- 1University of Montpellier, Montpellier, France
- 2Department of Psychology, McGill University, Montreal, QC, Canada
- 3Department of Geriatrics, CHRU of Montpellier, Montpellier, France
- 4Clinical Investigation Centre, CHRU of Montpellier, Montpellier, France
- 5Aix Marseille Univ., CNRS, LPL, Aix-en-Provence, France
- 6Department of Neurology, Beau Soleil Clinic, Montpellier, France
- 7International Laboratory for Brain, Music and Sound Research (BRAMS), Montreal, QC, Canada
- 8Department of Psychology, University of Montreal, Montreal, QC, Canada
- 9Centre for Research on Brain, Language and Music (CRBLM), Montreal, QC, Canada
- 10University of Economics and Human Sciences in Warsaw, Warsaw, Poland
Rhythm disorders are consistently reported in Parkinson’s disease (PD). They manifest across motor domains, such as in orofacial (oral diadochokinesis), manual (finger tapping), and gait tasks. It is still unclear, however, whether these disorders are domain- and task-specific, or result from impaired common mechanisms supporting rhythm processing (general dysrhythmia). We tested the possibility that an at-home intervention delivered via a rhythmic video game on tablet improves motor performance across motor domains in PD. Patients with PD (n = 12) played at home a rhythmic video game (Rhythm Workers) on tablet, in which they finger-tapped to the beat of music, for 6 weeks. A control group (n = 11) played an active non-rhythmic video game (Tetris). A third group (n = 10) did not receive any intervention. We measured rhythmic abilities in orofacial, manual and gait motor domains, as well as rhythm perception, before and after the intervention. Patients who performed the rhythmic training improved their orofacial and manual rhythmic performance. This beneficial effect was linked to improved rhythm perception only following the rhythmic training period. We did not observe any improvement in rhythmic abilities in the other two groups. In this pilot study, we demonstrated that at-home intervention with a rhythmic video game using finger tapping can have beneficial effects on motor performance across different motor domains (manual and orofacial). This finding provides evidence of a general dysrhythmia in PD and paves the way to technology-driven interventions aiming at alleviating rhythm-related motor deficits in PD.
Introduction
Beyond cardinal motor symptoms of Parkinson’s disease (PD), severe rhythm deficits encompassing different motor domains are consistently reported, potentially representing an early marker of the disease (Cochen De Cock et al., 2020). These deficits manifest in heightened rhythmic variability of upper-limb, orofacial, and gait coordination (Skodda et al., 2010; Dalla Bella et al., 2017; Lowit et al., 2018). Rhythm deficits are not confined to motor tasks. They also extend to rhythm perception, such as detecting a beat in a rhythmic auditory sequence in the absence of motor output (Grahn and Brett, 2009; Benoit et al., 2014). These disorders of rhythm production across motor effectors and poor beat perception are highly correlated in PD (Puyjarinet et al., 2019). A parsimonious explanation of rhythm deficits in PD is referred to as general dysrhythmia or unique dysrhythmia (Cantiniaux et al., 2010; Skodda et al., 2010; Tolleson et al., 2015). According to this hypothesis rhythm perception and production disorders in PD would stem from the impairment of common brain mechanisms underpinning rhythm perception and production. These mechanisms include distributed neuronal networks involving both sub-cortical areas (the basal ganglia and the cerebellum) and cortical regions (e.g., the SMA) (Repp and Su, 2013; Cannon and Patel, 2021). Impairment of such basal-ganglia-cortical networks is consistent with the pathophysiology of PD (Factor and Weiner, 2008; Wichmann, 2019).
Though appealing, the hypothesis of general dysrhythmia in PD was tested only in a handful of correlational studies. For example, it is known that gait and speech rhythmic features (walking cadence and speech rhythmicity) are tightly linked (Cantiniaux et al., 2010), and that motor rhythmic variability in PD is closely related across different effectors—upper and lower limbs (finger tapping, gait), and the orofacial system (oral diadochokinesis) (Puyjarinet et al., 2019). Most importantly, we demonstrated that the severity of rhythm deficits across such motor domains can be predicted by the degradation of rhythm perception (Puyjarinet et al., 2019). This intriguing finding confirmed the impairment of a central mechanism devoted to rhythm processing, independent of motor control per se. Nevertheless, it is still unknown whether a general rhythm impairment plays a causal role in motor and perceptual rhythm deficits in PD.
We examined this possibility in the present pilot study by testing whether the beneficial effects of a rhythm-based intervention on a given effector (i.e., manual) do transfer to other effectors (orofacial, gait). Interventions targeting rhythmic skills can take different forms, such as rhythmic auditory cueing (Ghai et al., 2018; Dalla Bella, 2020), full-body physical activities (e.g., dance, Hackney and Earhart, 2009; Hsu et al., 2022), or serious games (Bégel et al., 2017, 2018). A serious game is a video game combining serious intentions, such as educational, professional or medical purposes, with pleasant and recreational aspects (Rego et al., 2010, 2014; Marsh, 2011). The advantage of serious video games is being designed for targeting specific functions such as attentional/executive, motor, or rhythmic abilities (Wiemeyer and Kliem, 2012; Bégel et al., 2018). In this study, we trained auditory-motor rhythmic skills via finger tapping in a group of PD patients with a dedicated serious video game we developed (Rhythm Workers, Bégel et al., 2018). This serious video game was validated in PD in a previous study (Dauvergne C. et al., 2018). As controls, we trained a second group of patients with a non-rhythmic classic video game (Tetris, Green and Bavelier, 2003; Nouchi et al., 2012), while a third group received no intervention. To test the effect of the type of training, we compared patients’ motor performance in the orofacial, manual, and gait domains, and their rhythm perception before and after intervention.
