- 1Department of Psychiatry, The First Affiliated Hospital, Zhejiang University School of Medicine, Hangzhou, China
- 2MOE Frontier Science Center for Brain Science and Brain-Machine Integration, Zhejiang University, Hangzhou, China
- 3Department of Psychiatry, Wenzhou Medical University, Wenzhou, China
- 4The Key Laboratory of Mental Disorder Management in Zhejiang Province, Hangzhou, China
- 5Brain Research Institute, Zhejiang University, Hangzhou, China
- 6Department of Psychiatry, The Melbourne Clinic and St Vincent’s Hospital, University of Melbourne, Richmond, VIC, Australia
Bipolar disorder (BD) is one of the major psychiatric disorders that is characterized by recurrent episodes of depression and mania (or hypomania), leading to seriously adverse outcomes with unclear pathogenesis. There is an underlying relationship between bacterial communities residing in the gut and brain function, which together form the gut-brain axis (GBA). Recent studies have shown that changes in the gut microbiota have been observed in a large number of BD patients, so the axis may play a role in the pathogenesis of BD. This review summarizes briefly the relationship between the GBA and brain function, the composition and changes of gut microbiota in patients with BD, and further explores the potential role of GBA-related pathway in the pathogenesis of BD as well as the limitations in this field at present in order to provide new ideas for the future etiology research and drug development.
Introduction
Bipolar disorder (BD) belongs to the subcategory of mood disorders, characterized by repeated episodes of major depression (lasting at least for 2 weeks) and mania (or hypomania), which subtypes include Bipolar type I and type II, the difference between which mainly lies in the severity of mania, and obvious differences in treatment and prognosis are also noticed. Late adolescence is the average age at which BD develops (Bauer et al., 2018). Epidemiological statistics showed that the prevalence of BD was about 2% (Merikangas et al., 2011), and the risk of mortality rate due to suicide, which partly attributed to the mood states of BD patients, was 20–30 times higher than that of the normal (Pompili et al., 2013; Orsolini et al., 2016; Fornaro et al., 2018; De Berardis et al., 2021). The early diagnosis of BD is difficult, and the final clinical diagnosis is usually made 5–10 years after the recurrence of the initial symptoms (Fortinguerra et al., 2019). Poor prognosis is another feature of BD, while cognitive dysfunction and poor social regression can be observed in many patients with chronic BD (Passos et al., 2016). However, the pathogenesis of BD has not been fully and clearly elucidated.
Enormous number of microbial communities in the intestinal tract form a symbiotic relationship with the host, contributing to the maintenance of homeostasis and potentially influencing the function of other organs (Sen et al., 2021), due to the ability of gut microbiota metabolites to act on the host neurotransmitter system (Ogawa et al., 2020), vagal reflex (Zhang et al., 2021) and immunoreaction (Haack et al., 2007). For example, the improvement of sleep quality (Marotta et al., 2019; Sen et al., 2021) can also be achieved through the application of intestinal microbiota or fecal flora transplantation. In addition, soluble CD14 (sCD14) in the serum indicates ectopic intestinal bacteria. The serum level of this marker was significantly increased in patients with a variety of diseases mainly characterized by brain dysfunction, such as schizophrenia, depression, and BD (Nguyen et al., 2018). These results suggest that the close relationship between intestinal microecology and brain function, which was called the “gut-brain” axis (GBA).
In fact, preliminary progress has been made on the link between intestinal microbiome homeostasis and the onset of BD. Karling et al. (2016) conducted a retrospective study of gastrointestinal symptoms in 136 patients with BD, and found a positive correlation between depressive symptoms and the occurrence rate of gastrointestinal symptoms. In a more recent study, Kao et al. (2019) found that patients with inflammatory bowel disease (IBD) were more likely to develop BD. This is in accordance with findings by Kostic et al. (2014). Although the above studies could not clarify the specific causal association, we can speculate that intestinal factors may play a certain role in the pathogenesis of BD.
In this review, we systematically review previous relevant studies and attempt to establish the association between the GBA and BD from the perspectives of neuroinflammation, molecular biology and neuropathology. We hope this work will provide evidence for future research in related fields.
The Gut-Brain Axis: Why Choosing It?
It is well known that the human microbiome is vast. The microbes and their gene community together constitute the microbiome that maintains the physiological homeostasis of the organism, in which bacteria are the main component, and fungi, viruses and other mainly parasitic eukaryotic microorganisms are also included (Nguyen et al., 2018; Zhang et al., 2019). These microbes live in different parts of the body, including the skin, mouth and intestines (Costello et al., 2009). Colonization of gut microbes occurs during childbirth and is perfected during infancy (Dominguez-Bello et al., 2010). The importance of the microbiome has been gradually discovered through high-throughput sequencing techniques (Qin et al., 2010; Knight et al., 2017). The link between gut microbes and the development of the immune system was first proposed more than a decade ago (Round and Mazmanian, 2009). Subsequently, a number of studies have shown that changes in intestinal microbial composition and metabolic function can trigger a series of pathophysiological changes, such as activation of focal inflammatory responses and oxidative stress mechanisms (Kamada et al., 2013). Surprisingly, similar inflammatory changes were observed in psychiatric disorders represented by schizophrenia and BD (Kirkpatrick and Miller, 2013). These evidences link intestinal microorganisms with changes in physiological functions of the whole body, thus providing possible ideas for the etiological research of many diseases whose basic pathological mechanism is macro or micro inflammatory responses.
