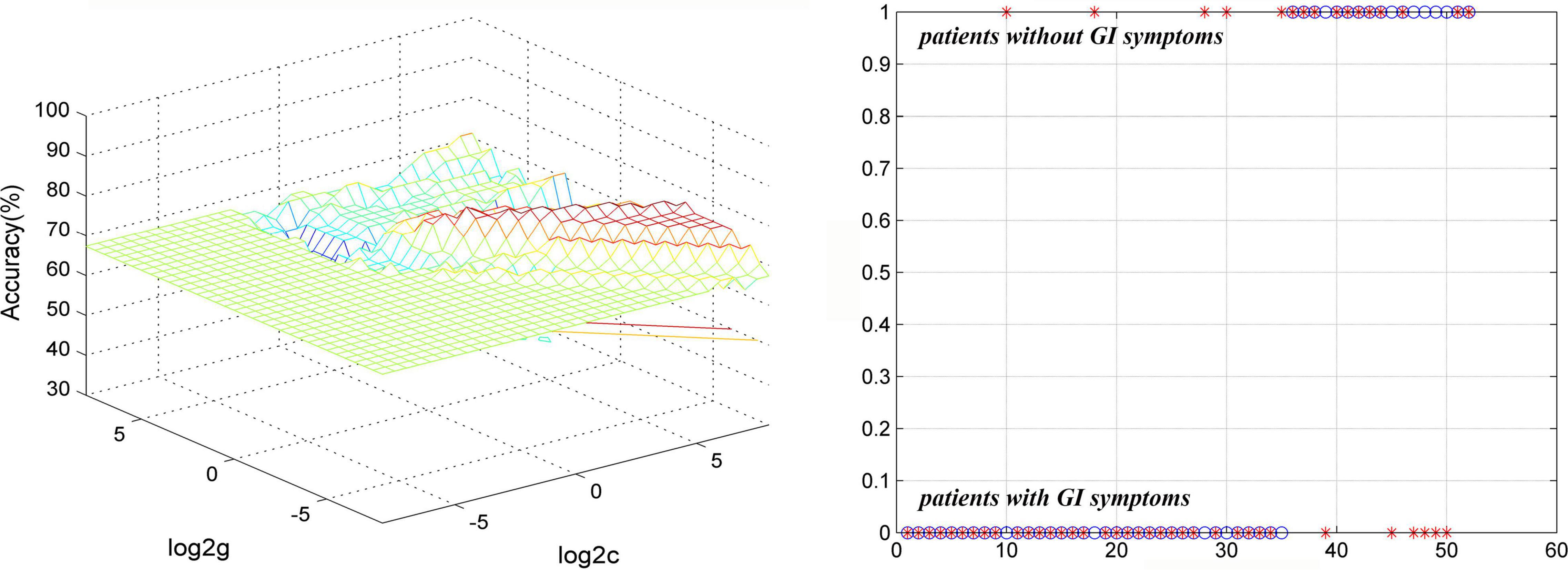
Figure 5. Visualization of classifications through SVM using abnormal GFC values of the left superior MPFC to discriminate MDD with and without GI symptoms. (Left) SVM parameters result of 3D view. (Right) Classified map of GFC of the left superior MPFC. SVM, support vector machine; GFC, global functional connectivity; MPFC, medial prefrontal gyrus; MDD, major depressive disorder; GI, gastrointestinal symptoms.
Major Depressive Disorder With Gastrointestinal Symptoms and Healthy Controls
The results of SVM analysis showed that a combination of GFC of the left PCC/Pcu and bilateral MTP exhibited the highest accuracy (87.30%, 55/63), sensitivity (85.71%, 30/35), and specificity (89.29%, 25/28) in differentiating patients with MDD with GI symptoms from HCs (Figure 6).
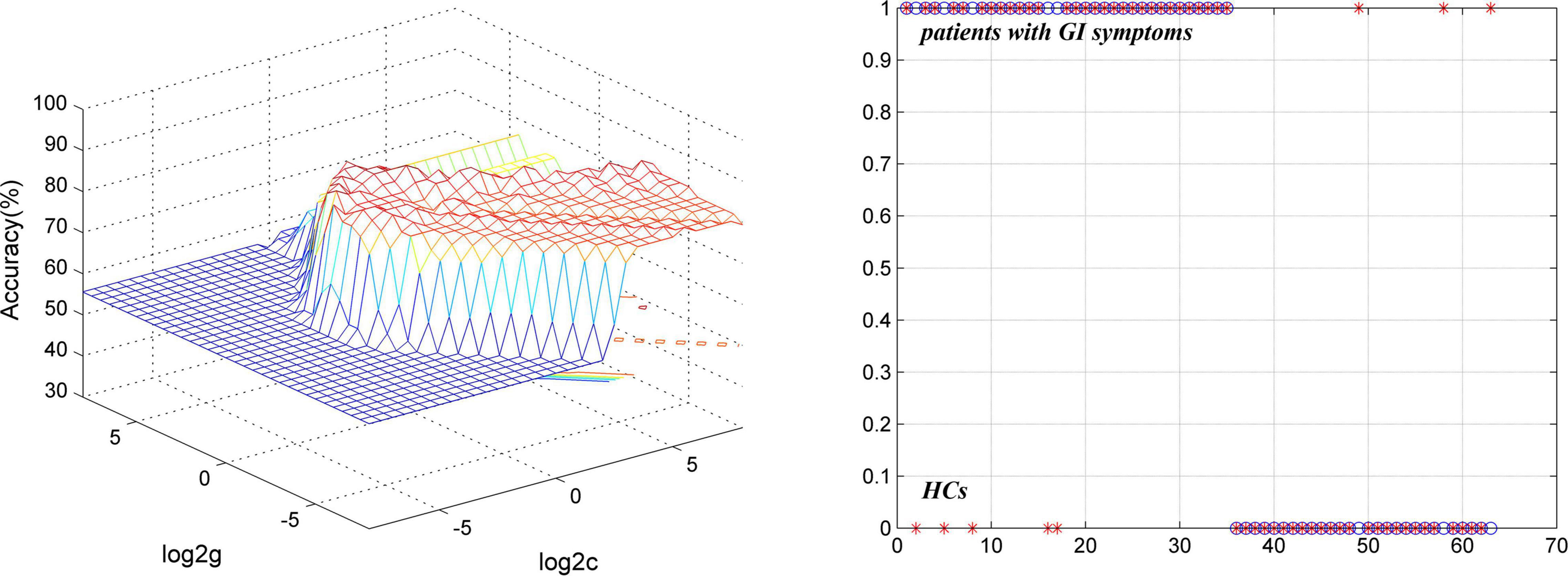
Figure 6. Visualization of classifications through SVM using a combination of GFC values of the left PCC/Pcu and bilateral MTP to discriminate MDD with GI symptoms and HCs. (Left) SVM parameters result of 3D view. (Right) Classified map of a combination of GFC of the left PCC/Pcu and bilateral MTP. SVM, support vector machine; GFC, global functional connectivity; HCs, healthy controls; PCC/PCu, posterior cingulate cortex/precuneus; MTP, middle temporal pole; MDD, major depressive disorder; GI, gastrointestinal symptoms.
Correlations Between Global Functional Connectivity and Clinical Characteristics
For all the patients, GFC of the left superior MPFC was negatively correlated with weight loss scores (r = –0.460, p = 0.001, the Benjamini–Hochberg correction p = 0.006).
For MDD with GI symptoms, GFC of the left superior MPFC was negatively correlated with weight loss scores (r = –0.450, p = 0.007, the Benjamini–Hochberg correction p = 0.021) and positively correlated with retardation symptoms scores (r = 0.464, p = 0.005, the Benjamini–Hochberg correction p = 0.03). GFC in the left MTP was negatively correlated with total HRSD-17 scores (r = –0.412, p = 0.014, the Benjamini–Hochberg correction p = 0.042) and increased GFC of MDD with GI symptoms in the bilateral thalamus was positively correlated with retardation symptoms (r = 0.498, p = 0.002, the Benjamini–Hochberg correction p = 0.012). There were no significant correlations between GFC of the right MTP, right insula, and left PCC/Pcu, and clinical characteristics after the Benjamini–Hochberg correction (see more details in Supplementary Table).