Materials and Methods
Patients
Thirty-three PD patients (6 women; mean age: 68.40 ± 7.32 years; age range: 50–82), participated in the experiment. PD was diagnosed from 2 to 25 years prior to the experiment (mean duration: 10.13 ± 5.69 years) in accordance with the UK Brain Bank criteria (Gibb and Lees, 1988). Patients were under optimal medication (60–90 min after their usual morning dose), which was stable for at least 4 weeks prior to the start of the experiment. This study was approved by the appropriate Committee (Comité de Protection des Personnes, Centre Hospitalier Universtaire, Montpellier: CPP N°2015-A01090-49). All participants signed an informed consent in accordance with the Helsinki Declaration (World Medical Association, 2013). Motor, perceptual, demographic, clinical characteristics, and cognitive status are provided in Table 1.
Experimental Design
In the absence of previous studies using rhythmic training in patients with PD using serious video games, we calculated the minimal sample size for our pilot study based on a previous study run in our laboratory using standard auditory cueing (Benoit et al., 2014; PD patients, n = 15; controls, n = 10). In that study patients with PD were submitted to a 1-month rhythmic training with auditory cueing, leading to beneficial effects on rhythm perception and performance, as well as gait. PD patients were randomly assigned to one of the three experimental arms: (i) Rhythm Workers (rhythm training group), (ii) Tetris (non-rhythm control training group), and (iii) no intervention group. Assignment to the groups was achieved using the free online application Group Maker (available athttps://livecloud.online/en/group-maker) that enables generating sequences of random numbers. The patients were instructed to play the games (Rhythm Workers or Tetris) using a tablet device (LG© G Pad 8.0 model) for at least 30 min, 4 times a week, during a period of 6 weeks ± 2 days. For checking whether participants followed these instructions, the number and duration of training sessions, as well as the performance with each of the games were automatically logged on the tablet at each session to be further compared between training types. Patients who played with Rhythm Workers were asked to finger tap to the beat of auditory rhythmic stimuli of variable complexity (metronome and musical excerpts) (Bégel et al., 2018; Dauvergne C. et al., 2018). The alignment between finger taps and the beat of rhythmic stimuli served to provide a feedback on the performance, and to progress through difficulty levels: the greater the synchronization of the taps to the beat, the higher the score, then allowing to move on to the next difficulty level. Patients in the Tetris group were required to play this classic game as an active control condition (Green and Bavelier, 2003; Nouchi et al., 2012). No specific, active, involvement was asked to the patients of the third group. A [post-pre]intervention design was used to assess orofacial, gait, manual, and perceptual rhythmic performance in the three groups.
Assessment of Rhythmic Skills
We tested rhythmic abilities with widely used orofacial, manual, gait and perceptual rhythmic tasks. To test orofacial rhythmic ability the patients performed an oral diadochokinesis task (i.e., oral sequential motion rate, namely the repetition of the tri-syllable pseudoword pataka for 30 s, at fast rate). We recorded the patients’ productions with a digital recorder (Zoom H4SP©). Audio files were pre-processed and analyzed using Praat software (Boersma and van Heuven, 2001). We assessed manual and perceptual rhythmic skills using the Battery for the Assessment of Auditory Sensorimotor and Timing Abilities (BAASTA, Dalla Bella et al., 2017). The patients performed two manual rhythmic tasks from BAASTA: unpaced and paced finger tapping tests. In unpaced tapping, the patients’ task was to finger tap regularly at a comfortable rate for 60 s in the absence of a pacing stimulus, while maintaining the tapping rate as constant as possible. In paced tapping, the patients had to synchronize their taps to the beat of a musical excerpt. In the rhythm perception task (Beat Alignment Test—BAT, Iversen and Patel, 2008; Dalla Bella et al., 2017), the patients had to judge whether a sequence of tones was aligned or not with the beat of short musical excerpts. BAASTA tests were administered using a tablet device (LG© G Pad 8.0 model), and auditory stimuli were delivered over headphones (Sennheiser© HD201). Finally, we tested gait rhythmicity by asking patients to walk along a computerized walkway (GAITRite© 301 system), at their preferred rate, for a distance of 8 m. To avoid variability (accelerations and decelerations) at the onset of the gait trial, participants started walking 2 m before the starting edge of the walkway and continued walking 2 m after the end of the walkway. Patients performed this task three times, and data were averaged. We have studied and described more extensively these tasks elsewhere (Dauvergne C. et al., 2018; Puyjarinet et al., 2019; Cuartero M. C. et al., 2021).
Variables and Analyses
We measured patients’ rhythmic performance by computing the variability, namely the standard deviation (SD) of inter-event intervals in the rhythmic tasks: inter-vowel intervals (IVIs) for orofacial rhythmic variability; inter-tap intervals (ITIs) for manual rhythmic variability; and stride time intervals (STIs) for gait rhythmic variability (see Puyjarinet et al., 2019 for details). IVIs, ITIs, and STIs variabilities account for the quality of motor coordination in each rhythmic domain, with higher scores indicating worse performances (greater variability or lower regularity).
For assessing rhythm perception, we calculated a beat perception score obtained in the rhythm perception task (d-prime, Dalla Bella et al., 2017). The higher the score, the better the performance. For both intervention groups (i.e., Rhythm Workers and Tetris groups), data were converted into Z-scores relatively to the performance of the no intervention group.
To test the effect of interventions on rhythmic variability across the different motor domains, we used mixed-design ANOVAs to compare the performance of the Rhythm Workers and Tetris groups, where Session (i.e., pre- vs. post-intervention) was the within-subject factor, and Group (Tetris vs. Rhythm Workers) the between-subject factor. We applied the Holm correction for multiple comparisons. We further assessed whether demographic, clinical, and cognitive variables would influence rhythmic performance by successively including them as covariates in the ANOVAs. In addition, to compare the effects of interventions on motor rhythmic performances with those on rhythm perception, we calculated [post-pre]intervention difference scores. We compared the two groups with a one-tailed Welch’s t-test. Moreover, one-tailed Pearson correlations between [post-pre]intervention difference scores for motor and perceptual tests were calculated. Most data were normally distributed according to the Shapiro–Wilk test, and whenever it was not the case, non-parametric tests were used. Statistics were computed using JASP software (JASP Team, 2016). All significant effects were set at P ≤ 0.05.
