- 1Department of Neurology, The First Affiliated Hospital of Chongqing Medical University, Chongqing, China
- 2Department of Neurology, Yongchuan Hospital of Chongqing Medical University, Chongqing, China
- 3National Health Commission (NHC) Key Laboratory of Diagnosis and Treatment on Brain Functional Diseases, The First Affiliated Hospital of Chongqing Medical University, Chongqing, China
- 4Department of Neurology, Tongji Hospital, Tongji Medical College, Huazhong University of Science and Technology, Wuhan, China
- 5Emergency Department, The Affiliated Hospital of Guizhou Medical University, Guiyang, China
- 6Department of Neurology, Harvard Medical School, Massachusetts General Hospital, Boston, MA, United States
Objective: To investigate the association between cerebral small vessel disease (SVD) and hematoma volume in primary intracerebral hemorrhage (ICH).
Methods: Patients from a prospective ICH cohort were enrolled. Admission and follow-up CT scan within 72 h after onset were reviewed to calculate the final hematoma volume. We evaluated cortical superficial siderosis and the global SVD score, including white matter hyperintensities, lacunes, enlarged perivascular space, and cerebral microbleeds on MRI. We conducted the multivariate logistic regression analyses to explore the association between SVD markers and small ICH, as well as hematoma volume. Hematoma location was stratified into lobar and non-lobar for subgroup analysis.
Results: A total of 187 patients with primary ICH (mean age 62.4 ± 13.4 years, 67.9% male) were enrolled. 94 (50.2%) patients had small ICH. The multivariate logistic regression analysis showed an association between global SVD score and small ICH [adjusted odds ratio (aOR) 1.27, 95% CI 1.03–1.57, p = 0.027] and a trend of higher global SVD score towards non-lobar small ICH (aOR 1.23, 95% CI 0.95–1.58, p = 0.122). In the multivariate linear regression analysis, global SVD score was inversely related to hematoma volume of all ICH (β = −0.084, 95% CI −0.142 to −0.025, p = 0.005) and non-lobar ICH (β = −0.112, 95% CI −0.186 to −0.037, p = 0.004). Lacune (β = −0.245, 95% CI −0.487 to −0.004, p = 0.046) was associated with lower non-lobar ICH volume.
Conclusion: Global SVD score is associated with small ICH and inversely correlated with hematoma volume. This finding predominantly exists in non-lobar ICH.
Introduction
Spontaneous intracerebral hemorrhage (ICH) is a mortal subtype of stroke affecting more than 2 million people worldwide (Qureshi et al., 2009; Cordonnier et al., 2018). Short-term case fatality rate is estimated to exceed 40%, whereas approximately 70% are demised after 5 years (van Asch et al., 2010; Poon et al., 2014). ICH is the most devastating manifestation of cerebral small vessel disease (SVD) (Pantoni, 2010). SVD has been considered as the underlying cause of more than 80% of ICH cases (Rodrigues et al., 2021).
Large hematoma volume and early hematoma expansion are the most robust predictors of neurological deterioration and unfavorable outcomes and, meanwhile, the crucial modifiable targets (Davis et al., 2006). Previously, we have demonstrated that non-contrast CT markers are reliable to identify ICH at high risk of expansion (Li et al., 2015, 2016, 2017, 2019a). Recently, we proposed the definition of small and benign ICH as a subgroup at low risk of hematoma expansion and higher likelihood of functional independence (Li et al., 2019b). Intensive care and aggressive antiexpansion therapies may not be necessary for this subgroup.
ICH location is associated with different underlying types of SVD. Hemorrhage in deep and infratentorial regions is attributed to hypertensive arteriolosclerosis, whereas lobar ICH is related to cerebral amyloid angiopathy (CAA) (Charidimou et al., 2012). SVD markers on MRI such as white matter hyperintensity (WMH), lacune, perivascular space (PVS), and cerebral microbleed (CMB) well represent the underlying microangiopathic damage. Although it is hypothesized that SVD may promote extensive bleeding in the acute phase, several studies discussing the effect of SVD to hematoma volume and expansion showed mixed results (Lou et al., 2010; Boulouis et al., 2016; Sykora et al., 2017; Uniken Venema et al., 2020; Xu et al., 2021). One study reported greater white matter damage in larger ICH (Lou et al., 2010), while others found insignificant or borderline association between WMH and ICH volume (Boulouis et al., 2016; Sykora et al., 2017; Uniken Venema et al., 2020). There are limited data illustrating the association between the overall SVD burden and ICH volume.
In this study, we aim to investigate whether global SVD score is associated with small ICH. We further conducted a subgroup analysis by stratifying the hematoma location into lobar and non-lobar ICH.
Materials and Methods
Study Design and Participants
We consecutively collected the clinical and imaging information from an ongoing prospective cohort of primary ICH admitted to the First Affiliated Hospital of Chongqing Medical University from January 2015 to November 2021. Patients aged > 18 years were included, if they had: (1) at least one CT scan within 72 h after symptomatic onset and (2) brain MRI scan, including blood-sensitive susceptibility-weighted imaging (SWI) within 30 days from onset. Patients were excluded, if they had: (1) secondary ICH attributable to structural lesions (e.g., arteriovenous malformation, intracranial aneurysm, Moyamoya disease, and cavernous angioma), medication-related anticoagulative disorder, trauma, and hemorrhagic transformation from ischemic stroke; (2) multiple ICH; (3) primary intraventricular hemorrhage; and (4) hematoma evacuation before MRI.
Data Collection
The baseline demographic and clinical characteristics, including age, sex, medical history, and vascular risk factors, were collected by interview and review of electronic medical records. Blood glucose and international normalized ratio (INR) were tested immediately at admission. Serum total cholesterol (TC), triglyceride (TG), high-density lipoprotein cholesterol (HDL-c), and low-density lipoprotein cholesterol (LDL-c) were obtained within 24 h after admission.
Image Acquisition and Analysis
We collected all the CT and MR images as Digital Imaging and Communications in Medicine (DICOM) format for further review.
