- 1Department of Clinical Psychology, The Affiliated Brain Hospital of Guangzhou Medical University, Guangzhou, China
- 2Department of Radiology, The Affiliated Brain Hospital of Guangzhou Medical University, Guangzhou, China
Childhood trauma is a non-specific risk factor for major depressive disorder (MDD). resting-state functional magnetic resonance imaging (R-fMRI) studies have demonstrated changes in regional brain activity in patients with MDD who experienced childhood trauma. However, previous studies have mainly focused on static characteristics of regional brain activity. This study aimed to determine the specific brain regions associated with MDD with childhood trauma by performing temporal dynamic analysis of R-fMRI data in three groups of patients: patients with childhood trauma-associated MDD (n = 48), patients without childhood trauma-associated MDD (n = 30), and healthy controls (n = 103). Dynamics and concordance of R-fMRI indices were calculated and analyzed. In patients with childhood trauma-associated MDD, a lower dynamic amplitude of low-frequency fluctuations was found in the left lingual gyrus, whereas a lower dynamic degree of centrality was observed in the right lingual gyrus and right calcarine cortex. Patients with childhood trauma-associated MDD showed a lower voxel-wise concordance in the left middle temporal and bilateral calcarine cortices. Moreover, group differences (depressed or not) significantly moderated the relationship between voxel-wise concordance in the right calcarine cortex and childhood trauma history. Overall, patients with childhood trauma-associated MDD demonstrated aberrant variability and concordance in intrinsic brain activity. These aberrances may be an underlying neurobiological mechanism that explains MDD from the perspective of temporal dynamics.
Introduction
Major depressive disorder (MDD) is a common mental illness that affects over 350 million people. It is a heterogeneous clinical syndrome that can include symptoms of disturbed mood, difficulty concentrating, bodily complaints, self-loathing, delusions of guilt, indecision, and even a strong wish to die (de Kwaasteniet et al., 2013; McCarron et al., 2021). MDD is a major leading cause of disability and has an approximate 12-month prevalence of 6% worldwide (Kessler and Bromet, 2013). The onset and development of MDD is a complicated process and involves various factors, including genetic vulnerability (Howard et al., 2019), stressful life events and circumstances (Hammen, 2005; Southwick et al., 2005), dysfunctional cognition (Gotlib and Joormann, 2010; Figueroa et al., 2015), interpersonal dysfunction (Hammen and Brennan, 2002), female sex (Kuehner, 2017; Salk et al., 2017), and childhood trauma (Huh et al., 2017; Nelson et al., 2017).
Childhood trauma is a non-specific risk factor for MDD. Patients with childhood trauma-associated MDD have a worse treatment response (Nikkheslat et al., 2020). According to existing studies, among individuals with childhood trauma, 54% suffer from depression, 64% are addicted to illicit drugs, and 67% have experienced suicidal ideation (Dube et al., 2003). Childhood trauma consists of emotional, physical, and sexual abuse, and emotional and physical neglect (Danese and Baldwin, 2017), and has been closely associated with numerous psychiatric disorders such as MDD (Yu M. et al., 2019), bipolar disorder (Begemann et al., 2021), post-traumatic stress disorder (Kisely et al., 2018), and borderline personality disorder (Nicol et al., 2015). The neurobiological mechanisms underlying the association remain unclear. Yu M. et al. (2019) found that traumatic childhood experiences and dimensional symptoms are linked to aberrant network architecture in MDD, providing strong evidence for the negative impact of childhood trauma. Furthermore, Heim et al. (2008) and Du et al. (2016), observed an aberrant amplitude of low-frequency fluctuation (ALFF) and fractional amplitude of low-frequency fluctuation (fALFF) in patients with MDD across widespread brain regions relative to healthy controls, demonstrating that childhood trauma might lead to brain dysfunction and increased risk of MDD. Similarly, in a multimodal study, Duncan et al. (2015) found that childhood trauma causes long-term functional and structural effects in the brain.
Although previous studies have provided insights into the neurobiological mechanisms underlying MDD in patients who experienced childhood trauma, they did not examine variability and concordance in intrinsic brain activity. Brain activity fluctuates and changes over time in response to context and activity and underlies temporal-dynamic integration in the brain (Park et al., 2018). A number of studies have captured the temporal dynamic patterns of intrinsic brain activity using the sliding window method. Evidence has indicated that aberrant variability and concordance of resting-state functional magnetic resonance imaging (R-fMRI) indices are related to the mechanisms underlying MDD (Hutchison et al., 2013; Allen et al., 2014; Xue et al., 2020). Regarding aberrant variability, Zhao, Lei and colleagues reported significantly decreased dynamic ALFF (dALFF) in the emotion network in depressed patients (Zhao et al., 2021). Xue et al. (2020) observed a consistently decreased dynamic regional homogeneity (dReHo) in patients with MDD in both fusiform gyri, the right temporal pole, and the hippocampus relative to healthy controls. Additionally, Zhang et al. (2022) revealed the relationship between brain dynamic working patterns and chronic stress in adolescent MDD using the dynamic functional connectivity (FC) method. Zhu et al. (2020) reported abnormal cerebellar-cerebral dynamic FC changes in MDD. As for abnormal concordance, Zhu et al. (2019) reported decreased volume-wise concordance in patients with MDD relative to healthy controls. To characterize the local characteristics of the single voxel, ALFF and its normalized version fALFF have been used to compute the mean value of amplitudes within the 0.01–0.1 Hz low-frequency range from a Fourier decomposition of the blood oxygenation level-dependent (BOLD) time course (Zang et al., 2007; Zou et al., 2008). Regional homogeneity (ReHo) was developed to represent the level of regional brain activity coherence (Zang et al., 2004). Voxel-mirrored homotopic connectivity (VMHC) was adopted as the Pearson’s correlation coefficient between the time series of each voxel in one hemisphere and the time series of its symmetrical counterpart in the opposite hemisphere (Zuo et al., 2010b). Global signal connectivity (GSCorr) was considered as the Pearson’s correlation coefficient between the averaged time series and the time series of each voxel within the entire gray matter (Hahamy et al., 2014; Yang et al., 2017; Zhang et al., 2019). To depict the functional importance of the specific voxel, degree centrality (DC) was developed to calculate FC within the whole brain using the graph-theoretical approach (Buckner et al., 2009; Tomasi and Volkow, 2010; Zuo et al., 2012; Liu et al., 2015). Collectively, those R-fMRI indices have been applied widely to investigate aberrant intrinsic brain activity in depressed patients, which has enabled significant breakthroughs in the exploration of MDD neurobiological mechanisms (Guo et al., 2012; Liu et al., 2013, 2014; Shen et al., 2015; Gong et al., 2020; Ebneabbasi et al., 2021; Zhou et al., 2021). Therefore, in this study, we extensively applied dALFF, dynamic fALFF (dfALFF), dReHo, dynamic voxel mirrored homotopic connectivity (dVMHC), dynamic global signal correlation (dGSCorr), and dynamic DC (dDC) to investigate functional alterations of the brain in patients with MDD who experienced childhood trauma.
Previous studies have not explored alterations in variability and concordance of brain activity in MDD with childhood trauma. This study compared temporal dynamics analysis data based on R-fMRI images acquired from patients with MDD who experienced childhood trauma with data from patients with MDD who did not experience childhood trauma as well as healthy controls. We hypothesized that patients with MDD who experienced childhood trauma exhibit aberrant dynamic regional brain activity and concordance and that the concordance is associated with the severity of childhood trauma.
Materials and Methods
Participants
We recruited 78 patients with MDD and 108 healthy subjects for this study. MDD diagnosis was made by two psychiatrists with extensive experience using the DSM-5 diagnostic criteria. We used the Hamilton Depressive Rating Scale (HAMD) (Helmreich et al., 2012) to assess the depression severity (for those with MDD). It is well established that the Childhood Trauma Questionnaire (CTQ) is a reliable tool to evaluate the negative influence of maltreatment experience (Wu et al., 2022). Prior studies have proved that the CTQ has high validity in different countries (Kim et al., 2013; Isvoranu et al., 2017; Zhang et al., 2020; Petrikova et al., 2021). Using the CTQ cutoff points for the CTQ subscale scores to determine whether participants with and without traumatic experience has been widely validated and accepted (Jansen et al., 2016; Xie et al., 2018; Monteleone et al., 2020). Hence, we followed the same criterion to identify whether the participants suffered childhood maltreatment. To summarize, we used the cutoff point for the CTQ subscales score to distinguish the participants with and without childhood trauma, and the CTQ total score was used to quantify the severity of childhood maltreatment history. The CTQ total score and its subscale scores were used as continuous variables in this study. According to different types of childhood maltreatment, the CTQ can be divided into the following subscales: (i) emotional neglect (EN), (ii) physical neglect (PN), (iii) emotional abuse (EA), (iv) physical abuse (PA), and (vi) sexual abuse (SA) (Xie et al., 2018). The detailed cutoff points of CTQ subscales are shown below: (i) EN score ≥ 15, (ii) PN score ≥ 10, (iii) EA score ≥ 13, (iv) PA score ≥ 10, and SA score ≥ 8 (Jansen et al., 2016; Xie et al., 2018). Participants with any above-threshold score in the childhood trauma subtype will be considered as exposed to childhood maltreatment and will be included in our study. All participants received an assessment of the negative impact of traumatic history.