For MDD without GI symptoms, GFC of the left superior MPFC was positively correlated with weight loss scores (r = 0.687, p = 0.002, the Benjamini–Hochberg correction p = 0.012). There were no significant correlations between GFC of both the left PCC/Pcu and right IFG and clinical characteristics after the Benjamini–Hochberg correction (see more details in Supplementary Table).
Discussion
In this study, the GI group showed more severe depressive symptoms than non-GI group, especially in the terms of anxiety/somatization, weight loss, and sleep disturbances. We observed that GI group showed decreased GFC in the left PCC/Pcu and bilateral MTP and increased GFC in the thalamic and insular areas compared with HCs. And the GI group showed decreased GFC in the left superior MPFC than non-GI group. Good classification results were shown in differentiating GI group from non-GI group and HCs by using SVM analyses.
In addition to the gut microbiota, the brain also regulates food intake through homeostatic control, mood, and cognition (Torres-Fuentes et al., 2017; Milaneschi et al., 2019). Hypothalamus, insula, amygdala, and other limbic and cortical areas participate in the complex processes (Behary and Miras, 2014). During the communication between the central nervous system and peripheral biological signals, the parasympathetic innervation of vagus nerves acts as a vital coordinating role, and vagal nerve blockade may be a surgical therapy in obesity in clinical (Browning et al., 2017). A previous study reported that the cortical neurons that influenced parasympathetic functions were overwhelmingly in the MPFC and insula (Levinthal and Strick, 2020). Thus, dysfunctions in these brain regions may influence body weight by the abovementioned pathways in MDD. In MDD with GI symptoms, function alterations of these brain regions may further affect these processes and lead to weight loss.
Previous studies indicated that somatic symptoms were correlated with more severe clinical symptoms in MDD (Novick et al., 2013) and MDD with GI symptoms showed more severe depression (Liu et al., 2020). Consistent with these studies, the HRSD-17 total scores, factor scores of anxiety/somatization, weight loss and sleep disturbances of GI group were all higher than those of non-GI group which indicated that patients with MDD with GI symptoms showed more severe depressive symptoms than those without GI symptoms. As noted previously, patients with MDD with GI symptoms underwent a prolonged lack of proper diagnoses and experienced lower remission rates and poorer prognoses. Therefore, early detection of patients with MDD with GI symptoms as their chief complaint and aggressive treatments of their depressive symptoms and GI symptoms may help improve the remission rate and positively impact their prognosis.