All the CT images were independently evaluated by two trained neurologists blinded to clinical information. ICH location was dichotomized into lobar (i.e., cortical and subcortical white matter) and non-lobar (i.e., basal ganglia, thalamus, cerebellum, and brainstem) (Charidimou et al., 2017b). The multiple ICH was defined as coexistence of more than two non-contiguous parenchymal hematoma on baseline CT scan (Wu et al., 2017). ICH volume on CT was measured using ABC/2 method (Kothari et al., 1996). The final hematoma volume was defined as the largest hematoma volume on brain CT scan within 72 h from ictus. Small ICH was assessed based on the final hematoma volume fulfilling the following criteria: (1) volume < 3 ml for brainstem ICH; (2) volume < 5 ml for cerebellar ICH; (3) volume < 10 ml for basal ganglia and thalamic ICH; and (4) volume < 15 ml for lobar ICH (Li et al., 2019b).
All the MR images were independently reviewed by two trained neurologists blinded to clinical data according to the STandards for ReportIng Vascular changes on nEuroimaging (STRIVE) criteria (Wardlaw et al., 2013). Deep and periventricular white matter hyperintensities (WMHs) were visually assessed using the Fazekas scale (Fazekas et al., 1987). Dilated perivascular space (PVS) was visually assessed on T2-weighted images (Doubal et al., 2010). Lacune was defined as round or ovoid cavities of low signal with or without light rim on fluid-attenuated inversion recovery (FLAIR) and corresponding cerebrospinal fluid (CSF) signal on T2-weighted images without hypointense signal on susceptibility-weighted imaging (SWI). Cerebral microbleed (CMB) was defined as round or ovoid lesion of homogeneous low signal on SWI (Greenberg et al., 2009b). CMB location was classified as deep (affecting basal ganglia, thalamus, and brainstem) and lobar (affecting cortex and subcortical white matter) and further categorized as strictly and non-strictly lobar CMB. Cortical superficial siderosis (cSS) was defined as curvilinear intrasulcal hypointensities on SWI (Charidimou et al., 2015). Any discrepancies were solved by further review and discussion.
Rating Scale of Overall Small Vessel Disease Severity
The SVD severity was assessed by global SVD score, a validated MRI-based ordinal rating scale from 0 to 6 points (Lau et al., 2017; Pasi et al., 2021). One point was allocated for: (1) moderate WMH (total Fazekas score 3–4); (2) moderate-to-severe basal ganglia-dilated perivascular space (BG-PVS) (> 20); (3) presence of one or more lacunes; and (4) 1–4 any CMBs. Two points were allocated for: (1) severe WMH (total Fazekas score 5–6) and (2) ≥ 5 any CMBs. We dichotomized the SVD severity as mild (global SVD score < 3 points) and severe (global SVD score ≥ 3 points) (Pasi et al., 2021).
Statistical Analysis
In the univariate analysis, we compared the clinical and neuroimaging characteristics between patients with small and non-small ICH. Categorical variables were presented as number and percentages. Continuous variables were presented as mean (SD) or median [interquartile range (IQR)] depending on distribution. We compared continuous and ordinal categorical variables with the Student’s t-test or the Mann–Whitney U-test and categorical variables with the chi-squared test or the Fisher’s exact test, as appropriate. We investigated the association between SVD and small ICH in the multivariate logistic regression analysis by entering prespecified covariates and variables with p < 0.1 in the univariate analysis. We further conducted the multivariate linear regression analysis, including log-transformed final hematoma and covariates from the univariate analysis. The model 1 included individual SVD markers and the model 2 included the global SVD score. cSS is a well-established imaging marker of large hematoma volume, we included it into the model despite of no significance in the univariate analysis. In subgroup analysis, we investigated the effect of SVD to small ICH in lobar and non-lobar ICH separately. A p < 0.05 was considered as statistically significant. All the analyses were performed with the SPSS for Windows, version 26.
Results
A total of 338 patients who met the inclusion criteria were admitted to our hospital between this study period. A total of 151 patients were excluded for meeting one or more of exclusion criteria. The flowchart of selecting patients eligible for analysis is given in Figure 1. Finally, 187 patients (mean age 62.4 ± 13.4 years, 67.9% male) were enrolled in this analysis (Table 1). Of them, 57 (30.5%) patients had lobar ICH. The median time from ICH onset to MRI was 6 days [interquartile range (IQR) 4–9 days].
Among the study population, small ICH was presented in 94 (50.2%) patients. The univariate analysis of patients with small and non-small ICH is given in Table 2. The median baseline and final hematoma volume were smaller in small ICH as compared with non-small ICH (p < 0.001). Hypertension was numerically more prevalent in small ICH (90.4 vs. 80.6%, p = 0.090). Other demographic, clinical, and laboratory characteristics were comparable between patients with small and non-small ICH (all p > 0.10). In the univariate analysis, patients with small ICH were more likely to have higher WMH burden (p = 0.058), lacune (p = 0.027), CMB ≥ 5 (p = 0.022), and higher global SVD score (p = 0.014), whereas lobar ICH (p = 0.004) was more frequent in patients with non-small ICH. The prevalence of cSS was higher in patients with non-small ICH, albeit no statistical significance. Severe SVD was presented in 53 of 94 patients with small ICH (56%) and 34 of 93 patients with non-small ICH (37%, p = 0.010, Figure 2).