Based on whether each participant with or without traumatic history (using the cutoff points of the CTQ subscales to identify the participants with trauma exposure), had or had not been diagnosed with MDD (diagnosis of the patient with MDD was made by two psychiatrists), participants were divided into MDD with childhood trauma group (n = 48), MDD without childhood trauma group (n = 30), and healthy control group (n = 108). Patients with MDD were recruited from the inpatient department of the Affiliated Brain Hospital of Guangzhou Medical University. Correspondingly, 108 age-, gender-, and education-matched healthy controls were recruited from the advertising and nearby community. We excluded patients who (i) did not have a first episode of depression, (ii) had a history of any other major mental illness and physical disorder, (iii) had a family history of any other major mental illness and physical disorder, (iv) were taking psychiatric medication before (non-drug-naive), (v) received systemic psychotherapy and electroconvulsive therapy before, and (vi) were with contraindication for R-fMRI. The study was approved by the Ethics Committee of the Affiliated Brain Hospital of Guangzhou Medical University. All participants offered their written informed consent before the data collection.
Magnetic Resonance Imaging Data Acquisition
MRI images were obtained using a 3T Philips scanner at the radiology department of The Affiliated Brain Hospital of Guangzhou Medical University in China. (i) Resting-state functional scans were performed using a gradient-echo echoplanar imaging sequence with the parameters listed below: TR = 2,000 ms, TE = 30 ms, number of slices = 33, flip angle = 90°, matrix = 64 × 64, field of view = 220 × 220 mm2, and slice thickness = 4 mm with 0.6 mm interslice gap. The whole scanning process included 240 time points, lasting for 8 min. (ii) High-resolution 3D T1 images were acquired with the parameters listed below: TR/TE = 8.2/3.7 ms, number of slices = 188, slice thickness = 1 mm, flip angle = 7°, acquisition matrix = 256 × 256, and voxel size = 1 mm × 1 mm × 1 mm. All participants were instructed to close their eyes, relax, remain motionless, and keep awake.
Magnetic Resonance Imaging Data Preprocessing
Using the DPARSF toolbox (DPARSFA1) to preprocess the R-fMRI images, first, we removed the first ten volumes to allow data to reach equilibrium; second, we performed slice timing and head motion. Notably, the mean framewise displacement (FD) based on the Jenkinson model (FD-Jenkinson) was computed by averaging the FD from every time point for each subject (Jenkinson et al., 2002). We included only subjects with relatively low head motion (criteria: mean FD < 0.2 mm). Third, we conducted structural image alignment with a six-degree-of-freedom linear transformation to align the T1 image to the functional image; subsequently, we segmented the transformed structural images into the cerebrospinal fluid, white matter, and gray matter (Ashburner and Friston, 2005); Then, we spatially normalized the motion-corrected functional images into standard MNI space with 3 mm × 3 mm × 3 mm using the normalization parameters estimated during unified segmentation. The normalized images of the resulting ALFF and fALFF were then smoothed using a 4-mm FWHM Gaussian kernel. Subsequently, we treated Friston 24-head motion parameters, the white matter signal, and the CSF signal as the nuisance covariates to regress out (Friston et al., 1996). As for calculating ReHo, VMHC, and DC, the normalized images were subjected to nuisance regression to regress out Friston 24-head motion parameters, the white matter signal, and the CSF signal (Friston et al., 1996). Finally, the images were filtered with a temporal band-pass filter between 0.01 and 0.08 Hz.
Dynamic Resting-State Functional Magnetic Resonance Imaging Indices Calculation
Dynamic indices were computed using the temporal dynamic analysis toolkits on DPABI (Yan et al., 2016) (DPABI,2 version 4.5). We used the sliding-window approach to explore alterations in variability and concordance of dynamic R-fMRI indices throughout the whole brain. For the calculation of the dynamic R-fMRI indices, window length is an essential but open parameter. Prior research has pointed out that 50 TRs window length is the most suitable parameter to maintain the balance between achieving reliable estimates of intrinsic brain activity (with a longer window length) and capturing high-speed shifting dynamic brain activity (with a shorter window length) (Liao et al., 2019; Cui et al., 2020; Liu et al., 2021). Thus, a sliding window length of 50 TRs and a step size of 1 TR were selected to analyze the dynamic R-fMRI indices in this study.
The time series of each subject was divided into 181 windows. In each window, R-fMRI metrics, including ALFF, fALFF, ReHo, GSCorr, VMHC, and DC, were calculated. Then, the following dynamic indices were analyzed: dALFF, dfALFF, dReHo, dGSCorr, dVMHC, and dDC. Images for calculating ALFF and fALFF were smoothed but not filtered; the images for calculating the other indices were filtered but not smoothed. A standard deviation (SD) across the windows was then computed to represent the dynamic indices. Finally, smoothing and Z standardization were executed on the SD maps (apart from dALFF and dfALFF, which were smoothed before). Window sizes of 30 TRs and 70 TRs were also computed (refer to Supplementary Data).
Computation of Multiple Resting-State Functional Magnetic Resonance Imaging Indices
Interdependence among the following six R-fMRI brain activity indices was investigated:
(i) ALFF and fALFF: Above all, we transformed the time course into the frequency domain to acquire the corresponding power spectrum by using a Fast Fourier Transform. Then, we computed the square root at each frequency of the power spectrum. In particular, the averaged square root within the 0.01–0.1 Hz low-frequency range was considered the ALFF value (Zang et al., 2007). Moreover, fALFF was accepted as the ratio of the power spectrum within the 0.01–0.1 Hz low-frequency range to that of the whole frequency range (Zou et al., 2008). Owing to the high colinearity between ALFF and fALFF, we only included the fALFF value in the subsequent concordance calculation, as it improves specificity and sensitivity when examining regional brain activity (Zou et al., 2008; Zuo et al., 2010a; Yan et al., 2013).
(ii) ReHo: ReHo was adopted to represent the level of regional brain activity coherence. It was accepted as Kendall’s coefficient of concordance of the BOLD time course of a specific voxel with its 26 neighboring voxels’ time course (Zang et al., 2004).
(iii) GSCorr: GSCorr was considered the Pearson’s correlation coefficient between the averaged time series and time series of each voxel within the entire gray matter (Hahamy et al., 2014; Yang et al., 2017; Zhang et al., 2019). Afterward, the above GSCorr values underwent Fisher’s z-transformation to reach distribution normality.
(iv) VMHC: VMHC was adopted as the Pearson’s correlation coefficient between the time series of each voxel in one hemisphere and the time series of its symmetrical counterpart in the opposite hemisphere (Zuo et al., 2010b). Subsequently, the above VMHC values underwent Fisher’s z-transformation to reach distribution normality.
(v) DC: We computed the Pearson’s correlation coefficients between the time series of all the pairwise voxels within the entire gray matter. This correspondingly resulted in the FC matrix of the entire gray matter. DC was accepted as the sum of positive FC (defined as FC values above a threshold of 0.25) between a given voxel and the rest of the voxels (Buckner et al., 2009; Zuo et al., 2012).
Our study is an exploratory analysis and aims to examine the aberrant variability and concordance of dynamic resting-state fMRI indices (i.e., dALFF, dfALFF, dReHo, dVMHC, dGSCorr, and dDC) in patients with MDD who experienced childhood trauma. Following extensive exploratory analysis, we observed significant variability differences in dALFF and dDC. However, no significant variability difference was identified for the other metrics.
Concordance Analysis
Concordance values were computed based on Kendall’s W coefficient. Two types of concordance indices were calculated: (i) volume-wise concordance, computed as the global level concordance index across voxels; and (ii) voxel-wise concordance, computed as the voxel-level concordance across time windows of each subject.
Statistical Analysis
Statistical analyses were conducted using SPSS software version 19.0 (IBM Corp., Armonk, NY, United States). Demographic data, clinical scale scores, and volume-wise concordance were compared between groups using the chi-square test and one-way ANOVA with post hoc Bonferroni correction. To compare voxel-wise concordance and standardized SD maps between groups, one-way ANOVA with post hoc Bonferroni correction for multiple comparisons was conducted. Significant results are obtained from the multiple comparisons with Bonferroni correction post hoc tests. Family-wise error correction (FWE) was conducted with a significance threshold of p < 0.05 and a cluster size of > 15 voxels (Wang et al., 2014). Mean dynamic index values were extracted from brain regions showing significant intergroup differences in the voxel-wise dynamic analyses. In the multiple comparisons in regions with differences in dDC, p < 0.05/2 = 0.025 was accepted as significant owing to dDC analysis resulting in two significant clusters. In the multiple comparisons in regions with differences in voxel-wise concordance, p < 0.05/3 = 0.016 was accepted as significant (voxel-wise concordance analysis resulting in three significant clusters). Pearson’s correlation analyses were used to explore the associations of voxel-wise concordance with CTQ score in all participants [p < 0.05/18 = 0.0027, with Bonferroni correction of 18 being due to three clusters and 6 scales (i.e., CTQ scale and its five subscales)]. In addition, to further quantitatively compare correlation coefficients between groups, we used a regression model with group moderating the associations of dynamic indices with CTQ score (p < 0.05/3 = 0.016, with Bonferroni correction of 3 due to three clusters). In this study, age, gender, and education were considered as control variables.
Results
Demographic Data
As shown in Table 1, no significant differences were found between the patients with MDD-associated childhood trauma, patients without MDD-associated childhood trauma, and control groups with respect to demographic data, Hamilton Anxiety Rating Scale score, and Hamilton Depressive Rating Scale score. However, the CTQ score and its subscale scores significantly differed between groups.
