- 1State Key Lab of Animal Nutrition, College of Animal Science and Technology, China Agricultural University, Beijing, China
- 2College of Animal Science, Fujian Agriculture and Forestry University, Fuzhou, China
- 3Department of Nutrition and Health, China Agricultural University, Beijing, China
The enteric nervous system (ENS) is important for the intestinal barrier to defend and regulate inflammation in the intestine. The aim of this study was to investigate the effect of pyrroloquinoline quinone (PQQ) on regulating neuropeptide secretion by ENS neurons of rats challenged with lipopolysaccharide (LPS) to create enteritis. Thirty Sprague Dawley rats were divided into five groups, namely, basal (CTRL), basal plus LPS challenge (LPS), basal with 2.5 mg/kg b.w./day of PQQ plus challenge with LPS (PQQ 2.5), basal with 5.0 mg/kg b.w./day PQQ plus challenge with LPS (PQQ 5), and basal with 10.0 mg/kg b.w./day PQQ plus challenge with LPS (PQQ 10). After treatment with basal diet or PQQ for 14 days, rats were challenged with LPS except for the CTRL group. Rats were euthanized 6 h after the LPS challenge. Rats showed an increased average daily gain in PQQ treatment groups (P < 0.05). Compared with the LPS group, PQQ 5 and PQQ 10 rats showed increased villus height and villus height/crypt depth of jejunum (P < 0.05). In PQQ treatment groups, concentrations of IL-1β and TNF-α in serum and intestine of rats were decreased, and IL-10 concentration was increased in serum compared with the LPS group (P < 0.05). Compared with the LPS group, the concentration of neuropeptide Y (NPY), nerve growth factor (NGF), vasoactive intestinal peptide (VIP), substance P (SP), calcitonin gene-related peptide (CGRP), and brain-derived neurotropic factor (BDNF) in serum were decreased in PQQ treatment groups (P < 0.05). Compared with the LPS group, ileal mRNA levels of BDNF, NPY, and NGF were decreased in PQQ treatment groups (P < 0.05). Jejunal concentrations of SP, CGRP, VIP, BDNF, NPY, and NGF were decreased in PQQ treatment groups compared with the LPS group (P < 0.05). Compared with the LPS group, phosphor-protein kinase B (p-Akt)/Akt levels in jejunum and colon were decreased in PQQ treatment groups (P < 0.05). In conclusion, daily treatment with PQQ improved daily gain, jejunal morphology, immune responses. PQQ-regulated enteric neurochemical plasticity of ENS via the Akt signaling pathway of weaned rats suffering from enteritis.
Introduction
The intestine is the body organ with the largest membrane surface area that serves to absorb nutrients and sense, and recognize and defend against pathogens, antigens, toxins, and other detrimental secretions. The intestine accomplishes these diverse functions through coordinated effects of the enteroendocrine system, the enteric nervous system (ENS), the gut immune system, and the non-immune defense system of the intestine (Ferraris et al., 1989; De Giorgio, 2006). Intestinal nerve cells and glial cells in the ENS secrete neuropeptides and immune factors to pass signals between the ENS and the central nervous system (CNS). These signals influence mucosal secretions and gastrointestinal peristalsis and affect rhythms and hormone secretion in the CNS (Rao and Gershon, 2018; Boesmans et al., 2019). The ENS plays an important role in intestinal diseases, such as colitis, irritable bowel syndrome, and inflammatory bowel disease (Lasrado et al., 2017; Resnikoff et al., 2019; Jarret et al., 2020). The ENS, which includes submucosal nerve plexus, myenteric nerve plexus, and glial cells, usually is functional and structurally mature during the early life of mammals (Montedonico et al., 2006; Parathan et al., 2020). The capacity to regulate neuropeptides is called neurochemical plasticity. Enteric neuroplasticity is an adaption to intestinal contents and the intestinal microenvironment and is pronounced during early development in mammals (Moeser et al., 2017).
The phosphatidylinositol-3 kinase (PI3K)/protein kinase B (Akt) signaling pathway regulates the activity ofglycogen synthase kinase-3β (GSK-3β), Tau protein, and N-methyl-D-aspartame receptor (NMDA), which promotes the development of nerve cells and regeneration of synapses (Iqbal et al., 2016; Majewska and Szeliga, 2017; Falcon et al., 2019). The PI3K/Akt signaling pathway also plays an important regulatory role in intestinal neurological diseases by reducing the oxidative stress and apoptosis of nerve cells, improving the secretion of neurotropic factors, regulating survival and differentiation of nerve cells, and promoting the proliferation of intestinal nerve cells and glial cells (Ichihara et al., 2004; Du et al., 2009; Becker et al., 2013).
Pyrroloquinoline quinone (PQQ), a water-soluble quinone compound, was discovered as a redox cofactor of methanol dehydrogenase in pseudomonas TP1 and other Gram-negative bacteria (Duine et al., 1979; Duine, 1991). We demonstrated previously that dietary supplementation with PQQ could regulate intestinal morphology, mucosal barrier function, colonic microbiota, and antioxidant status to reduce diarrhea and improve the growth performance of weaned pigs (Yin et al., 2019; Huang et al., 2020, 2021; Ming et al., 2021). PQQ is a potent neuroprotective nutrient in the CNS and peripheral nerves, which can mitigate sciatic nerve injury, reduce neurotoxin-induced neurotoxicity, and promote neuronal cell regeneration (Liu et al., 2005; Hara et al., 2007; Shanan et al., 2019). PQQ treatment can ameliorate signs of memory impairment in aging mice and schizophrenic rats via reducing the expression of phosphorylation Akt and maintaining the GSK-3β level in the hippocampus (Zhou X. Q. et al., 2018; Zhou et al., 2020).
We hypothesized that PQQ treatment could influence the ENS and regulate enteric neuroplasticity to improve intestinal health. We tested this hypothesis in the enteritis rat model via developed challenging rats with lipopolysaccharide (LPS). We detected neuropeptides in serum and intestine and Akt signaling pathway expression in the intestine to determine if PQQ could regulate enteric neuroplasticity via the Akt signaling pathway.
Materials and Methods
Animals and Experimental Treatment
All experimental protocols in this study were approved by the Animal Subjects Committee of China Agricultural University (Beijing, China) and carried out based on the National Research Council’s Guide for the Care and Use of Laboratory Animals (AW01211202-1-2). Sprague Dawley male rats (n = 30, 21 days old) were purchased from SPF (Beijing) Biotechnology Co. Ltd., and were housed in a pathogen-free animal room with a 12/12 h light-dark cycle at 23°C. After 3 days of acclimatization, rats were divided randomly into 5 groups (n = 6 per treatment group), namely, (1) basal unchallenged (CTRL), (2) LPS challenged (LPS), (3) 2.5 mg/kg b.w./day low dose of PQQ and challenged with LPS (PQQ 2.5), (4) 5.0 mg/kg b.w./day medium dose of PQQ and challenged with LPS (PQQ 5), and (5) 10.0 mg/kg b.w./day high dose of PQQ and challenged with LPS (PQQ 10) (Lu et al., 2015). PQQ⋅Na2 (purity, ≥98%; Changmao Biochemical Engineering Co. Ltd., Changzhou, China) dissolved in physiological saline was administrated intragastrically for 14 days. All rats were weighed every day and had free access to water and food. After the supplementation period, rats were injected with LPS [4 mg/kg b.w., isolated from Escherichia coli (serotype 055: B5) (Zani et al., 2008), purchased from Sigma, United States] except rats in the CTRL group on day 15. All rats were euthanized by intraperitoneal injection with pentobarbitone sodium (50 mg/kg b.w.) (Mohamed et al., 2020) at 6 h after the LPS dose.
Sample Collection
After euthanasia, serum samples were collected from the abdominal veins of rats and separated into serum and stored at −20°C for further analysis. Jejunal and colonic tissues (1 cm2) were excised and stored in 4% paraformaldehyde solution (40% formaldehyde solution dissolved in phosphate-buffered saline (PBS)) for analysis of intestinal morphology. Notably, 2 cm segments from the middle of the jejunum, ileum, and colon were collected in freezing tubes which were frozen rapidly in liquid nitrogen and stored at −80°C for further analysis.
Jejunal Morphology
After fixation with 4% paraformaldehyde solution for 24 h, tissue samples were dehydrated and embedded in paraffin. Jejunal sections (5 μm) were stained with hematoxylin and eosin. Villus height (VH), crypt depth (CD), and villus height/crypt depth ratio (VCR) were viewed and evaluated using a microscope (Eclipse CI, Nikon) and imaging software (DS-U3, Nikon). Data were collected from at least 10 well-oriented villi and crypts from 5 slides per sample.
Cytokines of Serum and Intestinal Segments
Cytokines, including interleukin (IL)-1β, IL-6, IL-10, and tumor necrosis factor (TNF)-α, were determined in serum, jejunum, ileum, and colon using enzyme-linked immunoassay (ELISA) kits (Beijing Kang Iia Hong Yuan Biological Technology Co., Ltd., Beijing, China) according to the manufacturer’s instructions and quantified using a Multiskan Microplate Reader (Thermo Fisher Scientific, United States). Absorbance for kits was all set at 450 nm, and the minimal detections were 31.25 pg/ml for IL-1β and IL-6, 15.63 pg/ml for IL-10, and 9.38 pg/ml for TNF-α. The intra- and inter-assay coefficients of variation (CV) were <10% for each assay.
Quantitative Real-Time Polymerase Chain Reaction
Total RNA was extracted from the snap-frozen ileal tissue using RNAiso Plus (9109, TaKaRa Bio, Inc., Japan)/chloroform extraction. Complementary DNA (cDNA) was synthesized using the PrimeScript RT Reagent Kit with gDNA Eraser (RR047A, TaKaRa Bio, Inc., Japan). Quantitative real-time polymerase chain reaction PCR (RT-PCR) was conducted using the Roche Light Cycler® System (Roche, South San Francisco, CA, Canada). The primer sequences are shown in Supplementary Table 1. Target genes were detected by normalizing with β-actin and calculating using the 2–ΔΔCT method (Pfaffl et al., 2002).
Determination of the Concentration of Neuropeptides
Neuropeptides including neuropeptide Y (NPY), nerve growth factor (NGF), vasoactive intestinal peptide (VIP), substance P (SP), calcitonin gene-related peptide (CGRP), and brain-derived neurotropic factor (BDNF) were determined in serum using assay kits according to the manufacturer’s instructions (Supplementary Table 2). These same neuropeptides were determined in the jejunum, ileum, and colon.
Immunohistochemistry
Fixed jejunum and colon tissues were embedded in paraffin and cut into sections (5 μm) followed by deparaffinization and rehydration. Tissue sections were placed in ethylenediaminetetraacetic acid (EDTA) antigen retrieval buffer (pH 8.0) to retrieve the antigen. Endogenous peroxidase activity was blocked with 3% H2O2 under dark conditions at room temperature and was then washed with PBS (PBS, pH 7.4). Bovine serum albumin (BSA, 5%) was added to tissues. Primary antibodies (Supplementary Table 3) diluted in PBS were incubated with tissue sections overnight at 4°. Tissue sections were covered with secondary antibodies for 50 min at room temperature, and positive expression for protein gene product 9.5 (PGP9.5), SP, CGRP, BDNF, NPY, and NGF was dyed brown with diaminobenzidine (DAB). Hematoxylin was used as a counterstain for nuclei dyed blue. Ganglia immunoreactive for neuropeptides was calculated in percentage in the total area of neurons.