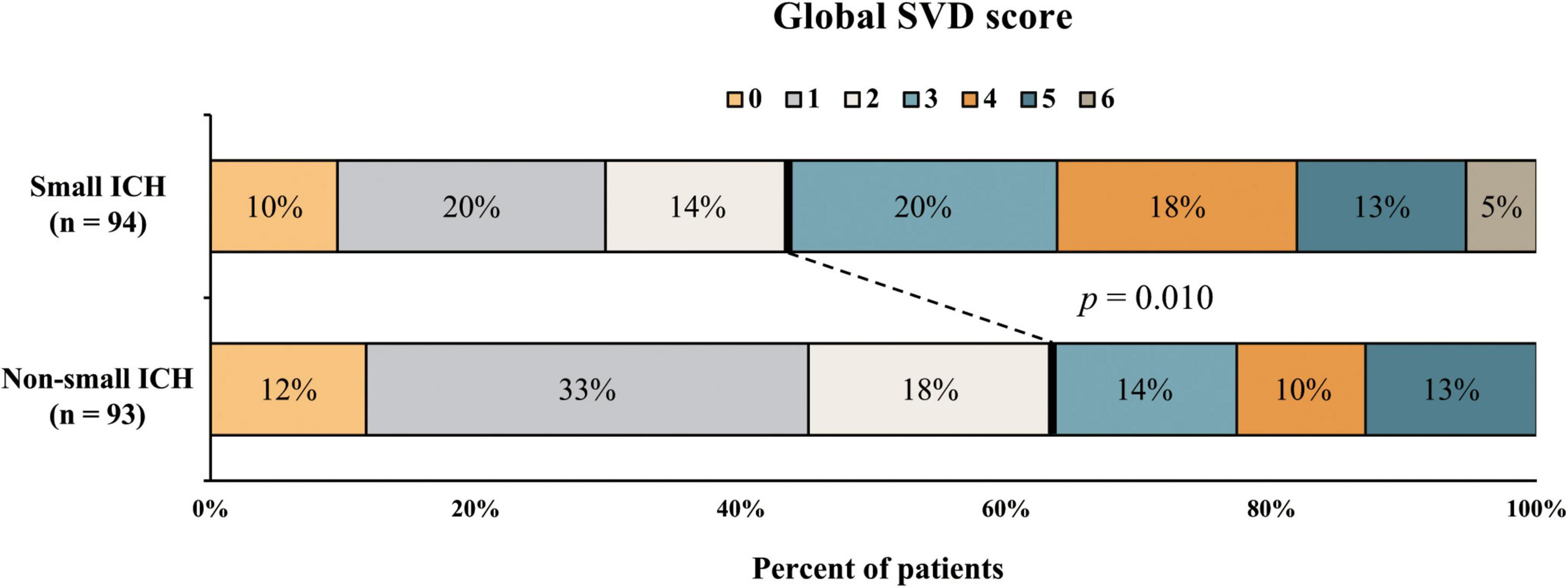
Figure 2. Distribution of small vessel disease (SVD) severity in patients with small and non-small intracerebral hemorrhage (ICH).
In the multivariate logistic regression analysis (Table 3), after adjusting for age, male sex, and hypertension, no individual SVD marker was independently associated with small ICH. Global SVD score [adjusted odds ratio (aOR) 1.27, 95% confidence interval (CI) 1.03–1.57, p = 0.027] was correlated with small ICH. By contrast, lobar ICH was correlated with non-small ICH. In subgroup analysis of hematoma location, we observed only a trend of global SVD score (aOR 1.23, 95% CI 0.95–1.58, p = 0.123) toward increasing odds of small ICH in patients with non-lobar ICH.
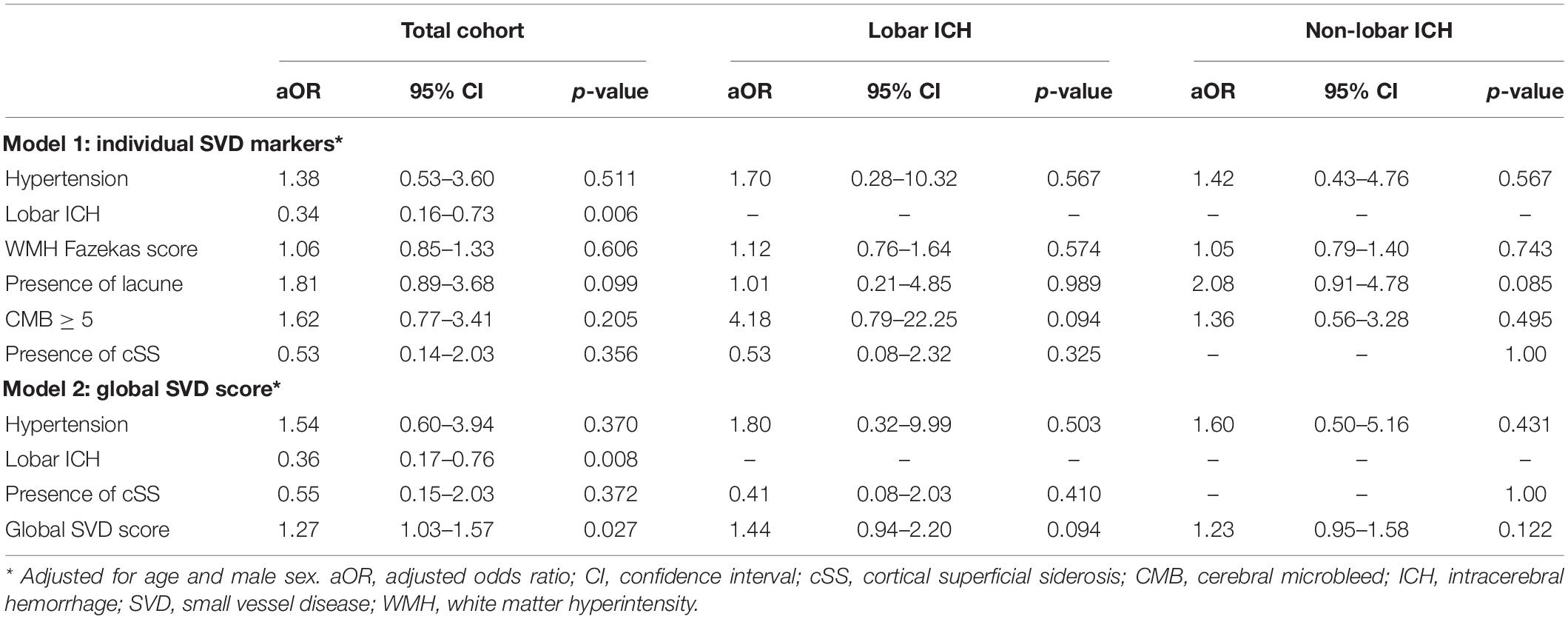
Table 3. The multivariate logistic regression analysis of variables associated with small ICH adjusted for age and male sex.
Figure 3 shows a highly inverse relationship between SVD burden and final hematoma volume in non-lobar (p < 0.001) rather than lobar ICH in a simple linear regression model. In the multivariate linear regression analysis (Table 4), there was a significant association of higher global SVD score and lower log-transformed hematoma volume (β = −0.084, 95% CI −0.142 to −0.025, p < 0.001) in the whole study and in subgroup of patients with non-lobar ICH (β = −0.112, 95% CI −0.186 to −0.037, p = 0.004) after adjusting for age, male sex, and hypertension. For individual SVD markers, lacune (β = −0.245, 95% CI −0.487 to −0.004, p = 0.046) was the only SVD marker independently associated with lower non-lobar ICH volume.
