- 1School of Health Sciences, Massey University, Wellington, New Zealand
- 2School of Biological Sciences, Victoria University of Wellington, Wellington, New Zealand
- 3Department of Psychology, Victoria University of Wellington, Wellington, New Zealand
Tobacco dependence remains one of the major preventable causes of premature morbidity and mortality worldwide. There are well over 8,000 compounds present in tobacco and tobacco smoke, but we do not know what effect, if any, many of them have on smokers. Major interest has been on nicotine, as well as on toxic and carcinogenic effects and several major and minor components of tobacco smoke responsible for the negative health effects of smoking have been elucidated. Smokers themselves report a variety of positive effects from smoking, including effects on depression, anxiety and mental acuity. Smoking has also been shown to have protective effects in Parkinson’s Disease. Are the subjective reports of a positive effect of smoking due to nicotine, of some other components of tobacco smoke, or are they a manifestation of the relief from nicotine withdrawal symptoms that smoking provides? This mini-review summarises what is currently known about the components of tobacco smoke with potential to have positive effects on smokers.
Introduction
Smoking is a major cause of preventable premature death and disability, believed to cause six million deaths worldwide each year with smokers, on average, losing 10 years of their lives (West, 2017). The negative health effects of prolonged smoking are well established. Smoking primarily damages lung and cardiovascular health, as well as impacting negatively on every organ of the body (U.S. Department of Health and Human Services., 2004). The reason people keep smoking, despite knowing that this habit is likely to eventually kill them, is that smoking is addictive (Henningfield and Fant, 1999). The major addictive component of tobacco smoke is nicotine, but it is increasingly evident that tobacco dependence is multi-faceted (West and Cox, 2021). Nicotine in laboratory tests is much less addictive than the lived experience of smokers would suggest (Jain, 2003; Balfour, 2009). The explanations are varied, ranging from societal and behavioural influences (Moolchan et al., 2003), to strong cue association and cognitive effects (Sacco et al., 2004; Chiamulera, 2005) and to the influence of tobacco companies (Hoek et al., 2012).
Smokers themselves report smoking relieves stress and anxiety, and aids concentration (Benowitz, 2010) and smokers with a variety of mental health conditions report using smoking as a form of self-medication (Leonard et al., 2001; Aubin et al., 2012). Unsurprisingly, the interpretation of these reports varies widely. However, smoking is proven to have protective effects in Parkinson’s Disease (Castagnoli and Murugesan, 2004; Gigante et al., 2017), with smoking having a well-established neuroprotective effect against this disease (Veljkovic et al., 2018).
A strong theme coming through the literature is that components in tobacco smoke other than nicotine, possibly monoamine oxidase (MAO) inhibitors, may enhance tobacco dependence, or may have positive effects on mood (Fowler et al., 2003; Rose, 2006; Hogg, 2016; Harris et al., 2020). Thus, apart from the effects of nicotine, the observed difficulty that people have in stopping smoking could be influenced by other chemical components of the tobacco smoke. Such interpretations are controversial. For instance, the high rate of relapse in smokers attempting to stop smoking is attributed to relief from nicotine withdrawal, rather than thinking of a positive effect on mood or concentration from smoking as reinforcing nicotine dependence (Moylan et al., 2013).
It is completely accepted that tobacco smoking is a harmful habit, due to the many toxic and carcinogenic components of the smoke. However, this review aims to focus attention on areas of the literature suggesting pharmacological drivers behind tobacco dependence other than the immediate effects of nicotine in inducing dependence, and our current understanding of the short-term effects of tobacco smoke components on smokers. Are some of these effects positive, as in neuroprotection against Parkinson’s Disease? Are there known tobacco components which could have a positive effect?
Nicotine
Nicotine acts at the nicotinic acetylcholine receptors (nAChRs) to cause flow-on effects in specific areas of the brain. These nAChRs are believed to be important in coordinating brain responses, by action of the natural transmitter, acetylcholine (ACh). Nicotine binds to nAChRs mimicking the action of ACh and altering responses within the brain (Balfour, 2009).
The key addictive response is stimulation of nAChRs in the ventral tegmental area of the brain, which causes the release of dopamine in the nucleus accumbens, believed to be central to the development of all addictive responses (Di Chiara and Imperato, 1988). Nicotine’s pharmacology has been well reviewed by others (Benowitz, 1996; Laviolette and van der Kooy, 2004) as have the complexities of the nicotinic receptors in the brain (Albuquerque et al., 2009; Wu and Lukas, 2011). The nAChRs belong to the ionotropic family of receptors, with five proteins forming a channel. Seventeen different proteins have been identified, leading to a wide diversity of nicotinic receptor subtypes. Thus the diversity of nicotinic receptor types and their varied localisation within the brain allows for much more nuanced and complex responses to nicotine than simple dopamine release.
Several milligrams of nicotine are present in each gram of tobacco, and nicotine reaches around 0.2 micromolar concentrations in the blood of smokers, sufficient to cause nAChR responses (Alkondon et al., 2000). Nicotine concentrations rise rapidly when tobacco smoke is inhaled, reaching the brain in under two minutes. It then dissipates slowly, having a half-life of around 2 h (Benowitz, 2009). As nicotine brain concentrations fall, in the addicted smoker, cravings for nicotine begin, leading to smokers repeating the experience. Many of the effects of smoking tobacco (dopamine release and dependence, and withdrawal effects and relapse back to smoking) can be related back to these key effects of nicotine on the brain.
As the major pharmacologically active component of tobacco smoke, nicotine has also been investigated to see whether it can cause other effects, reported from smoking, such as relief from anxiety and depression, improved concentration and symptom control in Schizophrenia, Parkinson’s Disease, Attention Deficit Hyperactivity Disorder, and Alzheimer’s Disease (Mihailescu and Drucker-Colin, 2000; Newhouse et al., 2004a; Veljkovic et al., 2018).
Nicotine and Cognition/Concentration
In utero exposure and exposure of children to tobacco smoke are both believed to interfere with cognitive development, causing deleterious effects on attention span and ability to concentrate, in children (Alhowail, 2021; Hajdusianek et al., 2021). With some caveats as to the strength of the evidence (Chan et al., 2020) it is generally accepted that smoking in pregnancy is bad for the unborn child, although there is some doubt as to whether nicotine causes all of the problems noted (Baler et al., 2008; Chan et al., 2020).
In adults, however, nicotine is believed to have a positive effect on mental acuity (Conley et al., 2021; Nop et al., 2021). Again, this has been suggested by studies in animals and in humans (Kumari et al., 2003; Newhouse et al., 2004b), and trials of the effect of nicotine in older adults have produced some evidence of benefit. A recent meta-analysis by Majdi and coworkers suggested that nicotine has a moderate but positive effect on attentional ability in healthy non-smoking adults (Majdi et al., 2021) and it has been suggested that nicotine could be used to treat late-life depression, with part of this action being mediated by effects on cognition (Gandelman et al., 2018).
Smoking as a long-term enhancer of cognition is not recommended, however, since smoking is a risk factor for vascular dementia (Lopez-Arrieta et al., 2001) as well as for many other health conditions.
Nicotine and Anxiety/Depression
The suggestion that nicotine can relieve stress and help with anxiety and depression is controversial. The key argument is whether the positive effects noted by smokers are real and act as a reinforcer of tobacco dependence by improving mood (Pomerleau et al., 1984; Choi et al., 2015) or whether nicotine dependence causes depression and/or anxiety over time, with relief of cravings being misread by smokers as relief from the related mood disorders (Moylan et al., 2013; Molas et al., 2017). A systematic review by Fluharty and co-workers (Fluharty et al., 2017) found evidence for causation in both directions and suggested the need for more studies. More recent work tends to look at effects of transdermal nicotine, and has found potential for nicotine to be used in cases of major depressive disorder (Janes et al., 2018), and for relief of later life depression (Conley et al., 2021).
Nicotine and Schizophrenia
Schizophrenia is also strongly associated with tobacco smoking, however, the direction of causation is controversial. Scott and coworkers examined the evidence that smoking might cause schizophrenia and suggested nicotine as the causative agent (Scott et al., 2018). Others have suggested that nicotine’s effects within the brain might give relief from the symptoms of schizophrenia, providing motivation to continue smoking (Postma et al., 2006). It is possible that nicotine’s positive effect on cognition is the mediating mechanism for this (Waterhouse et al., 2018).