Western Blot Assay
Jejunal and colonic tissues were ground in liquid nitrogen and lysed using radioimmunoprecipitation assay (RIPA) buffer with protease and phosphatase inhibitors. After sonication and centrifugation, protein concentration in the supernatant was quantified using a bicinchoninic acid (BCA) protein assay kit (02912E, CWbiotech, Beijing, China). Supernatant with 30 μg proteins from each sample was separated in sodium dodecyl sulfate (SDS) polyacrylamide gels and then transferred to polyvinylidene fluoride (PVDF) membranes (0.45 μm, Millipore, United States). Membranes were blocked and incubated with the primary antibodies of phosphatidylinositol 3-kinase (PI3K, #4257, Cell Signaling Technology, Danvers, MA, United States), protein kinase B (Akt, #9272, Cell Signaling Technology), phosphor-Akt (p-Akt, #9271, Cell Signaling Technology), and β-actin (#4970, Cell Signaling Technology) overnight at 4°C. The membranes were incubated with secondary antibodies (111-035-003, Jackson, United States) for 1 h at room temperature and reacted with electrochemiluminescence (ECL, WBKLS0500, Millipore, United States). The intensity of protein bands was analyzed using the ImageJ software.
Statistical Analysis
All data were analyzed with a one-way analysis of variance (ANOVA) using SAS (version 9.2, United States). Differences among mean values were evaluated using the Duncan’s multiple range test. The individual rat was the experimental unit for traits of interest. Values are expressed as means and considered statistically different if P ≤ 0.05. Figures were created using GraphPad Prism 9.
Results
Average Daily Gain
Compared with CTRL and LPS groups, rats assigned to PQQ 5 and PQQ 10 groups expressed increased average daily gain (ADG) (P < 0.05, Figure 1). Rats assigned to the PQQ 5 group showed the greatest difference in ADG compared with both CTRL and LPS groups.
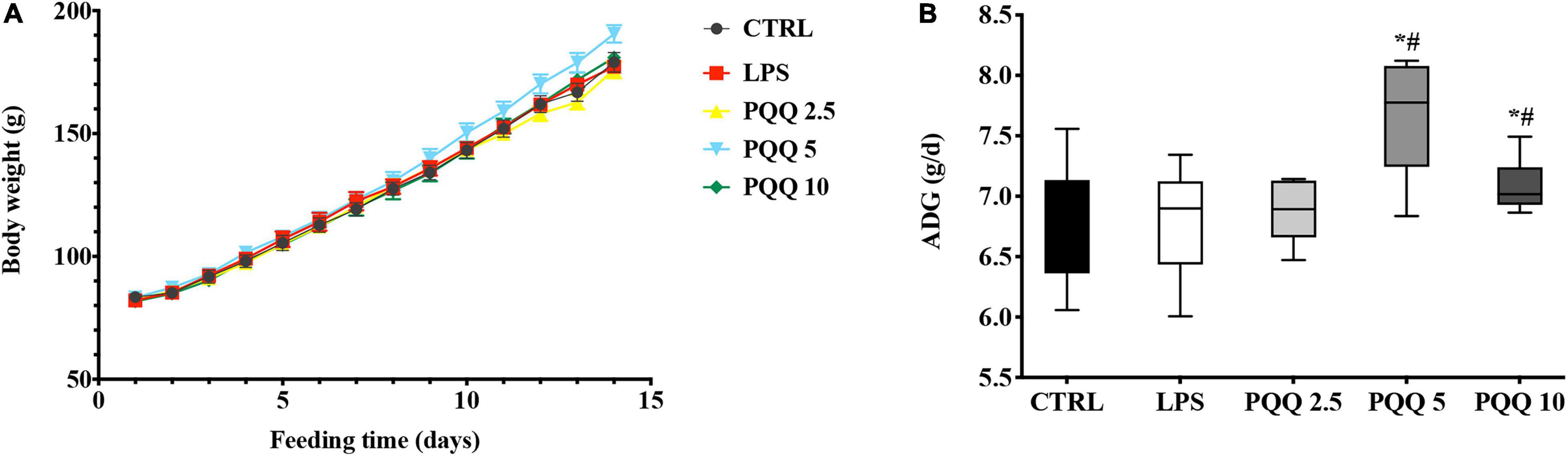
Figure 1. Average daily gain of weaned rats (n = 6). (A) Body weight of rats was recorded with feeding time. (B) Average daily gain(ADG) of rats was evaluated by body weight. CTRL, control treatment; lipopolysaccharide (LPS), control and LPS treatment; pyrroloquinoline quinone (PQQ) 2.5, intragastric administration with 2.5 mg/kg b.w./day PQQ⋅Na2 treatment; PQQ 5, intragastric administration with 5.0 mg/kg b.w./day PQQ⋅Na2treatment; PQQ 10, intragastric administration with 10.0 mg/kg b.w./day PQQ⋅Na2 treatment. *Means significant difference with the CTRL group (P ≤ 0.05); #means significant difference with the LPS group (P ≤ 0.05).
Jejunal Morphology
Rats challenged with LPS had non-distinct jejunal villus compared with the CTRL group (Figure 2). Rats in PQQ 2.5, PQQ 5, and PQQ 10 groups exhibited more complete and taller jejunal villus compared with the LPS group. Compared with the CTRL group, LPS treatment decreased jejunal VH and VCR in rats (P < 0.05, Table 1). Compared with the LPS group, PQQ 5 and PQQ 10 groups increased VH and VCR of the jejunum (P < 0.05). Neither LPS nor PQQ treatments affected CD.
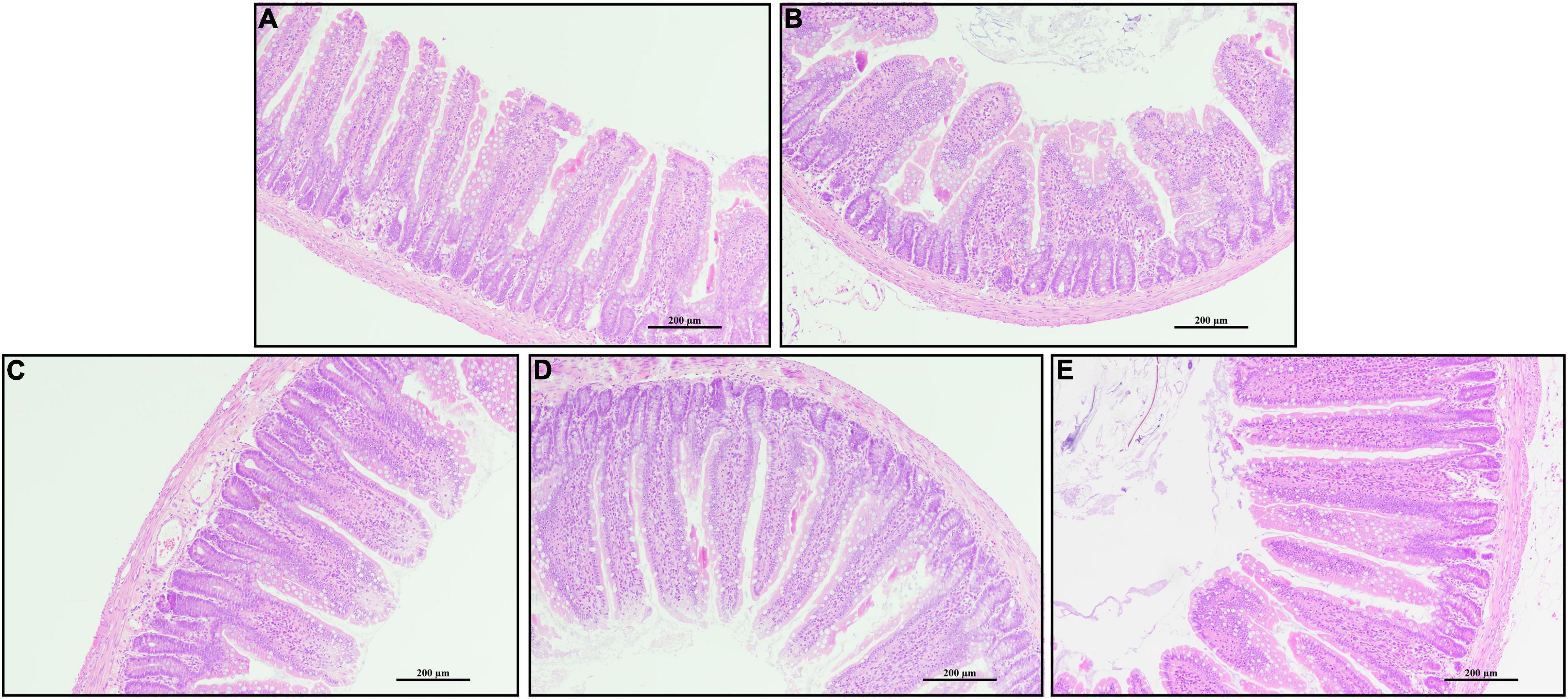
Figure 2. Jejunal morphology by H and E stains. (A) Control treatment; (B) control and LPStreatment; (C) intragastric administration with 2.5 mg/kg b.w./day PQQ⋅Na2 treatment; (D) intragastric administration with 5.0 mg/kg b.w./day PQQ⋅Na2 treatment; and (E) intragastric administration with 10.0 mg/kg b.w./day PQQ⋅Na2 treatment. Scale bars in pictures are 200 μm.
Cytokines in Serum and Intestine
Compared with the CTRL group, concentrations of IL-1β, IL-6, and TNF-α increased, and IL-10 decreased in the serum of rats challenged with LPS (P < 0.05, Figure 3). Feeding PQQ at 2.5, 5.0, and 10.0 mg/kg b.w. to rats decreased (P < 0.05) concentrations of IL-1β and TNF-α, and increased concentration of IL-10 in serum compared with the LPS group. With a similar pattern, concentrations of IL-1β and TNF-α in jejunum, ileum, and colon were increased (P < 0.05) in the LPS group compared with the CTRL group. Concentrations of IL-1β and TNF-α in jejunum, ileum, and colon of rats were decreased (P < 0.05)compared with rats assigned to the LPS group.