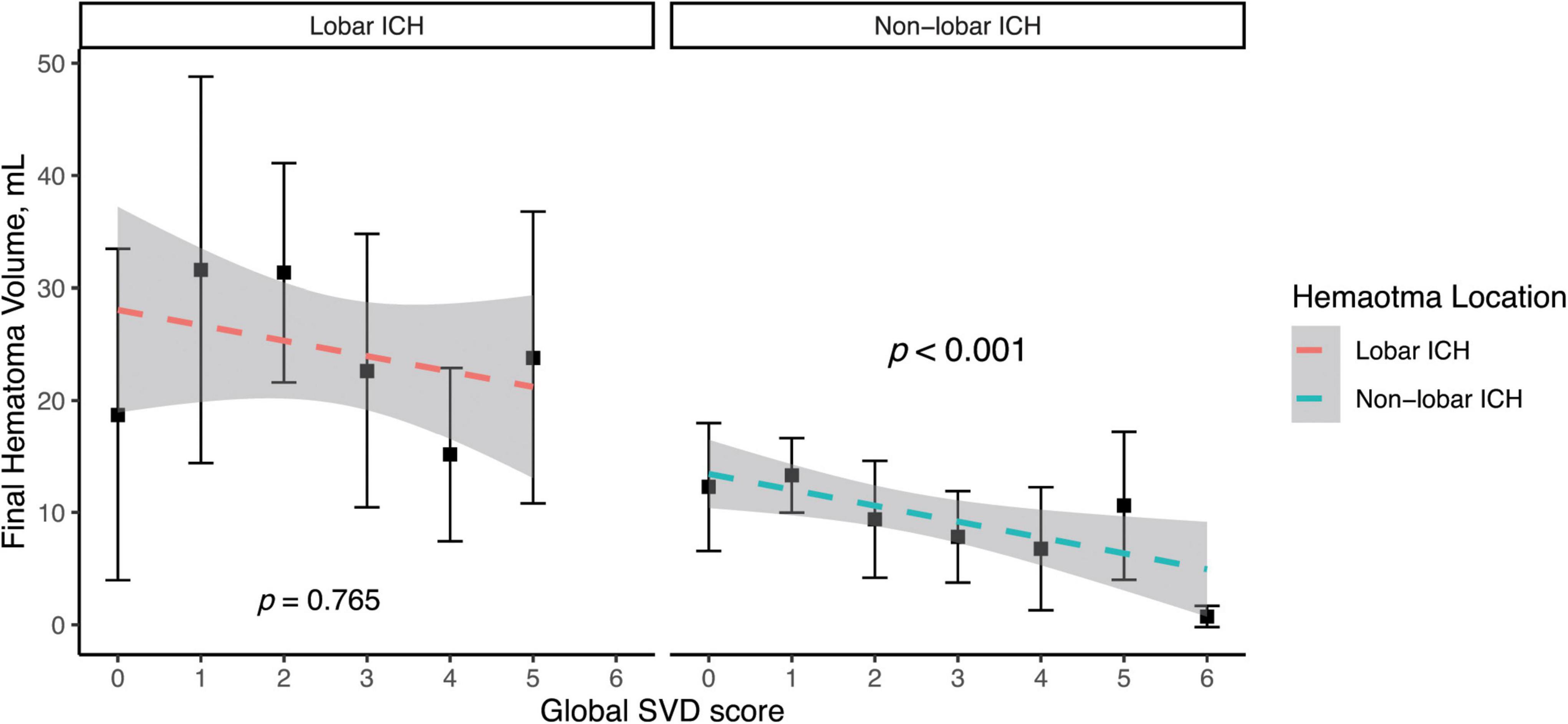
Figure 3. Simple linear regression model of log-transformed hematoma volume and global SVD score in lobar and non-lobar ICH. The dependent variable was base-10 logarithm of hematoma volume. Log of hematoma volume in non-lobar ICH = -0.133 × Global SVD score + 0.993, p < 0.001. Log of hematoma volume in lobar ICH = -0.013 × Global SVD score + 1.226, p = 0.765.
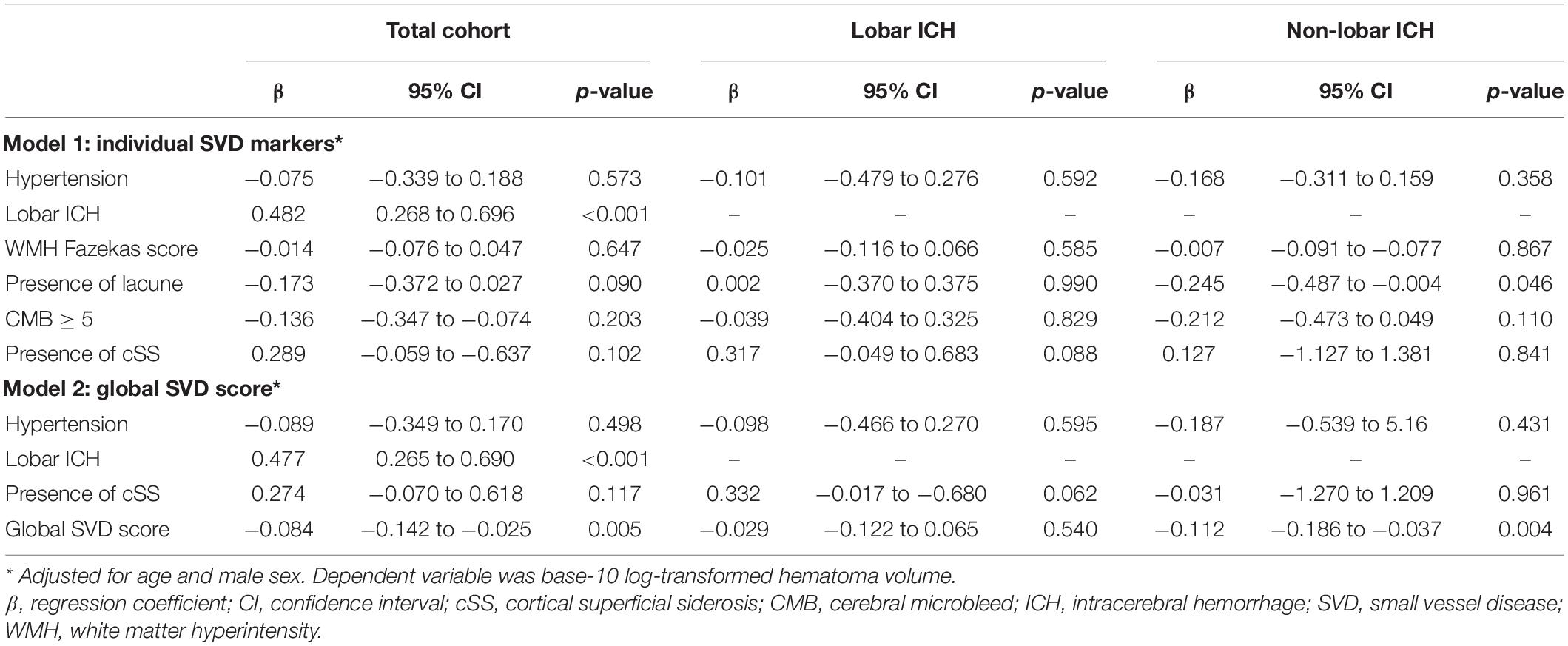
Table 4. The multivariate linear regression analysis of associations between SVD markers and hematoma volume adjusted for age and male sex.