Nicotine and Alzheimer’s Disease
The possibility that nicotine might be useful in treating early stages of Alzheimer’s Disease has been studied for some years. As with Parkinson’s Disease, smokers are under-represented among those with Alzheimer’s Disease. Impaired cholinergic function is an early feature of Alzheimer’s Disease and nAChR agonists have been suggested as potentially helpful in ameliorating symptoms (Albuquerque et al., 2001). Transdermal nicotine has been used in trials with positive effects on attention and other cognitive measures (Newhouse et al., 2004b). Nicotine has also been shown to be effective in reducing behavioural and synaptic plasticity deficits in a rat model of Alzheimer’s disease (Esteves et al., 2017). In a separate line of enquiry nicotine is also thought to delay formation of amyloid plaque (Zhang et al., 2006), giving another mechanism by which smoking (nicotine) could be helpful in delaying Alzheimer’s Disease onset.
Nicotine and Parkinson’s Disease
While smoking is well known to be protective against Parkinson’s Disease (Gigante et al., 2017), the causative agents in this case are likely to include both nicotine, though its action on dopaminergic pathways (Thiriez et al., 2011), and monoamine oxidase inhibitors (Castagnoli and Murugesan, 2004). Additionally, Kardani et al. (2017) have demonstrated that nicotine can slow the formation of α-synuclein fibrils. However, although nicotine appeared to be neuroprotective in Parkinsonian animals it had no effect in restoring damage in the same experimental system (Huang et al., 2009) and was not significantly effective in Phase II clinical trials (Villafane et al., 2018).
Minor Tobacco Alkaloids
Like nicotine, the other nicotine analogues found in tobacco smoke have also been found to act on the nicotinic receptors. The “minor alkaloids” in tobacco smoke other than nicotine include nornicotine, myosmine, cotinine, anabasine and anatabine. Where nicotine is found at around 10 milligrams per cigarette in tobacco, these minor alkaloids are found in microgram per cigarette amounts (Smith et al., 2015).
Several groups have looked at the effect of these alkaloids in vivo (Harris et al., 2015; Marusich et al., 2017; Tan et al., 2022). Such studies have found that the minor tobacco alkaloids can partially substitute for nicotine in behavioural tests but have lower potency than nicotine itself. The different alkaloids are not equivalent in their behavioural effects. Differences in binding to different nAChR variants between the various nicotinic alkaloids would allow for differences in mode of action and potency even if all act through binding nAChRs. The combination of lower potency and lower concentration in tobacco make it unlikely that these alkaloids have significant effects on smoker behaviour.
Cotinine, a breakdown product of nicotine, has relatively long half-life in the blood [c.a. 16 h compared to c.a. 2 h for nicotine (Hukkanen et al., 2005)] and reaches low micromolar concentrations in a smoker’s blood, several-fold higher than the concentration of nicotine. It may, therefore, be present in sufficient amounts to have a modulating effect on smoker behaviour, particularly for heavy smokers. Cotinine is a weak agonist of nAChRs, binding less strongly than nicotine (Tan et al., 2021) but has recently been shown to support self-administrative behaviour (Tan et al., 2022) although less robustly than nicotine. Cotinine upregulates the α7nAChRs and this activity is neuroprotective to glial cells (Iarkov et al., 2021). Cotinine also slows down the formation of α-synuclein fibrils, with a potency similar to that of nicotine, and may also have positive cognitive benefits.
Table 1 summarises the suggested beneficial effects of nicotine and cotinine.
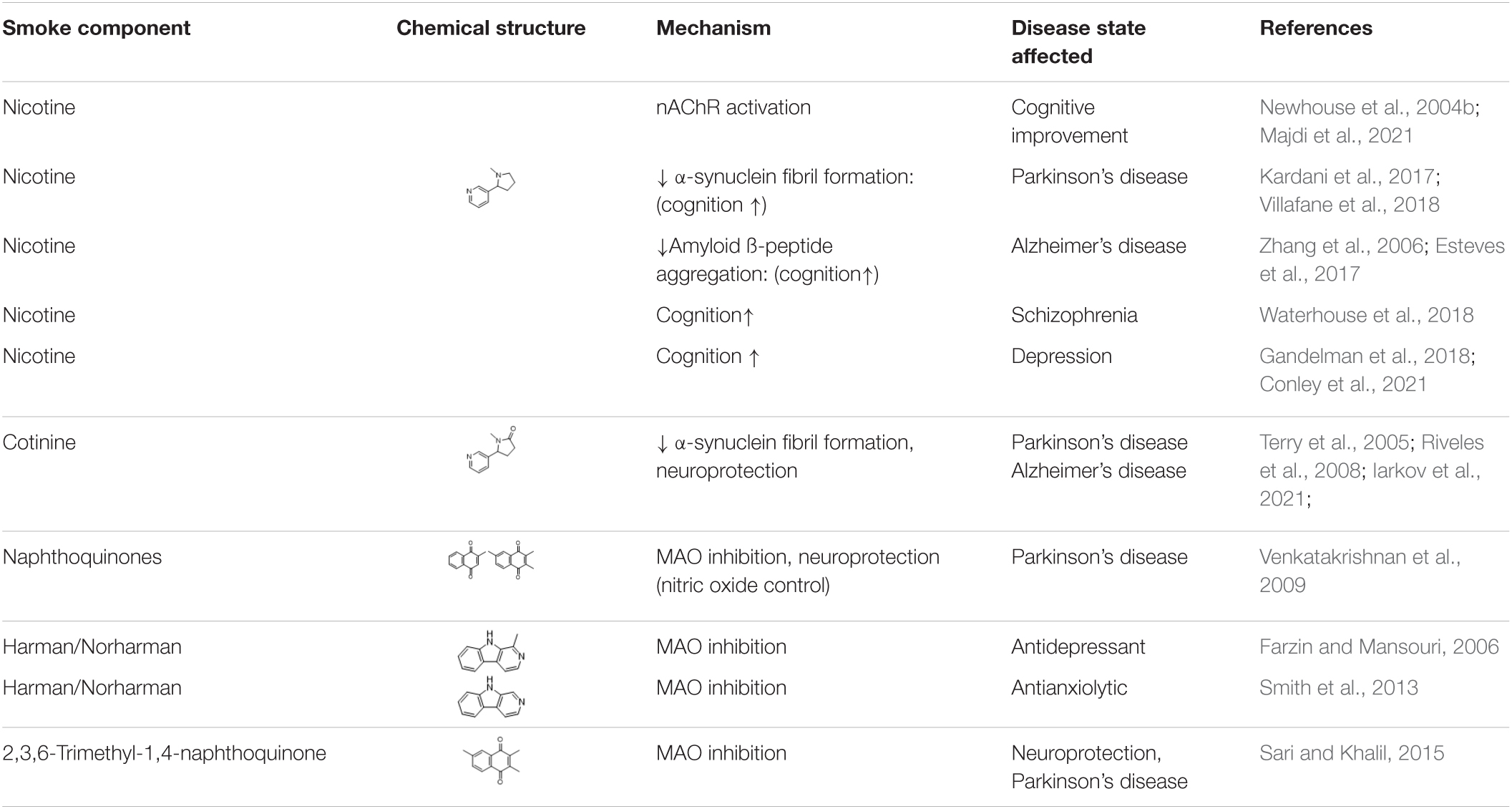
Table 1. Nicotine, cotinine, and monoamine oxidase inhibitors in tobacco and tobacco smoke: potential positive effects.
Monoamine Oxidase Inhibitors
Monoamine oxidase inhibitors (MAOIs) in tobacco smoke have long been regarded as having potential significance in modulating the effects of smoking on the brain (Fowler et al., 2003; Dome et al., 2010; Hogg, 2016). Monoamine oxidase (MAO) enzymes in the brain are responsible for clearance of brain transmitters, notably dopamine, serotonin, adrenaline and noradrenaline (Lewis et al., 2007). Since inhibition of MAO activity will lead to reduced clearance of neurotransmitters such as dopamine it is suggested that MAOIs in the tobacco smoke might enhance the dopamine reward from nicotine and the addictiveness of smoking.