As one of the core regions of the DMN and part of cortico-limbic MRC, MPFC has attracted a lot of attention in recent years (Raichle, 2015). Involved in a variety of self-referencing, affective, and cognitive functions (such as perceptual memory and executive control) (Lemogne et al., 2012; Schwiedrzik et al., 2018; Lieberman et al., 2019), MPFC performs as a part of the central stress circuit, participating in the process of stress response (Bhatia and Tandon, 2005). In the neuroscience researches, MPFC showed neuroplasticity impairments under chronic stress and depressive-like behaviors (Duman and Aghajanian, 2012; Abdallah et al., 2015; Duman et al., 2016) and similar phenomena existed in researches of cognitive science and clinical psychology level (Price and Duman, 2020). Stress could have both short-term and long-term influences on gastrointestinal tract function and GI symptoms are physical reactions to stressful and emotional conditions (Bhatia and Tandon, 2005). Thus, the function of the MPFC might be associated with depressive symptoms, as well as GI symptoms. At the same time, structural changes in the MPFC may occur with the onset of somatic symptoms, such as gray matter loss in the MPFC, which has been consistently reported to correlate with chronic pain stress (Kang et al., 2019). Previous studies indicated that stress (whatever acute or chronic stress) may induce structural and functional impairments in the MPFC (Bhatia and Tandon, 2005; Wellman and Moench, 2019). These findings suggest that the decrease of GFC in the MPFC in the GI group may be related to the structural changes such as gray matter loss. In addition, portions of the MPFC influenced parasympathetic output to the stomach and related prefrontal neurons were in the left hemisphere more than three times as in the right hemisphere (Levinthal and Strick, 2020), which was consistent with the finding that microstimulation in this region could induce alterations in the gastric function (Hurley-Gius and Neafsey, 1986). Therefore, we speculate that the structure and function of the MPFC may be altered in the vicious cycle of recurrent bad mood and GI symptoms. In the present study, patients with MDD with GI symptoms showed lower GFC in the left superior MPFC than that in patients with MDD without GI symptoms, which may show that decreased GFC in the MPFC might be correlated with the onset of GI symptoms in MDD. Besides, we observed that decreased GFC of MDD with GI symptoms in the left superior MPFC was negatively correlated with weight loss scores and positively correlated with retardation symptoms scores. These further confirmed the important role of the MPFC in MDD with GI symptoms.
A previous study has reported that the volume of the middle temporal gyrus (MTG) was strongly associated with deficits in language and reasoning ability, and both were the state- and trait-related markers of depression (Ang et al., 2020). In our previous studies, we found that melancholic MDD showed lower NH in the bilateral MTG than HCs, especially in the right MTG/MTP (Cui et al., 2017; Yan et al., 2021). Decreased ReHo in the right MTG was also reported in remitted geriatric depression (Yuan et al., 2008). All these findings might reflect the MTG changes as one state characteristic of depression. Consistent with these studies, we observed that patients with MDD with GI symptoms showed decreased GFC in the bilateral MTP compared with HCs in the present study, and decreased GFC in the left MTP was negatively correlated with the severity of depression. Apart from language and memory functions (Squire and Zola-Morgan, 1991; Squire et al., 2004; Baxter, 2009; Friederici and Gierhan, 2013), the temporal lobe has been proposed to be involved in endogenous attention control by one special node and this node was directly connected to the frontal and parietal attention control regions (Sani et al., 2021). PCC has been proposed to be involved in controlling attention by connecting to the frontal lobe (Leech and Sharp, 2014). But whether there is any link between these two regions is unknown. As one of the components of the DMN, PCC/Pcu has attracted much attention in many studies of MDD (Raichle, 2015). Many previous studies have reported decreased activity in the PCC/Pcu in MDD, such as decreased FC, voxel-mirrored homotopic connectivity (VMHC) and ReHo (Bluhm et al., 2009; Chen et al., 2012; Zhu et al., 2012; Guo et al., 2013). Consistently, we observed that both two patient with MDD groups showed decreased GFC in the left PCC/Pcu compared with HCs in the present study. Although it is unclear whether there is a link between the temporal lobe and PCC in attentional control function, we concurrently observed GFC changes in both two brain regions in MDD. Therefore, we speculate that abnormalities in both two brain regions are associated with attention-deficit-induced cognition impairments in MDD.