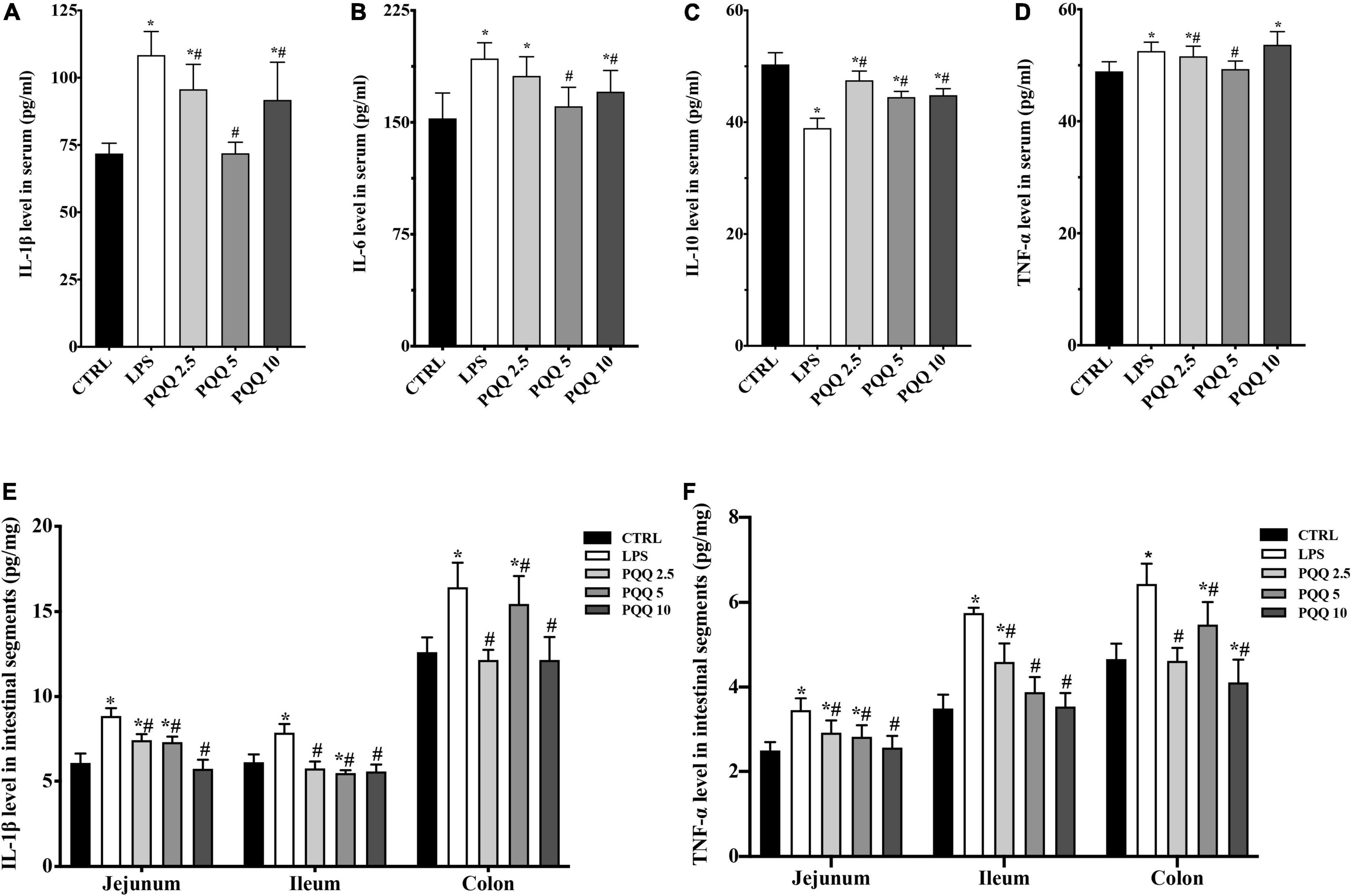
Figure 3. Cytokine level in serum and intestinal segments of weaned rats. (A) IL-1β level in serum (n = 6); (B) IL-6 level in serum (n = 6); (C) IL-10 level in serum (n = 5); (D) TNF-α level in serum (n = 6); (E) IL-1β level in jejunum, ileum and colon (n = 6); and (F) TNF-α level in jejunum, ileum and colon (n = 6). CTRL, control treatment; LPS, control and LPS treatment; PQQ 2.5, intragastric administration with 2.5 mg/kg b.w./day PQQ⋅Na2 treatment; PQQ 5, intragastric administration with 5.0 mg/kg b.w./day PQQ⋅Na2 treatment; PQQ 10, intragastric administration with 10.0 mg/kg b.w./day PQQ⋅Na2 treatment. *Means significant difference with the CTRL group (P ≤ 0.05); #means significant difference with the LPS group (P ≤ 0.05).
Concentration of Neuropeptides in Serum
Compared with the CTRL group, concentrations of NPY, NGF, VIP, SP, and CGRP were increased (P < 0.05, Figure 4) in the serum of rats in the LPS group. There were no significant differences in BDNF between LPS and CTRL groups. Concentrations of NPY, NGF, VIP, SP, CGRP, and BDNF were decreased (P < 0.05) with PQQ intake compared with the LPS group. Feeding PQQ at 5.0 mg/kg b.w. decreased concentrations of NPY, NGF, VIP, and CGRP more than 2.5 or 10.0 mg/kg b.w. when compared with the LPS group.
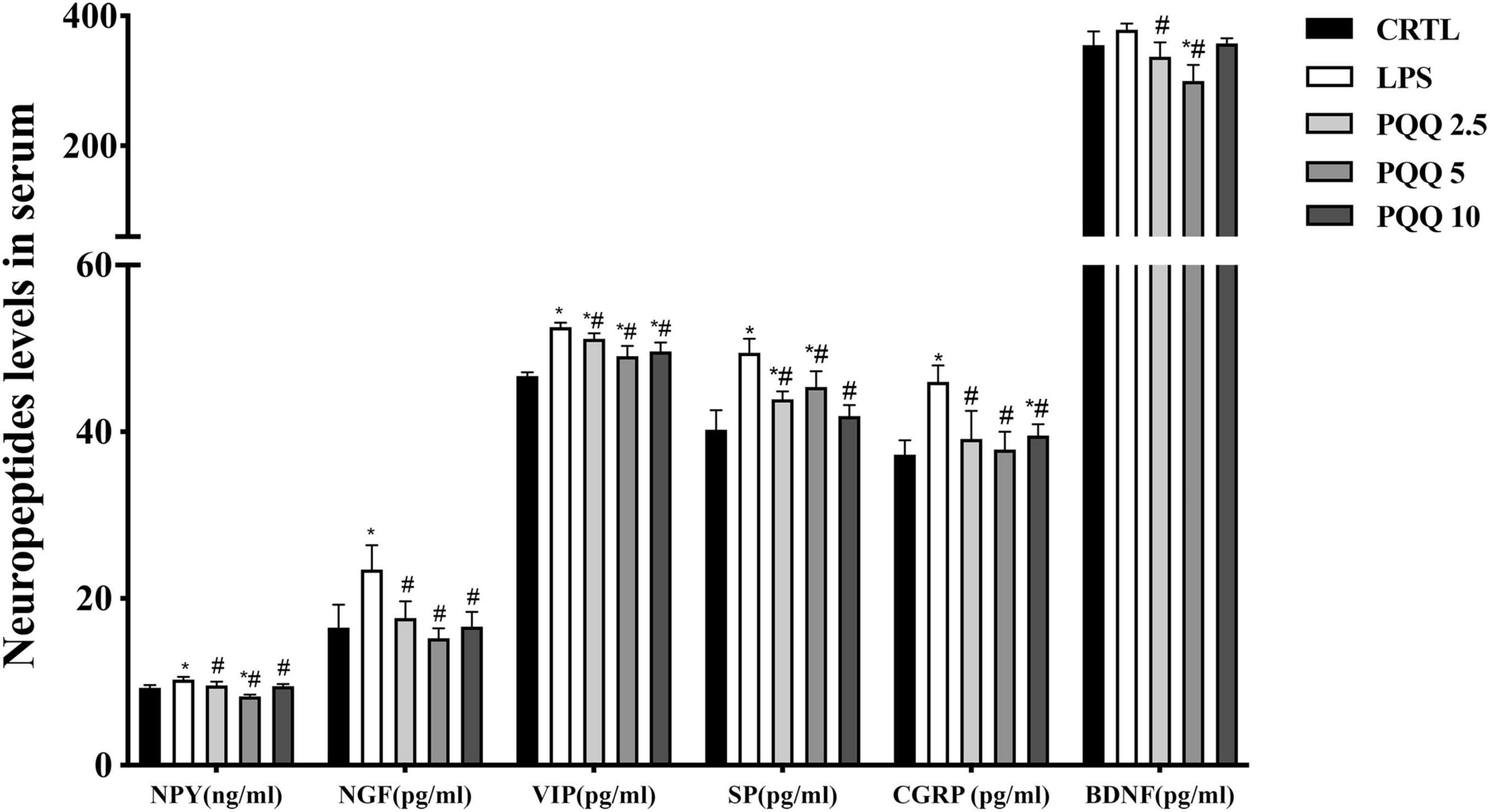
Figure 4. Ileal neuropeptide mRNA expression levels of weaned rats (n = 6). SP, substance P; CGRP, calcitonin gene-related peptide; BDNF, brain-derived neurotropic factor; NPY, neuropeptide Y; NGF, nerve growth factor. CTRL, control treatment; LPS, control and LPS treatment; PQQ 2.5, intragastric administration with 2.5 mg/kg b.w./day PQQ⋅Na2 treatment; PQQ 5, intragastric administration with 5.0 mg/kg b.w./day PQQ⋅Na2 treatment; PQQ 10, intragastric administration with 10.0 mg/kg b.w./day PQQ⋅Na2 treatment. *Means significant difference with the CTRL group (P ≤ 0.05); #means significant difference with the LPS group (P ≤ 0.05).
mRNA Abundance of Neuropeptides in the Ileum
Compared with the CTRL group, mRNA concentrations for NPY and NGF were increased (P < 0.05, Figure 5) in the LPS group. Compared with the LPS group, mRNA concentrations for BDNF and NGF were decreased (P < 0.05) in PQQ 2.5, and mRNA concentration for NPY decreased (P < 0.05) in PQQ 5.