A variety of experimental evidence has been produced showing that MAO inhibition enhances behavioural responses to nicotine in rats (Guillem et al., 2005; Villegier et al., 2006, 2011; Smith et al., 2016b; Harris et al., 2020) but the extension to human smoking behaviour is less clear. MAO activity is well known to be reduced in smokers (Fowler et al., 1996a,b) with the inhibition being believed to be irreversible (Yu and Boulton, 1987). The time course of recovery of activity after smoking cessation extends over several weeks after smoking cessation, consistent with the equivalent recovery time from inhibition by known irreversible MAO inhibitory drugs (Fowler et al., 2003). Although epigenetic (Launay et al., 2009) and microRNA (Higuchi et al., 2018) mechanisms for MAO activity reduction have been suggested, direct inhibition by components of tobacco smoke as the major cause of the observed reduction remains a likely mechanism by which MAO inhibition is accomplished in smokers.
The known MAOIs in tobacco smoke include the ß-carbolines harman and norharman, α-naphthylamine, farnesyl acetone and tetrahydroisoquinolines (TIQ’s) (Lewis et al., 2007; Hogg, 2016). Harman and norharman have been proposed as the major contributors to the observed MAOI activity in tobacco smoke, causing the observed decrease in MAO activity (Rommelspacher et al., 2002; Herraiz and Chaparro, 2005), with the discrepancy between the amounts of ß-carboline measured in smokers, and the inhibition observed being ascribed to their accumulation in platelets (Rommelspacher et al., 2002) and in brain (Fekkes and Bode, 1993). However, harman and norharman make up less than 1/10th of the total direct MAO inhibitory activity in tobacco smoke (Truman et al., 2017) so the opportunity for other MAOIs in tobacco smoke to contribute substantially to the MAO activity reduction seen in smokers must exist. No irreversible MAO inhibitors have yet been reported from tobacco or tobacco smoke.
The question of whether MAO inhibitors in tobacco smoke can affect behaviour remains unresolved. As well as the potential for effects on addiction, MAO enzymes are drug targets for a variety of neurological disorders including depression, mood, anxiety, attention deficit hyperactivity, Tourette’s syndrome, Parkinson’s disease and Alzheimer’s disease (Sharama, 2016; Borroni et al., 2017). Of the known MAO inhibitors in tobacco smoke, high concentrations of harman and norharman can affect responses to nicotine (Harris et al., 2020) and may act as antidepressants (Farzin and Mansouri, 2006; Smith et al., 2013) in animals. However, when used in amounts relevant to smokers, they were not seen to affect rat self-administration of nicotine (Smith et al., 2015) and no pharmacological effects have been reported from the known tobacco MAO inhibitors at physiologically relevant concentrations. In contrast, use of tobacco smoke extracts in self-administration or intracranial self-stimulation experiments has been found to affect responses to nicotine (Harris et al., 2010; Costello et al., 2014; Brennan et al., 2015). This discrepancy may be in part because the full range of tobacco smoke MAO inhibitors has not yet been identified.
It has also been suggested that further MAO inhibitory activity is formed from smoke components in the body. Acetaldehyde is formed during tobacco combustion, from the sugars in the plant material, and from sugars added as humectants and flavour additives during tobacco and cigarette manufacture. Acetaldehyde is typically present in cigarette smoke amounts ranging from 0.6 to over 2 milligrams per cigarette (Seeman et al., 2002). Acetaldehyde yields locomotor stimulation and reinforcing effects (Quertemont and Tambour, 2004) at concentrations higher than those seen in tobacco smoke and enhances self-administration of nicotine at concentrations similar to those in tobacco smoke (Belluzzi et al., 2005), although this finding was not confirmed by Smith and co-workers in similar trials (Smith et al., 2015).
It is suggested that acetaldehyde acts by reacting with chemicals naturally occurring in the brain to form MAO inhibitors (Talhout et al., 2007). A wide variety of TIQs are formed from acetaldehyde and catecholamines (dopamine, noradrenalin, adrenalin) (Naoi et al., 2004; Patsenka and Antkiewicz-Michaluk, 2004). Of particular note are the cyano derivatives, which inhibit MAO-A and -B with Ki values between 18 and 38 μM (Mendez-Alvarez et al., 1997). A group of tetrahydro-ß-carbolines (THBCs) are also formed from condensation of acetaldehyde and indoleamines (serotonin, tryptamine, tryptophan) (Talhout et al., 2007) and are more potent, inhibiting with Ki values under 10 μM. It seems likely that concentrations of these MAOIs, formed in the body after smoking, are sufficient to have some effect on smoking addiction in humans, as well as in rats, since a randomized double-blind trial of a method to reduce the amount of acetaldehyde entering a smoker’s body had some success in encouraging cessation (Syrjanen et al., 2017). Thus far, together with the ß-carbolines, acetaldehyde is a leading candidate as a tobacco smoke component causing monoamine oxidase inhibition in smokers sufficient to modulate tobacco dependence, even though it does not directly cause MAO inhibition. The inhibitors formed from acetaldehyde appear to be reversible (Naoi et al., 2004).
The immediate effect on MAO activity of smoking a single cigarette could not be detected using PET methods (Fowler et al., 2003), whereas longer term effects of continued smoking results in an overall, apparently irreversible reduction of 30–40% in both MAO-A and -B activity, suggesting that long term exposure to tobacco smoke is required for this effect. Until we know which components of tobacco smoke cause the observed inhibition of MAO activity, and their mechanism of action, it will remain difficult to determine the extent, timing and overall effects of MAO inhibition in smokers.
Monoamine oxidase inhibitors in tobacco smoke may well have effects other than enhancing the addictive potential of nicotine. Smith and coworkers (Smith et al., 2016a) have examined the effects of MAO inhibitors and nicotine on brain function, measured using EEG techniques in non-smoking humans. They suggest that MAO inhibition alters brain function leading to lapses in cognition. Nicotine’s effect in enhancing cognition is suggested to alleviate this.
Monoamine oxidase inhibitors are a major drug target, of interest for treatment of depression and anxiety, Parkinson’s disease and Alzheimer’s disease (Sharama, 2016). It has been suggested that the MAOI activity in tobacco smoke may enhance a smoker’s mood (Pomerleau et al., 1984; Farzin and Mansouri, 2006; Smith et al., 2013; Choi et al., 2015) independently of their effects on dopamine reward from nicotine, and may contribute to smoker’s dependence on tobacco by this indirect route (Arnold et al., 2014). However, rather than a positive effect on mood, it is possible that MAO inhibition serves to intensify withdrawal symptoms (Malin et al., 2013). Relief of withdrawal symptoms then serves to improve mood. This potential interplay of influences is not yet fully understood.
The potential role for MAO inhibition in tobacco dependence has led to suggestions that MAO inhibitors could be used for smoking cessation (Biberman et al., 2003; George and Weinberger, 2008). Current consensus is that, after some promising preliminary results, the known MAO inhibitory drugs are not particularly useful for smoking cessation (Howes et al., 2020).
The proposed effects of MAO inhibitors on smokers are listed in Table 1.
Other Components of Tobacco Smoke
Additional components of tobacco and tobacco smoke which have been associated with beneficial effects are listed in Table 2, below. They have been identified as a result of investigations of the active components supporting herbal use, but from plants other than tobacco. These compounds are all known components of tobacco (Rodgman and Perfetti, 2013), however, while their biological activity is of interest, their contribution to the overall effects of tobacco smoking is unknown.
Conclusion
It is accepted that nicotine has positive effects on cognition and attention in adults, even though having negative effects in infant and child development. For this reason, nicotine could be helpful in the management of a variety of mental health conditions and provide an explanation for high levels of smoking in disorders such as schizophrenia. Nicotine and its metabolite cotinine both stimulate cholinergic systems, which can be neuroprotective, and may also prevent ß-amyloid fibre aggregation in Alzheimer’s disease, and α-synuclein fibre formation in Parkinson’s disease. While the long-term overall effect of smoking is deleterious, the same may not be true for nicotine and cotinine. The therapeutic use of cotinine deserves further work, since it is expected to have a lower abuse potential than nicotine but may provide similar neuroprotective and cognitive effects.