By direct electrical stimulation of the insula cortex, a variety of visceral responses (containing gastric sensory or motor phenomena, such as nausea, vomiting, belching) and somatic sensation responses were elicited (Penfield and Faulk, 1955; Afif et al., 2010), which promoted the insula to be seen as a primarily visceral–somatic region (Uddin et al., 2017). Besides, cortical neurons that participated in the parasympathetic control of the stomach were overwhelmingly located in the insula (Levinthal and Strick, 2020). Therefore, GI symptoms may occur when the structure or function of the insula were aberrant. For instance, the insula has been commonly found to be involved in the pathophysiologic mechanism of functional dyspepsia (FD) (Van Oudenhove et al., 2010; Zeng et al., 2011; Liu et al., 2018). In addition, the insula has been reported to be associated with abnormal visceral sensitivity in irritable bowel syndrome (IBS) (Icenhour et al., 2017). Thalamus, as the “relay station” of the brain, is recognized as one of the key roles in gastrointestinal sensory processing, because primary interoceptive information is usually afferent to the thalamus, and then it will act as a “gateway” to relay signals to the insula (Zhou et al., 2013; Iannilli et al., 2014; Liu et al., 2018). A previous study observed that patients with FD showed lower connection probability in the right anterior insula with the right thalamus and decreased functional connectivity of them (Liu et al., 2018). Thus, the structure and function of the insula and thalamus may work in the process of the onset and sensory transmission of GI symptoms in MDD. In addition to the “visceral–somatic” functions mentioned earlier, the insula is involved in cognitive control and emotional processes by connection with higher-level cortices and limbic areas (Deen et al., 2011; Chang et al., 2013). Besides, the thalamus, one part of the cortico-limbic MRC, is widely recognized to be involved in the process of attention control, and thus affects cognition (Saalmann and Kastner, 2011; Halassa and Kastner, 2017). And it has been reported that attention deficit was associated with the clinical psychomotor retardation in MDD (Lemelin and Baruch, 1998). To some extent, this is consistent with the findings of the present study that increased GFC in the bilateral thalamus in the GI group was positively correlated with retardation symptoms. Structural and functional changes in the insula and thalamus were reported in many previous studies of MDD (Wise et al., 2017; Peng et al., 2018; Wang et al., 2018; Zhang et al., 2018; Hu et al., 2019). In the present study, we observed that MDD with GI symptoms showed increased GFC in the right insula and bilateral thalamus compared with HCs. And this may account for many of the differences in the clinical manifestations between the GI group and HCs. Reviewing all the aforementioned previous studies, the complexity of the neuropsychiatric system function is well demonstrated.
No less than 60% of the sensitivity or specificity is required for an eligible diagnostic indicator and more than 70% is conducive to the establishment of diagnostic indicators (Swets, 1988; Gong et al., 2011). Thus, results of SVM analysis in differentiating patients with MDD with GI symptoms from those without GI symptoms by using GFC of the left superior MPFC were moderate. And results of SVM analysis in distinguishing patients with MDD with GI symptoms and HCs by using a combination of GFC of the left PCC/Pcu and bilateral MTP were relatively effective. Therefore, we could try to use this method to discriminate MDD with GI symptoms from MDD without GI symptoms and HCs in our clinical work under the dilemma that we can only diagnose through subjective experience and lack objective indicators. However, the clinical symptoms of many patients are complex and there are many confounding factors, so the application scope of this method may be limited.
A previous study indicated that MDD was sensitive to the grading of spectra across resting-state networks (Ries et al., 2018). And Yang et al. (2021) found abnormal FC density in some brain networks in both lower and higher frequency bands in the bipolar disorder during depressive episodes. Thus, whether there are frequency-specific FC changes in MDD with GI symptoms needs further exploration. There are also some limitations. The sample size is small, so the generalization of the research results is limited to some extent. Furthermore, we did not subdivide each of the different GI symptoms because we assumed that they had similar mechanisms. Therefore, we did not know whether different symptoms made different outcomes.
Conclusion
The present study found that patients with MDD with GI symptoms showed abnormal GFC in multiple networks, including the DMN and cortico-limbic MRC, which suggested that these brain regions may play an important role in the biological mechanisms of GI symptoms in MDD. Abnormal GFC values were correlated with some clinical characteristics. Besides, we observed that MDD with GI symptoms demonstrated more severe depressive symptoms than MDD without GI symptoms. Early identification of patients with MDD with GI symptoms as their chief complaint and aggressive treatments of their depressive symptoms and GI symptoms may help improve the remission rate and positively impact their prognosis. In our clinical practice, it might work well to discriminate MDD with GI symptoms from HCs by using a combination of GFC of the left PCC/Pcu and bilateral MTP.
Data Availability Statement
The raw data supporting the conclusions of this article will be made available by the authors, without undue reservation.
Ethics Statement
The studies involving human participants were reviewed and approved by the Second Xiangya Hospital of Central South University, China. The patients/participants provided their written informed consent to participate in this study.
Author Contributions
MY, XF, and WG contributed to the conception and design of the study. YO, XF, and HL contributed to the data collections. MY, FL, and WG performed the data analysis. MY wrote the manuscript. All authors contributed to manuscript revision, read, and approved the submitted version.
Funding
This study was supported by grants from the National Natural Science Foundation of China (Grant No. 82171508), the Natural Science Foundation of Hunan (Grant No. 2020JJ4784), the Science and Technology Program of Hunan Province (Grant No. 2020SK53413), and the Natural Science Foundation of Tianjin (Grant No. 18JCQNJC10900).
Conflict of Interest
The authors declare that the research was conducted in the absence of any commercial or financial relationships that could be construed as a potential conflict of interest.
Publisher’s Note
All claims expressed in this article are solely those of the authors and do not necessarily represent those of their affiliated organizations, or those of the publisher, the editors and the reviewers. Any product that may be evaluated in this article, or claim that may be made by its manufacturer, is not guaranteed or endorsed by the publisher.
Acknowledgments
We thank all the participants who served as research participants.