To investigate whether the correlation of global SVD burden with small ICH remains consistent according to history of hypertension or ICH location, we further analyzed any potential differences of association between SVD burden and small ICH by hypertension and hematoma location. There was no significant interaction between hypertension and SVD burden (p for interaction = 0.195) or between ICH location and SVD burden (p for interaction = 0.878, Table 5).
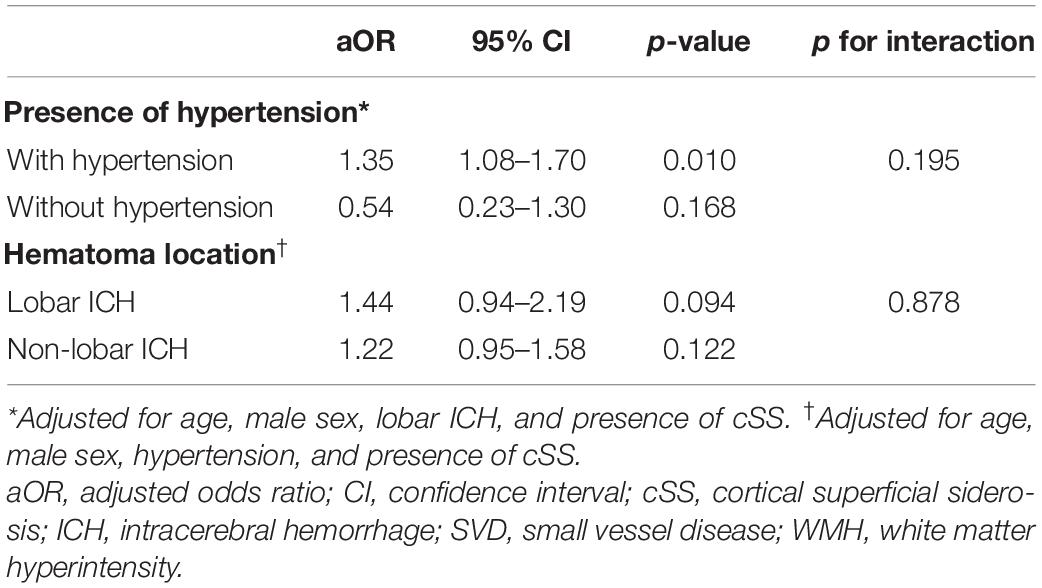
Table 5. Associations between global SVD score and odds of small ICH by presence of hypertension and hematoma location.
Discussion
The main finding of this study is that higher SVD burden is associated with small ICH. Data indicated that this association persisted predominantly in non-lobar ICH. With regard to individual SVD markers, only lacune is correlated with lower ICH volume.
Accumulating evidence suggests that large hematoma volume robustly predicts unfavorable outcomes in patients with ICH (Davis et al., 2006). Conversely, smaller ICH volume had lower risk of expansion, neurological worsening, and greater odds of good outcomes (Dowlatshahi et al., 2016). In this study, we defined the criteria of small ICH based on different location and reported that small ICH independently predicts 3-month good outcomes (Li et al., 2019b).
Small vessel disease markers have been demonstrated to predict future ICH, while our data suggesting that they may not lead to severe hemorrhagic event, which is of great interest (Debette et al., 2019). Prior studies investigating microangiopathy and hematoma volume showed inconsistent results. In a retrospective analysis of 79 patients, extensive WMH was associated with greater hematoma volume and early hematoma growth (Lou et al., 2010). Other studies found neutral effect of WMH to hematoma volume (Boulouis et al., 2016; Sykora et al., 2017; Hansen et al., 2020; Uniken Venema et al., 2020). A CT-based study of 2,579 patients with ICH found a borderline opposing association between leukoaraiosis and ICH volume, while MRI markers were not included in this study (Uniken Venema et al., 2020). As a hemorrhagic SVD marker, CMB is associated with elevated risk of ICH in community dwellers (Akoudad et al., 2015), patients with ischemic stroke (Wilson et al., 2019), and those with ICH (Charidimou et al., 2017a). However, there is emerging evidence indicating that CMB may not predict large ICH. A study of 59 patients found significant decreased CMB number in patients with CT angiography spot sign, a hallmark of active blood extravasation (Evans et al., 2010). Another MRI-based study found absence of CMB that was associated with larger ICH volume and no relationship between WMH and hematoma volume in both the deep and lobar ICH (Boulouis et al., 2016). In this study, we found higher global SVD score predisposed to small ICH. In addition, we observed a significant opposing association in the multivariate linear analysis and a marginal correlation between greater SVD burden and non-lobar small ICH in the multivariate logistic regression analysis. Our results corroborate with a recent study, which found an opposing linear relationship between SVD burden and hematoma volume (Xu et al., 2021). With regard to individual SVD markers, WMH, lacune, and severe CMB burden were more frequently observed in small ICH in the univariate analysis, whereas only lacune persisted an association with lower non-lobar ICH volume in the multivariate linear regression analysis. Although there was a trend of increasing CMB number toward small ICH and cSS toward large ICH, SVD markers and global SVD score were not associated with lobar ICH volume in the multivariate logistic regression analysis.