Results
Descriptive statistics for rhythmic performance assessed pre- and post-intervention are summarized in Table 2 and the effects of interventions on rhythmic performance are presented in Figure 1. Note that the three groups were comparable in terms of motor and perceptual rhythmic performance at baseline (all Ps > 0.19 when comparing the three groups’ performance with ANOVAs). On average the patients that trained with Tetris spent more time in playing the game (mean = 21.3 h, range = 1.39–44.32 h) than patients trained with Rhythm Workers (mean = 4.8 h; range = 1.07–15.07 h) (W = 18, P < 0.01; rank-biserial correlation = –0.72).
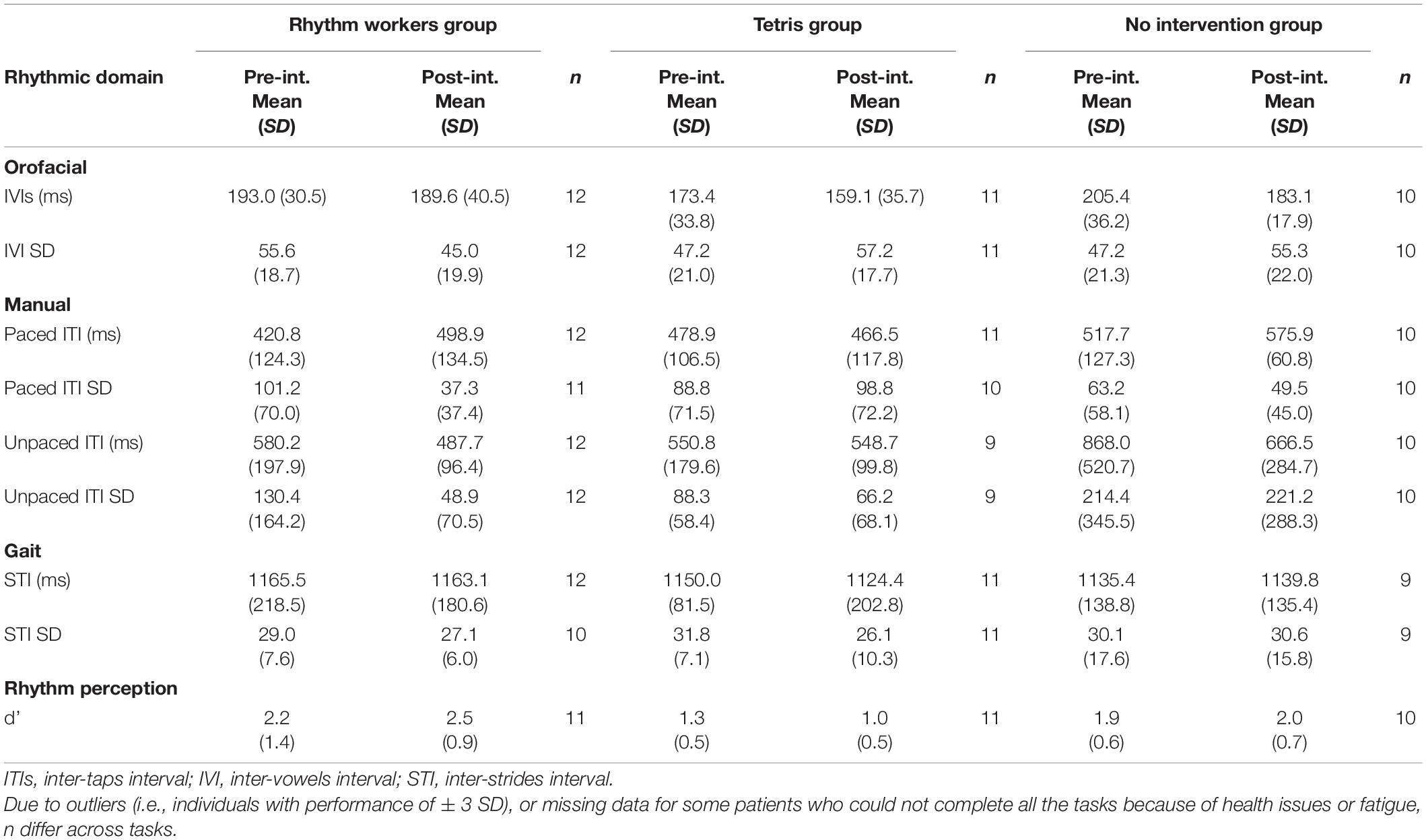
Table 2. Rhythm performance for PD patients in the two experimental groups (Rhythm Workers and Tetris) and in the no intervention group.