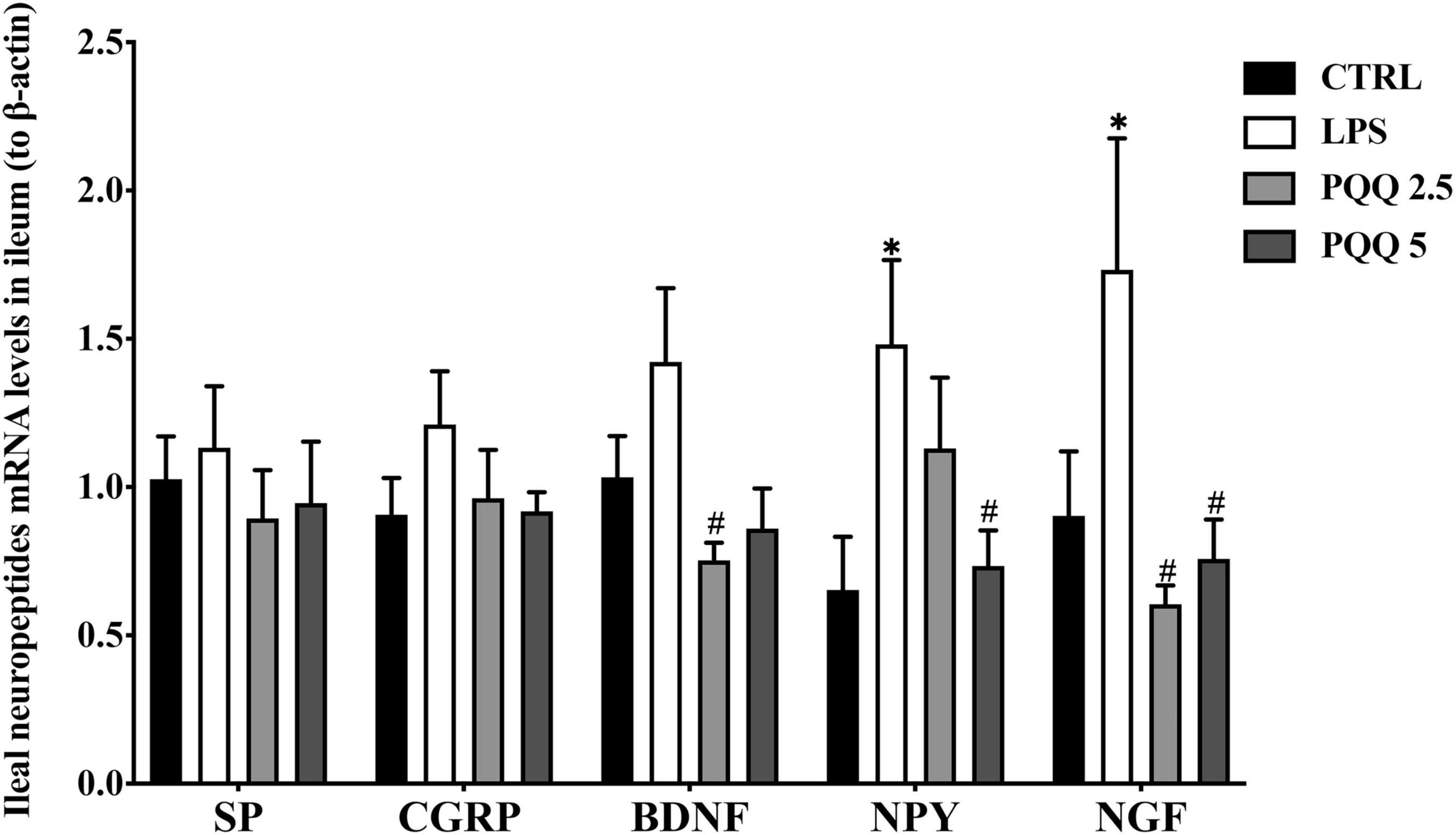
Figure 5. Neuropeptide levels in serum of weaned rats. NPY, neuropeptide Y (n = 6); NGF, nerve growth factor (n = 5); VIP, vasoactive intestinal peptide (n = 6); SP, substance P (n = 5); CGRP, calcitonin gene-related peptide (n = 5); BDNF, brain-derived neurotropic factor (n = 5). CTRL, control treatment; LPS, control and LPS treatment; PQQ 2.5, intragastric administration with 2.5 mg/kg b.w./day PQQ⋅Na2 treatment; PQQ 5, intragastric administration with 5.0 mg/kg b.w./day PQQ⋅Na2 treatment; PQQ 10, intragastric administration with 10.0 mg/kg b.w./day PQQ⋅Na2 treatment. *Means significant difference with the CTRL group (P ≤ 0.05); #means significant difference with the LPS group (P ≤ 0.05).
Concentration of Neuropeptides in Jejunum, Ileum and Colon
Compared with the CTRL group, concentrations of all detected neuropeptides were increased (P < 0.05, Table 2) in the jejunum and ileum of rats in the LPS group. Compared with the LPS group, concentrations of CGRP, VIP, NPY, and NGF were decreased (P < 0.05) in PQQ-fed groups in the jejunum, and SP and BDNF concentrations were decreased (P < 0.05) in PQQ 5 and PQQ 10 groups. In the ileum, concentrations of all neuropeptides measured were decreased (P < 0.05) in all PQQ-fed groups. In the colon, VIP concentration was increased, and BDNF concentration was decreased in the LPS group compared with the CTRL group (P < 0.05). Compared with the LPS group, colonic SP concentration was decreased (P < 0.05) in PQQ 10, CGRP concentration was increased (P < 0.05) in PQQ 5, VIP concentration was decreased (P < 0.05) in PQQ 2.5 and PQQ 5, BDNF concentration was increased (P < 0.05) in PQQ 5, NPY concentration was increased (P < 0.05) in PQQ 10, and NGF concentration was increased in PQQ 5.
Immunostaining of Neuropeptides in Jejunum and Colon
Compared with the CTRL group, the percentage of ganglia immunoreactive for PGP9.5 in the jejunum was decreased (P < 0.05, Table 3) in the LPS group and showed a lighter PGP9.5-positive area dyed with brown (Figure 6). Compared with the LPS group, the PGP9.5 immunoreactive percentage of ganglia in the jejunum of rats was increased (P < 0.05, Table 3) in the PQQ 5 group. Compared with the CTRL group, NGF-positive surface area in the jejunum was increased (P < 0.05, Table 3) and dyed darker (Figure 6) in the LPS group.
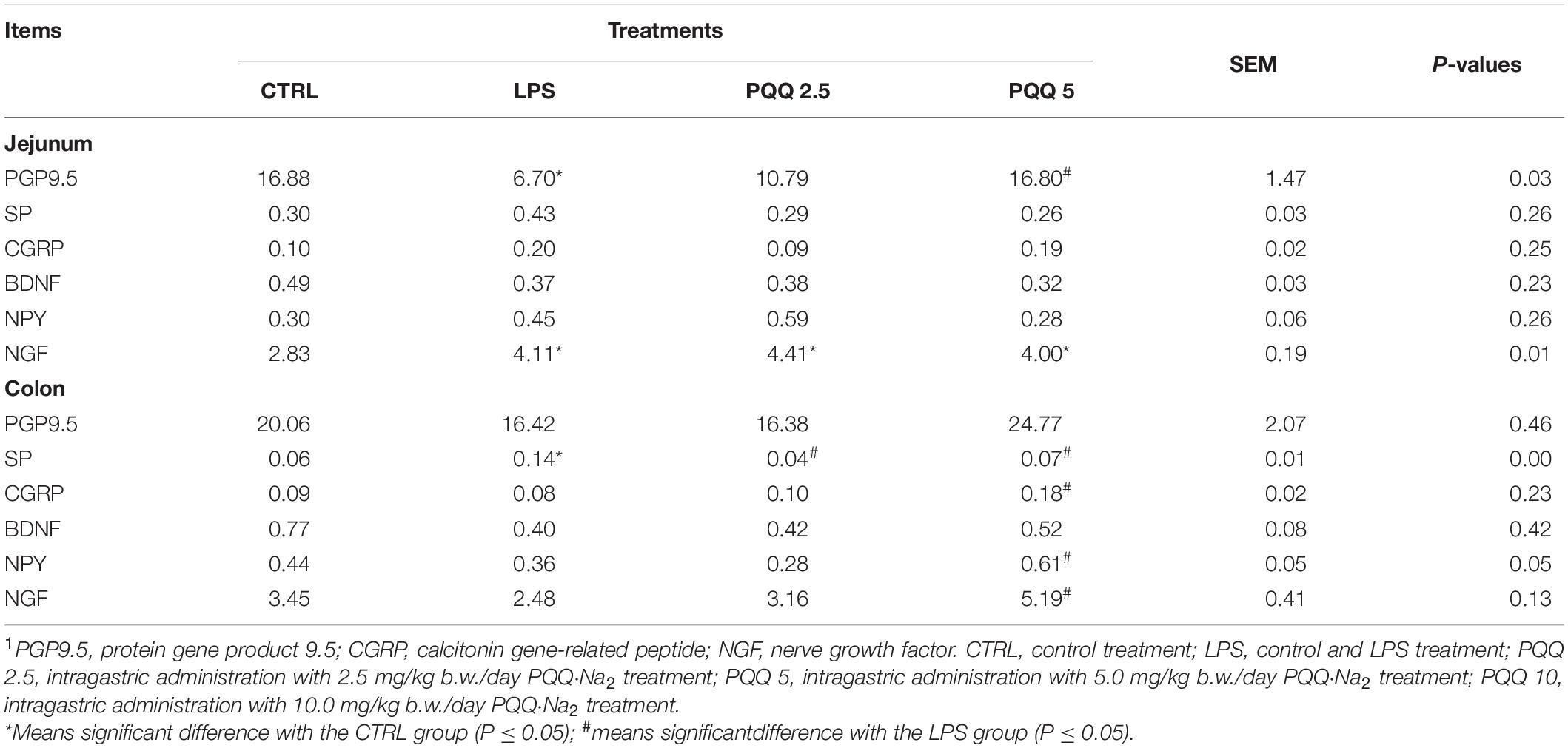
Table 3. Percentage of ganglia immunoreactive for neuropeptides in the total area by immunohistochemical staining (n = 6, %).1
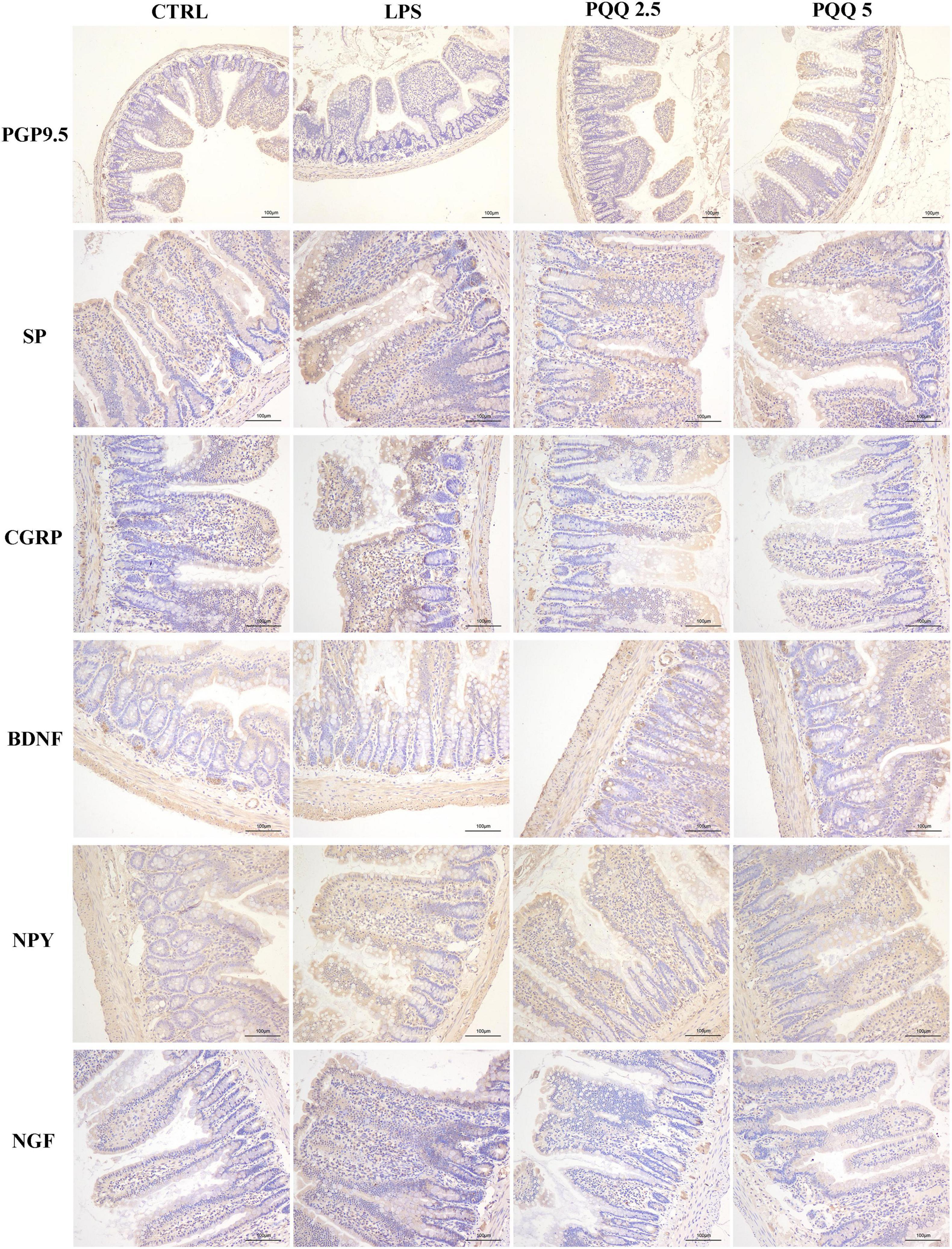
Figure 6. Immunohistochemical staining of jejunal neurons (n = 6). PGP9.5, protein gene product 9.5; SP, substance P; CGRP, calcitonin gene-related peptide; BDNF, brain-derived neurotropic factor; NPY, neuropeptide Y; NGF, nerve growth factor. CTRL, control treatment; LPS, control and LPS treatment; PQQ 2.5, intragastric administration with 2.5 mg/kg b.w./day PQQ⋅Na2 treatment; PQQ 5, intragastric administration with 5.0 mg/kg b.w./day PQQ⋅Na2 treatment; PQQ 10, intragastric administration with 10.0 mg/kg b.w./day PQQ⋅Na2 treatment. Scale bar = 100 μm.