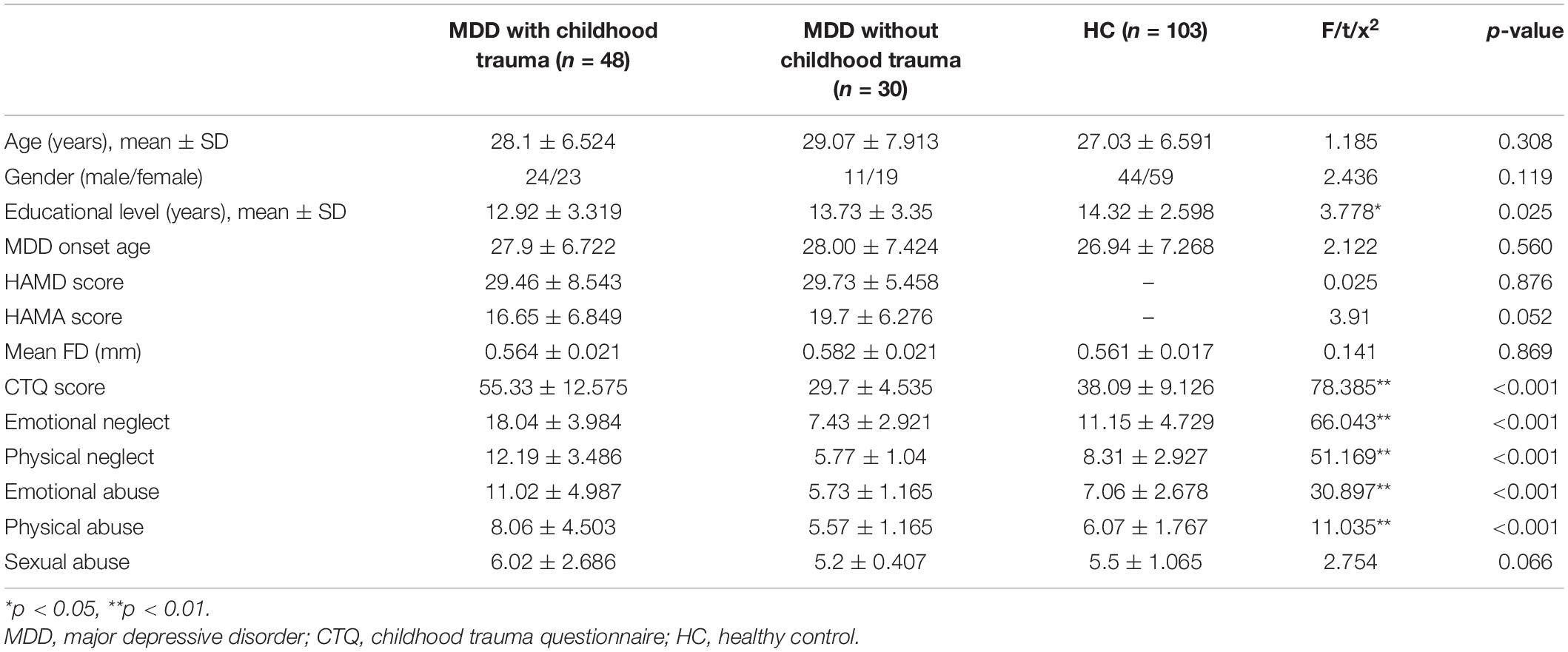
Table 1. Demographic and clinical scale scores of MDD with childhood trauma, MDD without childhood trauma, and HC group.
Dynamics of Resting-State Functional Magnetic Resonance Imaging Indices
Intergroup differences in dALFF were detected in the left lingual gyrus (Table 2 and Figure 1), whereas differences in dDC were observed in the right lingual gyrus and the right calcarine cortex (Table 2 and Figure 1). The post hoc testing showed that dALFF and dDC were lower in the patients with childhood trauma-associated MDD group than in the patients without childhood trauma-associated MDD and the control group (Table 3 and Figure 1). Other dynamic indices did not significantly differ between groups.
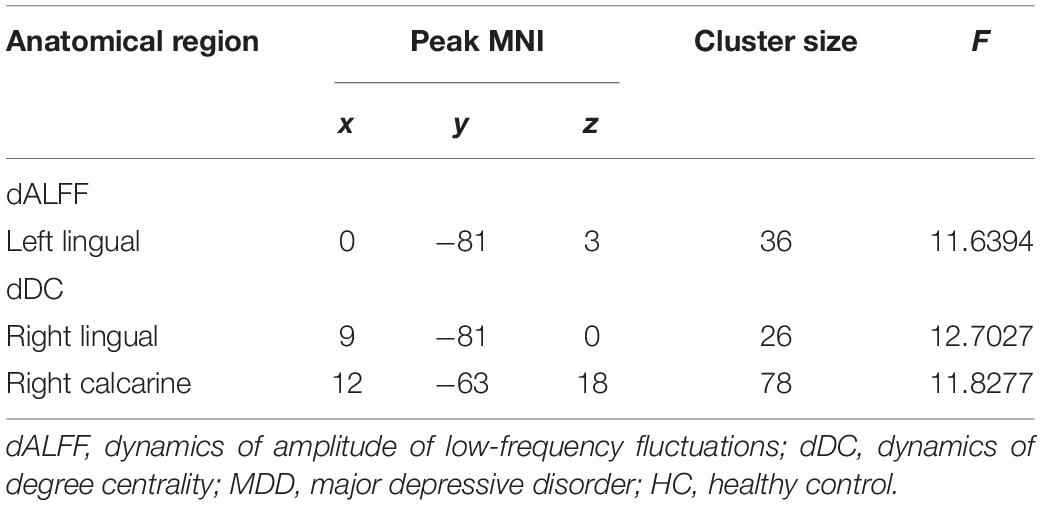
Table 2. Regions with differences in dynamic R-fMRI indices among the MDD with childhood trauma, MDD without childhood trauma, and HC groups.
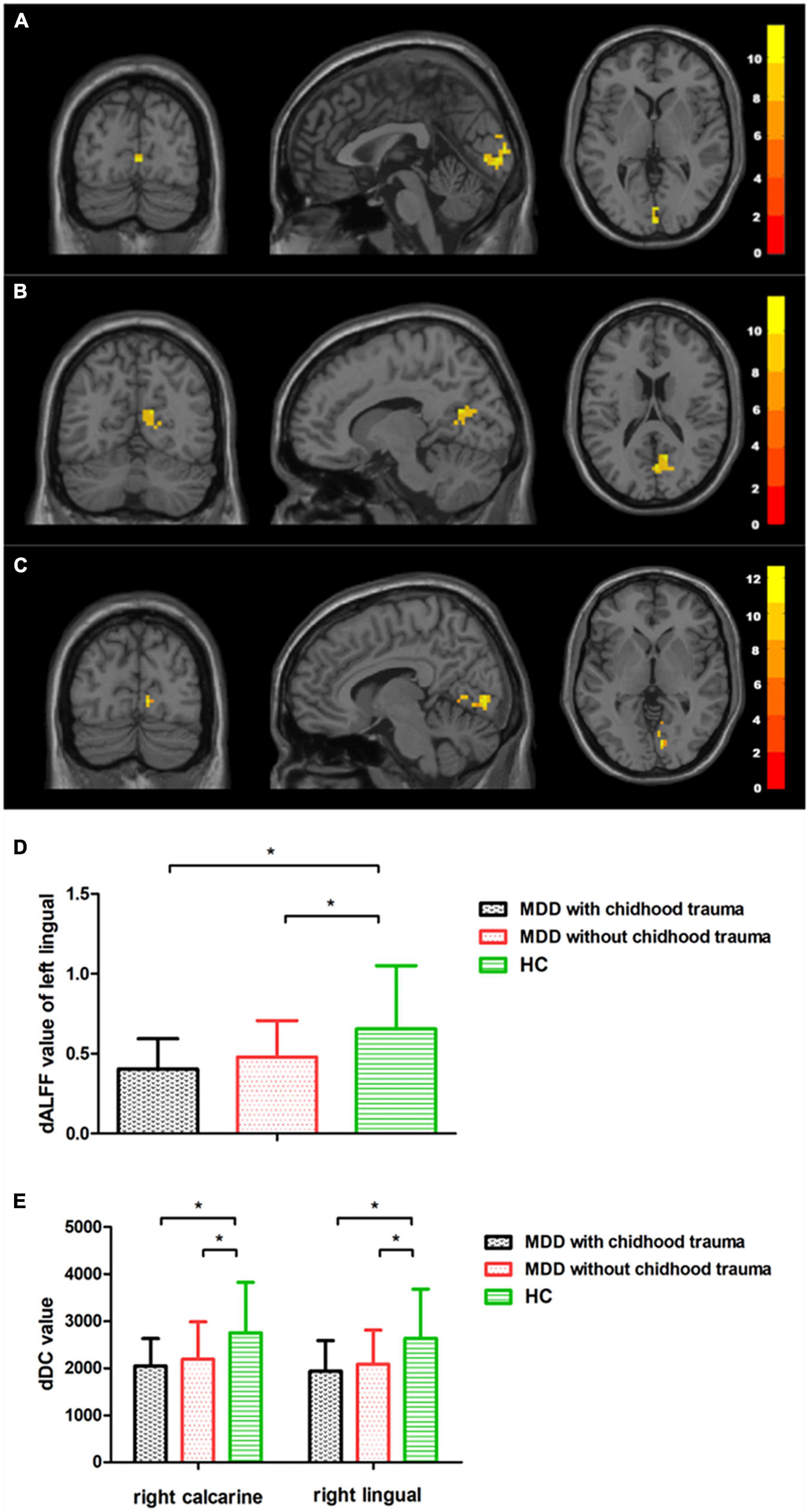
Figure 1. Regions with differences in dALFF and dDC between the MDD with childhood trauma, MDD without childhood trauma, and HC groups and post hoc analysis. Relative to HC, MDD with childhood trauma showed decreased dALFF in left lingual and decreased dDC in right calcarine and right lingual. MDD: major depressive disorder; HC: healthy control; dALFF, dynamics of amplitude of low-frequency fluctuations; dDC, dynamics of degree centrality. * means the p-value has reached a significant level. In the multiple comparisons in regions with differences in dDC, p < 0.05/1 = 0.05 was accepted as significant; In the multiple comparisons in regions with differences in dDC, p < 0.05/2 = 0.025 was accepted as significant.