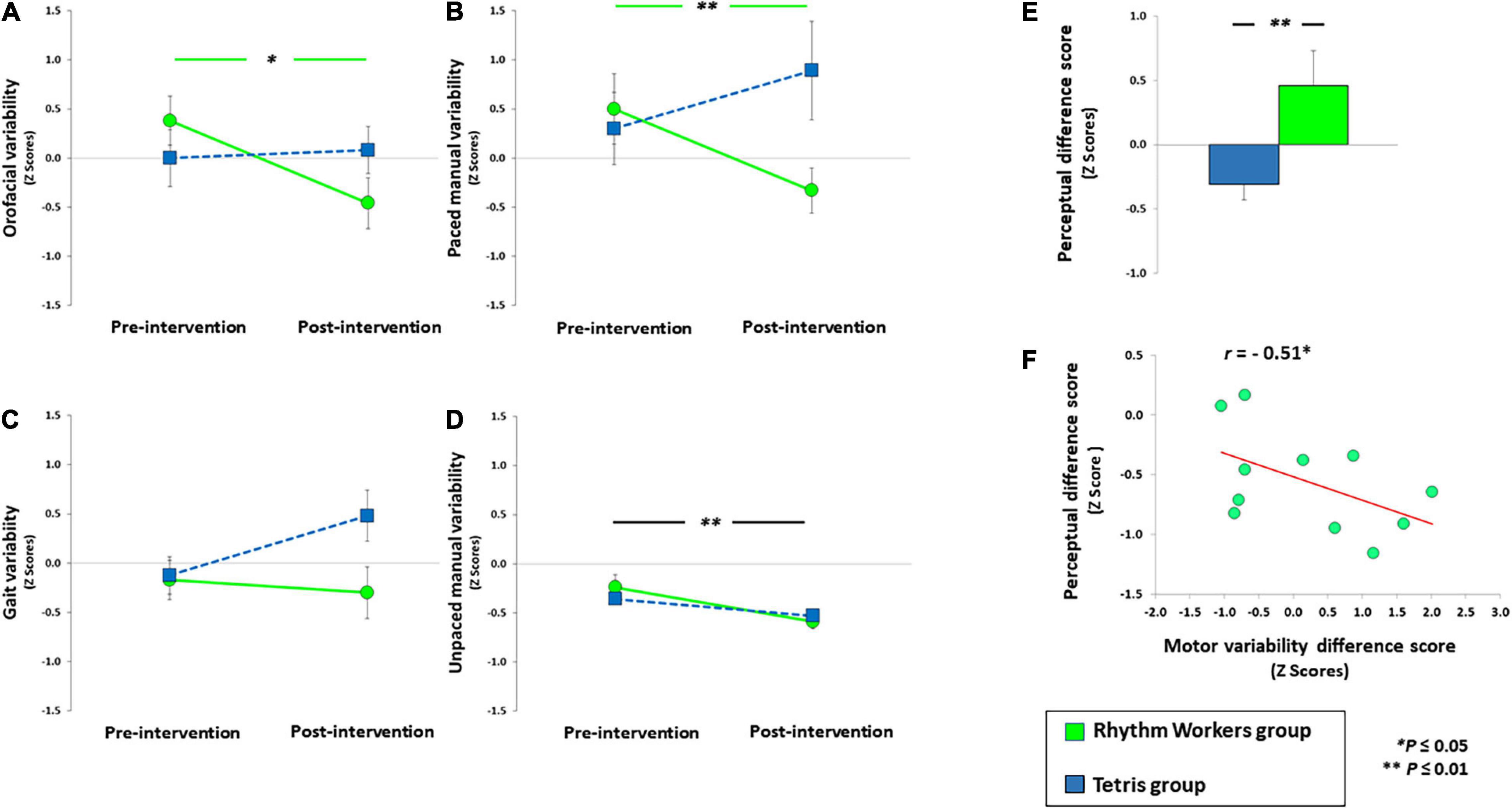
Figure 1. Performance of patients playing Rhythm Workers and Tetris in the rhythmic oral diadochokinesis (A), paced manual (B), gait (C), and unpaced manual (D) tasks. (E,F) The post- minus pre-intervention difference score in the rhythm perception task, for each group. A positive difference score indicates an improvement of rhythm perception following the intervention. (F) The relation between an improvement in rhythm perception (perceptual difference score) and the change in rhythmic variability post- minus pre-intervention averaged across motor domains (post-motor variability difference score) for the Rhythm Workers group. A negative motor variability difference score indicates an improvement.
Rhythmic Motor Performance
Both ANOVAs on orofacial and paced manual rhythmic variability showed a Session x Group interaction [F(1,21) = 6.04, P = 0.02, η2 = 0.19; Figure 1A; F(1,21) = 5.74, P = 0.02, η2 = 0.23; Figure 1B]. Patients in the Rhythm Workers group showed lower orofacial rhythmic variability [t(11) = 2.55, P = 0.01, d = 0.73] and lower manual tapping variability [t(11) = 2.50, P = 0.01, d = 0.75] after the intervention than before. No effect of intervention was found in the Tetris group on orofacial (P = 0.70) and manual rhythmic variability (P = 0.86). Both Tetris and Rhythm Workers reduced the unpaced manual rhythmic variability [Session effect: F(1,19) = 13.67, P < 0.01, η2 = 0.39; Figure 1D]. In the same task, there was neither a Session × Group interaction, nor a main effect of Group (Ps > 0.21). When considering the effect of rhythmic training on gait rhythmic variability, the main effects of Session and Group were not significant (Ps > 0.12), and the Session x Group interaction failed to reach significance [F(1,19) = 3.55, P = 0.07, η2 = 0.14; Figure 1C]. Patients in the group without intervention did not show any change in their rhythmic variabilities, whatever the motor domain and the time of testing (all Ps > 0.32 in orofacial, manual, and gait domains).
Rhythm Perception, Correlation With Motor Performance, and Clinical Tests
The two interventions differed in their effect on rhythm perception [t(15.63) = 2.62, P < 0.01, d = 1.09] (Figure 1E). Playing with Rhythm Workers improved patients’ rhythm perception (Z = 1.62, P = 0.05, d = 0.46), unlike playing with Tetris (W = –1.08, P = 0.14). The reduction of rhythmic variability in the motor domains, whatever the effector, was linked to an improvement in rhythm perception in the Rhythm Workers group only (r = –0.51; P = 0.05; Figure 1F), whereas it was not the case in the Tetris group (r = 0.36; P = 0.84). Considering the age, disease duration, MDS-UPDRS total and motor scores, Hoehn and Yahr stage, and MoCA score as covariates in the aforementioned ANOVAs did not modify the results relative to orofacial, manual (paced and unpaced) and gait rhythmic variability (Session x Group interactions remained significant in orofacial and manual domains, with Ps < 0.05, and all Ps = n.s. in the gait domain).
Clinical Findings
For the MDS-UPDRS motor subscore (part 3), the interaction between the effect and type of intervention failed to reach significance [Session × Group interaction: F(1,21) = 3.51, P = 0.07, η2 = 0.12]. Due to the marginally significant interaction, we ran further analyses showing overall less severe motor symptoms, as showed by lower MDS-UPDRS motor subscores (part III), in patients who played Rhythm Workers [t(11) = 2.57, P = 0.02, d = 0.74] relative to their performance pre-intervention. We found no such difference in the Tetris patient group (P = 0.96). We did not find similar trends for MDS-UPDRS specific items related to speech (3.1), upper (3.4a–3.6b) and lower (3.7a–3.8b) limbs, for none of the patient groups.