The other most significant and potentially beneficial biological activity in tobacco smoke is monoamine oxidase inhibitory activity. This is likely to have an impact on the response to nicotine, and to increase the addictiveness of smoking, both by increasing the dopamine rewards from the nicotine, and by its effects (if any) on mood. The extent to which these effects on mood from smoking are due to relief of nicotine cravings, or directly caused by the modulation of monoamine oxidase enzyme activity in the brain remains uncertain. In this respect it is important to recall that the first generation of antidepressant drugs were selective MAO inhibitors, with some still currently used for treatment of major depressive disorders. This question will only be resolved when the causative agents of the MAO inhibition seen in smokers have been identified, and their effects can be studied independently of the effects of nicotine. It may prove possible to separate immediate MAO inhibitory activity from the long-term irreversible inhibitory factors and assess their effects separately once any irreversible inhibitors have been identified.
It is likely that MAO-B inhibitory activity in tobacco smoke, perhaps together with the combined neuroprotective effect of a variety of smoke components may be useful for symptom control in Parkinson’s disease, while MAO-A inhibitory activity is more likely to contribute to the alleviation of mood disorders.
While the contribution of biologically active molecules other than nicotine remains to be established it is clear that tobacco smoke contains many components which might have beneficial effects. While this in no way compensates for the overall deleterious effects of smoking, these effects may help explain the strength of tobacco dependence many smokers experience and are worthy of further study, so that we can disentangle the deleterious and beneficial effects, to better help smokers to stop smoking.
Author Contributions
PT, RP, and PT-S guided the initial literature search, performed by SH. SH and PT wrote the manuscript. BE added expert advice. All authors contributed to the final shape of the manuscript.
Funding
SH was supported by a Massey University Ph.D. scholarship. Publishing fees are provided via a grant from the Ministry of Business, Innovation and Enterprise, MAUX1904.
Conflict of Interest
The authors declare that the research was conducted in the absence of any commercial or financial relationships that could be construed as a potential conflict of interest.
Publisher’s Note
All claims expressed in this article are solely those of the authors and do not necessarily represent those of their affiliated organizations, or those of the publisher, the editors and the reviewers. Any product that may be evaluated in this article, or claim that may be made by its manufacturer, is not guaranteed or endorsed by the publisher.
References
Albuquerque, E. X., Pereira, E. F., Alkondon, M., and Rogers, S. W. (2009). Mammalian nicotinic acetylcholine receptors: from structure to function. Physiol. Rev. 89, 73–120. doi: 10.1152/physrev.00015.2008
Albuquerque, E. X., Santos, M. D., Alkondon, M., Pereira, E. F., and Maelicke, A. (2001). Modulation of nicotinic receptor activity in the central nervous system: a novel approach to the treatment of Alzheimer disease. Alzheimer Dis. Assoc. Disord. 15, S19–S25. doi: 10.1097/00002093-200108001-00004
Alhowail, A. H. (2021). Mechanisms underlying cognitive impairment induced by prenatal nicotine exposure: a literature review. Eur. Rev. Med. Pharmacol. Sci. 25, 6057–6064. doi: 10.26355/eurrev_202110_26884
Alkondon, M., Pereira, E. F., Almeida, L. E., Randall, W. R., and Albuquerque, E. X. (2000). Nicotine at concentrations found in cigarette smokers activates and desensitizes nicotinic acetylcholine receptors in CA1 interneurons of rat hippocampus. Neuropharmacol 39, 2726–2739. doi: 10.1016/s0028-3908(00)00156-8
Arnold, M. M., Loughlin, S. E., Belluzzi, J. D., and Leslie, F. M. (2014). Reinforcing and neural activating effects of norharmane, a non-nicotine tobacco constituent, alone and in combination with nicotine. Neuropharmacol 85, 293–304. doi: 10.1016/j.neuropharm.2014.05.035
Aubin, H. J., Rollema, H., Svensson, T. H., and Winterer, G. (2012). Smoking, quitting, and psychiatric disease: a review. Neurosci. Biobehav. Rev. 36, 271–284. doi: 10.1016/j.neubiorev.2011.06.007
Baler, R. D., Volkow, N. D., Fowler, J. S., and Benveniste, H. (2008). Is fetal brain monoamine oxidase inhibition the missing link between maternal smoking and conduct disorders? J. Psychiatr. Neurosci. 33, 187–195.
Balfour, D. J. (2009). The neuronal pathways mediating the behavioral and addictive properties of nicotine. Handb. Exp. Pharmacol. 192, 209–233. doi: 10.1007/978-3-540-69248-5_8
Belluzzi, J. D., Wang, R., and Leslie, F. M. (2005). Acetaldehyde enhances acquisition of nicotine self-administration in adolescent rats. Neuropsychopharmacol 30, 705–712. doi: 10.1038/sj.npp.1300586
Benowitz, N. L. (1996). Pharmacology of nicotine: Addiction and therapeutics. Annu. Rev. Pharmacol. Toxicol. 36, 597–613. doi: 10.1146/annurev.pa.36.040196.003121
Benowitz, N. L. (2009). Pharmacology of nicotine: addiction, smoking-induced disease, and therapeutics. Annu. Rev. Pharmacol. Toxicol. 49, 57–71. doi: 10.1146/annurev.pharmtox.48.113006.094742
Benowitz, N. L. (2010). Nicotine Addiction. New England J. Med. 362, 2295–2303. doi: 10.1056/NEJMra0809890
Biberman, R., Neumann, R., Katzir, I., and Gerber, Y. (2003). A randomized controlled trial of oral selegiline plus nicotine skin patch compared with placebo plus nicotine skin patch for smoking cessation. Addiction 98, 1403–1407. doi: 10.1046/j.1360-0443.2003.00524.x
Borroni, E., Bohrmann, B., Grueninger, F., Prinssen, E., Nave, S., and Loetscher, H. (2017). Sembragiline: A Novel, Selective Monoamine Oxidase Type B Inhibitor for the Treatment of Alzheimer’s Disease. J. Pharmacol. Exp. Ther. 362, 413–423. doi: 10.1124/jpet.117.241653
Brennan, K. A., Crowther, A., Putt, F., Roper, V., Waterhouse, U., and Truman, P. (2015). Tobacco particulate matter self-administration in rats: differential effects of tobacco type. Addict Biol. 20, 227–235. doi: 10.1111/adb.12099
Castagnoli, K., and Murugesan, T. (2004). Tobacco leaf, smoke and smoking, MAO inhibitors, Parkinson’s disease and neuroprotection; are there links? Neurotoxicol. 25, 279–291. doi: 10.1016/S0161-813X(03)00107-4
Chan, Y. L., Oliver, B. G., and Chen, H. (2020). What lessons have we learnt about the impact of maternal cigarette smoking from animal models? Clin. Exp. Pharmacol. Physiol. 47, 337–344. doi: 10.1111/1440-1681.13182
Chang, W., and Teng, J. (2018). Combined application of tenuigenin and beta-asarone improved the efficacy of memantine in treating moderate-to-severe Alzheimer’s disease. Drug Des. Devel. Ther. 12, 455–462. doi: 10.2147/DDDT.S155567
Chen, J., Lin, D., Zhang, C., Li, G., Zhang, N., Ruan, L., et al. (2015). Antidepressant-like effects of ferulic acid: involvement of serotonergic and norepinergic systems. Metab. Brain Dis. 30, 129–136. doi: 10.1007/s11011-014-9635-z