Volume-Wise Concordance of Resting-State Functional Magnetic Resonance Imaging Indices
Mean volume-wise concordance values significantly differed among the three groups (p = 0.001). The post hoc testing showed that mean concordance was lower in the patients with childhood trauma-associated MDD group than in the control group. SD values of volume-wise concordance did not significantly differ between the three groups (p = 0.996; Figure 2 and Table 4).
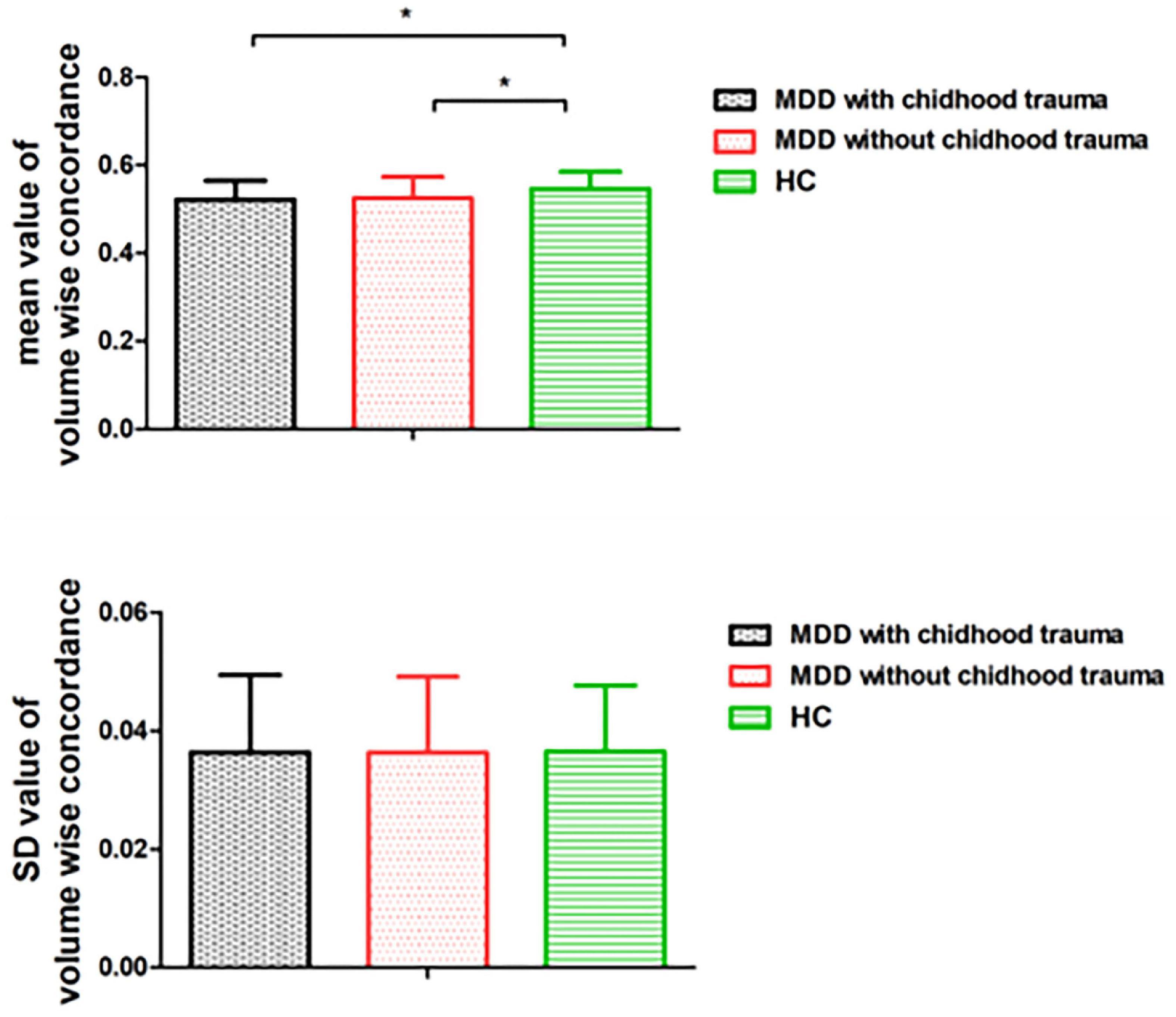
Figure 2. Comparison of volume wise concordance among the MDD with childhood trauma, MDD without childhood trauma, and HC groups. MDD, major depressive disorder; HC, healthy control. *p < 0.05.
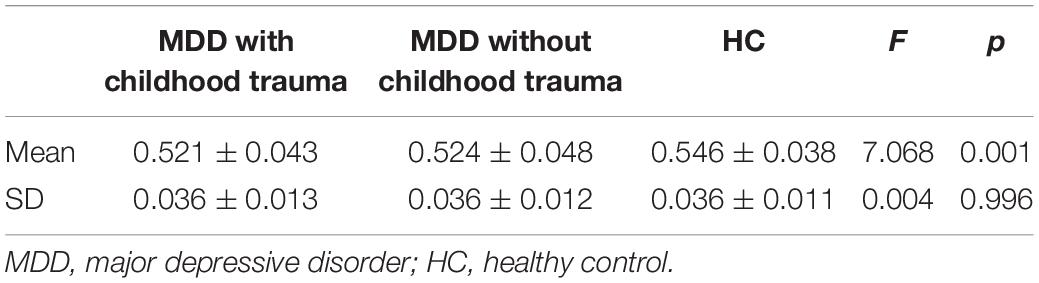
Table 4. Comparison of volume wise concordance among the MDD with childhood trauma, MDD without childhood trauma, and HC groups.
Voxel-Wise Concordance of Resting-State Functional Magnetic Resonance Imaging Indices
Significant differences were observed in the left middle temporal and bilateral calcarine cortices when comparing voxel-wise concordance between the groups (Figure 3 and Table 5). Multiple comparisons showed that the concordance of these regions in the patients with childhood trauma-associated MDD group was lower than that in the other two groups (Figure 3 and Table 6).
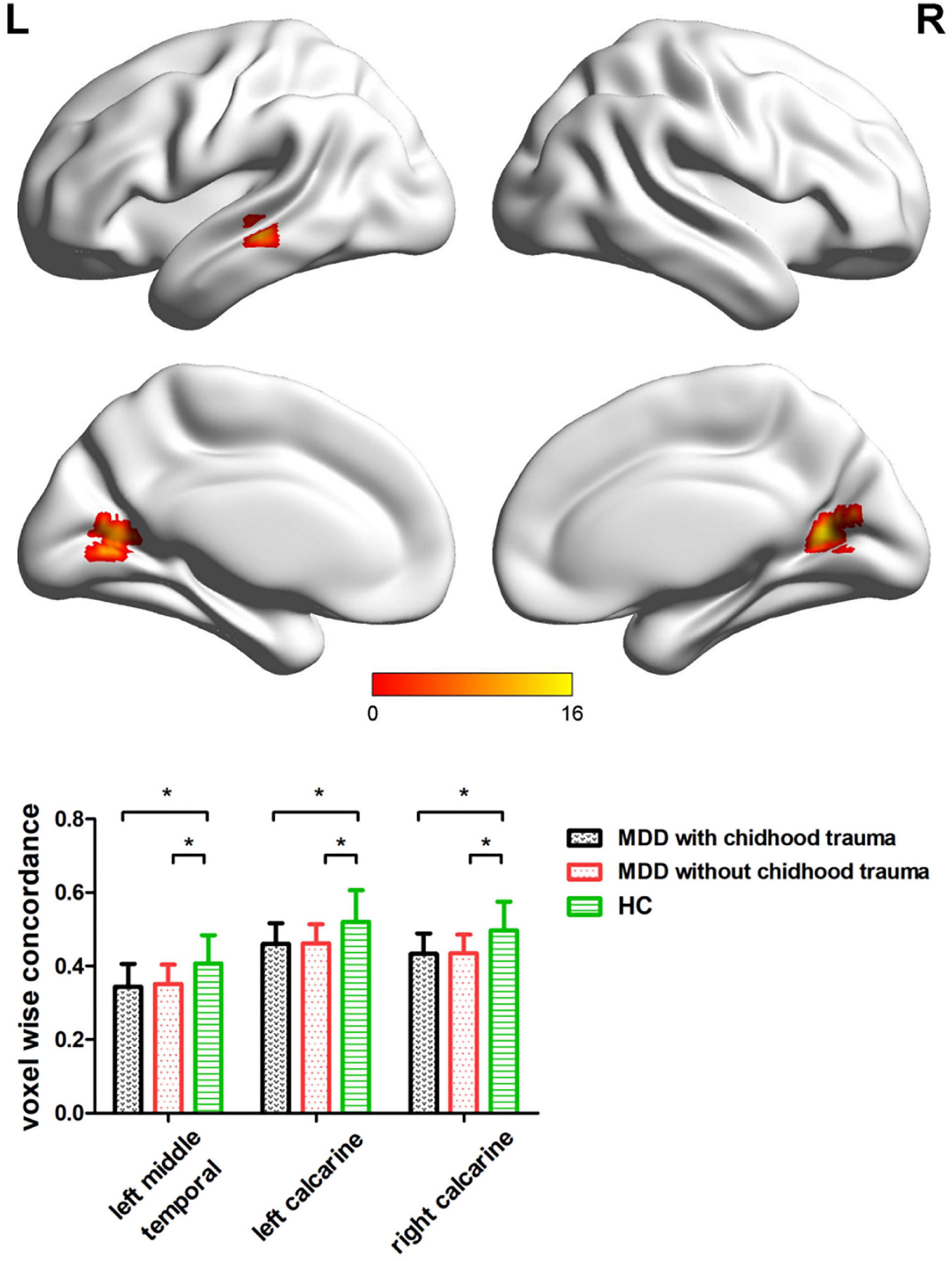
Figure 3. Regions with differences in the voxel wise concordance of R-fMRI indices among the MDD with childhood trauma, MDD without childhood trauma, and HC groups and post-hoc analysis. MDD, major depressive disorder; HC, healthy control. * means the p-value has reached a significant level. In the multiple comparisons in regions with differences in voxel-wise concordance, p < 0.05/3 = 0.016 was accepted as significant (voxel-wise concordance analysis resulting in three significant clusters).