Supplementary Material
The Supplementary Material for this article can be found online at: https://www.frontiersin.org/articles/10.3389/fnins.2022.897707/full#supplementary-material
Footnotes
References
Abdallah, C. G., Sanacora, G., Duman, R. S., and Krystal, J. H. (2015). Ketamine and rapid-acting antidepressants: a window into a new neurobiology for mood disorder therapeutics. Annu. Rev. Med. 66, 509–523. doi: 10.1146/annurev-med-053013-062946
Afif, A., Minotti, L., Kahane, P., and Hoffmann, D. (2010). Anatomofunctional organization of the insular cortex: a study using intracerebral electrical stimulation in epileptic patients. Epilepsia 51, 2305–2315. doi: 10.1111/j.1528-1167.2010.02755.x
Ang, Y. S., Frontero, N., Belleau, E., and Pizzagalli, D. A. (2020). Disentangling vulnerability, state and trait features of neurocognitive impairments in depression. Brain 143, 3865–3877. doi: 10.1093/brain/awaa314
Baxter, M. G. (2009). Involvement of medial temporal lobe structures in memory and perception. Neuron 61, 667–677. doi: 10.1016/j.neuron.2009.02.007
Behary, P., and Miras, A. D. (2014). Brain responses to food and weight loss. Exp. Physiol. 99, 1121–1127. doi: 10.1113/expphysiol.2014.078303
Bekhuis, E., Boschloo, L., Rosmalen, J. G., de Boer, M. K., and Schoevers, R. A. (2016). The impact of somatic symptoms on the course of major depressive disorder. J. Affect. Disord. 205, 112–118. doi: 10.1016/j.jad.2016.06.030
Bhatia, V., and Tandon, R. K. (2005). Stress and the gastrointestinal tract. J. Gastroenterol. Hepatol. 20, 332–339. doi: 10.1111/j.1440-1746.2004.03508.x
Bluhm, R., Williamson, P., Lanius, R., Théberge, J., Densmore, M., Bartha, R., et al. (2009). Resting state default-mode network connectivity in early depression using a seed region-of-interest analysis: decreased connectivity with caudate nucleus. Psychiatry Clin. Neurosci. 63, 754–761. doi: 10.1111/j.1440-1819.2009.02030.x
Browning, K. N., Verheijden, S., and Boeckxstaens, G. E. (2017). The vagus nerve in appetite regulation, mood, and intestinal inflammation. Gastroenterology 152, 730–744. doi: 10.1053/j.gastro.2016.10.046
Chang, L. J., Yarkoni, T., Khaw, M. W., and Sanfey, A. G. (2013). Decoding the role of the insula in human cognition: functional parcellation and large-scale reverse inference. Cereb. Cortex 23, 739–749. doi: 10.1093/cercor/bhs065
Chen, J. D., Liu, F., Xun, G. L., Chen, H. F., Hu, M. R., Guo, X. F., et al. (2012). Early and late onset, first-episode, treatment-naive depression: same clinical symptoms, different regional neural activities. J. Affect. Disord. 143, 56–63. doi: 10.1016/j.jad.2012.05.025
Cui, X., Guo, W., Wang, Y., Yang, T. X., Yang, X. H., Wang, Y., et al. (2017). Aberrant default mode network homogeneity in patients with first-episode treatment-naive melancholic depression. Int. J. Psychophysiol. 112, 46–51. doi: 10.1016/j.ijpsycho.2016.12.005
Cui, X., Liu, F., Chen, J., Xie, G., Wu, R., Zhang, Z., et al. (2018). Voxel-wise brain-wide functional connectivity abnormalities in first-episode, drug-naive patients with major depressive disorder. Am. J. Med. Genet. B Neuropsychiatr. Genet. 177, 447–453. doi: 10.1002/ajmg.b.32633
Deen, B., Pitskel, N. B., and Pelphrey, K. A. (2011). Three systems of insular functional connectivity identified with cluster analysis. Cereb. Cortex 21, 1498–1506. doi: 10.1093/cercor/bhq186
Ding, Y., Ou, Y., Su, Q., Pan, P., Shan, X., Chen, J., et al. (2019). Enhanced global-brain functional connectivity in the left superior frontal gyrus as a possible endophenotype for schizophrenia. Front. Neurosci. 13:145. doi: 10.3389/fnins.2019.00145
Duman, R. S., and Aghajanian, G. K. (2012). Synaptic dysfunction in depression: potential therapeutic targets. Science 338, 68–72. doi: 10.1126/science.1222939
Duman, R. S., Aghajanian, G. K., Sanacora, G., and Krystal, J. H. (2016). Synaptic plasticity and depression: new insights from stress and rapid-acting antidepressants. Nat. Med. 22, 238–249. doi: 10.1038/nm.4050
Fan, W., Zhang, S., Hu, J., Liu, B., Wen, L., Gong, M., et al. (2019). Aberrant brain function in active-stage ulcerative colitis patients: a resting-state functional MRI study. Front. Hum. Neurosci. 13:107. doi: 10.3389/fnhum.2019.00107
Friederici, A. D., and Gierhan, S. M. (2013). The language network. Curr. Opin. Neurobiol. 23, 250–254. doi: 10.1016/j.conb.2012.10.002
García-Campayo, J., Ayuso-Mateos, J. L., Caballero, L., Romera, I., Aragonés, E., Rodríguez-Artalejo, F., et al. (2008). Relationship of somatic symptoms with depression severity, quality of life, and health resources utilization in patients with major depressive disorder seeking primary health care in Spain. Prim. Care Companion J. Clin. Psychiatry 10, 355–362. doi: 10.4088/pcc.v10n0502