It is highly speculated that coexistence of multiple SVD markers is a sign of cerebrovascular fragility. Non-lobar ICH is related to age and vascular risk factors such as hypertension, diabetes mellitus, dyslipidemia, smoking, and alcohol use (Pantoni, 2010). There is strong evidence that deposition of hyaline material in the thickened tunica media is a major feature of arteriosclerotic changes, which may diminish the risk of active bleeding (Mitchell, 2008). Fisher found that secondary growth surrounding original bleeding site was more likely to be present with vessel without arteriolosclerosis (Fisher, 1971). Source of bleeding in non-lobar ICH has been pathologically proved to be thin-walled aneurysmal dilatation on deep small vessels (Miller Fisher, 2003). In addition, cerebrovascular reactivity (CVR), a parameter of vessel constriction and dilatation ability, was impaired in patients with hypertensive ICH and advanced SVD, which may decrease the risk of progressive bleeding, while the exact impact of reduced CVR to ICH volume remains unknown (Lee et al., 2021). Clinical and experimental studies are needed to validate the potential effect of hemodynamic features to ICH volume. Lobar ICH is CAA related. CMB and cSS may have different clinical and pathological entities (Shoamanesh et al., 2014). An autopsy study of patients with CAA found significantly thicker vessel walls in subjects with high microbleed burden as compared with those with low microbleed burden, which predisposes to protective effects against extensive bleeding (Greenberg et al., 2009a). On the contrary, cSS is a key SVD marker in CAA with high risk of lobar ICH (Charidimou et al., 2019). Neuropathological study of patients with CAA found that cSS was associated with advanced leptomeningeal vessel damage, but reduced cortical amyloid deposition (Charidimou et al., 2020). This study adds evidence to distinct disease entities of CMB and cSS. We observed an increasing CMB number in small ICH and higher prevalence of cSS in non-small ICH, but no significant association between SVD markers and lobar ICH volume was found in the multivariate logistic regression analysis. It is noted that only 57 (30.5%) patients with lobar ICH were included, thus this subgroup may be underrepresented in our analysis.
This study has important clinical implications. First, this study adds evidence to the association between the overall SVD burden and ICH volume. Future studies, including more SVD features such as cerebral microinfarct, may provide more information on the relationship between SVD severity and ICH volume. Second, this study gains insight into the potential role of different SVD markers to hematoma size. Traditional SVD markers, including WMH, lacune, and CMB, may restrict hematoma formation at the acute phase, whereas cSS is prone to active bleeding following superficial small vessel rupture.
There are several limitations. First, this is a single-center study with relatively small sample size, which may attenuate the generalizability. Second, patients with extremely lethal conditions did not receive MRI scan, which may cause selection bias. Third, the global SVD score may not cover the whole spectrum of SVD change. However, the global SVD score included well-recognized SVD markers with stratified severity such as WMH and CMB.
In summary, this study provides better insight into the influence of overall SVD severity to hematoma volume. An inverse correlation between global SVD score and ICH volume was observed. The associations remain significant for non-lobar ICH only. Future studies validate this relationship and exploring the pathophysiological mechanisms is required.
Data Availability Statement
The data that support the findings of this study are available from the corresponding author on reasonable request by contacting QL, qili_md@126.com.
Ethics Statement
The studies involving human participants were reviewed and approved by the Ethics Committee of the First Affiliated Hospital of Chongqing Medical University. The patients/participants provided their written informed consent to participate in this study.
Author Contributions
QL and Z-JW: study concept and design. Z-JW: statistical analysis and writing the manuscript. RZ, XH, W-SY, LD, X-NL, Z-QL, JC, and M-JP: acquisition of data. PX: administrative support. QL: critical revision of the manuscript for intellectual content and obtained funding. All authors: analysis and interpretation of data.
Funding
This study was supported by the National Natural Science Foundation of China (Grant No. 82071337), the National Key R&D Program of China (Grant Nos. 2018YFC1312200 and 2018YFC1312203), the Chongqing High-end Young Investigator Project (Grant No. 2019GDRC005), the Science and Technology Innovation Project of “Chengdu-Chongqing Economic Circle” (Grant No. KJCXZD2020019), and the Chongqing Innovation Support Program for Returned Overseas Chinese Scholars (Grant No. cx2020002).
Conflict of Interest
The authors declare that the research was conducted in the absence of any commercial or financial relationships that could be construed as a potential conflict of interest.
Publisher’s Note
All claims expressed in this article are solely those of the authors and do not necessarily represent those of their affiliated organizations, or those of the publisher, the editors and the reviewers. Any product that may be evaluated in this article, or claim that may be made by its manufacturer, is not guaranteed or endorsed by the publisher.
References
Akoudad, S., Portegies, M. L. P., Koudstaal, P. J., Hofman, A., Van Der Lugt, A., Ikram, M. A., et al. (2015). Cerebral Microbleeds Are Associated with an Increased Risk of Stroke: The Rotterdam Study. Circulation 132, 509–516. doi: 10.1161/CIRCULATIONAHA.115.016261