In the colon, SP-positive surface area increased (P < 0.05, Table 3) in the LPS group compared with the CTRL group. PQQ treatments decreased (P < 0.05, Table 3) SP-positive surface area in the colon compared with the LPS group. Colonic CGRP, NPY, and NGF were increased in the PQQ 5 group compared with the LPS group (P < 0.05, Table 3) and dyed darker (Figure 7) in the PQQ 5 group.
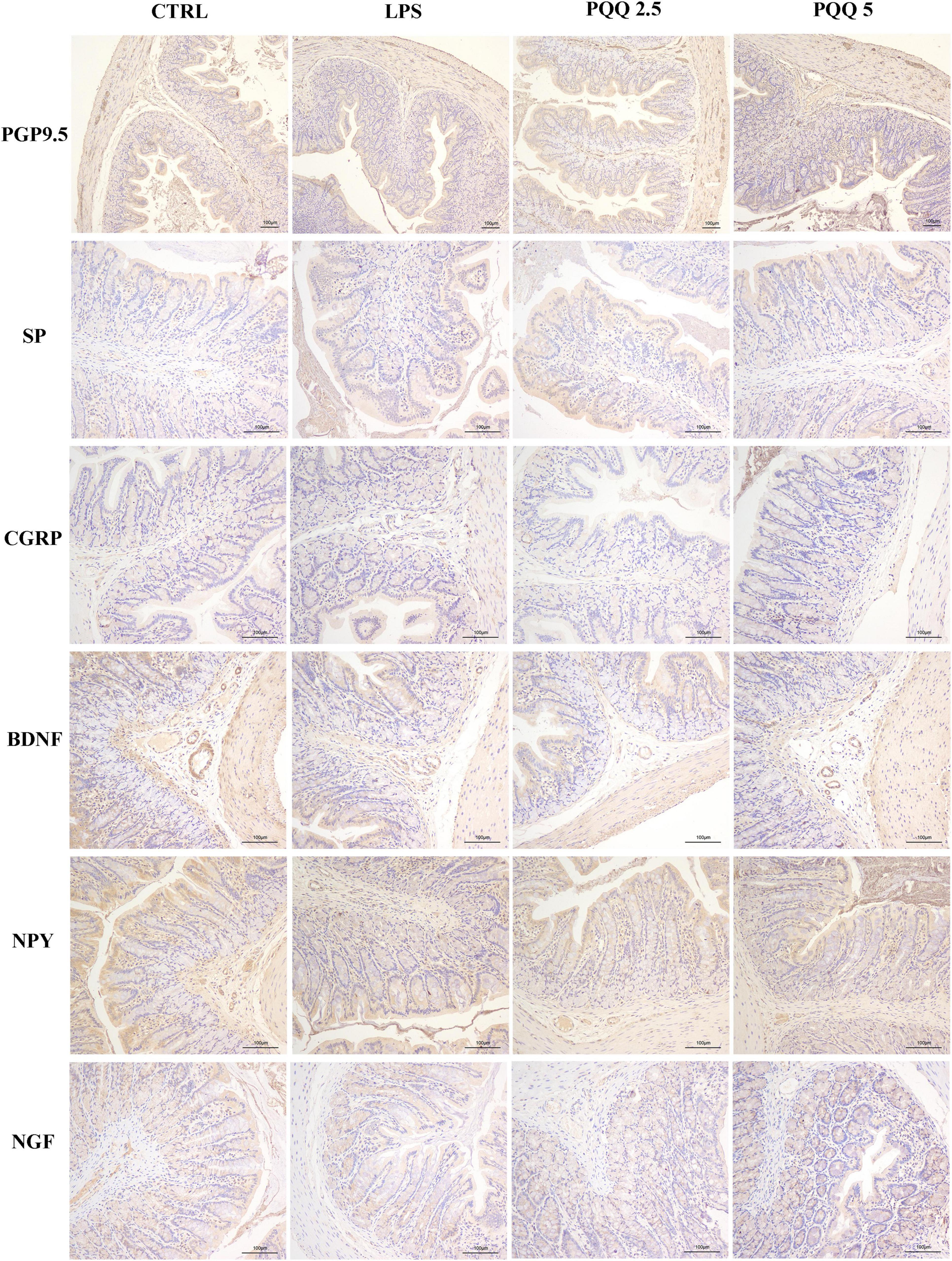
Figure 7. Immunohistochemical staining of colonic neurons (n = 6). PGP9.5, protein gene product 9.5; SP, substance P; CGRP, calcitonin gene-related peptide; BDNF, brain-derived neurotropic factor; NPY, neuropeptide Y; NGF, nerve growth factor. CTRL, control treatment; LPS, control and LPS treatment; PQQ 2.5, intragastric administration with 2.5 mg/kg b.w./day PQQ⋅Na2 treatment; PQQ 5, intragastric administration with 5.0 mg/kg b.w./day PQQ⋅Na2 treatment; PQQ 10, intragastric administration with 10.0 mg/kg b.w./day PQQ⋅Na2 treatment. Scale bar = 100 μm.
Activation of the Akt Pathway in Jejunum and Colon
Compared with the CTRL group, p-Akt/Akt was increased in LPS groups both in the jejunum and colon ofrats (P < 0.05, Figures 8A,B). Compared with the LPS group, p-Akt/Akt was decreased (P < 0.05, Figure 8A) in the jejunum of rats both in PQQ 2.5 and PQQ 5 groups. Additionally, p-Akt/Akt was decreased in the colon of rats in the PQQ 5 group compared with the LPS group (P < 0.05, Figure 8B). The abundance of PI3K was not affected by any treatments in both the jejunum and colon.
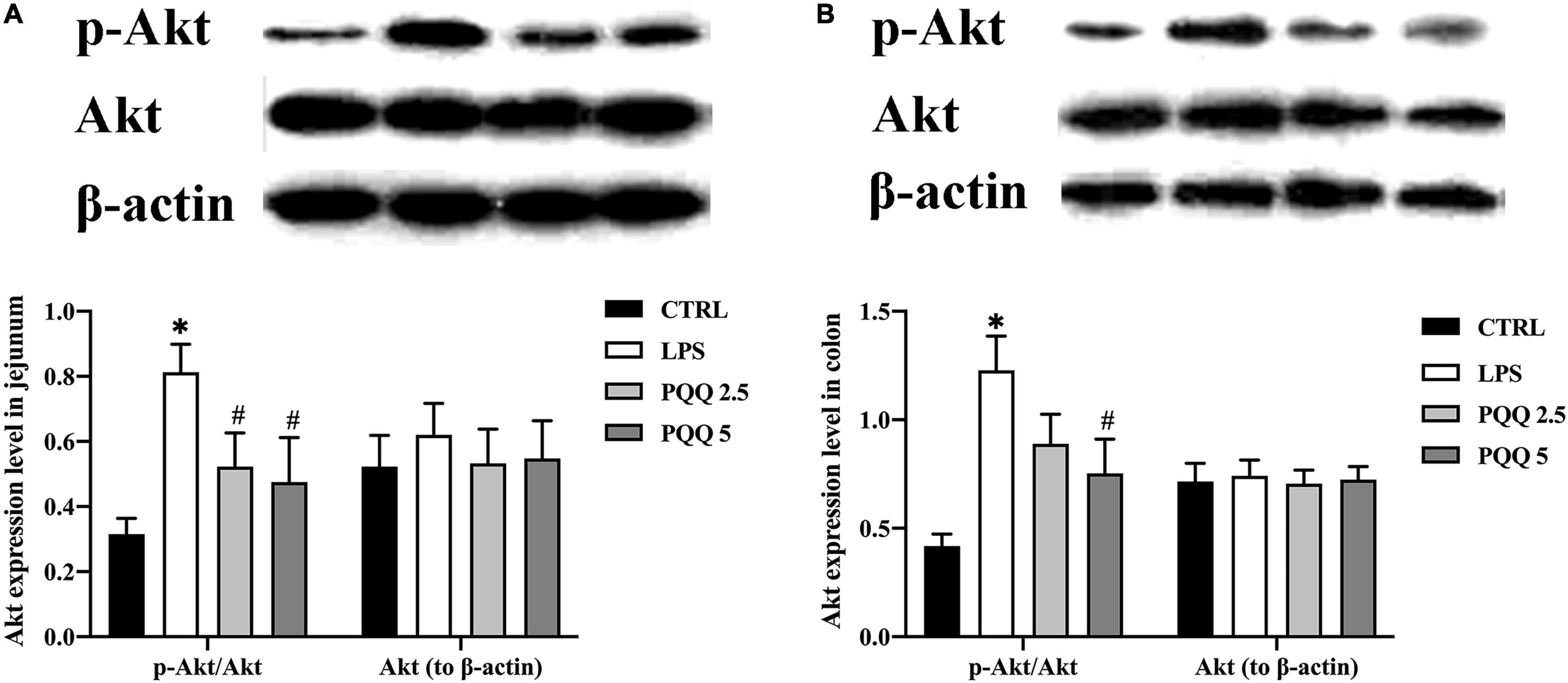
Figure 8. Abundance of the Akt pathway in jejunal and colonic tissues of weaned rats (n = 6). (A) p-Akt, Akt, and PI3K abundances to β-actin in jejunum; (B) p-Akt, Akt, andPI3K abundances to β-actin in the colon. PI3K, phosphatidylinositol-3 kinase; Akt, protein kinase B; CTRL, control treatment; LPS, control and LPS treatment; PQQ 2.5, intragastric administration with 2.5 mg/kg b.w./day PQQ⋅Na2 treatment; PQQ 5, intragastric administration with 5.0 mg/kg b.w./day PQQ⋅Na2 treatment; PQQ 10, intragastric administration with 10.0 mg/kg b.w./day PQQ⋅Na2 treatment. *Means significant difference with the CTRL group (P ≤ 0.05); #means significant difference with the LPS group (P ≤ 0.05).