Discussion
In this pilot study, we provide first evidence that an at-home intervention with a rhythmic video game improves rhythm performance (i.e., it reduces motor variability) in PD patients, when evaluating both manual and orofacial motor tasks. We do not observe any improvement with a comparable non-rhythmic video game (i.e., Tetris group), and in absence of training (i.e., no intervention group). These effects persist after controlling for patients’ clinical characteristics such as severity of motor symptoms, disease duration, and cognitive functioning. Critically, we demonstrate that the motor improvement is linked to enhanced rhythm perception resulting from the rhythmic training. The effect of rhythmic training is even more surprising considering that patients played the rhythmic video game less time than the non-rhythmic one. Overall, we provide first causal evidence in support of a general dysrhythmia in PD, in keeping with our previous work (Puyjarinet et al., 2019). Showing that the beneficial effects of rhythmic training can transfer from one effector to the others paves the way to innovative clinical applications.
Which cerebral mechanisms are likely to underpin the observed transfer effect of rhythmic training across motor domains? A pivotal notion in explaining this finding is the concept of predictive timing, namely the ability to predict accurately the time of occurrence of an upcoming event (e.g., the next syllable, or the next step), based on the regular temporal structure of a sequence (Large and Jones, 1999; Piras and Coull, 2011; Schwartze and Kotz, 2013). The mechanisms underlying predictive timing engage distributed subcortico-cortical pathways, including the basal ganglia, the cerebellum, the supplementary motor area, and parietal and dorsal-premotor cortices (Coull and Nobre, 2008; Kotz and Schwartze, 2011; Piras and Coull, 2011; Schwartze and Kotz, 2013). Rhythmic interventions are likely to engage these networks, such as in gait rehabilitation via rhythmic auditory cueing (Dalla Bella, 2020), which is also found to improve PD patients’ rhythm performance both in motor and perceptual tasks (Benoit et al., 2014; Ghai et al., 2018). This form of auditory rhythm-based intervention is likely to capitalize on relatively spared predictive timing (Dalla Bella et al., 2017; Dalla Bella, 2018), which would improve as a result of the training (as shown in a rhythm perception task), and drive beneficial effects across different motor effectors (Puyjarinet et al., 2019; Dalla Bella, 2020). This hypothesis is in keeping with the results of a previous study (Puyjarinet et al., 2019) showing that motor variability in PD is correlated across motor domains and can be predicted by the performance in a beat perception task, thus irrespective of the specific motor output. The causal evidence that rhythm perception can be improved selectively by rhythmic training, as opposed to training in a non-rhythmic game, and that this improvement is related to reduced motor variability across domains indicates that the beneficial effects of rhythmic training may be driven by a central predictive mechanism relatively independent of the motor output.
Our results do not show improvement in rhythmic performance tested in a gait task after the intervention. This finding may be surprising considering that rhythm-based interventions are supposed to improve gait parameters in PD, notably speed and stride length (for reviews, Ghai et al., 2018; Janzen et al., 2019; Dalla Bella, 2020). Unlike gait cadence (Janzen et al., 2019), rhythm variability assessed in the current study appeared to be a variable less sensitive to such intervention in gait. Nevertheless, rhythm variability was the most appropriate measure to consider for cross-domain (oral, manual, and gait) comparison. We could compute the same measures of timing for motor events and their variability across domains, and we already showed that these measures are correlated in PD (Puyjarinet et al., 2019). Another possibility to explain the lack of improvement on gait rhythmic variability following the rhythmic training may be the explicit nature of the rhythmic game. The game targets sensorimotor synchronization, namely an explicit timing task with the goal of producing finger taps aligned to the beat of musical sequences. Explicit timing tasks rely on basal-ganglia-cortical networks, and can be considered as a form of “cognitive controlled timing.” Conversely, spontaneous gait tested in the present study may rely more on “implicit timing” mechanisms, where temporal information is not targeted by the task per se, and can be used to optimize performance in non-temporal tasks (Coull and Nobre, 2008; Avanzino et al., 2016). Implicit timing critically relies on cerebello-thalamo-cortical pathways, with parietal cortex involvement (Lewis and Miall, 2003; Avanzino et al., 2016). This dissociation between explicit and implicit timing neural mechanisms could at least partially account for difference between the effect of our intervention on rhythm variability in gait, oral and manual tasks. Our findings suggest that training in an explicit timing task using finger tapping may not transfer to spontaneous gait rhythmic variability. Future studies should compare the effects of training timing abilities using an implicit task vs. an explicit task on gait.
Other explanations may rely on the nature of the video game training that does not require the participants to walk. Finger tapping may have not been sufficient to observe cross-effector beneficial effects for gait, while walking in real condition to the rhythm of metronome or music beat is known to induce cross-effector effects including spontaneous gait, at least for spatio-temporal gait features such as speed and stride length (Janzen et al., 2019). In the future, it would be interesting to increase the duration of the rhythmic training or its intensity, as more than 6 weeks of practice may be needed to induce beneficial effects in gait (i.e., reduce rhythm variability).
We also found a tendency of rhythmic training to improve the global motor state of patients, as assessed by the MDS-UPDRS. If confirmed, this tendency could have important clinical consequences. A relevant example of clinical application would be to use rhythmic video games in the context of a motor rehabilitation program. Serious games are an evolving field demonstrating already promising results for healthcare (Rego et al., 2014), and in motor rehabilitation in PD (Perrochon et al., 2019). Using games like Rhythm Workers holds a lot of promise for devising at-home, auto-rehabilitation programs widely accessible and low-cost. Many advantages can be associated with the practice of such serious games, among which the motivating and funny nature of the training. Feedback from patients who played with Rhythm Workers mainly concerned the challenging and playful aspects of the game. Another advantage of training at home is that there are no constraints associated to traveling to rehabilitation centers (e.g., travel time, fatigue). Several times a week, the patient can practice independently, in a safe environment, under the supervision of a therapist who can provide a personalized program at distance. Integrating rhythmic training sessions via serious game could complement the effects of more traditional rehabilitations while avoiding muscular fatigue due to repetitive physical effort (e.g., gait rehabilitation programs for preventing the risk of falling) (Hausdorff et al., 2001). The regular practice of a rhythmic video game at home may alleviate symptoms such as altered orofacial coordination—which typically manifests in PD hypokinetic dysarthria (Atkinson-Clement et al., 2015; Lowit et al., 2018).