Boulouis, G., Van Etten, E. S., Charidimou, A., Auriel, E., Morotti, A., Pasi, M., et al. (2016). Association of key magnetic resonance imaging markers of cerebral small vessel disease with hematoma volume and expansion in patients with lobar and deep intracerebral hemorrhage. JAMA Neurol. 73, 1440–1447. doi: 10.1001/jamaneurol.2016.2619
Charidimou, A., Boulouis, G., Greenberg, S. M., and Viswanathan, A. (2019). Cortical superficial siderosis and bleeding risk in cerebral amyloid angiopathy: A meta-analysis. Neurology 93, e2192–e2202. doi: 10.1212/WNL.0000000000008590
Charidimou, A., Gang, Q., and Werring, D. J. (2012). Sporadic cerebral amyloid angiopathy revisited: Recent insights into pathophysiology and clinical spectrum. J. Neurol. Neurosurg. Psychiatry 83, 124–137. doi: 10.1136/jnnp-2011-301308
Charidimou, A., Imaizumi, T., Moulin, S., Biffi, A., Samarasekera, N., Yakushiji, Y., et al. (2017a). Brain hemorrhage recurrence, small vessel disease type, and cerebral microbleeds: A meta-analysis. Neurology 89, 820–829. doi: 10.1212/WNL.0000000000004259
Charidimou, A., Schmitt, A., Wilson, D., Yakushiji, Y., Gregoire, S. M., Fox, Z., et al. (2017b). The Cerebral Haemorrhage Anatomical RaTing inStrument (CHARTS): Development and assessment of reliability. J. Neurol. Sci. 372, 178–183. doi: 10.1016/j.jns.2016.11.021
Charidimou, A., Linn, J., Vernooij, M. W., Opherk, C., Akoudad, S., Baron, J. C., et al. (2015). Cortical superficial siderosis: detection and clinical significance in cerebral amyloid angiopathy and related conditions. Brain 138, 2126–2139. doi: 10.1093/brain/awv162
Charidimou, A., Perosa, V., Frosch, M. P., Scherlek, A. A., Greenberg, S. M., van Veluw, S. J., et al. (2020). Neuropathological correlates of cortical superficial siderosis in cerebral amyloid angiopathy. Brain 143, 3343–3351. doi: 10.1093/brain/awaa266
Cordonnier, C., Demchuk, A., Ziai, W., and Anderson, C. S. (2018). Intracerebral haemorrhage: current approaches to acute management. Lancet 392, 1257–1268. doi: 10.1016/S0140-6736(18)31878-6
Davis, S. M., Broderick, J., Hennerici, M., Brun, N. C., Diringer, M. N., Mayer, S. A., et al. (2006). Hematoma growth is a determinant of mortality and poor outcome after intracerebral hemorrhage. Neurology 66, 1175–1181. doi: 10.1212/01.wnl.0000208408.98482.99
Debette, S., Schilling, S., Duperron, M. G., Larsson, S. C., and Markus, H. S. (2019). Clinical Significance of Magnetic Resonance Imaging Markers of Vascular Brain Injury: A Systematic Review and Meta-analysis. JAMA Neurol. 76, 81–94. doi: 10.1001/jamaneurol.2018.3122
Doubal, F. N., MacLullich, A. M. J., Ferguson, K. J., Dennis, M. S., and Wardlaw, J. M. (2010). Enlarged perivascular spaces on MRI are a feature of cerebral small vessel disease. Stroke 41, 450–454. doi: 10.1161/STROKEAHA.109.564914
Dowlatshahi, D., Yogendrakumar, V., Aviv, R. I., Rodriguez-Luna, D., Molina, C. A., Silva, Y., et al. (2016). Small intracerebral hemorrhages have a low spot sign prevalence and are less likely to expand. Int. J. Stroke 11, 191–197. doi: 10.1177/1747493015616635
Evans, A., Demchuk, A., Symons, S. P., Dowlatshahi, D., Gladstone, D. J., Zhang, L., et al. (2010). The spot sign is more common in the absence of multiple prior microbleeds. Stroke 41, 2210–2217. doi: 10.1161/STROKEAHA.110.593970
Fazekas, F., Chawluk, J. B., and Alavi, A. (1987). MR signal abnormalities at 1.5 T in Alzheimer’s dementia and normal aging. Am. J. Roentgenol. 149, 351–356.
Fisher, C. M. (1971). Pathological observations in hypertensive cerebral hemorrhage. J. Neuropathol. Exp. Neurol. 30, 536–550. doi: 10.1097/00005072-197107000-00015
Greenberg, S. M., Kaveer Nandigam, R. N., Delgado, P., Betensky, R. A., Rosand, J., Viswanathan, A., et al. (2009a). Microbleeds versus macrobleeds: Evidence for distinct entities. Stroke 40, 2382–2386. doi: 10.1161/STROKEAHA.109.548974
Greenberg, S. M., Vernooij, M. W., Cordonnier, C., Viswanathan, A., Al-Shahi Salman, R., Warach, S., et al. (2009b). Cerebral microbleeds: a guide to detection and interpretation. Lancet Neurol. 8, 165–174. doi: 10.1016/S1474-4422(09)70013-4
Hansen, B. M., Ullman, N., Muschelli, J., Norrving, B., Dlugash, R., Avadhani, R., et al. (2020). Relationship of White Matter Lesions with Intracerebral Hemorrhage Expansion and Functional Outcome: MISTIE II and CLEAR III. Neurocrit. Care 33, 516–524. doi: 10.1007/s12028-020-00916-4
Kothari, R. U., Brott, T., Broderick, J. P., Barsan, W. G., Sauerbeck, L. R., Zuccarello, M., et al. (1996). The ABCs of Measuring Intracerebral Hemorrhage Volumes. Stroke 27, 1304–1305. doi: 10.1161/01.STR.27.8.1304
Lau, K. K., Li, L., Schulz, U., Simoni, M., Chan, K. H., Ho, S. L., et al. (2017). Total small vessel disease score and risk of recurrent stroke. Neurology 88, 2260–2267. doi: 10.1212/WNL.0000000000004042
Lee, B. C., Tsai, H. H., Huang, A. P. H., Lo, Y. L., Tsai, L. K., Chen, Y. F., et al. (2021). Arterial Spin Labeling Imaging Assessment of Cerebrovascular Reactivity in Hypertensive Small Vessel Disease. Front. Neurol. 12:640069. doi: 10.3389/fneur.2021.640069
Li, Q., Liu, Q. J., Yang, W. S., Wang, X. C., Zhao, L. B., Xiong, X., et al. (2017). Island sign: An imaging predictor for early hematoma expansion and poor outcome in patients with intracerebral hemorrhage. Stroke 48, 3019–3025. doi: 10.1161/STROKEAHA.117.017985
Li, Q., Shen, Y. Q., Xie, X. F., Xue, M. Z., Cao, D., Yang, W. S., et al. (2019a). Expansion-Prone Hematoma: Defining a Population at High Risk of Hematoma Growth and Poor Outcome. Neurocrit. Care 30, 601–608. doi: 10.1007/s12028-018-0644-3
Li, Q., Yang, W. S., Shen, Y. Q., Xie, X. F., Li, R., Deng, L., et al. (2019b). Benign Intracerebral Hemorrhage: A Population at Low Risk for Hematoma Growth and Poor Outcome. J. Am. Heart Assoc. 8:e011892. doi: 10.1161/JAHA.118.011892