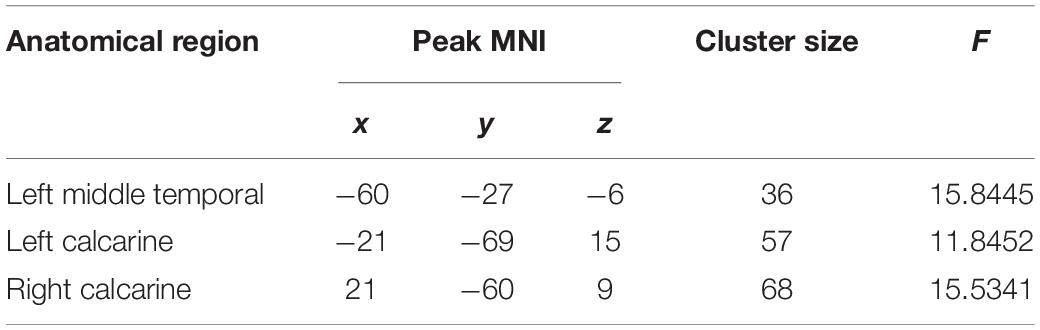
Table 5. Regions with differences in the voxel-wise concordance of R-fMRI indices among the MDD with childhood trauma, MDD without childhood trauma, and HC groups.
Correlation Analysis and Multiple Linear Regression Analysis
We further examined the associations of dALFF and dDC with the CTQ total score. As shown in Figure 4 and Table 7, the correlation analyses revealed that childhood trauma history was negatively correlated with voxel-wise concordance in the left middle temporal (r = −0.166, p = 0.026), left calcarine (r = −0.160, p = 0.032), and right calcarine (r = −0.165, p = 0.027), respectively.
Moreover, multiple linear regression analysis (Baron and Kenny, 1986) was used to investigate whether a history of childhood trauma has the same effect on dALFF and dDC in individuals with and without depression. We defined group difference (depressed or not), CTQ total score, and their interaction as independent variables; functional concordance was defined as the dependent variable; age, gender, and education were considered as nuisance covariates. To avoid multicollinearity, we performed mean centering for all the independent variables before constructing the interaction terms (Holmbeck, 1997). As shown in Table 8, further regression analyses showed an interaction of group and childhood trauma history on dALFF of the left lingual gyrus (F = 6.798, p < 0.001), dDC of the right lingual gyrus (F = 5.423, p < 0.001), and dDC of the right calcarine cortex (F = 5.529, p < 0.001).
Discussion
This study adopted temporal dynamic analysis to examine aberrant variability and concordance of intrinsic brain activity in patients with childhood trauma-associated MDD. Several findings were interesting: (i) patients with childhood trauma-associated MDD exhibited lower dALFF in the left lingual gyrus and lower dDC in the right calcarine cortex as well as the right lingual gyrus relative to healthy subjects; (ii) patients with childhood trauma-associated MDD showed decreased volume-wise concordance compared with healthy controls; (iii) decreased voxel-wise concordance was observed in the left middle temporal cortex and bilateral calcarine cortices in patients with childhood trauma-associated MDD; and (iv) multiple linear regression analysis revealed that history of childhood trauma had a different impact on aberrant brain functional concordance in depressed patients and healthy subjects. However, dynamic R-fMRI index and functional concordance analyses showed no significant differences between the MDD with childhood trauma group and the MDD without childhood trauma group, which may be related to our small sample size.
Patients with childhood trauma-associated MDD had lower dALFF (mainly detected in the left lingual gyrus) and dDC (mainly detected in the right lingual gyrus and right calcarine cortex) than healthy subjects, suggesting stable but inflexible intrinsic brain activity in patients with childhood trauma-associated MDD. Previous studies have suggested that dysfunction in the lingual gyrus and calcarine cortex is closely linked to the development of MDD in patients with previous childhood trauma. Childhood trauma has been associated with impairment of emotion regulation, involving the multiprocess of emotion regulatory stages that precede and follow psychological regulatory implementation (Bonanno and Burton, 2013). Preliminary investigations that focused on the neural basis of emotion dysregulation have reported that activation in specific brain areas (including the lingual gyrus and calcarine cortex) is associated with attentional deployment, cognitive change, and response modulation (Sheppes et al., 2015).
Greater activation in the left lingual gyrus has been observed during the processing of the sadness emotion (Groves et al., 2018), suggesting that different patterns of brain activation in the lingual gyrus might be related to the underlying neural mechanisms of depression. Moreover, Daniels et al. (2012) identified a positive relationship between the history of childhood trauma and higher activation in the lingual gyrus. Evidence from temporal dynamics analysis has also revealed a key role for the lingual gyrus in processing negative emotions. In a neuroimaging study of brain dynamics in depressed patients, Zhang et al. (2021) detected significantly lower dALFF in patients with MDD relative to healthy subjects, which is in line with our findings. In a dynamic functional network connectivity analysis, Zhi et al. (2018) found that depressed patients exhibited decreased harmonic centrality values in the lingual gyrus, which was correlated with clinical symptom severity and self-cognition. In depressed patients with suicidal ideation, an aberrant dynamic functional connection between the lingual gyrus and habenula has been detected (Qiao et al., 2020). Considering the findings of prior studies as well as this study, abnormal brain activity variability in the lingual gyrus might indicate disrupted dynamic intrinsic brain activity in patients with MDD. Moreover, the lingual gyrus is widely involved in distinguishing emotional facial expressions and verbal declarative memory (Kitada et al., 2010); difficulties with these processes are common in patients with childhood trauma-associated MDD. The lingual gyrus dysfunction in patients with childhood trauma-associated MDD might reflect an increased ability to identify and encode adverse experiences in verbal declarative memory (Kitada et al., 2010). In conclusion, the alterations in variability in the lingual gyrus might be specific to the additive effects of MDD and childhood trauma history.
We detected a significant decrease in dDC in the right lingual gyrus and right calcarine cortex. Calcarine cortex dysfunction is frequently observed in patients with MDD and is closely related to depression severity. A previous study has confirmed a relationship between increased depressive symptoms and increased FC between the calcarine cortex and basolateral amygdala in veterans with MDD (McGlade et al., 2020). Additionally, a previous meta-analysis reported decreased cortical thickness in the left calcarine cortex and lingual gyrus in depressed patients compared with healthy controls (Suh et al., 2019). Similarly, decreased normalized cerebral blood flow in the right calcarine cortex in early-onset MDD patients has been observed, providing more experimental evidence for the contribution of calcarine dysfunction to the development of depression (Liao et al., 2017). More powerful evidence from FC analysis detected significantly reduced FC between the right posterior insular gyrus, calcarine cortex, and lingual gyrus in adolescents with MDD (Hu et al., 2019). Notably, childhood trauma might also cause calcarine cortex dysfunction (Luo et al., 2022). In addition to statistical analysis, dynamic analysis has also revealed a key role of abnormal calcarine variability in contributing to the negative impact of depression from the perspective of temporal dynamics. Decreased dALFF has been previously detected in the calcarine cortex (Zhang et al., 2021), which is consistent with our findings and further confirms the relationship between the development of depression and abnormal brain variability in the calcarine cortex. Similarly, by examining alterations in dfALFF, Hu, L. and colleagues identified altered variability in the calcarine cortex in depressed patients with mild cognitive impairment relative to those without mild cognitive impairment (Yu Y. et al., 2019). Furthermore, Li et al. (2021) surprisingly found that baseline functional stability in the calcarine cortex could effectively predict improvement of clinical symptoms in depressed patients. The altered variability in the calcarine cortex observed in our study may be a core neurobiological feature of MDD with childhood trauma.
Intergroup differences were found in functional voxel-wise and volume-wise concordance. Specifically, patients with childhood trauma-associated MDD showed decreased voxel-wise concordance in the left middle temporal, left calcarine, and right calcarine cortices compared with healthy controls; volume-wise concordance was also lower in patients with childhood trauma-associated MDD. The temporal gyrus is involved in language and memory function (Eichenbaum et al., 2007), whereas the calcarine cortex plays a key role in integrating “visuopsychic” and “visuosensory” processing (Ffytche and Catani, 2005). In a previous study, adults who experienced childhood trauma had increased activation in the left middle temporal gyrus and left superior frontal gyrus, indicating an association between middle temporal gyrus dysfunction and underlying neurophysiological MDD mechanisms (Heany et al., 2018). Furthermore, increased FC between the calcarine cortex and amygdala has been shown in patients with post-traumatic stress disorder, which demonstrates that the calcarine cortex plays an essential role in the processing of fear and threat cues (Morey et al., 2015). Moreover, R-fMRI metrics have been shown to have a high concordance in cortical and subcortical areas across the whole time window (Yan et al., 2017). Voxel-wise concordance might characterize the homogeneity between the various R-fMRI metrics (Lou et al., 2021). Therefore, aberrant functional concordance in the left middle temporal and left and right calcarine cortices might reflect the impaired integrative function of intrinsic brain activity.