Yet, there are still challenges that will motivate further research. Notably, adherence to the program is central to the success of an intervention (Perrochon et al., 2019), and even more important in serious video games than in standard clinical interventions, owing to the paucity of guidelines. Thus, adherence of the specific serious video game protocol should be evaluated on a case-by-case basis. In the present experiment, and despite PD patients benefited from the rhythmic training, about 60% of them were far from reaching the required playing time. The fact that some players did not reach the minimum time required could be explained by reasons independent of the characteristics of the game. Several patients showed cognitive deficits associated with PD, which may have induced fatigue and may explain their reduced adherence to the intervention. These issues will have to be considered in future studies to increase patients’ adherence to the rhythmic training game. For example, an individualized approach adapting the game features (e.g., its level of difficulty) to the patient’s spared cognitive capacities and rhythmic abilities (Dalla Bella et al., 2018; Dotov et al., 2019; Dalla Bella, 2020) is likely to increase adherence to the intervention. Complementary to this approach is the assessment of rhythmic abilities prior to the intervention, so as to identify the patients who are the most likely to benefit from the intervention, and those who may experience adverse effects (Cochen De Cock et al., 2018). Finally, one of the advantages related to the current health context (i.e., the COVID-19 epidemic) is to allow patients to take part in the rehabilitation program remotely without contact with other people, thus limiting the risk of infection. In sum, there are several points in favor of using rhythmic serious video games in home-based therapeutic practice, in addition to more traditional approaches. One of the limitations of our study is the small sample size, which is typical of pilot studies. In spite of this limitation, the results were very promising, and the observed effect sizes were moderate to large. The pilot study will pave the way to a randomized clinical trial in which a larger sample of patients will be considered. Second, in our study we measured the improvement of the rhythmic variability due to the intervention in behavioral tests, while lacking an investigation of the underlying brain changes and involved neuronal mechanisms. Our planned future investigation will include an assessment of structural and functional brain changes before and after the intervention, in order to highlight the contribution of the perceptual and motor regulation loops involved in rhythmic performance. Longitudinal studies with follow-up assessments on larger cohorts of patients will help also to investigate potential long-term effects of rhythmic training via serious video games and their effect on social activities (e.g., conversational skills, daily commuting).
To conclude, we demonstrated in this pilot study that an at-home intervention especially designed for training rhythmic skills with a serious video game improves rhythmic performance in PD, in both manual and orofacial domains, as well as rhythm perception. Therefore, serious games played at home may present a highly promising and cost-effective solution for complementing more traditional motor rehabilitation protocols for PD patients. Finally, from a more clinical point of view, our study provides additional arguments for considering dysrhythmia as an important feature to be more systematically assessed in PD.
Data Availability Statement
The raw data supporting the conclusions of this article will be made available by the authors, without undue reservation.
Ethics Statement
The studies involving human participants were reviewed and approved by the Comité de Protection des Personnes Sud-Méditerranée I Marseille, France (CPP N°2015-A01090-49). The patients/participants provided their written informed consent to participate in this study.
Author Contributions
FP, VB, CG, VD, M-CC, VCD, SP, and SD substantially contributed to the design of the experiment, revised the work, approved the completed version, and addressed the questions related to the accuracy and integrity of the work. FP, VB, CG, and SD conducted the experiments. FP and SD analyzed the results. FP, SP, and SD contributed to writing the manuscript. All authors contributed to the article and approved the submitted version.
Funding
This research was funded by the Junior Grant to the Institut Universitaire de France (IUF) to SDB, and the Maturation Technological Transfer grant (YouRhythm project) from SATT AxLR to SDB and the company NaturalPad. The funders had no role in study design, data collection or analysis, decision to publish, or manuscript preparation.
Conflict of Interest
The authors declare that the research was conducted in the absence of any commercial or financial relationships that could be construed as a potential conflict of interest.
Publisher’s Note
All claims expressed in this article are solely those of the authors and do not necessarily represent those of their affiliated organizations, or those of the publisher, the editors and the reviewers. Any product that may be evaluated in this article, or claim that may be made by its manufacturer, is not guaranteed or endorsed by the publisher.