Chiamulera, C. (2005). Cue reactivity in nicotine and tobacco dependence: a “multiple-action” model of nicotine as a primary reinforcement and as an enhancer of the effects of smoking-associated stimuli. Brain Res. Rev. 48, 74–97. doi: 10.1016/j.brainresrev.2004.08.005
Choi, D., Ota, S., and Watanuki, S. (2015). Does cigarette smoking relieve stress? Evidence from the event-related potential (ERP). Int. J. Psychophysiol. 98, 470–476. doi: 10.1016/j.ijpsycho.2015.10.005
Conley, A. C., Key, A. P., Taylor, W. D., Albert, K. M., Boyd, B. D., Vega, J. N., et al. (2021). EEG as a Functional Marker of Nicotine Activity: Evidence From a Pilot Study of Adults With Late-Life Depression. Front. Psychiatr. 12:721874. doi: 10.3389/fpsyt.2021.721874
Costello, M. R., Reynaga, D. D., Mojica, C. Y., Zaveri, N. T., Belluzzi, J. D., and Leslie, F. M. (2014). Comparison of the reinforcing properties of nicotine and cigarette smoke extract in rats. Neuropsychopharmacol 39, 1843–1851. doi: 10.1038/npp.2014.31
Dhanalakshmi, C., Janakiraman, U., Manivasagam, T., Justin Thenmozhi, A., Essa, M. M., Kalandar, A., et al. (2016). Vanillin Attenuated Behavioural Impairments, Neurochemical Deficts, Oxidative Stress and Apoptosis Against Rotenone Induced Rat Model of Parkinson’s Disease. Neurochem. Res. 41, 1899–1910. doi: 10.1007/s11064-016-1901-5
Di Chiara, G., and Imperato, A. (1988). Drugs abused by humans preferentially increase synaptic dopamine concentrations in the mesolimbic system of freely moving rats. Proc. Natl. Acad. Sci. U.S.A. 85, 5274–5278. doi: 10.1073/pnas.85.14.5274
Dome, P., Lazary, J., Kalapos, M. P., and Rihmer, Z. (2010). Smoking, nicotine and neuropsychiatric disorders. Neurosci. Biobehav. Rev. 34, 295–342. doi: 10.1016/j.neubiorev.2009.07.013
Esteves, I. M., Lopes-Aguiar, C., Rossignoli, M. T., Ruggiero, R. N., Broggini, A. C. S., and Bueno-Junior, L. S. (2017). Chronic nicotine attenuates behavioral and synaptic plasticity impairments in a streptozotocin model of Alzheimer’s disease. Neurosci 353, 87–97. doi: 10.1016/j.neuroscience.2017.04.011
Farzin, D., and Mansouri, N. (2006). Antidepressant-like effect of harmane and other beta-carbolines in the mouse forced swim test. Eur. Neuropsychopharmacol. 16, 324–328. doi: 10.1016/j.euroneuro.2005.08.005
Fekkes, D., and Bode, W. T. (1993). Occurrence and partition of the β-carboline norharman in rat organs. Life Sci. 52, 2045–2054. doi: 10.1016/0024-3205(93)90689-Z
Ferchmin, P. A., Pagan, O. R., Ulrich, H., Szeto, A. C., Hann, R. M., and Eterovic, V. A. (2009). Actions of octocoral and tobacco cembranoids on nicotinic receptors. Toxicon 54, 1174–1182. doi: 10.1016/j.toxicon.2009.02.033
Fluharty, M., Taylor, A. E., Grabski, M., and Munafo, M. R. (2017). The Association of Cigarette Smoking With Depression and Anxiety: A Systematic Review. Nicotine. Tob. Res. 19, 3–13. doi: 10.1093/ntr/ntw140
Fowler, J. S., Logan, J., Wang, G. J., and Volkow, N. D. (2003). Monoamine oxidase and cigarette smoking. Neurotoxicol 24, 75–82. doi: 10.1016/s0161-813x(02)00109-2
Fowler, J. S., Volkow, N. D., Wang, G. J., Pappas, N., Logan, J., and MacGregor, R. (1996a). Inhibition of monoamine oxidase B in the brains of smokers. Nature 379, 733–736. doi: 10.1038/379733a0
Fowler, J. S., Volkow, N. D., Wang, G. J., Pappas, N., Logan, J., and Shea, C. (1996b). Brain monoamine oxidase A inhibition in cigarette smokers. Proc. Natl. Acad. Sci. U.S.A. 93, 14065–14069. doi: 10.1073/pnas.93.24.14065
Gandelman, J. A., Newhouse, P., and Taylor, W. D. (2018). Nicotine and networks: Potential for enhancement of mood and cognition in late-life depression. Neurosci. Biobehav. Rev. 84, 289–298. doi: 10.1016/j.neubiorev.2017.08.018
George, T. P., and Weinberger, A. H. (2008). Monoamine oxidase inhibition for tobacco pharmacotherapy. Clin. Pharmacol. Ther. 83, 619–621. doi: 10.1038/sj.clpt.6100474
Gigante, A. F., Martino, T., Iliceto, G., and Defazio, G. (2017). Smoking and age-at-onset of both motor and non-motor symptoms in Parkinson’s disease. Parkinsonism Relat. Disord. 45, 94–96. doi: 10.1016/j.parkreldis.2017.09.022
Guillem, K., Vouillac, C., Azar, M. R., Parsons, L. H., Koob, G. F., Cador, M., et al. (2005). Monoamine oxidase inhibition dramatically increases the motivation to self-administer nicotine in rats. J. Neurosci. 25, 8593–8600. doi: 10.1523/JNEUROSCI.2139-05.2005
Hajdusianek, W., Zorawik, A., Waliszewska-Prosol, M., Poreba, R., and Gac, P. (2021). Tobacco and Nervous System Development and Function-New Findings 2015-2020. Brain Sci. 11:797. doi: 10.3390/brainsci11060797
Han, Y., Wang, N., Kang, J., and Fang, Y. (2020). beta-Asarone improves learning and memory in Abeta1-42-induced Alzheimer’s disease rats by regulating PINK1-Parkin-mediated mitophagy. Metab. Brain Dis. 35, 1109–1117. doi: 10.1007/s11011-020-00587-2
Harris, A. C., Mattson, C., LeSage, M. G., Keyler, D. E., and Pentel, P. R. (2010). Comparison of the behavioral effects of cigarette smoke and pure nicotine in rats. Pharmacol. Biochem. Behav. 96, 217–227. doi: 10.1016/j.pbb.2010.05.008
Harris, A. C., Muelken, P., and LeSage, M. G. (2020). beta-Carbolines found in cigarette smoke elevate intracranial self-stimulation thresholds in rats. Pharmacol. Biochem. Behav. 198:173041. doi: 10.1016/j.pbb.2020.173041
Harris, A. C., Tally, L., Muelken, P., Banal, A., Schmidt, C. E., Cao, Q., et al. (2015). Effects of nicotine and minor tobacco alkaloids on intracranial-self-stimulation in rats. Drug Alcohol Depend 153, 330–334. doi: 10.1016/j.drugalcdep.2015.06.005
Henningfield, J. E., and Fant, R. V. (1999). Tobacco use as drug addiction: the scientific foundation. NicotineTob. Res. 1, S31–S35. doi: 10.1080/14622299050011781
Herraiz, T., and Chaparro, C. (2005). Human monoamine oxidase is inhibited by tobacco smoke: beta-carboline alkaloids act as potent and reversible inhibitors. Biochem. Biophys. Res. Commun. 326, 378–386. doi: 10.1016/j.bbrc.2004.11.033
Higuchi, Y., Soga, T., and Parhar, I. S. (2018). Potential Roles of microRNAs in the Regulation of Monoamine Oxidase A in the Brain. Front. Mol. Neurosci. 11:339. doi: 10.3389/fnmol.2018.00339
Hoek, J., Gendall, P., Gifford, H., Pirikahu, G., McCool, J., Pene, G., et al. (2012). Tobacco branding, plain packaging, pictorial warnings, and symbolic consumption. Qual. Health Res. 22, 630–639. doi: 10.1177/1049732311431070
Hogg, R. C. (2016). Contribution of Monoamine Oxidase Inhibition to Tobacco Dependence: A Review of the Evidence. Nicotine Tob. Res. 18, 509–523. doi: 10.1093/ntr/ntv245
Hong, D. P., Fink, A. L., and Uversky, V. N. (2009). Smoking and Parkinson’s disease: does nicotine affect alpha-synuclein fibrillation? Biochim. Biophys. Acta 1794, 282–290. doi: 10.1016/j.bbapap.2008.09.026
Howes, S., Hartmann-Boyce, J., Livingstone-Banks, J., Hong, B., and Lindson, N. (2020). Antidepressants for smoking cessation. Cochrane Database Syst. Rev. 4:CD000031. doi: 10.1002/14651858.CD000031.pub5
Huang, L. Z., Parameswaran, N., Bordia, T., Michael, McIntosh, J., and Quik, M. (2009). Nicotine is neuroprotective when administered before but not after nigrostriatal damage in rats and monkeys. J. Neurochem. 109, 826–837. doi: 10.1111/j.1471-4159.2009.06011.x