Gong, Q., Wu, Q., Scarpazza, C., Lui, S., Jia, Z., Marquand, A., et al. (2011). Prognostic prediction of therapeutic response in depression using high-field MR imaging. Neuroimage 55, 1497–1503. doi: 10.1016/j.neuroimage.2010.11.079
Guo, W., Liu, F., Dai, Y., Jiang, M., Zhang, J., Yu, L., et al. (2013). Decreased interhemispheric resting-state functional connectivity in first-episode, drug-naive major depressive disorder. Prog. Neuropsychopharmacol. Biol. Psychiatry 41, 24–29. doi: 10.1016/j.pnpbp.2012.11.003
Hahamy, A., Calhoun, V., Pearlson, G., Harel, M., Stern, N., Attar, F., et al. (2014). Save the global: global signal connectivity as a tool for studying clinical populations with functional magnetic resonance imaging. Brain Connect 4, 395–403. doi: 10.1089/brain.2014.0244
Halassa, M. M., and Kastner, S. (2017). Thalamic functions in distributed cognitive control. Nat. Neurosci. 20, 1669–1679. doi: 10.1038/s41593-017-0020-1
Hamilton, M. (1967). Development of a rating scale for primary depressive illness. Br. J. Soc. Clin. Psychol. 6, 278–296. doi: 10.1111/j.2044-8260.1967.tb00530.x
Hu, L., Xiao, M., Ai, M., Wang, W., Chen, J., Tan, Z., et al. (2019). Disruption of resting-state functional connectivity of right posterior insula in adolescents and young adults with major depressive disorder. J. Affect. Disord. 257, 23–30. doi: 10.1016/j.jad.2019.06.057
Hurley-Gius, K. M., and Neafsey, E. J. (1986). The medial frontal cortex and gastric motility: microstimulation results and their possible significance for the overall pattern of organization of rat frontal and parietal cortex. Brain Res. 365, 241–248. doi: 10.1016/0006-8993(86)91635-5
Iannilli, E., Noennig, N., Hummel, T., and Schoenfeld, A. M. (2014). Spatio-temporal correlates of taste processing in the human primary gustatory cortex. Neuroscience 273, 92–99. doi: 10.1016/j.neuroscience.2014.05.017
Icenhour, A., Witt, S. T., Elsenbruch, S., Lowén, M., Engström, M., Tillisch, K., et al. (2017). Brain functional connectivity is associated with visceral sensitivity in women with Irritable Bowel Syndrome. Neuroimage Clin. 15, 449–457. doi: 10.1016/j.nicl.2017.06.001
Jain, R. (2009). The epidemiology and recognition of pain and physical symptoms in depression. J. Clin. Psychiatry 70:e04. doi: 10.4088/JCP.8001tx1c.e04
Kang, D., McAuley, J. H., Kassem, M. S., Gatt, J. M., and Gustin, S. M. (2019). What does the grey matter decrease in the medial prefrontal cortex reflect in people with chronic pain? Eur. J. Pain 23, 203–219. doi: 10.1002/ejp.1304
Karling, P., Danielsson, A., Adolfsson, R., and Norrback, K. F. (2007). No difference in symptoms of irritable bowel syndrome between healthy subjects and patients with recurrent depression in remission. Neurogastroenterol. Motil. 19, 896–904. doi: 10.1111/j.1365-2982.2007.00967.x
Kelly, C., Biswal, B. B., Craddock, R. C., Castellanos, F. X., and Milham, M. P. (2012). Characterizing variation in the functional connectome: promise and pitfalls. Trends Cogn. Sci. 16, 181–188. doi: 10.1016/j.tics.2012.02.001
Kirmayer, L. J., Robbins, J. M., Dworkind, M., and Yaffe, M. J. (1993). Somatization and the recognition of depression and anxiety in primary care. Am. J. Psychiatry 150, 734–741. doi: 10.1176/ajp.150.5.734
Leech, R., and Sharp, D. J. (2014). The role of the posterior cingulate cortex in cognition and disease. Brain 137(Pt 1), 12–32. doi: 10.1093/brain/awt162
Lemelin, S., and Baruch, P. (1998). Clinical psychomotor retardation and attention in depression. J. Psychiatr. Res. 32, 81–88. doi: 10.1016/s0022-3956(98)00002-8
Lemogne, C., Delaveau, P., Freton, M., Guionnet, S., and Fossati, P. (2012). Medial prefrontal cortex and the self in major depression. J. Affect. Disord. 136, e1–e11. doi: 10.1016/j.jad.2010.11.034
Lépine, J. P., and Briley, M. (2004). The epidemiology of pain in depression. Hum. Psychopharmacol. 19(Suppl. 1), S3–S7. doi: 10.1002/hup.618
Levinthal, D. J., and Strick, P. L. (2020). Multiple areas of the cerebral cortex influence the stomach. Proc. Natl. Acad. Sci. U.S.A 117, 13078–13083. doi: 10.1073/pnas.2002737117
Lieberman, M. D., Straccia, M. A., Meyer, M. L., Du, M., and Tan, K. M. (2019). Social, self, (situational), and affective processes in medial prefrontal cortex (MPFC): causal, multivariate, and reverse inference evidence. Neurosci. Biobehav. Rev. 99, 311–328. doi: 10.1016/j.neubiorev.2018.12.021