References
Atkinson-Clement, C., Sadat, J., and Pinto, S. (2015). Behavioral treatments for speech in Parkinson’s disease: meta-analyses and review of the literature. Neurodegener. Dis. Manag. 5, 233–248. doi: 10.2217/NMT.15.16
Avanzino, L., Pelosin, E., Vicario, C. M., Lagravinese, G., Abbruzzese, G., Martino, D., et al. (2016). Time processing and motor control in movement disorders. Front. Hum. Neurosci. 10:631. doi: 10.3389/fnhum.2016.00631
Bégel, V., Di Loreto, I., Seilles, A., and Dalla Bella, S. (2017). Music games: potential application and considerations for rhythmic training. Front. Hum. Neurosci. 11:273. doi: 10.3389/fnhum.2017.00273
Bégel, V., Seilles, A., and Dalla Bella, S. (2018). Rhythm Workers: a music-based serious game for training rhythm skills. Music Sci. 1, 1–16. doi: 10.1177/2059204318794369
Benoit, C. E., Dalla Bella, S., Farrugia, N., Obrig, H., Mainka, S., Kotz, S. A., et al. (2014). Musically cued gait-training improves both perceptual and motor timing in Parkinson’s disease. Front. Hum. Neurosci. 8:494. doi: 10.3389/fnhum.2014.00494
Cannon, J. J., and Patel, A. D. (2021). How beat perception co-opts motor neurophysiology. Trends Cogn. Sci. 25, 137–150. doi: 10.1016/j.tics.2020.11.002
Cantiniaux, S., Vaugoyeau, M., Robert, D., Horrelou-Pitek, C., Mancini, J., Witjas, T., et al. (2010). Comparative analysis of gait and speech in Parkinson’s disease: hypokinetic or dysrhythmic disorders? J. Neurol. Neurosurg. Psychiatry 81, 177–184. doi: 10.1136/jnnp.2009.174375
Cochen De Cock, V, Dotov, D. G., Ihalainen, P, Bégel, V, Galtier, F., Lebrun, C., et al. (2018). Rhythmic abilities and musical training in Parkinson’s disease: do they help? npj Parkinson’s Dis. 4:8. doi: 10.1038/s41531-018-0043-7
Cochen De Cock, D., Picot, M. C., Damm, L., Abril, B., Galtier, F., et al. (2020). Rhythm disturbances as a potential early marker of Parkinson’s disease in idiopathic REM sleep behavior disorder. Ann. Clin. Transl. Neurol. 7, 280–287. doi: 10.1002/acn3.50982
Cuartero, M. C., and Bertrand, R, Rauzy, S, Véron-Delor, L, Atkinson-Clement, C, Grabli, D, et al. (2021). Acoustic, perceptual and clinical correlates of speech and voice in isolated dystonia: preliminary findings. Int. J. Lang. Commun. Disorders 56, 1204–1217. doi: 10.1111/1460-6984.12661
Coull, J. T., and Nobre, A. (2008). Dissociating explicit timing from temporal expectation with fMRI. Curr. Opin. Neurol. 18, 137–144. doi: 10.1016/j.conb.2008.07.011
Dalla Bella, S (2018). Music and movement: Towards a translational approach. Clin Neurophysiol 48, 377–386. doi: 10.1016/j.neucli.2018.10.067
Dalla Bella, S, Benoit, C. E., Farrugia, N., Keller, P. E., Obrig, H., Mainka, S., et al. (2017). Gait improvement via rhythmic stimulation in Parkinson’s disease is linked to rhythmic skills. Sci. Rep. 7:42005. doi: 10.1038/srep42005
Dalla Bella, S, Dotov, D., and Bardy, B. and Cochen de Cock, V. (2018). Individualization of music-based rhythmic auditory cueing in Parkinson’s disease. Ann. N.Y. Acad. Sci. 1423, 308–317. doi: 10.1111/nyas.13859
Dalla Bella, S. (2020). “The use of rhythm in rehabilitation for patients with movement disorders,” in Music and the Aging Brain, eds L. Cuddy, S. Belleville, and A. Moussard (Cambridge: Elsevier), 383–406.
Dalla Bella, S., Farrugia, N., Benoit, C. E., Begel, V., Verga, L., Harding, E., et al. (2017). BAASTA: Battery for the Assessment of Auditory Sensorimotor and Timing Abilities. Behav. Res. Met. 49, 1128–1145.
Dauvergne, C., Bégel, V., and Gény, C. (2018). Home-based training of rhythmic skills with a serious game in Parkinson’s disease: Usability and acceptability. Ann. Phys. Rehabil. Med. 61, 380-385. doi: 10.1016/j.rehab.2018.08.002
Dotov, D. G., Cochen de Cock, V., Geny, C., Ihalainen, P., Moens, B., Leman, M., Dalla Bella, S., et al. (2019). The role of mutual synchronization and predictability in entraining walking to an auditory beat. J. Exp. Psychol. 148, 1041–1057. doi: 10.1037/xge0000609
Factor, S. A., and Weiner, W. J. (2008). Parkinson’s Disease. Diagnosis and Clinical Management. New York: Demos Medical Publishing.
Ghai, S., Ghai, I., Schmitz, G., and Effenberg, A. O. (2018). Effect of rhythmic auditory cueing on parkinsonian gait: a systematic review and meta-analysis. Sci. Rep. 8:506. doi: 10.1038/s41598-017-16232-5
Gibb, W. R. G., and Lees, A. J. (1988). The relevance of the Lewy body to the pathogenesis of idiopathic Parkinson’s disease. J. Neurol. Neurosurg. Psychiatry 51, 745–752.
Goetz, C. G., Tilley, B. C., Shaftman, S. R., Stebbins, G. T., Fahn, S., Martinez-Martin, P., et al. (2008). Movement Disorder Society-sponsored revision of the Unified Parkinson’s Disease Rating Scale (MDS-UPDRS): scale presentation and clinimetric testing results. Mov. Dis. 23, 2129–2170. doi: 10.1002/mds.22340
Grahn, J. A., and Brett, M. (2009). Impairment of beat-based rhythm discrimination in Parkinson’s disease. Cortex 45, 54-61. doi: 10.1016/j.cortex.2008.01.005
Green, C. S., and Bavelier, D. (2003). Action video game modifies visual selective attention. Nature 423, 534–537.
Hackney, M. E., and Earhart, G. M. (2009). Effects of dance on movement control in Parkinson’s disease: a comparison of Argentine tango and American ballroom. J. Rehab. Med. 41, 475–481. doi: 10.2340/16501977-0362
Hausdorff, J. M., Rios, D. A., and Edelberg, H. K. (2001). Gait variability and fall risk in community-living older adults: a 1-year prospective study. Arch. Phys. Med. Rehabil. 82, 1050–1056. doi: 10.1053/apmr.2001.24893
Hsu, P., Ready, E. A., and Grahn, J. A. (2022). The effects of Parkinson’s disease, music training, and dance training on beat perception and production abilities. PLoS One 17:e0264587. doi: 10.1371/journal.pone.0264587
Iversen, J. R., and Patel, A. D. (2008). “The beat alignment test (BAT): surveying beat processing abilities in the general population,” in Proceedings of the 10th International Conference on Music Perception and Cognition (ICMPC 10), eds K. Miyazaki, Y. Hiraga, M. Adachi, Y. Nakajima, and M. Tsuzaki (Sapporo).
Janzen, T. B., Haase, M., and Thaut, M. H. (2019). Rhythmic priming across effector systems: a randomized controlled trial with Parkinson’s disease patients. Hum. Mov. Sci. 64, 355–365. doi: 10.1016/j.humov.2019.03.001
JASP Team. (2016). JASP, Version 0.9.2.0. https://jasp-stats.org [Accessed January 22, 2019].