Hukkanen, J., Jacob, P. III, and Benowitz, N. L. (2005). Metabolism and disposition kinetics of nicotine. Pharmacol. Rev. 57, 79–115. doi: 10.1124/pr.57.1.3
Huong, V. T., Shimanouchi, T., Shimauchi, N., Yagi, H., Umakoshi, H., Goto, Y., et al. (2010). Catechol derivatives inhibit the fibril formation of amyloid-beta peptides. J. Biosci. Bioeng. 109, 629–634. doi: 10.1016/j.jbiosc.2009.11.010
Iarkov, A., Mendoza, C., and Echeverria, V. (2021). Cholinergic Receptor Modulation as a Target for Preventing Dementia in Parkinson’s Disease. Front. Neurosci. 15:665820. doi: 10.3389/fnins.2021.665820
Irie, Y., Itokazu, N., Anjiki, N., Ishige, A., Watanabe, K., and Keung, W. M. (2004). Eugenol exhibits antidepressant-like activity in mice and induces expression of metallothionein-III in the hippocampus. Brain Res. 1011, 243–246. doi: 10.1016/j.brainres.2004.03.040
Janes, A. C., Zegel, M., Ohashi, K., Betts, J., Molokotos, E., Olson, D., et al. (2018). Nicotine normalizes cortico-striatal connectivity in non-smoking individuals with major depressive disorder. Neuropsychopharmacol 43, 2445–2451. doi: 10.1038/s41386-018-0069-x
Kardani, J., Sethi, R., and Roy, I. (2017). Nicotine slows down oligomerisation of alpha-synuclein and ameliorates cytotoxicity in a yeast model of Parkinson’s disease. Biochim. Biophys. Acta Mol. Basis Dis. 1863, 1454–1463. doi: 10.1016/j.bbadis.2017.02.002
Khan, H., Ullah, H., Aschner, M., Cheang, W. S., and Akkol, E. K. (2019). Neuroprotective Effects of Quercetin in Alzheimer’s Disease. Biomolecules 10:59. doi: 10.3390/biom10010059
Kumari, V., Gray, J. A., ffytche, D. H., Mitterschiffthaler, M. T., Das, M., and Zachariah, E. (2003). Cognitive effects of nicotine in humans: an fMRI study. Neuroimage 19, 1002–1013. doi: 10.1016/s1053-8119(03)00110-1
Launay, J. M., Del Pino, M., Chironi, G., Callebert, J., Peoc’h, K., Megnien, J. L., et al. (2009). Smoking Induces Long-Lasting Effects through a Monoamine-Oxidase Epigenetic Regulation. PLoS One 4:e7959. doi: 10.1371/journal.pone.0007959
Laviolette, S. R., and van der Kooy, D. (2004). The neurobiology of nicotine addiction: bridging the gap from molecules to behaviour. Nat. Rev. Neurosci. 5, 55–65. doi: 10.1038/nrn1298
Leonard, S., Adler, L. E., Benhammou, K., Berger, R., Breese, C. R., and Drebing, C. (2001). Smoking and mental illness. Pharmacol. Biochem. Behav. 70, 561–570. doi: 10.1016/s0091-3057(01)00677-3
Lewis, A., Miller, J. H., and Lea, R. A. (2007). Monoamine oxidase and tobacco dependence. Neurotoxicol 28, 182–195. doi: 10.1016/j.neuro.2006.05.019
Li, S., and Pu, X. P. (2011). Neuroprotective effect of kaempferol against a 1-methyl-4-phenyl-1,2,3,6-tetrahydropyridine-induced mouse model of Parkinson’s disease. Biol. Pharm Bull. 34, 1291–1296. doi: 10.1248/bpb.34.1291
Liu, L., Liu, Y., Zhao, J., Xing, X., Zhang, C., and Meng, H. (2020). Neuroprotective Effects of D-(-)-Quinic Acid on Aluminum Chloride-Induced Dementia in Rats. Evid. Based Complement Alternat. Med. 2020:5602597. doi: 10.1155/2020/5602597
Liu, S. J., Yang, C., Zhang, Y., Su, R. Y., Chen, J. L., Jiao, M. M., et al. (2016). Neuroprotective effect of beta-asarone against Alzheimer’s disease: regulation of synaptic plasticity by increased expression of SYP and GluR1. Drug Des. Devel. Ther. 10, 1461–1469. doi: 10.2147/DDDT.S93559
Lopez-Arrieta, J. M., Rodriguez, J. L., and Sanz, F. (2001). Efficacy and safety of nicotine on Alzheimer’s disease patients. Cochrane Database Syst. Rev. 2001:CD001749. doi: 10.1002/14651858.CD001749
Luo, L., Sun, T., Yang, L., Liu, A., Liu, Q. Q., Tian, Q. Q., et al. (2020). Scopoletin ameliorates anxiety-like behaviors in complete Freund’s adjuvant-induced mouse model. Mol. Brain 13:15. doi: 10.1186/s13041-020-0560-2
Majdi, A., Sadigh-Eteghad, S., and Gjedde, A. (2021). Effects of transdermal nicotine delivery on cognitive outcomes: A meta-analysis. Acta Neurol. Scand. 144, 179–191. doi: 10.1111/ane.13436
Malin, D. H., Moon, W. D., Goyarzu, P., Barclay, E., Magallanes, N., and Vela, A. J. (2013). Inhibition of monoamine oxidase isoforms modulates nicotine withdrawal syndrome in the rat. Life Sci. 93, 448–453. doi: 10.1016/j.lfs.2013.08.006
Marusich, J. A., Darna, M., Wilson, A. G., Denehy, E. D., Ebben, A., and Deaciuc, A. G. (2017). Tobacco’s minor alkaloids: Effects on place conditioning and nucleus accumbens dopamine release in adult and adolescent rats. Eur. J. Pharmacol. 814, 196–206. doi: 10.1016/j.ejphar.2017.08.029
Md, S., Gan, S. Y., Haw, Y. H., Ho, C. L., Wong, S., and Choudhury, H. (2018). In vitro neuroprotective effects of naringenin nanoemulsion against beta-amyloid toxicity through the regulation of amyloidogenesis and tau phosphorylation. Int. J. Biol. Macromol. 118, 1211–1219. doi: 10.1016/j.ijbiomac.2018.06.190
Mendez-Alvarez, E., Soto-Otero, R., Sanchez-Sellero, I., and Lopez-Rivadulla Lamas, M. (1997). Inhibition of brain monoamine oxidase by adducts of 1,2,3,4-tetrahydroisoquinoline with components of cigarette smoke. Life Sci. 60, 1719–1727. doi: 10.1016/s0024-3205(97)00114-8
Mihailescu, S., and Drucker-Colin, R. (2000). Nicotine, brain nicotinic receptors, and neuropsychiatric disorders. Arch. Med. Res. 31, 131–144. doi: 10.1016/s0188-4409(99)00087-9
Molas, S., DeGroot, S. R., Zhao-Shea, R., and Tapper, A. R. (2017). Anxiety and Nicotine Dependence: Emerging Role of the Habenulo-Interpeduncular Axis. Trends Pharmacol. Sci. 38, 169–180. doi: 10.1016/j.tips.2016.11.001
Moolchan, E. T., Aung, A. T., and Henningfield, J. E. (2003). Treatment of adolescent tobacco smokers: issues and opportunities for exposure reduction approaches. Drug Alcohol Depend 70, 223–232. doi: 10.1016/s0376-8716(03)00012-7
Moylan, S., Jacka, F. N., Pasco, J. A., and Berk, M. (2013). How cigarette smoking may increase the risk of anxiety symptoms and anxiety disorders: a critical review of biological pathways. Brain Behav. 3, 302–326. doi: 10.1002/brb3.137
Naoi, M., Maruyama, W., and Nagy, G. M. (2004). Dopamine-derived salsolinol derivatives as endogenous monoamine oxidase inhibitors: occurrence, metabolism and function in human brains. Neurotoxicol 25, 193–204. doi: 10.1016/S0161-813X(03)00099-8
Newhouse, P., Singh, A., and Potter, A. (2004a). Nicotine and nicotinic receptor involvement in neuropsychiatric disorders. Curr. Top. Med. Chem. 4, 267–282. doi: 10.2174/1568026043451401
Newhouse, P. A., Potter, A., and Singh, A. (2004b). Effects of nicotinic stimulation on cognitive performance. Curr. Opin. Pharmacol. 4, 36–46. doi: 10.1016/j.coph.2003.11.001
Nop, O., Senft Miller, A., Culver, H., Makarewicz, J., and Dumas, J. A. (2021). Nicotine and Cognition in Cognitively Normal Older Adults. Front. Aging Neurosci. 13:640674. doi: 10.3389/fnagi.2021.640674
Parashar, A., Mehta, V., and Udayabanu, M. (2017). Rutin alleviates chronic unpredictable stress-induced behavioral alterations and hippocampal damage in mice. Neurosci. Lett. 656, 65–71. doi: 10.1016/j.neulet.2017.04.058
Patsenka, A., and Antkiewicz-Michaluk, L. (2004). Inhibition of rodent brain monoamine oxidase and tyrosine hydroxylase by endogenous compounds - 1,2,3,4-tetrahydro-isoquinoline alkaloids. Pol. J. Pharmacol. 56, 727–734.