Liu, F., Zhu, C., Wang, Y., Guo, W., Li, M., Wang, W., et al. (2015). Disrupted cortical hubs in functional brain networks in social anxiety disorder. Clin. Neurophysiol. 126, 1711–1716. doi: 10.1016/j.clinph.2014.11.014
Liu, P., Fan, Y., Wei, Y., Zeng, F., Li, R., Fei, N., et al. (2018). Altered structural and functional connectivity of the insula in functional dyspepsia. Neurogastroenterol. Motil. 30:e13345. doi: 10.1111/nmo.13345
Liu, P., Li, G., Zhang, A., Yang, C., Liu, Z., Sun, N., et al. (2020). Brain structural and functional alterations in MDD patient with gastrointestinal symptoms: a resting-state MRI study. J. Affect. Disord. 273, 95–105. doi: 10.1016/j.jad.2020.03.107
Milaneschi, Y., Simmons, W. K., van Rossum, E. F. C., and Penninx, B. W. (2019). Depression and obesity: evidence of shared biological mechanisms. Mol. Psychiatry 24, 18–33. doi: 10.1038/s41380-018-0017-5
Novick, D., Montgomery, W., Aguado, J., Kadziola, Z., Peng, X., Brugnoli, R., et al. (2013). Which somatic symptoms are associated with an unfavorable course in Asian patients with major depressive disorder? J. Affect. Disord. 149, 182–188. doi: 10.1016/j.jad.2013.01.020
Painchault, C., Brignone, M., Lamy, F. X., Diamand, F., and Saragoussi, D. (2014). Economic burden of major depressive disorder (Mdd) in five European Countries: description of resource use by health state. Value Health 17:A465. doi: 10.1016/j.jval.2014.08.1300
Pan, P., Ou, Y., Su, Q., Liu, F., Chen, J., Zhao, J., et al. (2019). Voxel-based global-brain functional connectivity alterations in first-episode drug-naive patients with somatization disorder. J. Affect. Disord. 254, 82–89. doi: 10.1016/j.jad.2019.04.099
Penfield, W., and Faulk, M. E. Jr. (1955). The insula; further observations on its function. Brain 78, 445–470. doi: 10.1093/brain/78.4.445
Peng, X., Lin, P., Wu, X., Gong, R., Yang, R., and Wang, J. (2018). Insular subdivisions functional connectivity dysfunction within major depressive disorder. J. Affect. Disord. 227, 280–288. doi: 10.1016/j.jad.2017.11.018
Power, J. D., Barnes, K. A., Snyder, A. Z., Schlaggar, B. L., and Petersen, S. E. (2012). Spurious but systematic correlations in functional connectivity MRI networks arise from subject motion. Neuroimage 59, 2142–2154. doi: 10.1016/j.neuroimage.2011.10.018
Price, R. B., and Duman, R. (2020). Neuroplasticity in cognitive and psychological mechanisms of depression: an integrative model. Mol. Psychiatry 25, 530–543. doi: 10.1038/s41380-019-0615-x
Raichle, M. E. (2015). The brain’s default mode network. Annu. Rev. Neurosci. 38, 433–447. doi: 10.1146/annurev-neuro-071013-014030
Ries, A., Chang, C., Glim, S., Meng, C., Sorg, C., and Wohlschläger, A. (2018). Grading of frequency spectral centroid across resting-state networks. Front. Hum. Neurosci. 12:436. doi: 10.3389/fnhum.2018.00436
Saalmann, Y. B., and Kastner, S. (2011). Cognitive and perceptual functions of the visual thalamus. Neuron 71, 209–223. doi: 10.1016/j.neuron.2011.06.027
Sani, I., Stemmann, H., Caron, B., Bullock, D., Stemmler, T., Fahle, M., et al. (2021). The human endogenous attentional control network includes a ventro-temporal cortical node. Nat. Commun. 12:360. doi: 10.1038/s41467-020-20583-5
Schwiedrzik, C. M., Sudmann, S. S., Thesen, T., Wang, X., Groppe, D. M., Mégevand, P., et al. (2018). Medial prefrontal cortex supports perceptual memory. Curr. Biol. 28, R1094–R1095. doi: 10.1016/j.cub.2018.07.066
Skrobisz, K., Piotrowicz, G., Naumczyk, P., Sabisz, A., Markiet, K., Rydzewska, G., et al. (2020). Imaging of morphological background in selected functional and inflammatory gastrointestinal diseases in fMRI. Front. Psychiatry 11:461. doi: 10.3389/fpsyt.2020.00461
Squire, L. R., Stark, C. E., and Clark, R. E. (2004). The medial temporal lobe. Annu. Rev. Neurosci. 27, 279–306. doi: 10.1146/annurev.neuro.27.070203.144130
Squire, L. R., and Zola-Morgan, S. (1991). The medial temporal lobe memory system. Science 253, 1380–1386. doi: 10.1126/science.1896849
Swets, J. A. (1988). Measuring the accuracy of diagnostic systems. Science 240, 1285–1293. doi: 10.1126/science.3287615
Torres-Fuentes, C., Schellekens, H., Dinan, T. G., and Cryan, J. F. (2017). The microbiota-gut-brain axis in obesity. Lancet Gastroenterol. Hepatol. 2, 747–756. doi: 10.1016/s2468-1253(17)30147-4
Uddin, L. Q., Nomi, J. S., Hébert-Seropian, B., Ghaziri, J., and Boucher, O. (2017). Structure and function of the human insula. J. Clin. Neurophysiol. 34, 300–306. doi: 10.1097/wnp.0000000000000377