In our multiple linear regression analyses of dynamic indices and childhood trauma history, group differences (depressed or not) significantly moderated the relationship between dALFF and dDC and childhood trauma history, indicating that childhood trauma has a significantly different impact on aberrant brain functional concordance in depressed patients and healthy subjects. This result highlights the key role of childhood trauma in mental health development and provides evidence that it is detrimental.
Limitations and Future Directions
This study had several limitations. First, we used a cross-sectional approach, which does not examine cause and effect. Second, the sample size was small, which is probably why we could not detect a difference between MDD patients with and without childhood trauma. Future large-scale studies are warranted. Moreover, childhood trauma subtype analyses were not conducted due to the small sample size. Future studies should focus on the impact of a single subtype of childhood trauma, such as neglect or abuse. Third, childhood trauma was assessed retrospectively via self-report; although the CTQ is reliable and widely used, evaluation of traumatic history using an objective tool would have been preferable.
Conclusion
Patients with childhood trauma-associated MDD demonstrated aberrant variability and concordance in intrinsic brain activity. These aberrances may be an underlying neurobiological mechanism that explains MDD from the perspective of temporal dynamics.
Data Availability Statement
The original contributions presented in the study are included in the article/Supplementary Material, further inquiries can be directed to the corresponding author/s.
Ethics Statement
The studies involving human participants were reviewed and approved by the Ethics Committee of the Affiliated Brain Hospital of Guangzhou Medical University. The patients/participants provided their written informed consent to participate in this study.
Author Contributions
HP, HW, and QL designed the study and drafted the primary manuscript. HW, ZW, and QL supervised the recruitment and made statistical analyses. JC, QL, and YL took part in recruitment and data management. HP and HW made further revisions to the manuscript. All authors had read and approved the final manuscript.
Funding
This study design was supported by the Guangdong Natural Science Foundation, China (2015A030313800) to HP. The data collection of the study was also supported by the Guangzhou Municipal Key Discipline in Medicine for Guangzhou Brain Hospital (GBH2014-ZD04) to HP.
Conflict of Interest
The authors declare that the research was conducted in the absence of any commercial or financial relationships that could be construed as a potential conflict of interest.
Publisher’s Note
All claims expressed in this article are solely those of the authors and do not necessarily represent those of their affiliated organizations, or those of the publisher, the editors and the reviewers. Any product that may be evaluated in this article, or claim that may be made by its manufacturer, is not guaranteed or endorsed by the publisher.
Acknowledgments
We thank Liwen Bianji (Edanz) (https://www.liwenbianji.cn) for editing the language of a draft of this manuscript.
Supplementary Material
The Supplementary Material for this article can be found online at: https://www.frontiersin.org/articles/10.3389/fnins.2022.852799/full#supplementary-material
Footnotes
References
Allen, E. A., Damaraju, E., Plis, S. M., Erhardt, E. B., and Eichele, T. (2014). Tracking whole-brain connectivity dynamics in the resting state. Cereb. Cortex 24, 663–676. doi: 10.1093/cercor/bhs352
Ashburner, J., and Friston, K. J. (2005). Unified segmentation. Neuroimage 26, 839–851. doi: 10.1016/j.neuroimage.2005.02.018
Baron, R. M., and Kenny, D. A. (1986). The moderator-mediator variable distinction in social psychological research: conceptual, strategic, and statistical considerations. J. Pers. Soc. Psychol. 51, 1173–1182. doi: 10.1037//0022-3514.51.6.1173
Begemann, M., Schutte, M., van Dellen, E., Abramovic, L., and Boks, M. P. (2021). Childhood trauma is associated with reduced frontal gray matter volume: a large transdiagnostic structural MRI study. Psychol. Med. [Online ahead of print]. doi: 10.1017/S0033291721002087
Bonanno, G. A., and Burton, C. L. (2013). Regulatory flexibility: an individual differences perspective on coping and emotion regulation. Perspect. Psychol. Sci. 8, 591–612. doi: 10.1177/1745691613504116
Buckner, R. L., Sepulcre, J., Talukdar, T., Krienen, F. M., and Liu, H. (2009). Cortical hubs revealed by intrinsic functional connectivity: mapping, assessment of stability, and relation to Alzheimer’s disease. J. Neurosci. 29, 1860–1873. doi: 10.1523/JNEUROSCI.5062-08.2009
Cui, Q., Sheng, W., Chen, Y., Pang, Y., and Lu, F. (2020). Dynamic changes of amplitude of low-frequency fluctuations in patients with generalized anxiety disorder. Hum. Brain Mapp. 41, 1667–1676. doi: 10.1002/hbm.24902
Danese, A., and Baldwin, J. R. (2017). Hidden wounds? inflammatory links between childhood trauma and psychopathology. Annu. Rev. Psychol. 68, 517–544. doi: 10.1146/annurev-psych-010416-044208
Daniels, J. K., Coupland, N. J., Hegadoren, K. M., Rowe, B. H., and Densmore, M. (2012). Neural and behavioral correlates of peritraumatic dissociation in an acutely traumatized sample. J. Clin. Psychiatry 73, 420–426. doi: 10.4088/JCP.10m06642
de Kwaasteniet, B., Ruhe, E., Caan, M., Rive, M., and Olabarriaga, S. (2013). Relation between structural and functional connectivity in major depressive disorder. Biol. Psychiatry 74, 40–47. doi: 10.1016/j.biopsych.2012.12.024
Du, L., Wang, J., Meng, B., Yong, N., and Yang, X. (2016). Early life stress affects limited regional brain activity in depression. Sci. Rep. 6:25338. doi: 10.1038/srep25338
Dube, S. R., Felitti, V. J., Dong, M., Chapman, D. P., and Giles, W. H. (2003). Childhood abuse, neglect, and household dysfunction and the risk of illicit drug use: the adverse childhood experiences study. Pediatrics 111, 564–572. doi: 10.1542/peds.111.3.564
Duncan, N. W., Hayes, D. J., Wiebking, C., Tiret, B., and Pietruska, K. (2015). Negative childhood experiences alter a prefrontal-insular-motor cortical network in healthy adults: a preliminary multimodal rsfMRI-fMRI-MRS-dMRI study. Hum. Brain Map. 36, 4622–4637. doi: 10.1002/hbm.22941
Ebneabbasi, A., Mahdipour, M., Nejati, V., Li, M., and Liebe, T. (2021). Emotion processing and regulation in major depressive disorder: a 7T resting-state fMRI study. Hum. Brain Map. 42, 797–810. doi: 10.1002/hbm.25263
Eichenbaum, H., Yonelinas, A. P., and Ranganath, C. (2007). The medial temporal lobe and recognition memory. Annu. Rev. Neurosci. 30, 123–152. doi: 10.1146/annurev.neuro.30.051606.094328
Ffytche, D. H. andCatani, M. (2005). Beyond localization: from hodology to function. Philos Trans. R Soc. Lond. B Biol. Sci. 360, 767–779. doi: 10.1098/rstb.2005.1621
Figueroa, C. A., Ruhe, H. G., Koeter, M. W., Spinhoven, P., and Van der Does, W. (2015). Cognitive reactivity versus dysfunctional cognitions and the prediction of relapse in recurrent major depressive disorder. J. Clin. Psychiatry 76, e1306–e1312. doi: 10.4088/JCP.14m09268
Friston, K. J., Williams, S., Howard, R., Frackowiak, R. S., and Turner, R. (1996). Movement-related effects in fMRI time-series. Magn. Reson. Med. 35, 346–355. doi: 10.1002/mrm.1910350312
Gong, J., Wang, J., Qiu, S., Chen, P., and Luo, Z. (2020). Common and distinct patterns of intrinsic brain activity alterations in major depression and bipolar disorder: voxel-based meta-analysis. Transl. Psychiatry 10:353. doi: 10.1038/s41398-020-01036-5
Gotlib, I. H., and Joormann, J. (2010). Cognition and depression: current status and future directions. Annu. Rev. Clin. Psychol. 6, 285–312. doi: 10.1146/annurev.clinpsy.121208.131305
Groves, S. J., Pitcher, T. L., Melzer, T. R., Jordan, J., and Carter, J. D. (2018). Brain activation during processing of genuine facial emotion in depression: preliminary findings. J. Affect. Disord. 225, 91–96. doi: 10.1016/j.jad.2017.07.049
Guo, W. B., Liu, F., Xue, Z. M., Xu, X. J., and Wu, R. R. (2012). Alterations of the amplitude of low-frequency fluctuations in treatment-resistant and treatment-response depression: a resting-state fMRI study. Prog. Neuropsychopharmacol. Biol. Psychiatry 37, 153–160. doi: 10.1016/j.pnpbp.2012.01.011
Hahamy, A., Calhoun, V., Pearlson, G., Harel, M., and Stern, N. (2014). Save the global: global signal connectivity as a tool for studying clinical populations with functional magnetic resonance imaging. Brain Connect. 4, 395–403. doi: 10.1089/brain.2014.0244