Discussion
Pyrroloquinoline quinone is a natural antioxidant that has been used in the treatment of osteoporosis, muscle atrophy, radiation poisoning, and arthritis and promotes the growth of the organisms by regulating oxidation, repairing DNA damage, reducing apoptosis, and maintaining mitochondrial function (Kasahara and Kato, 2003; Huang et al., 2017; Wu et al., 2017; Xu et al., 2018; Geng et al., 2019; Jiang et al., 2019). We previously demonstrated that dietary PQQ could increase ADG and gain to feed ratio and reduce diarrhea incidence in weaned pigs (Yin et al., 2019; Huang et al., 2021; Ming et al., 2021). In this study, daily treatment with 5.0 mg/kg and 10.0 mg/kg PQQ increased ADG of rats compared with rats not supplemented with PQQ.
We developed the enteritis model by challenging rats with LPS and verified the existence of enteritis by evaluating jejunal morphology and cytokines in serum and intestine. LPS is a component of Gram-negative bacteria that can damage the intestinal barrier cause immune dysfunction, initiate apoptosis of intestine epithelial cells, and develop enteritis (Yoshioka et al., 2009; Li et al., 2018; Dong et al., 2020). In this study, rats challenged with LPS developed inflamed intestines as evidenced by damaged jejunal morphology, increased concentrations of IL-1β, IL-6, and TNF-α in serum and IL-1β and TNF-α in intestine, and decreased IL-10 in serum. These observations confirmed that rats challenged with LPS developed intestinal inflammation.
Intestinal morphology and immune function improved with PQQ supplementation of LPS-challenged rats. Intestinal damage reduces absorption and metabolism of nutrients and compromises the integrity of the intestinal barrier (Baumgart and Carding, 2007; Turner, 2009; Wang et al., 2015; Zhou et al., 2018). Intestinal morphology can be improved in weaned rats when they were supplemented with PQQ (Zhang et al., 2019). Our previous study showed that dietary supplementation with PQQ can increase intestinal VH and VCR in weaned pigs (Yin et al., 2019). In this study, PQQ treatment increased jejunal VH and VCR compared with rats challenged with LPS. PQQ has anti-inflammatory effects and can reduce arthritis by inhibiting the production of pro-inflammatory cytokines such as TNF-α and IL-6 (Harris et al., 2013; Liu et al., 2016). In our previous study, PQQ supplementation reduced gut inflammation which improved intestinal health and growth of weaned pigs (Yin et al., 2019; Huang et al., 2020). In this study, PQQ supplementation decreased concentrations of pro-inflammatory cytokines in serum and intestine and increased concentrations of the anti-inflammatory cytokine and IL-10 in serum, which confirmed that PQQ regulates immune responses.
Pyrroloquinoline quinone can regulate enteric nervous damage induced by LPS challenge. The ENS regulates intestinal secretions, peristalsis, and immunity through endocrine substances and neuropeptides secreted by myenteric neurons, submucosal neurons, and glial cells (Rao and Gershon, 2018; Boesmans et al., 2019). Normally, the neuropeptides are divided into inhibitory neurotransmitters and excitatory neurotransmitters to control intestinal relaxation and contraction, respectively (Rao and Gershon, 2018). When the intestine suffers pressure, mucosal damage, and inflammation, the ENS can release neuropeptides to regulate intestinal peristaltic and immune factors (Jakob et al., 2020). PGP 9.5 is a neuroendocrine marker and is reduced with intestinal inflammation and damage (Resnikoff et al., 2019; Heymans et al., 2020). In this study, the immunoreactive percentage of PGP9.5 in the jejunum was decreased by LPS treatment and increased by PQQ treatment, suggesting that PQQ reduced the damage of jejunal neurons caused by LPS.
Enteric neurochemical plasticity was regulated with PQQ treatment by regulating immunoreactive neurons and concentrations of neuropeptides. Neurochemical plasticity is the variable or modifiable changes of the nervous system elicited by adaptation to the environment. During the early development of rats, the ENS matures gradually and has the strongest neurochemical plasticity (Rza̧p et al., 2020). As the first isolated neuropeptide and known as the prototypic tachykinin (TK), SP stimulates systemic pain and vasodilation, regulates immunity, promotes contraction as an excitatory neurotransmitter, and inhibits the secretion of digestive juices in the intestine (Euler and Gaddum, 1931; Holzer, 1998; Engel et al., 2012; Nässel et al., 2019). The concentration of SP is enhanced in the blood of rats suffering from colitis (Holzer, 1998). As a systemic vasodilator, CGRP simulates pain and regulates intestinal immunity similar to SP (Holzer, 1998; Engel et al., 2012; Lai et al., 2020). Sensory neurons in the gut of mice release CGRP to defend against Salmonella infection, and neurogenic inflammation challenged with LPS can promote CGRP release via activation of Toll-like receptor 4 (TLR4) in sensory neurons (Meseguer et al., 2014; Lai et al., 2020). As an inhibitory neurotransmitter and neuroprotective agent in the intestine, VIP is also an immunomodulator (Gonzalez-Rey and Delgado, 2005; Arciszewski et al., 2008). Rat myenteric neurons challenged with LPS increase the expression of VIP (Arciszewski et al., 2008). Except for promoting differentiation, development, and survival of neurons, BDNF regulates the sensitivity of the colon and rectum to constipation (Delafoy et al., 2006). It is synthesized by sensory neurons to mediate inflammatory pain and regulate the sensitivity of visceral afferents in rats suffering from colitis (Matayoshi et al., 2005; Qiao et al., 2008). With systemic stress, NPY is released, regulates endocrine, behavior, stress, anxiety, appetite, and circadian rhythms, and functions in defecation and food intake (Adrian et al., 1983; Zhou et al., 2008). The level of NPY is increased in the hypothalamus and serum of mice suffering from colitis (Hassan et al., 2014; Reichmann et al., 2015). NGF is a nutrient protein for nerve cells and promotes the repair of damaged nerve fibers (Bilderback et al., 1999). At the site of inflammation, NGF concentration is increased, and cytokines promote the synthesis of NGF by neurons and other cells such as epithelial and endothelial cells (März et al., 1999; Minnone et al., 2017). In this study, concentrations of these neuropeptides were increased in serum and small intestine of rats in the LPS group. The increased levels were reduced with PQQ treatment. The optional PQQ dose approved to be 5.0 mg/kg b.w. The concentrations of IL-1β and TNF-α display a similar pattern to neuropeptides, suggesting that neuropeptides regulated immune factors concentrations. In the colon, SP and VIP concentrations showed the same trends as observed in the jejunum. This might be due to the main site of inflammation challenged with LPS. Additionally, P and VIP play the main role of immunomodulators in the nervous system. Treatments with PQQ increased concentrations of BDNF, NPY, and NGF in the colon which might be related to their neurotropic effects and the damage caused by the LPS challenge. These neuropeptides function in stress and neurotropic. When the intestine is damaged, the neuropeptides secreted by the ENS aim to promote neuronal survival and maintain normal functions (Holzer, 1998; Arciszewski et al., 2008; Qiao et al., 2008). We deduced that PQQ played a different role in different pathological states, and we would investigate further. In conclusion, the results reported in this study suggest that PQQ influenced the release of neuropeptides which regulated the small intestine. We conclude that PQQ regulated enteric neurochemical plasticity of SP-, CGRP-, VIP-, BDNF-, NPY-, and NGF-immunoreactive neurons of weaned rats.
Expression of p-Akt decreased in jejunum and colon with PQQ supplementation. Akt can be phosphorylated by PI3K. Activated Akt can reduce and improve the activity of glycogen synthase kinase-3β (GSK-3β) by phosphorylation of Ser9 and Tyr216 sites, respectively (Majewska and Szeliga, 2017). GSK-3β is associated with cell survival and apoptosis, which can promote peripheral nerve regeneration, improve regrowth of synapses after peripheral nerve damage, and play a role in neurodegenerative diseases (Kitagishi et al., 2014; Huang et al., 2017). Activated GSK-3β can induce Tau perphosphate. Tau protein, a microtube-related protein, mainly acts on the far end of the axon, participates in axon transport, and interacts with the microtube protein, Tubulin, to stabilize the microtube and regulate NMDA receptor signaling pathways (Goedert et al., 1996; Iqbal et al., 2016; Falcon et al., 2019). By regulating GSK-3β and Tau protein, the PI3K/Akt signaling pathway affects a variety of CNS diseases, such as Alzheimer’s disease, Parkinson’s disease, and Huntington’s disease (Kitagishi et al., 2014; Rai et al., 2019). PQQ inhibits apoptosis in the rat hippocampus by regulating the Akt/GSK-3β pathway (Zhou et al., 2020). In addition, PQQ can regulate memory via maintaining activation of GSK-3β while reducing the expression of p-AKT (Zhou X. Q. et al., 2018). In this study, PQQ reduced the magnitude of increased p-Akt in jejunum and colon of rats caused by LPS treatment which suggests that PQQ might regulate the secretory functions and structure of the ENS via the Akt signaling pathway.
In conclusion, based on ADG, jejunal morphology, immune responses, and enteric neuropeptide expression, we conclude that the intestinal health of weaned rats was damaged by the LPS challenge. Dietary PQQ supplementation reduced inflammatory injury and regulated neurochemical plasticity via the Akt signaling pathway in the intestine of rats suffering from enteritis.
Data Availability Statement
The original contributions presented in the study are included in the article/Supplementary Material, further inquiries can be directed to the corresponding author.
Ethics Statement
The animal study was reviewed and approved by the Laboratory Animal Welfare and Animal Experimental Ethical Inspection Committee of China Agricultural University (AW01211202-1-2).
Author Contributions
CS: conceptualization, data curation, formal analysis, investigation, methodology, and writing—original draft. SX, CH, ZW, WW, DM, XY, and HL: data curation and methodology. FW: conceptualization, writing – review, supervision, and funding acquisition. All authors have read and agreed to the published version of the manuscript.
Funding
This study was financially supported by the National Natural Science Foundation of China (Grant Nos. 32072772 and 31672459).