Paula, P. C., Angelica Maria, S. G., Luis, C. H., and Gloria Patricia, C. G. (2019). Preventive Effect of Quercetin in a Triple Transgenic Alzheimer’s Disease Mice Model. Molecules 24:2287. doi: 10.3390/molecules24122287
Pomerleau, O. F., Turk, D. C., and Fertig, J. B. (1984). The effects of cigarette smoking on pain and anxiety. Addict Behav. 9, 265–271. doi: 10.1016/0306-4603(84)90018-2
Postma, P., Gray, J. A., Sharma, T., Geyer, M., Mehrotra, R., Das, M., et al. (2006). A behavioural and functional neuroimaging investigation into the effects of nicotine on sensorimotor gating in healthy subjects and persons with schizophrenia. Psychopharmacol 184, 589–599. doi: 10.1007/s00213-006-0307-5
Quertemont, E., and Tambour, S. (2004). Is ethanol a pro-drug? The role of acetaldehyde in the central effects of ethanol. Trends Pharmacol. Sci. 25, 130–134. doi: 10.1016/j.tips.2004.01.00
Rajdev, K., Siddiqui, E. M., Jadaun, K. S., and Mehan, S. (2020). Neuroprotective potential of solanesol in a combined model of intracerebral and intraventricular hemorrhage in rats. IBRO Rep. 8, 101–114. doi: 10.1016/j.ibror.2020.03.001
Riveles, K., Huang, L. Z., and Quik, M. (2008). Cigarette smoke, nicotine and cotinine protect against 6-hydroxydopamine-induced toxicity in SH-SY5Y cells. Neurotoxicol 29, 421–427. doi: 10.1016/j.neuro.2008.02.001
Rodgman, A., and Perfetti, T. A. (2013). The Chemical Components of Tobacco and Tobacco Smoke, 2nd Edn. Boca Raton: CRC Press. doi: 10.1201/b13973
Rommelspacher, H., Meier-Henco, M., Smolka, M., and Kloft, C. (2002). The levels of norharman are high enough after smoking to affect monoamineoxidase B in platelets. Eur. J. Pharmacol. 441, 115–125. doi: 10.1016/s0014-2999(02)01452-8
Rose, J. E. (2006). Nicotine and nonnicotine factors in cigarette addiction. Psychopharmacol 184, 274–285. doi: 10.1007/s00213-005-0250-x
Sacco, K. A., Bannon, K. L., and George, T. P. (2004). Nicotinic receptor mechanisms and cognition in normal states and neuropsychiatric disorders. J. Psychopharmacol. 18, 457–474. doi: 10.1177/026988110401800403
Sachdeva, A. K., Kuhad, A., and Chopra, K. (2014). Naringin ameliorates memory deficits in experimental paradigm of Alzheimer’s disease by attenuating mitochondrial dysfunction. Pharmacol. Biochem. Behav. 127, 101–110. doi: 10.1016/j.pbb.2014.11.002
Sari, Y., and Khalil, A. (2015). Monoamine Oxidase Inhibitors Extracted from Tobacco Smoke as Neuroprotective Factors for Potential Treatment of Parkinson’s Disease. CNS Neurol. Disord. Drug Targets 14, 777–785. doi: 10.2174/1871527314666150325235608
Scott, J. G., Matuschka, L., Niemela, S., Miettunen, J., Emmerson, B., and Mustonen, A. (2018). Evidence of a Causal Relationship Between Smoking Tobacco and Schizophrenia Spectrum Disorders. Front. Psychiatr. 9:607. doi: 10.3389/fpsyt.2018.00607
Seeman, J. I., Dixon, M., and Haussmann, H.-J. (2002). Acetaldehyde in mainstream tobacco smoke: formation and occurrence in smoke and bioavailability in the smoker. Chem. Res. Toxicol. 15, 1331–1350. doi: 10.1021/tx020069f
Sharama, S. (2016). Monoamine Oxidase Inhibitors : clinical Pharmacology, Benefits, and Potential Health Risks. New York: Nova Science Publishers Inc.