Hammen, C. (2005). Stress and depression. Annu. Rev. Clin. Psychol. 1, 293–319. doi: 10.1146/annurev.clinpsy.1.102803.143938
Hammen, C., and Brennan, P. A. (2002). Interpersonal dysfunction in depressed women: impairments independent of depressive symptoms. J. Affect. Disord. 72, 145–156. doi: 10.1016/s0165-0327(01)00455-4
Heany, S. J., Groenewold, N. A., Uhlmann, A., Dalvie, S., and Stein, D. J. (2018). The neural correlates of Childhood Trauma Questionnaire scores in adults: a meta-analysis and review of functional magnetic resonance imaging studies. Dev. Psychopathol. 30, 1475–1485. doi: 10.1017/S0954579417001717
Heim, C., Newport, D. J., Mletzko, T., Miller, A. H., and Nemeroff, C. B. (2008). The link between childhood trauma and depression: insights from HPA axis studies in humans. Psychoneuroendocrinology 33, 693–710. doi: 10.1016/j.psyneuen.2008.03.008
Helmreich, I., Wagner, S., Mergl, R., Allgaier, A. K., and Hautzinger, M. (2012). Sensitivity to changes during antidepressant treatment: a comparison of unidimensional subscales of the Inventory of Depressive Symptomatology (IDS-C) and the Hamilton Depression Rating Scale (HAMD) in patients with mild major, minor or subsyndromal depression. Eur. Arch. Psychiatry Clin. Neurosci. 262, 291–304. doi: 10.1007/s00406-011-0263-x
Holmbeck, G. N. (1997). Toward terminological, conceptual, and statistical clarity in the study of mediators and moderators: examples from the child-clinical and pediatric psychology literatures. J Consult Clin Psychol 65, 599–610. doi: 10.1037//0022-006x.65.4.599
Howard, D. M., Adams, M. J., Clarke, T. K., Hafferty, J. D., and Gibson, J. (2019). Genome-wide meta-analysis of depression identifies 102 independent variants and highlights the importance of the prefrontal brain regions. Nat. Neurosci. 22, 343–352. doi: 10.1038/s41593-018-0326-7
Hu, L., Xiao, M., Ai, M., Wang, W., and Chen, J. (2019). Disruption of resting-state functional connectivity of right posterior insula in adolescents and young adults with major depressive disorder. J. Affect. Disord. 257, 23–30. doi: 10.1016/j.jad.2019.06.057
Huh, H. J., Kim, K. H., Lee, H. K., and Chae, J. H. (2017). The relationship between childhood trauma and the severity of adulthood depression and anxiety symptoms in a clinical sample: the mediating role of cognitive emotion regulation strategies. J. Affect. Disord. 213, 44–50. doi: 10.1016/j.jad.2017.02.009
Hutchison, R. M., Womelsdorf, T., Allen, E. A., Bandettini, P. A., and Calhoun, V. D. (2013). Dynamic functional connectivity: promise, issues, and interpretations. Neuroimage 80, 360–378. doi: 10.1016/j.neuroimage.2013.05.079
Isvoranu, A. M., van Borkulo, C. D., Boyette, L. L., Wigman, J. T., and Vinkers, C. H. (2017). A network approach to psychosis: pathways between childhood trauma and psychotic symptoms. Schizophr. Bull. 43, 187–196. doi: 10.1093/schbul/sbw055
Jansen, K., Cardoso, T. A., Fries, G. R., Branco, J. C., and Silva, R. A. (2016). Childhood trauma, family history, and their association with mood disorders in early adulthood. Acta Psychiatr. Scand. 134, 281–286. doi: 10.1111/acps.12551
Jenkinson, M., Bannister, P., Brady, M., and Smith, S. (2002). Improved optimization for the robust and accurate linear registration and motion correction of brain images. Neuroimage 17, 825–841. doi: 10.1016/s1053-8119(02)91132-8
Kessler, R. C., and Bromet, E. J. (2013). The epidemiology of depression across cultures. Annu. Rev. Public Health 34, 119–138. doi: 10.1146/annurev-publhealth-031912-114409
Kim, D., Bae, H., Han, C., Oh, H. Y., and Macdonald, K. (2013). Psychometric properties of the Childhood Trauma Questionnaire-Short Form (CTQ-SF) in Korean patients with schizophrenia. Schizoph. Res. 144, 93–98. doi: 10.1016/j.schres.2012.12.020
Kisely, S., Abajobir, A. A., Mills, R., Strathearn, L., and Clavarino, A. (2018). Child maltreatment and mental health problems in adulthood: birth cohort study. Br. J. Psychiatry 213, 698–703. doi: 10.1192/bjp.2018.207
Kitada, R., Johnsrude, I. S., Kochiyama, T., and Lederman, S. J. (2010). Brain networks involved in haptic and visual identification of facial expressions of emotion: an fMRI study. Neuroimage 49, 1677–1689. doi: 10.1016/j.neuroimage.2009.09.014
Kuehner, C. (2017). Why is depression more common among women than among men? Lancet Psychiatry 4, 146–158. doi: 10.1016/S2215-0366(16)30263-2
Li, X., Zhang, Y., Meng, C., Zhang, C., and Zhao, W. (2021). Functional stability predicts depressive and cognitive improvement in major depressive disorder: a longitudinal functional MRI study. Prog. Neuropsychopharmacol. Biol. Psychiatry 111:110396. doi: 10.1016/j.pnpbp.2021.110396
Liao, W., Li, J., Ji, G. J., Wu, G. R., and Long, Z. (2019). Endless fluctuations: temporal dynamics of the amplitude of low frequency fluctuations. IEEE Trans. Med. Imaging 38, 2523–2532. doi: 10.1109/TMI.2019.2904555
Liao, W., Wang, Z., Zhang, X., Shu, H., and Wang, Z. (2017). Cerebral blood flow changes in remitted early- and late-onset depression patients. Oncotarget 8, 76214–76222. doi: 10.18632/oncotarget.19185
Liu, F., Guo, W., Liu, L., Long, Z., and Ma, C. (2013). Abnormal amplitude low-frequency oscillations in medication-naive, first-episode patients with major depressive disorder: a resting-state fMRI study. J. Affect. Disord. 146, 401–406. doi: 10.1016/j.jad.2012.10.001
Liu, F., Zhu, C., Wang, Y., Guo, W., and Li, M. (2015). Disrupted cortical hubs in functional brain networks in social anxiety disorder. Clin. Neurophysiol. 126, 1711–1716. doi: 10.1016/j.clinph.2014.11.014
Liu, J., Bu, X., Hu, X., Li, H., and Cao, L. (2021). Temporal variability of regional intrinsic neural activity in drug-naive patients with obsessive-compulsive disorder. Hum. Brain Map. 42, 3792–3803. doi: 10.1002/hbm.25465
Liu, J., Ren, L., Womer, F. Y., Wang, J., and Fan, G. (2014). Alterations in amplitude of low frequency fluctuation in treatment-naive major depressive disorder measured with resting-state fMRI. Hum. Brain Map. 35, 4979–4988. doi: 10.1002/hbm.22526
Lou, F., Tao, J., Zhou, R., Chen, S., and Qian, A. (2021). Altered variability and concordance of dynamic resting-state fMRI indices in patients with attention deficit hyperactivity disorder. Front. Neurosci. 15:731596. doi: 10.3389/fnins.2021.731596
Luo, Q., Chen, J., Li, Y., Wu, Z., and Lin, X. (2022). Altered regional brain activity and functional connectivity patterns in major depressive disorder: a function of childhood trauma or diagnosis? J. Psychiatr. Res. 147, 237–247. doi: 10.1016/j.jpsychires.2022.01.038
McCarron, R. M., Shapiro, B., Rawles, J., and Luo, J. (2021). Depression. Ann. Intern. Med. 174, C65–C80. doi: 10.7326/AITC202105180
McGlade, E., Rogowska, J., DiMuzio, J., Bueler, E., and Sheth, C. (2020). Neurobiological evidence of sexual dimorphism in limbic circuitry of US Veterans. J. Affect. Disord. 274, 1091–1101. doi: 10.1016/j.jad.2020.05.016
Monteleone, A. M., Marciello, F., Cascino, G., Cimino, M., and Ruzzi, V. (2020). Early traumatic experiences impair the functioning of both components of the endogenous stress response system in adult people with eating disorders. Psychoneuroendocrinology 115:104644. doi: 10.1016/j.psyneuen.2020.104644
Morey, R. A., Dunsmoor, J. E., Haswell, C. C., Brown, V. M., and Vora, A. (2015). Fear learning circuitry is biased toward generalization of fear associations in posttraumatic stress disorder. Transl. Psychiatry 5:e700. doi: 10.1038/tp.2015.196
Nelson, J., Klumparendt, A., Doebler, P., and Ehring, T. (2017). Childhood maltreatment and characteristics of adult depression: meta-analysis. Br. J. Psychiatry 210, 96–104. doi: 10.1192/bjp.bp.115.180752
Nicol, K., Pope, M., Romaniuk, L., and Hall, J. (2015). Childhood trauma, midbrain activation and psychotic symptoms in borderline personality disorder. Transl. Psychiatry 5:e559. doi: 10.1038/tp.2015.53
Nikkheslat, N., McLaughlin, A. P., Hastings, C., Zajkowska, Z., and Nettis, M. A. (2020). Childhood trauma, HPA axis activity and antidepressant response in patients with depression. Brain Behav. Immun. 87, 229–237. doi: 10.1016/j.bbi.2019.11.024
Park, H. J., Friston, K. J., Pae, C., Park, B., and Razi, A. (2018). Dynamic effective connectivity in resting state fMRI. Neuroimage 180(Pt B), 594–608. doi: 10.1016/j.neuroimage.2017.11.033
Petrikova, M., Kascakova, N., Furstova, J., Hasto, J., and Tavel, P. (2021). Validation and adaptation of the slovak version of the Childhood Trauma Questionnaire (CTQ). Int. J. Environ. Res. Public Health 18:2440 doi: 10.3390/ijerph18052440