Conflict of Interest
The authors declare that the research was conducted in the absence of any commercial or financial relationships that could be construed as a potential conflict of interest.
Publisher’s Note
All claims expressed in this article are solely those of the authors and do not necessarily represent those of their affiliated organizations, or those of the publisher, the editors and the reviewers. Any product that may be evaluated in this article, or claim that may be made by its manufacturer, is not guaranteed or endorsed by the publisher.
Acknowledgments
We express our real thanks to Lee Johnston (University of Minnesota) and Bing Dong (China Agricultural University) for giving suggestions for revisions.
Supplementary Material
The Supplementary Material for this article can be found online at: https://www.frontiersin.org/articles/10.3389/fnins.2022.878541/full#supplementary-material
References
Adrian, T. E., Allen, J. M., Bloom, S. R., Ghatei, M. A., Rossor, M. N., Roberts, G. W., et al. (1983). Neuropeptide Y distribution in human brain. Nature 306, 584–586. doi: 10.1038/306584a0
Arciszewski, M. B., Sand, E., and Ekblad, E. (2008). Vasoactive intestinal peptide rescues cultured rat myenteric neurons from lipopolysaccharide induced cell death. Regul. Pept. 146, 218–223. doi: 10.1016/j.regpep.2007.09.021
Baumgart, D. C., and Carding, S. R. (2007). Inflammatory bowel disease: cause and immunobiology. Lancet 369, 1627–1640. doi: 10.1016/S0140-6736(07)60750-8
Becker, L., Peterson, J., Kulkarni, S., and Pasricha, P. J. (2013). Ex vivo neurogenesis within enteric ganglia occurs in a PTEN dependent manner. PLoS One 8:e59452.
Bilderback, T. R., Gazula, V. R., Lisanti, M. P., and Dobrowsky, R. T. (1999). Caveolin interacts with Trk A and p75(NTR) and regulates neurotrophin signaling pathways. J. Biol. Chem. 274, 257–263. doi: 10.1074/jbc.274.1.257
Boesmans, W., Hao, M. M., Fung, C., Li, Z., Van den Haute, C., Tack, J., et al. (2019). Structurally defined signaling in neuro-glia units in the enteric nervous system. Glia 67, 1167–1178. doi: 10.1002/glia.23596
De Giorgio, R. (2006). The enteric nervous system, J. B. furness. Blackwell publishing, oxford (2006), (274 pp., price gbp 55.00, isbn 1-4051-3376-7). Dig. Liver Dis. 38:441. doi: 10.1016/j.dld.2006.03.010
Delafoy, L., Gelot, A., Ardid, D., Eschalier, A., Bertrand, C., Doherty, A. M., et al. (2006). Interactive involvement of brain derived neurotrophic factor, nerve growth factor, and calcitonin gene related peptide in colonic hypersensitivity in the rat. Gut 55, 940–945. doi: 10.1136/gut.2005.064063
Dong, N., Li, X., Xue, C., Zhang, L., Wang, C., Xu, X., et al. (2020). Astragalus polysaccharides alleviates LPS-induced inflammation via the NF-κB/MAPK signaling pathway. J. Cell Physiol. 235, 5525–5540. doi: 10.1002/jcp.29452
Du, F., Wang, L., Qian, W., and Liu, S. (2009). Loss of enteric neurons accompanied by decreased expression of GDNF and PI3K/Akt pathway in diabetic rats. Neurogastroenterol. Motil. 21, 1229–e1114.
Duine, J. A. (1991). Quinoproteins: enzymes containing the quinonoid cofactor pyrroloquinoline quinone, topaquinone or tryptophan-tryptophan quinone. Eur. J. Biochem. 200, 271–284. doi: 10.1111/j.1432-1033.1991.tb16183.x
Duine, J. A., Frank, J., and van Zeeland, J. K. (1979). Glucose dehydrogenase from Acinetobacter calcoaceticus: a ‘quinoprotein’. FEBS Lett. 108, 443–446. doi: 10.1016/0014-5793(79)80584-0
Engel, M. A., Khalil, M., Siklosi, N., Mueller-Tribbensee, S. M., Neuhuber, W. L., Neurath, M. F., et al. (2012). Opposite effects of substance P and calcitonin gene-related peptide in oxazolone colitis. Dig. Liver Dis. 44, 24–29. doi: 10.1016/j.dld.2011.08.030
Falcon, B., Zivanov, J., Zhang, W., Murzin, A. G., Garringer, H. J., Vidal, R., et al. (2019). Novel tau filament fold in chronic traumatic encephalopathy encloses hydrophobic molecules. Nature 568, 420–423. doi: 10.1038/s41586-019-1026-5
Ferraris, R. P., Lee, P. P., and Diamond, J. M. (1989). Origin of regional and species differences in intestinal glucose uptake. Am. J. Physiol. 257(5 Pt 1), G689–G697. doi: 10.1152/ajpgi.1989.257.5.G689
Geng, Q., Gao, H., Yang, R., Guo, K., and Miao, D. (2019). Pyrroloquinoline quinone prevents estrogen deficiency-induced osteoporosis by inhibiting oxidative stress and osteocyte senescence. Int. J. Biol. Sci. 15, 58–68. doi: 10.7150/ijbs.25783
Goedert, M., Jakes, R., Spillantini, M. G., Hasegawa, M., Smith, M. J., and Crowther, R. A. (1996). Assembly of microtubule-associated protein tau into Alzheimer-like filaments induced by sulphated glycosaminoglycans. Nature 383, 550–553. doi: 10.1038/383550a0
Gonzalez-Rey, E., and Delgado, M. (2005). Role of vasoactive intestinal peptide in inflammation and autoimmunity. Curr. Opin. Investig. Drugs 6, 1116–1123.
Hara, H., Hiramatsu, H., and Adachi, T. (2007). Pyrroloquinoline quinone is a potent neuroprotective nutrient against 6-hydroxydopamine-induced neurotoxicity. Neurochem. Res. 32, 489–495. doi: 10.1007/s11064-006-9257-x
Harris, C. B., Chowanadisai, W., Mishchuk, D. O., Satre, M. A., Slupsky, C. M., and Rucker, R. B. (2013). Dietary pyrroloquinoline quinone (PQQ) alters indicators of inflammation and mitochondrial-related metabolism in human subjects. J. Nutr. Biochem. 24, 2076–2084. doi: 10.1016/j.jnutbio.2013.07.008
Hassan, A. M., Jain, P., Reichmann, F., Mayerhofer, R., Farzi, A., Schuligoi, R., et al. (2014). Repeated predictable stress causes resilience against colitis-induced behavioral changes in mice. Front. Behav. Neurosci. 8:386. doi: 10.3389/fnbeh.2014.00386
Heymans, C., de Lange, I. H., Hütten, M. C., Lenaerts, K., de Ruijter, N. J. E., Kessels, L., et al. (2020). Chronic intra-uterine ureaplasma parvum infection induces injury of the enteric nervous system in ovine fetuses. Front. Immunol. 11:189. doi: 10.3389/fimmu.2020.00189
Holzer, P. (1998). Implications of tachykinins and calcitonin gene-related peptide in inflammatory bowel disease. Digestion 59, 269–283. doi: 10.1159/000007504
Huang, C., Fan, Z., Han, D., Johnston, L. J., Ma, X., and Wang, F. (2021). Pyrroloquinoline quinone regulates the redox status in vitro and in vivo of weaned pigs via the Nrf2/HO-1 pathway. J. Anim. Sci. Biotechnol. 12:77. doi: 10.1186/s40104-021-00595-x
Huang, C., Ming, D., Wang, W., Wang, Z., Hu, Y., Ma, X., et al. (2020). Pyrroloquinoline quinone alleviates jejunal mucosal barrier function damage and regulates colonic microbiota in piglets challenged with enterotoxigenic Escherichia coli. Front. Microbiol. 11:1754. doi: 10.3389/fmicb.2020.01754
Huang, H., Liu, H., Yan, R., and Hu, M. (2017). PI3K/Akt and ERK/MAPK signaling promote different aspects of neuron survival and axonal regrowth following rat facial nerve axotomy. Neurochem. Res. 42, 3515–3524. doi: 10.1007/s11064-017-2399-1
Huang, Y., Chen, N., and Miao, D. (2017). Effect and mechanism of pyrroloquinoline quinone on anti-osteoporosis in Bmi-1 knockout mice-Anti-oxidant effect of pyrroloquinoline quinone. Am. J. Transl. Res. 9, 4361–4374.