Shin, M., Liu, Q. F., Choi, B., Shin, C., Lee, B., Yuan, C., et al. (2020). Neuroprotective Effects of Limonene (+) against Abeta42-Induced Neurotoxicity in a Drosophila Model of Alzheimer’s Disease. Biol. Pharm. Bull. 43, 409–417. doi: 10.1248/bpb.b19-00495
Singh, S. S., Rai, S. N., Birla, H., Zahra, W., Rathore, A. S., Dilnashin, H., et al. (2020). Neuroprotective Effect of Chlorogenic Acid on Mitochondrial Dysfunction-Mediated Apoptotic Death of DA Neurons in a Parkinsonian Mouse Model. Oxid Med. Cell Longev. 2020:6571484. doi: 10.1155/2020/6571484
Smith, D. M., Fisher, D., Blier, P., Ilivitsky, V., and Knott, V. (2016a). The separate and combined effects of monoamine oxidase A inhibition and nicotine on resting state EEG. J. Psychopharmacol. 30, 56–62. doi: 10.1177/0269881115613518
Smith, T. T., Rupprecht, L. E., Cwalina, S. N., Onimus, M. J., Murphy, S. E., Donny, E. C., et al. (2016b). Effects of Monoamine Oxidase Inhibition on the Reinforcing Properties of Low-Dose Nicotine. Neuropsychopharmacol 41, 2335–2343. doi: 10.1038/npp.2016.36
Smith, K. L., Ford, G. K., Jessop, D. S., and Finn, D. P. (2013). Behavioural, neurochemical and neuroendocrine effects of the endogenous beta-carboline harmane in fear-conditioned rats. J. Psychopharmacol 27, 162–170. doi: 10.1177/0269881112460108
Smith, T. T., Schaff, M. B., Rupprecht, L. E., Schassburger, R. L., Buffalari, D. M., Murphy, S. E., et al. (2015). Effects of MAO inhibition and a combination of minor alkaloids, beta-carbolines, and acetaldehyde on nicotine self-administration in adult male rats. Drug Alcohol Depend 155, 243–252. doi: 10.1016/j.drugalcdep.2015.07.002
Sulakhiya, K., Keshavlal, G. P., Bezbaruah, B. B., Dwivedi, S., Gurjar, S. S., Munde, N., et al. (2016). Lipopolysaccharide induced anxiety- and depressive-like behaviour in mice are prevented by chronic pre-treatment of esculetin. Neurosci. Lett. 611, 106–111. doi: 10.1016/j.neulet.2015.11.031
Syrjanen, K., Eronen, K., Hendolin, P., Paloheimo, L., Eklund, C., Backstrom, A., et al. (2017). Slow-release L-Cysteine (Acetium(R)) Lozenge Is an Effective New Method in Smoking Cessation. A Randomized, Double-blind, Placebo-controlled Intervention. Anticancer Res. 37, 3639–3648. doi: 10.21873/anticanres.11734
Takeda, H., Tsuji, M., Inazu, M., Egashira, T., and Matsumiya, T. (2002). Rosmarinic acid and caffeic acid produce antidepressive-like effect in the forced swimming test in mice. Eur. J. Pharmacol. 449, 261–267. doi: 10.1016/s0014-2999(02)02037-x
Talhout, R., Opperhuizen, A., and van Amsterdam, J. G. (2007). Role of acetaldehyde in tobacco smoke addiction. Eur. Neuropsychopharmacol. 17, 627–636. doi: 10.1016/j.euroneuro.2007.02.013
Tan, X., Ingraham, C. M., McBride, W. J., and Ding, Z. M. (2022). The involvement of mesolimbic dopamine system in cotinine self-administration in rats. Behav. Brain Res. 417:113596. doi: 10.1016/j.bbr.2021.113596
Tan, X., Vrana, K., and Ding, Z. M. (2021). Cotinine: Pharmacologically Active Metabolite of Nicotine and Neural Mechanisms for Its Actions. Front. Behav. Neurosci. 15:758252. doi: 10.3389/fnbeh.2021.758252
Terry, A. V. Jr., Hernandez, C. M., Hohnadel, E. J., Bouchard, K. P., and Buccafusco, J. J. (2005). Cotinine. A Neuroactive Metabolite of Nicotine: Potential for Treating Disorders of Impaired Cognition. CNS Drug Rev. 11, 229–252. doi: 10.1111/j.1527-3458.2005.tb00045.x
Thiriez, C., Villafane, G., Grapin, F., Fenelon, G., Remy, P., and Cesaro, P. (2011). Can nicotine be used medicinally in Parkinson’s disease? Expert. Rev. Clin. Pharmacol. 4, 429–436. doi: 10.1586/ecp.11.27
Truman, P., Grounds, P., and Brennan, K. A. (2017). Monoamine oxidase inhibitory activity in tobacco particulate matter: Are harman and norharman the only physiologically relevant inhibitors? Neurotoxicol 59, 22–26. doi: 10.1016/j.neuro.2016.12.010
U.S. Department of Health and Human Services. (2004). The Health Consequences of Smoking: A Report of the Surgeon General. Atlanta, GA: U.S. Department of Health and Human Services
Veljkovic, E., Xia, W., Phillips, B., Wong, E. T., Ho, J., Oviedo, A., et al. (2018). “Parkinson’s Disease,” in Nicotine and Other Tobacco Compounds in Neurodegenerative and Psychiatric Diseases. Part 1: Overview of Epidemiological Data on Smoking and Preclinical and Clinical Data on Nicotine, Chap. 1, eds E. Veljkovic, W. Xia, B. Phillips, E. T. Wong, J. Ho, A. Oviedo, et al. (Cambridge: Academic Press). doi: 10.1016/B978-0-12-812922-7.00001-9
Venkatakrishnan, P., Gairola, C. G., Castagnoli, N. Jr., and Miller, R. T. (2009). Naphthoquinones and bioactive compounds from tobacco as modulators of neuronal nitric oxide synthase activity. Phytother. Res. 23, 1663–1672. doi: 10.1002/ptr.2789
Villafane, G., Thiriez, C., Audureau, E., Straczek, C., Kerschen, P., Cormier-Dequaire, F., et al. (2018). High-dose transdermal nicotine in Parkinson’s disease patients: a randomized, open-label, blinded-endpoint evaluation phase 2 study. Eur. J. Neurol. 25, 120–127. doi: 10.1111/ene.13474
Villegier, A. S., Belluzzi, J. D., and Leslie, F. M. (2011). Serotonergic mechanism underlying tranylcypromine enhancement of nicotine self-administration. Synapse 65, 479–489. doi: 10.1002/syn.20864
Villegier, A. S., Salomon, L., Granon, S., Changeux, J. P., Belluzzi, J. D., Leslie, F. M., et al. (2006). Monoamine oxidase inhibitors allow locomotor and rewarding responses to nicotine. Neuropsychopharmacol 31, 1704–1713. doi: 10.1038/sj.npp.1300987
Wang, N. Y., Li, J. N., Liu, W. L., Huang, Q., Li, W. X., Tan, Y. H., et al. (2021). Ferulic Acid Ameliorates Alzheimer’s Disease-like Pathology and Repairs Cognitive Decline by Preventing Capillary Hypofunction in APP/PS1 Mice. Neurotherapeutics 18, 1064–1080. doi: 10.1007/s13311-021-01024-7
Waterhouse, U., Brennan, K. A., and Ellenbroek, B. A. (2018). Nicotine self-administration reverses cognitive deficits in a rat model for schizophrenia. Addict Biol. 23, 620–630. doi: 10.1111/adb.12517
West, R. (2017). Tobacco smoking: Health impact, prevalence, correlates and interventions. Psychol. Health 32, 1018–1036. doi: 10.1080/08870446.2017.1325890
West, R., and Cox, S. (2021). The 1988 US Surgeon General’s report Nicotine Addiction: how well has it stood up to three more decades of research? Addiction [Epub Online ahead of print]. doi: 10.1111/add.15754
Wu, J., and Lukas, R. J. (2011). Naturally-expressed nicotinic acetylcholine receptor subtypes. Biochem. Pharmacol. 82, 800–807. doi: 10.1016/j.bcp.2011.07.067
Yang, W., Ma, J., Liu, Z., Lu, Y., Hu, B., and Yu, H. (2014). Effect of naringenin on brain insulin signaling and cognitive functions in ICV-STZ induced dementia model of rats. Neurol. Sci. 35, 741–751. doi: 10.1007/s10072-013-1594-3
Yi, L. T., Li, J., Li, H. C., Su, D. X., Quan, X. B., He, X. C., et al. (2012). Antidepressant-like behavioral, neurochemical and neuroendocrine effects of naringenin in the mouse repeated tail suspension test. Prog. Neuropsychopharmacol. Biol. Psychiatr. 39, 175–181.
Yu, P. H., and Boulton, A. A. (1987). Irreversible inhibition of monoamine oxidase by some components of cigarette smoke. Life Sci. 41, 675–682. doi: 10.1016/0024-3205(87)90446-2
Yusha’u, Y., Muhammad, U. A., Nze, M., Egwuma, J. M., Igomu, O. J., and Abdulkadir, M. (2017). Modulatory Role of Rutin Supplement on Open Space Forced Swim Test Murine Model of Depression. Niger J. Physiol. Sci. 32, 201–205.
Keywords: tobacco dependence, nicotine, tobacco smoke components, monoamine oxidase inhibition, mental health, Parkinson’s Disease
Citation: Hong SW, Teesdale-Spittle P, Page R, Ellenbroek B and Truman P (2022) Biologically Active Compounds Present in Tobacco Smoke: Potential Interactions Between Smoking and Mental Health. Front. Neurosci. 16:885489. doi: 10.3389/fnins.2022.885489
Received: 28 February 2022; Accepted: 28 March 2022;
Published: 26 April 2022.
Edited by:
Jacques Joubert, University of the Western Cape, South AfricaReviewed by:
Selena Bartlett, The University of Queensland, AustraliaCopyright © 2022 Hong, Teesdale-Spittle, Page, Ellenbroek and Truman. This is an open-access article distributed under the terms of the Creative Commons Attribution License (CC BY). The use, distribution or reproduction in other forums is permitted, provided the original author(s) and the copyright owner(s) are credited and that the original publication in this journal is cited, in accordance with accepted academic practice. No use, distribution or reproduction is permitted which does not comply with these terms.
*Correspondence: Penelope Truman, cC50cnVtYW5AbWFzc2V5LmFjLm56