Van Oudenhove, L., Vandenberghe, J., Dupont, P., Geeraerts, B., Vos, R., Dirix, S., et al. (2010). Regional brain activity in functional dyspepsia: a H(2)(15)O-PET study on the role of gastric sensitivity and abuse history. Gastroenterology 139, 36–47. doi: 10.1053/j.gastro.2010.04.015
Wang, C., Wu, H., Chen, F., Xu, J., Li, H., Li, H., et al. (2018). Disrupted functional connectivity patterns of the insula subregions in drug-free major depressive disorder. J. Affect. Disord. 234, 297–304. doi: 10.1016/j.jad.2017.12.033
Wang, L., Hermens, D. F., Hickie, I. B., and Lagopoulos, J. (2012). A systematic review of resting-state functional-MRI studies in major depression. J. Affect. Disord. 142, 6–12. doi: 10.1016/j.jad.2012.04.013
Wang, L., Xia, M., Li, K., Zeng, Y., Su, Y., Dai, W., et al. (2015). The effects of antidepressant treatment on resting-state functional brain networks in patients with major depressive disorder. Hum. Brain Mapp. 36, 768–778. doi: 10.1002/hbm.22663
Wellman, C. L., and Moench, K. M. (2019). Preclinical studies of stress, extinction, and prefrontal cortex: intriguing leads and pressing questions. Psychopharmacology 236, 59–72. doi: 10.1007/s00213-018-5023-4
Wise, T., Radua, J., Via, E., Cardoner, N., Abe, O., Adams, T. M., et al. (2017). Common and distinct patterns of grey-matter volume alteration in major depression and bipolar disorder: evidence from voxel-based meta-analysis. Mol. Psychiatry 22, 1455–1463. doi: 10.1038/mp.2016.72
Yan, C. G., Chen, X., Li, L., Castellanos, F. X., Bai, T. J., Bo, Q. J., et al. (2019). Reduced default mode network functional connectivity in patients with recurrent major depressive disorder. Proc. Natl. Acad. Sci. U.S.A 116, 9078–9083. doi: 10.1073/pnas.1900390116
Yan, C. G., Wang, X. D., Zuo, X. N., and Zang, Y. F. (2016). DPABI: data processing and analysis for (Resting-State) brain imaging. Neuroinformatics 14, 339–351. doi: 10.1007/s12021-016-9299-4
Yan, M., Cui, X., Liu, F., Li, H., Huang, R., Tang, Y., et al. (2021). Abnormal default-mode network homogeneity in melancholic and nonmelancholic major depressive disorder at rest. Neural Plast 2021:6653309. doi: 10.1155/2021/6653309
Yang, Y., Cui, Q., Pang, Y., Chen, Y., Tang, Q., Guo, X., et al. (2021). Frequency-specific alteration of functional connectivity density in bipolar disorder depression. Prog. Neuropsychopharmacol. Biol. Psychiatry 104:110026. doi: 10.1016/j.pnpbp.2020.110026
Yuan, Y., Zhang, Z., Bai, F., Yu, H., Shi, Y., Qian, Y., et al. (2008). Abnormal neural activity in the patients with remitted geriatric depression: a resting-state functional magnetic resonance imaging study. J. Affect. Disord. 111, 145–152. doi: 10.1016/j.jad.2008.02.016
Zeng, F., Qin, W., Liang, F., Liu, J., Tang, Y., Liu, X., et al. (2011). Abnormal resting brain activity in patients with functional dyspepsia is related to symptom severity. Gastroenterology 141, 499–506. doi: 10.1053/j.gastro.2011.05.003
Zhang, F. F., Peng, W., Sweeney, J. A., Jia, Z. Y., and Gong, Q. Y. (2018). Brain structure alterations in depression: psychoradiological evidence. CNS Neurosci. Ther. 24, 994–1003. doi: 10.1111/cns.12835
Zhou, G., Liu, P., Zeng, F., Yuan, K., Yu, D., von Deneen, K. M., et al. (2013). Increased interhemispheric resting-state functional connectivity in functional dyspepsia: a pilot study. NMR Biomed. 26, 410–415. doi: 10.1002/nbm.2878
Keywords: global functional connectivity, major depressive disorder, gastrointestinal symptoms, resting state, functional magnetic resonance imaging
Citation: Yan M, Fu X, Ou Y, Liu F, Li H and Guo W (2022) Multiple-Network Alterations in Major Depressive Disorder With Gastrointestinal Symptoms at Rest Revealed by Global Functional Connectivity Analysis. Front. Neurosci. 16:897707. doi: 10.3389/fnins.2022.897707
Received: 31 March 2022; Accepted: 06 June 2022;
Published: 24 June 2022.
Edited by:
Mingxia Liu, University of North Carolina at Chapel Hill, United StatesReviewed by:
Yifeng Wang, Sichuan Normal University, ChinaChunxia Yang, First Hospital of Shanxi Medical University, China
Copyright © 2022 Yan, Fu, Ou, Liu, Li and Guo. This is an open-access article distributed under the terms of the Creative Commons Attribution License (CC BY). The use, distribution or reproduction in other forums is permitted, provided the original author(s) and the copyright owner(s) are credited and that the original publication in this journal is cited, in accordance with accepted academic practice. No use, distribution or reproduction is permitted which does not comply with these terms.
*Correspondence: Wenbin Guo, Z3Vvd2VuYmluNzZAY3N1LmVkdS5jbg==