Ichihara, M., Murakumo, Y., and Takahashi, M. (2004). RET and neuroendocrine tumors. Cancer Lett. 204, 197–211. doi: 10.1016/s0304-3835(03)00456-7
Iqbal, K., Liu, F., and Gong, C. X. (2016). Tau and neurodegenerative disease: the story so far. Nat. Rev. Neurol. 12, 15–27. doi: 10.1038/nrneurol.2015.225
Jakob, M. O., Murugan, S., and Klose, C. S. N. (2020). Neuro-immune circuits regulate immune responses in tissues and organ homeostasis. Front. Immunol. 11:308. doi: 10.3389/fimmu.2020.00308
Jarret, A., Jackson, R., Duizer, C., Healy, M. E., Zhao, J., Rone, J. M., et al. (2020). Enteric nervous system-derived il-18 orchestrates mucosal barrier immunity. Cell 180, 50–63.e12. doi: 10.1016/j.cell.2019.12.016
Jiang, C., Jiang, L., Li, Q., Liu, X., Zhang, T., Yang, G., et al. (2019). Pyrroloquinoline quinine ameliorates doxorubicin-induced autophagy-dependent apoptosis via lysosomal-mitochondrial axis in vascular endothelial cells. Toxicology 425, 152238. doi: 10.1016/j.tox.2019.152238
Kasahara, T., and Kato, T. (2003). Nutritional biochemistry: a new redox-cofactor vitamin for mammals. Nature 422:832. doi: 10.1038/422832a
Kitagishi, Y., Nakanishi, A., Ogura, Y., and Matsuda, S. (2014). Dietary regulation of PI3K/AKT/GSK-3β pathway in Alzheimer’s disease. Alzheimers Res. Ther. 6:35. doi: 10.1186/alzrt265
Lai, N. Y., Musser, M. A., Pinho-Ribeiro, F. A., Baral, P., Jacobson, A., Ma, P., et al. (2020). Gut-innervating nociceptor neurons regulate peyer’s patch microfold cells and SFB levels to mediate Salmonella host defense. Cell 180, 33–49.e22. doi: 10.1016/j.cell.2019.11.014
Lasrado, R., Boesmans, W., Kleinjung, J., Pin, C., Bell, D., Bhaw, L., et al. (2017). Lineage-dependent spatial and functional organization of the mammalian enteric nervous system. Science 356, 722–726. doi: 10.1126/science.aam7511
Li, L., Wan, G., Han, B., and Zhang, Z. (2018). Echinacoside alleviated LPS-induced cell apoptosis and inflammation in rat intestine epithelial cells by inhibiting the mTOR/STAT3 pathway. Biomed. Pharmacother. 104, 622–628. doi: 10.1016/j.biopha.2018.05.072
Liu, S., Li, H., Ou Yang, J., Peng, H., Wu, K., Liu, Y., et al. (2005). Enhanced rat sciatic nerve regeneration through silicon tubes filled with pyrroloquinoline quinone. Microsurgery 25, 329–337. doi: 10.1002/micr.20126
Liu, Z., Sun, C., Tao, R., Xu, X., Xu, L., Cheng, H., et al. (2016). Pyrroloquinoline quinone decelerates rheumatoid arthritis progression by inhibiting inflammatory responses and joint destruction via modulating NF-κB and MAPK pathways. Inflammation 39, 248–256. doi: 10.1007/s10753-015-0245-7
Lu, H., Shen, J., Song, X., Ge, J., Cai, R., Dai, A., et al. (2015). protective effect of pyrroloquinoline quinone (PQQ) in rat model of intracerebral hemorrhage. Cell Mol. Neurobiol. 35, 921–930. doi: 10.1007/s10571-015-0187-5
Majewska, E., and Szeliga, M. (2017). AKT/GSK3β signaling in glioblastoma. Neurochem. Res. 42, 918–924. doi: 10.1007/s11064-016-2044-4
März, P., Heese, K., Dimitriades-Schmutz, B., Rose-John, S., and Otten, U. (1999). Role of interleukin-6 and soluble IL-6 receptor in region-specific induction of astrocytic differentiation and neurotrophin expression. Glia 26, 191–200. doi: 10.1002/(sici)1098-1136(199905)26:3<191::aid-glia1>3.0.co;2-#
Matayoshi, S., Jiang, N., Katafuchi, T., Koga, K., Furue, H., Yasaka, T., et al. (2005). Actions of brain-derived neurotrophic factor on spinal nociceptive transmission during inflammation in the rat. J. Physiol. 569(Pt 2), 685–695. doi: 10.1113/jphysiol.2005.095331
Meseguer, V., Alpizar, Y. A., Luis, E., Tajada, S., Denlinger, B., Fajardo, O., et al. (2014). TRPA1 channels mediate acute neurogenic inflammation and pain produced by bacterial endotoxins. Nat. Commun. 5:3125. doi: 10.1038/ncomms4125
Ming, D., Huang, C., Wang, W., Wang, Z., Shi, C., Yin, X., et al. (2021). Effects of diet supplemented with excess pyrroloquinoline quinone disodium on growth performance, blood parameters and redox status in weaned pigs. Animals (Basel) 11:359. doi: 10.3390/ani11020359
Minnone, G., De Benedetti, F., and Bracci-Laudiero, L. (2017). NGF and its receptors in the regulation of inflammatory response. Int. J. Mol. Sci. 18:1028. doi: 10.3390/ijms18051028
Moeser, A. J., Pohl, C. S., and Rajput, M. (2017). Weaning stress and gastrointestinal barrier development: implications for lifelong gut health in pigs. Anim. Nutr. 3, 313–321. doi: 10.1016/j.aninu.2017.06.003
Mohamed, A. S., Hosney, M., Bassiony, H., Hassanein, S. S., Soliman, A. M., Fahmy, S. R., et al. (2020). Sodium pentobarbital dosages for exsanguination affect biochemical, molecular and histological measurements in rats. Sci. Rep. 10:378. doi: 10.1038/s41598-019-57252-7
Montedonico, S., Sri Paran, T., Pirker, M., Rolle, U., and Puri, P. (2006). Developmental changes in submucosal nitrergic neurons in the porcine distal colon. J. Pediatr. Surg. 41, 1029–1035. doi: 10.1016/j.jpedsurg.2005.12.063
Nässel, D. R., Zandawala, M., Kawada, T., and Satake, H. (2019). Tachykinins: neuropeptides that are ancient, diverse, widespread and functionally pleiotropic. Front. Neurosci. 13:1262. doi: 10.3389/fnins.2019.01262
Parathan, P., Wang, Y., Leembruggen, A. J., Bornstein, J. C., and Foong, J. P. (2020). The enteric nervous system undergoes significant chemical and synaptic maturation during adolescence in mice. Dev. Biol. 458, 75–87. doi: 10.1016/j.ydbio.2019.10.011
Pfaffl, M. W., Horgan, G. W., and Dempfle, L. (2002). Relative expression software tool (REST) for group-wise comparison and statistical analysis of relative expression results in real-time PCR. Nucleic Acids Res. 30:e36.
Qiao, L. Y., Gulick, M. A., Bowers, J., Kuemmerle, J. F., and Grider, J. R. (2008). Differential changes in brain-derived neurotrophic factor and extracellular signal-regulated kinase in rat primary afferent pathways with colitis. Neurogastroenterol. Motil. 20, 928–938.
Rai, S. N., Dilnashin, H., Birla, H., Singh, S. S., Zahra, W., Rathore, A. S., et al. (2019). The role of PI3K/Akt and ERK in neurodegenerative disorders. Neurotox Res. 35, 775–795. doi: 10.1007/s12640-019-0003-y
Rao, M., and Gershon, M. D. (2018). Enteric nervous system development: what could possibly go wrong? Nat. Rev. Neurosci. 19, 552–565. doi: 10.1038/s41583-018-0041-0
Reichmann, F., Hassan, A. M., Farzi, A., Jain, P., Schuligoi, R., and Holzer, P. (2015). Dextran sulfate sodium-induced colitis alters stress-associated behaviour and neuropeptide gene expression in the amygdala-hippocampus network of mice. Sci. Rep. 5:9970. doi: 10.1038/srep09970
Resnikoff, H., Metzger, J. M., Lopez, M., Bondarenko, V., Mejia, A., Simmons, H. A., et al. (2019). Colonic inflammation affects myenteric alpha-synuclein in nonhuman primates. J. Inflamm. Res. 12, 113–126. doi: 10.2147/jir.S196552
Rza̧p, D., Czajkowska, M., and Całka, J. (2020). Neurochemical plasticity of nNOS-, VIP- and CART-immunoreactive neurons following prolonged acetylsalicylic acid supplementation in the porcine jejunum. Int. J. Mol. Sci. 21:2157. doi: 10.3390/ijms21062157
Shanan, N., GhasemiGharagoz, A., Abdel-Kader, R., and Breitinger, H. G. (2019). The effect of pyrroloquinoline quinone and resveratrol on the survival and regeneration of cerebellar granular neurons. Neurosci. Lett. 694, 192–197. doi: 10.1016/j.neulet.2018.12.002
Turner, J. R. (2009). Intestinal mucosal barrier function in health and disease. Nat. Rev. Immunol. 9, 799–809. doi: 10.1038/nri2653
Euler, U. S. V., and Gaddum, J. H. (1931). An unidentified depressor substance in certain tissue extracts. J. Physiol. 72, 74–87. doi: 10.1113/jphysiol.1931.sp002763
Wang, B., Wu, G., Zhou, Z., Dai, Z., Sun, Y., Ji, Y., et al. (2015). Glutamine and intestinal barrier function. Amino Acids 47, 2143–2154. doi: 10.1007/s00726-014-1773-4
Wu, X., Li, J., Zhang, H., Wang, H., Yin, G., and Miao, D. (2017). Pyrroloquinoline quinone prevents testosterone deficiency-induced osteoporosis by stimulating osteoblastic bone formation and inhibiting osteoclastic bone resorption. Am. J. Transl. Res. 9, 1230–1242.
Xu, T., Yang, X., Wu, C., Qiu, J., Fang, Q., Wang, L., et al. (2018). Pyrroloquinoline quinone attenuates cachexia-induced muscle atrophy via suppression of reactive oxygen species. J. Thoracic Dis. 10, 2752–2759. doi: 10.21037/jtd.2018.04.112
Yin, X., Ming, D., Bai, L., Wu, F., Liu, H., Chen, Y., et al. (2019). Effects of pyrroloquinoline quinone supplementation on growth performance and small intestine characteristics in weaned pigs. J. Anim. Sci. 97, 246–256. doi: 10.1093/jas/sky387
Yoshioka, N., Taniguchi, Y., Yoshida, A., Nakata, K., Nishizawa, T., Inagawa, H., et al. (2009). Intestinal macrophages involved in the homeostasis of the intestine have the potential for responding to LPS. Anticancer Res. 29, 4861–4865.
Zani, A., Cordischi, L., Cananzi, M., De Coppi, P., Smith, V. V., Eaton, S., et al. (2008). Assessment of a neonatal rat model of necrotizing enterocolitis. Eur. J. Pediatr. Surg. 18, 423–426. doi: 10.1055/s-2008-1038951
Zhang, B., Yang, W., Zhang, H., He, S., Meng, Q., Chen, Z., et al. (2019). Effect of pyrroloquinoline quinone disodium in female rats during gestating and lactating on reproductive performance and the intestinal barrier functions in the progeny. Br. J. Nutr. 121, 818–830. doi: 10.1017/s0007114519000047
Zhou, W., Ramachandran, D., Mansouri, A., and Dailey, M. J. (2018). Glucose stimulates intestinal epithelial crypt proliferation by modulating cellular energy metabolism. J. Cell Physiol. 233, 3465–3475. doi: 10.1002/jcp.26199
Zhou, X. Q., Yao, Z. W., Peng, Y., Mao, S. S., Xu, D., Qin, X. F., et al. (2018). PQQ ameliorates D-galactose induced cognitive impairments by reducing glutamate neurotoxicity via the GSK-3beta/Akt signaling pathway in mouse. Sci. Rep. 8:8894. doi: 10.1038/s41598-018-26962-9
Zhou, X., Cai, G., Mao, S., Xu, D., Xu, X., Zhang, R., et al. (2020). Modulating NMDA receptors to treat MK-801-induced schizophrenic cognition deficit: effects of clozapine combining with PQQ treatment and possible mechanisms of action. BMC Psychiatry 20:106. doi: 10.1186/s12888-020-02509-z
Keywords: pyrroloquinoline quinone, enteric nervous system, neurochemical plasticity, Akt signaling pathway, enteritis rats
Citation: Shi C, Xu S, Huang C, Wang Z, Wang W, Ming D, Yin X, Liu H and Wang F (2022) Pyrroloquinoline Quinone Regulates Enteric Neurochemical Plasticity of Weaned Rats Challenged With Lipopolysaccharide. Front. Neurosci. 16:878541. doi: 10.3389/fnins.2022.878541
Received: 18 February 2022; Accepted: 15 March 2022;
Published: 03 May 2022.
Edited by:
Chunhui Bao, Shanghai University of Traditional Chinese Medicine, ChinaReviewed by:
Katarzyna Palus, University of Warmia and Mazury in Olsztyn, PolandYulan Liu, Wuhan Polytechnic University, China
Copyright © 2022 Shi, Xu, Huang, Wang, Wang, Ming, Yin, Liu and Wang. This is an open-access article distributed under the terms of the Creative Commons Attribution License (CC BY). The use, distribution or reproduction in other forums is permitted, provided the original author(s) and the copyright owner(s) are credited and that the original publication in this journal is cited, in accordance with accepted academic practice. No use, distribution or reproduction is permitted which does not comply with these terms.
*Correspondence: Fenglai Wang, d2FuZ2ZsQGNhdS5lZHUuY24=