Li, Q., Zhang, G., Huang, Y. J., Dong, M. X., Lv, F. J., Wei, X., et al. (2015). Blend Sign on Computed Tomography: Novel and Reliable Predictor for Early Hematoma Growth in Patients With Intracerebral Hemorrhage. Stroke 46, 2119–2123. doi: 10.1161/STROKEAHA.115.009185
Li, Q., Zhang, G., Xiong, X., Wang, X. C., Yang, W. S., Li, K. W., et al. (2016). Black hole sign: Novel imaging marker that predicts hematoma growth in patients with intracerebral hemorrhage. Stroke 47, 1777–1781. doi: 10.1161/STROKEAHA.116.013186
Lou, M., Al-Hazzani, A., Goddeau, R. P., Novak, V., and Selim, M. (2010). Relationship between white-matter hyperintensities and hematoma volume and growth in patients with intracerebral hemorrhage. Stroke 41, 34–40. doi: 10.1161/STROKEAHA.109.564955
Miller Fisher, C. (2003). Hypertensive cerebral hemorrhage. Demonstration of the source of bleeding. J. Neuropathol. Exp. Neurol. 62, 104–107. doi: 10.1093/jnen/62.1.104
Mitchell, G. F. (2008). Effects of central arterial aging on the structure and function of the peripheral vasculature: Implications for end-organ damage. J. Appl. Physiol. 105, 1652–1660. doi: 10.1152/japplphysiol.90549.2008
Pantoni, L. (2010). Cerebral small vessel disease: from pathogenesis and clinical characteristics to therapeutic challenges. Lancet Neurol. 9, 689–701. doi: 10.1016/S1474-4422(10)70104-6
Pasi, M., Sugita, L., Xiong, L., Charidimou, A., Boulouis, G., Pongpitakmetha, T., et al. (2021). Association of Cerebral Small Vessel Disease and Cognitive Decline After Intracerebral Hemorrhage. Neurology 96, e182–e192. doi: 10.1212/WNL.0000000000011050
Poon, M. T. C., Fonville, A. F., and Salman, R. A. S. (2014). Long-term prognosis after intracerebral haemorrhage: Systematic review and meta-analysis. J. Neurol. Neurosurg. Psychiatry 85, 660–667. doi: 10.1136/jnnp-2013-306476
Qureshi, A. I., Mendelow, A. D., and Hanley, D. F. (2009). Intracerebral haemorrhage. Lancet 373, 1632–1644. doi: 10.1016/S0140-6736(09)60371-8
Rodrigues, M. A., Samarasekera, N. E., Lerpiniere, C., Perry, L. A., Moullaali, T. J., Loan, J. M. J., et al. (2021). Association between Computed Tomographic Biomarkers of Cerebral Small Vessel Diseases and Long-Term Outcome after Spontaneous Intracerebral Hemorrhage. Ann. Neurol 89, 266–279. doi: 10.1002/ana.25949
Shoamanesh, A., Martinez-Ramirez, S., Oliveira-Filho, J., Reijmer, Y., Falcone, G. J., Ayres, A., et al. (2014). Interrelationship of superficial siderosis and microbleeds in cerebral amyloid angiopathy. Neurology 83, 1838–1843. doi: 10.1212/WNL.0000000000000984
Sykora, M., Herweh, C., and Steiner, T. (2017). The Association Between Leukoaraiosis and Poor Outcome in Intracerebral Hemorrhage Is Not Mediated by Hematoma Growth. J. Stroke Cerebrovasc. Dis. 26, 1328–1333. doi: 10.1016/j.jstrokecerebrovasdis.2017.02.003
Uniken Venema, S. M., Marini, S., Brouwers, H. B., Morotti, A., Woo, D., Anderson, C. D., et al. (2020). Associations of Radiographic Cerebral Small Vessel Disease with Acute Intracerebral Hemorrhage Volume, Hematoma Expansion, and Intraventricular Hemorrhage. Neurocrit. Care 32, 383–391. doi: 10.1007/s12028-019-00876-4
van Asch, C. J., Luitse, M. J., Rinkel, G. J., van der Tweel, I., Algra, A., and Klijn, C. J. (2010). Incidence, case fatality, and functional outcome of intracerebral haemorrhage over time, according to age, sex, and ethnic origin: a systematic review and meta-analysis. Lancet Neurol. 9, 167–176. doi: 10.1016/S1474-4422(09)70340-0
Wardlaw, J. M., Smith, E. E., Biessels, G. J., Cordonnier, C., Fazekas, F., Frayne, R., et al. (2013). Neuroimaging standards for research into small vessel disease and its contribution to ageing and neurodegeneration. Lancet Neurol. 12, 822–838. doi: 10.1016/S1474-4422(13)70124-8
Wilson, D., Ambler, G., Lee, K. J., Lim, J. S., Shiozawa, M., Koga, M., et al. (2019). Cerebral microbleeds and stroke risk after ischaemic stroke or transient ischaemic attack: a pooled analysis of individual patient data from cohort studies. Lancet Neurol. 18, 653–665. doi: 10.1016/S1474-4422(19)30197-8
Wu, T. Y., Yassi, N., Shah, D. G., Ma, M., Sharma, G., Putaala, J., et al. (2017). Simultaneous Multiple Intracerebral Hemorrhages (SMICH). Stroke 48, 581–586. doi: 10.1161/STROKEAHA.116.015186
Keywords: intracerebral hemorrhage, cerebral small vessel disease, computed tomography, magnetic resonance imaging, neuroimaging, stroke
Citation: Wang Z-J, Zhao R, Hu X, Yang W-S, Deng L, Lv X-N, Li Z-Q, Cheng J, Pu M-J, Tang Z-P, Wu G-F, Zhao L-B, Xie P and Li Q (2022) Higher Cerebral Small Vessel Disease Burden in Patients With Small Intracerebral Hemorrhage. Front. Neurosci. 16:888198. doi: 10.3389/fnins.2022.888198
Received: 02 March 2022; Accepted: 14 April 2022;
Published: 12 May 2022.
Edited by:
Hua Feng, Army Medical University, ChinaCopyright © 2022 Wang, Zhao, Hu, Yang, Deng, Lv, Li, Cheng, Pu, Tang, Wu, Zhao, Xie and Li. This is an open-access article distributed under the terms of the Creative Commons Attribution License (CC BY). The use, distribution or reproduction in other forums is permitted, provided the original author(s) and the copyright owner(s) are credited and that the original publication in this journal is cited, in accordance with accepted academic practice. No use, distribution or reproduction is permitted which does not comply with these terms.
*Correspondence: Qi Li, qili_md@126.com
‡These authors have contributed equally to this work
†ORCID: Zi-Jie Wang, orcid.org/0000-0001-7974-7075; Xiao Hu, orcid.org/0000-0002-1295-3293; Wen-Song Yang, orcid.org/0000-0003-3703-4565; Lan Deng, orcid.org/0000-0002-8243-4210; Xin-Ni Lv, orcid.org/0000-0002-0647-8844; Zuo-Qiao Li, orcid.org/0000-0002-4943-3101; Ming-Jun Pu, orcid.org/0000-0003-1304-1509; Zhou-Ping Tang, orcid.org/0000-0002-4153-8590; Qi Li, orcid.org/0000-0002-9144-148X