Kotz, S. A., and Schwartze, M. (2011). Differential input of the supplementary motor area to a dedicated temporal processing network: functional and clinical implications. Front. Integr. Neurosci. 5:86. doi: 10.3389/fnint.2011.00086
Large, E. W., and Jones, M. R. (1999). The dynamics of attending: How people track time-varying events. Psychol. Rev. 106, 119–159.
Lewis, P. A., and Miall, R. C. (2003). Distinct systems for automatic and cognitively controlled time measurement: evidence from neuroimaging. Curr. Opin. Neurobiol. 13, 250–255. doi: 10.1016/s0959-4388(03)00036-9
Lowit, A., Marchetti, A., Corson, S., and Kuschmann, A. (2018). Rhythmic performance in hypokinetic dysarthria: Relationship between reading, spontaneous speech and diadochokinetic tasks. J. Commun. Disord. 72, 26–39. doi: 10.1016/j.jcomdis.2018.02.005
Marsh, T. (2011). Serious games continuum: Between games for purpose and experiential environments for purpose. Entertain. Comput. 2, 61–68. doi: 10.1016/j.entcom.2010.12.004
Nasreddine, Z. S., Phillips, N. A., Bédirian, V., Charbonneau, S., Whitehead, V., Collin, I., et al. (2005). The Montreal Cognitive Assessment, MoCA: a brief screening tool for mild cognitive impairment. J. Am. Geriat. Soc. 53, 695–699. doi: 10.1111/j.1532-5415.2005.53221.x
Nouchi, R., Taki, Y., Takeuchi, H., Hashizume, H., Akitsuki, Y., Shigemune, Y., et al. (2012). Brain training game improves executive functions and processing speed in the elderly: a randomized controlled trial. PloS One 7:e29676. doi: 10.1371/journal.pone.0029676
Perrochon, A., Borel, B., Istrate, D., Compagnat, M., and Daviet, J. C. (2019). Exercise-based games interventions at home in individuals with a neurological disease: A systematic review and meta-analysis. Ann. Phys. Rehab. Med. 62, 366–378. doi: 10.1016/j.rehab.2019.04.004
Piras, F., and Coull, J. T. (2011). Implicit, predictive timing draws upon the same scalar representation of time as explicit timing. PloS One 6:e18203. doi: 10.1371/journal.pone.0018203
Puyjarinet, F., Bégel, V., Gény, C., Driss, V., Cuartero, M. C., Kotz, S. A., et al. (2019). Heightened orofacial, manual, and gait variability in Parkinson’s disease results from a general rhythmic impairment. Parkinson’s Dis. 5:19. doi: 10.1038/s41531-019-0092-6
Rego, P., Moreira, P., and Reis, L. P. (2010). “Serious games for rehabilitiation: a survey and classification towards a taxonomy,” in Proceedings of the 5th Iberian Conference on Information Systems and Technologies, (Santiago de: IEEE), 1–6.
Rego, P., Moreira, P., and Reis, L. P. (2014). A serious games framework for health rehabilitation. Int. J. Healthc. Inf. Syst. Inform 9, 1–21. doi: 10.4018/ijhisi.2014070101
Repp, B. H., and Su, Y. H. (2013). Sensorimotor synchronization: a review of recent research (2006–2012). Psychon. Bull. Rev. 20, 403–452. doi: 10.3758/s13423-012-0371-2
Schwartze, M., and Kotz, S. A. (2013). A dual-pathway neural architecture for specific temporal prediction. Neurosci. Biobehav. Rev. 37, 2587–2596. doi: 10.1016/j.neubiorev.2013.08.005
Skodda, S., Flasskamp, A., and Schlegel, U. (2010). Instability of syllable repetition as a model for impaired motor processing: is Parkinson’s disease a « rhythm disorder »? J. Neural Transm 117, 605-612. doi: 10.1007/s00702-010-0390-y
Team, J. A. S. P. (2016). JASP, Version 0.9.2.0. Available onlie at https://jasp-stats.org [accessed January 22, 2019].
Tolleson, C. M., Dobolyi, D. G., Roman, O. C., Kanoff, K., Barton, S., Wylie, S. A., et al. (2015). Dysrhythmia of timed movements in Parkinson’s disease and freezing of gait. Brain Res. 1624, 222-231. doi: 10.1016/j.brainres.2015.07.041
Wichmann, T. (2019). Changing views of the pathophysiology of Parkinsonism. Mov. Disord. 34, 1130–1143. doi: 10.1002/mds.27741
Wiemeyer, J., and Kliem, A. (2012). Serious games in prevention and rehabilitation—a new panacea for elderly people? Eur. Rev. Aging Phys. Act. 9, 41–50.
Keywords: Parkinson’s disease, rhythmic training, music-based interventions, mobile technologies, serious video games
Citation: Puyjarinet F, Bégel V, Geny C, Driss V, Cuartero M-C, De Cock VC, Pinto S and Dalla Bella S (2022) At-Home Training With a Rhythmic Video Game for Improving Orofacial, Manual, and Gait Abilities in Parkinson’s Disease: A Pilot Study. Front. Neurosci. 16:874032. doi: 10.3389/fnins.2022.874032
Received: 11 February 2022; Accepted: 12 May 2022;
Published: 13 June 2022.
Edited by:
Diego Minciacchi, University of Florence, ItalyReviewed by:
Zhanhua Liang, The First Affiliated Hospital of Dalian Medical University, ChinaThenille Braun Janzen, Federal University of ABC, Brazil
Copyright © 2022 Puyjarinet, Bégel, Geny, Driss, Cuartero, De Cock, Pinto and Dalla Bella. This is an open-access article distributed under the terms of the Creative Commons Attribution License (CC BY). The use, distribution or reproduction in other forums is permitted, provided the original author(s) and the copyright owner(s) are credited and that the original publication in this journal is cited, in accordance with accepted academic practice. No use, distribution or reproduction is permitted which does not comply with these terms.
*Correspondence: Frédéric Puyjarinet, frederic.puyjarinet@umontpellier.fr; Simone Dalla Bella, simone.dalla.bella@umontreal.ca