Qiao, D., Zhang, A., Sun, N., Yang, C., and Li, J. (2020). Altered static and dynamic functional connectivity of habenula associated with suicidal ideation in first-episode, drug-naive patients with major depressive disorder. Front. Psychiatry 11:608197. doi: 10.3389/fpsyt.2020.608197
Salk, R. H., Hyde, J. S., and Abramson, L. Y. (2017). Gender differences in depression in representative national samples: meta-analyses of diagnoses and symptoms. Psychol. Bull. 143, 783–822. doi: 10.1037/bul0000102
Shen, Y., Yao, J., Jiang, X., Zhang, L., and Xu, L. (2015). Sub-hubs of baseline functional brain networks are related to early improvement following two-week pharmacological therapy for major depressive disorder. Hum. Brain Map. 36, 2915–2927. doi: 10.1002/hbm.22817
Sheppes, G., Suri, G., and Gross, J. J. (2015). Emotion regulation and psychopathology. Annu. Rev. Clin. Psychol. 11, 379–405. doi: 10.1146/annurev-clinpsy-032814-112739
Southwick, S. M., Vythilingam, M., and Charney, D. S. (2005). The psychobiology of depression and resilience to stress: implications for prevention and treatment. Annu. Rev. Clin. Psychol. 1, 255–291. doi: 10.1146/annurev.clinpsy.1.102803.143948
Suh, J. S., Schneider, M. A., Minuzzi, L., MacQueen, G. M., and Strother, S. C. (2019). Cortical thickness in major depressive disorder: a systematic review and meta-analysis. Prog. Neuropsychopharmacol. Biol. Psychiatry 88, 287–302. doi: 10.1016/j.pnpbp.2018.08.008
Tomasi, D., and Volkow, N. D. (2010). Functional connectivity density mapping. Proc. Natl. Acad. Sci. U. S. A. 107, 9885–9890. doi: 10.1073/pnas.1001414107
Wang, D., Qin, W., Liu, Y., Zhang, Y., and Jiang, T. (2014). Altered resting-state network connectivity in congenital blind. Hum. Brain Map. 35, 2573–2581. doi: 10.1002/hbm.22350
Wu, Z., Liu, Z., Jiang, Z., Fu, X., and Deng, Q. (2022). Overprotection and overcontrol in childhood: an evaluation on reliability and validity of 33-item expanded Childhood Trauma Questionnaire (CTQ-33), Chinese version. Asian J. Psychiatr. 68:102962. doi: 10.1016/j.ajp.2021.102962
Xie, P., Wu, K., Zheng, Y., Guo, Y., and Yang, Y. (2018). Prevalence of childhood trauma and correlations between childhood trauma, suicidal ideation, and social support in patients with depression, bipolar disorder, and schizophrenia in southern China. J. Affect. Disord. 228, 41–48. doi: 10.1016/j.jad.2017.11.011
Xue, K., Liang, S., Yang, B., Zhu, D., and Xie, Y. (2020). Local dynamic spontaneous brain activity changes in first-episode, treatment-naive patients with major depressive disorder and their associated gene expression profiles. Psychol. Med. [Online ahead of print]. doi: 10.1017/S0033291720003876
Yan, C., Yang, Z., Colcombe, S. J., Zuo, X., and Milham, M. P. (2017). Concordance among indices of intrinsic brain function: insights from inter-individual variation and temporal dynamics. Sci. Bull. 62, 1572–1584. doi: 10.1016/j.scib.2017.09.015
Yan, C. G., Craddock, R. C., Zuo, X. N., Zang, Y. F., and Milham, M. P. (2013). Standardizing the intrinsic brain: towards robust measurement of inter-individual variation in 1000 functional connectomes. Neuroimage 80, 246–262. doi: 10.1016/j.neuroimage.2013.04.081
Yan, C. G., Wang, X. D., Zuo, X. N., and Zang, Y. F. (2016). DPABI: data processing & analysis for (resting-state) brain imaging. Neuroinformatics 14, 339–351. doi: 10.1007/s12021-016-9299-4
Yang, G. J., Murray, J. D., Glasser, M., Pearlson, G. D., and Krystal, J. H. (2017). Altered global signal topography in Schizophrenia. Cereb. Cortex 27, 5156–5169. doi: 10.1093/cercor/bhw297
Yu, M., Linn, K. A., Shinohara, R. T., Oathes, D. J., and Cook, P. A. (2019a). Childhood trauma history is linked to abnormal brain connectivity in major depression. Proc. Natl. Acad. Sci. U. S. A. 116, 8582–8590. doi: 10.1073/pnas.1900801116
Yu, Y., Li, Z., Lin, Y., Yu, J., and Peng, G. (2019b). Depression affects intrinsic brain activity in patients with mild cognitive impairment. Front. Neurosci. 13:1333. doi: 10.3389/fnins.2019.01333
Zang, Y., Jiang, T., Lu, Y., He, Y., and Tian, L. (2004). Regional homogeneity approach to fMRI data analysis. Neuroimage 22, 394–400. doi: 10.1016/j.neuroimage.2003.12.030
Zang, Y. F., He, Y., Zhu, C. Z., Cao, Q. J., and Sui, M. Q. (2007). Altered baseline brain activity in children with ADHD revealed by resting-state functional MRI. Brain Dev. 29, 83–91. doi: 10.1016/j.braindev.2006.07.002
Zhang, H., Tao, Y., Xu, H., Zou, S., and Deng, F. (2022). Associations between childhood chronic stress and dynamic functional connectivity in drug-naive, first-episode adolescent MDD. J. Affect. Disord. 299, 85–92. doi: 10.1016/j.jad.2021.11.050
Zhang, J., Magioncalda, P., Huang, Z., Tan, Z., and Hu, X. (2019). Altered global signal topography and its different regional localization in motor cortex and hippocampus in mania and depression. Schizophr. Bull. 45, 902–910. doi: 10.1093/schbul/sby138
Zhang, L., Zhang, R., Han, S., Womer, F. Y., and Wei, Y. (2021). Three major psychiatric disorders share specific dynamic alterations of intrinsic brain activity. Schizophr. Res. [Online ahead of print]. doi: 10.1016/j.schres.2021.06.014
Zhang, S., Lin, X., Yang, T., Zhang, S., and Pan, Y. (2020). Prevalence of childhood trauma among adults with affective disorder using the Childhood Trauma Questionnaire: a meta-analysis. J. Affect. Disord. 276, 546–554. doi: 10.1016/j.jad.2020.07.001
Zhao, L., Wang, D., Xue, S. W., Tan, Z., and Wang, Y. (2021). Aberrant state-related dynamic amplitude of low-frequency fluctuations of the emotion network in major depressive disorder. J. Psychiatr. Res. 133, 23–31. doi: 10.1016/j.jpsychires.2020.12.003
Zhi, D., Calhoun, V. D., Lv, L., Ma, X., and Ke, Q. (2018). Aberrant dynamic functional network connectivity and graph properties in major depressive disorder. Front. Psychiatry 9:339. doi: 10.3389/fpsyt.2018.00339
Zhou, W., Yuan, Z., Yingliang, D., Chaoyong, X., and Ning, Z. (2021). Differential patterns of dynamic functional connectivity variability in major depressive disorder treated with cognitive behavioral therapy. J. Affect. Disord. 291, 322–328. doi: 10.1016/j.jad.2021.05.017
Zhu, D. M., Yang, Y., Zhang, Y., Wang, C., and Wang, Y. (2020). Cerebellar-cerebral dynamic functional connectivity alterations in major depressive disorder. J. Affect. Disord. 275, 319–328. doi: 10.1016/j.jad.2020.06.062
Zhu, J., Zhang, Y., Zhang, B., Yang, Y., and Wang, Y. (2019). Abnormal coupling among spontaneous brain activity metrics and cognitive deficits in major depressive disorder. J. Affect. Disord. 252, 74–83. doi: 10.1016/j.jad.2019.04.030
Zou, Q. H., Zhu, C. Z., Yang, Y., Zuo, X. N., and Long, X. Y. (2008). An improved approach to detection of amplitude of low-frequency fluctuation (ALFF) for resting-state fMRI: fractional ALFF. J. Neurosci. Methods 172, 137–141. doi: 10.1016/j.jneumeth.2008.04.012
Zuo, X. N., Di Martino, A., Kelly, C., Shehzad, Z. E., and Gee, D. G. (2010a). The oscillating brain: complex and reliable. Neuroimage 49, 1432–1445. doi: 10.1016/j.neuroimage.2009.09.037
Zuo, X. N., Kelly, C., Di Martino, A., Mennes, M., and Margulies, D. S. (2010b). Growing together and growing apart: regional and sex differences in the lifespan developmental trajectories of functional homotopy. J. Neurosci. 30, 15034–15043. doi: 10.1523/JNEUROSCI.2612-10.2010
Keywords: major depressive disorder, childhood trauma, resting-state functional magnetic resonance imaging, concordance, temporal dynamics
Citation: Luo Q, Yu H, Chen J, Lin X, Wu Z, Yao J, Li Y, Wu H and Peng H (2022) Altered Variability and Concordance of Dynamic Resting-State Functional Magnetic Resonance Imaging Indices in Patients With Major Depressive Disorder and Childhood Trauma. Front. Neurosci. 16:852799. doi: 10.3389/fnins.2022.852799
Received: 11 January 2022; Accepted: 18 March 2022;
Published: 09 May 2022.
Edited by:
Feng Liu, Tianjin Medical University General Hospital, ChinaReviewed by:
Yann Quidé, University of New South Wales, AustraliaBochao Cheng, Sichuan University, China
Copyright © 2022 Luo, Yu, Chen, Lin, Wu, Yao, Li, Wu and Peng. This is an open-access article distributed under the terms of the Creative Commons Attribution License (CC BY). The use, distribution or reproduction in other forums is permitted, provided the original author(s) and the copyright owner(s) are credited and that the original publication in this journal is cited, in accordance with accepted academic practice. No use, distribution or reproduction is permitted which does not comply with these terms.
*Correspondence: Huawang Wu, aHVhd2FuZ3d1MUAxNjMuY29t; Hongjun Peng, cGVuZ2RvY3RvcjJAMTYzLmNvbQ==