Theoretically, GBA contains multiple systems, i.e., nervous system, immune system, neuroendocrine system, etc. Microbial metabolism plays a role in initiating and communicating various systems in the GBA (Figure 1).
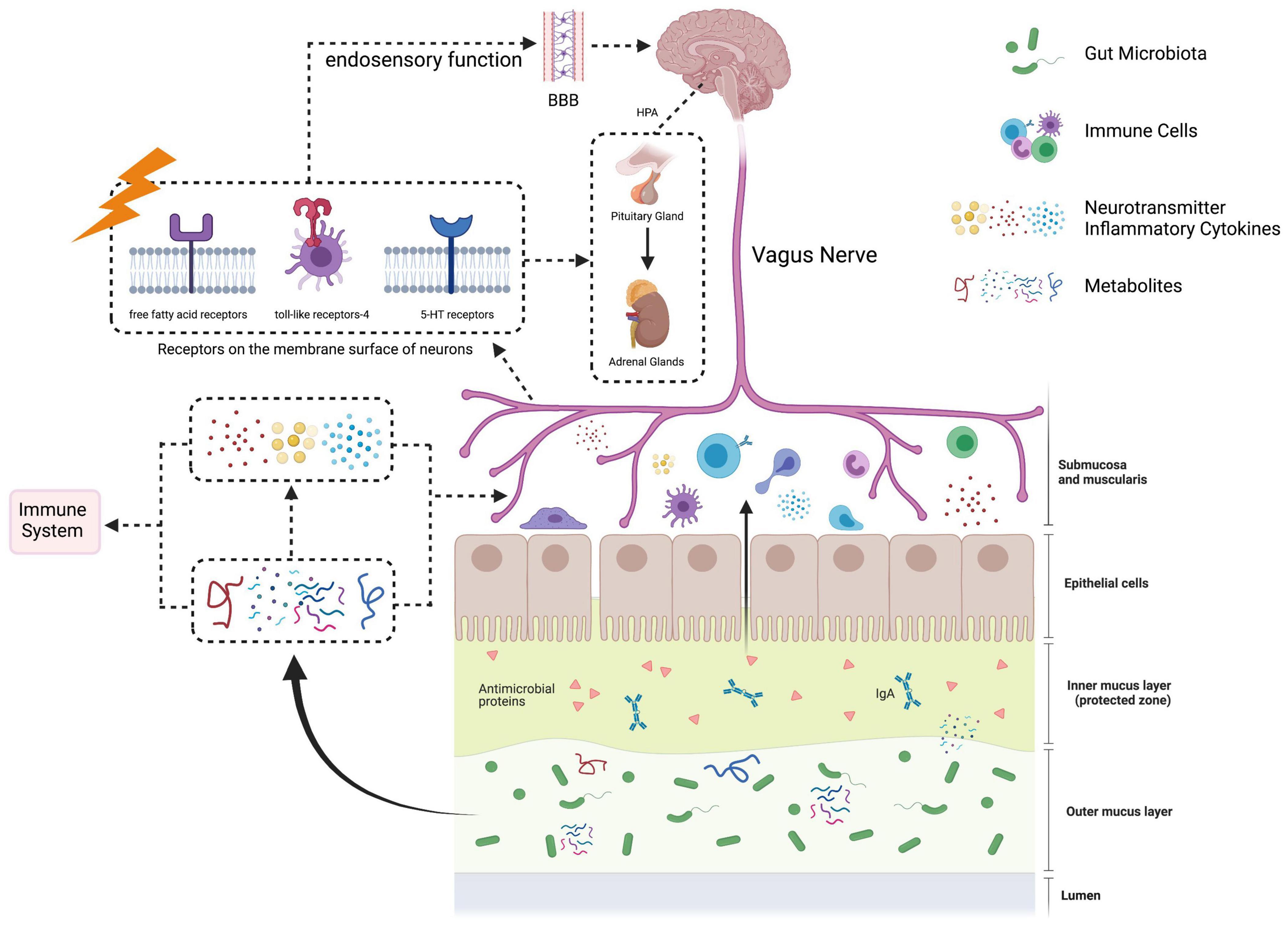
Figure 1. The paradigm of gut-brain axis. The gut-brain axis, referring to the signals’ bidirectional communication between gut microbiota and the brain, is mediated by neuronal, immune, and neuroendocrine pathways. In the gastrointestinal tract, certain gut microbiota can produce neuroactive substances, such as dopamine, serotine and short-chain fatty acids. Signals from abnormal gut microbiota and its metabolites can be sensed and transported by afferent fibers of vagus nerve into the brain. In addition, the integrity of gut barrier is disrupted to form the “leaky gut,” which facilitates gut microbiota-derived components and metabolites to enter the circulatory system. These substances can directly or indirectly activate the immune system to release inflammatory factors. Under the state of immune dysfunction, the permeability of the blood–brain barrier (BBB) is increased. Inflammatory factors and neuroactive substances produced by gut microbiota go through the BBB, leading to neuroinflammatory, activating the hypothalamus-pituitary-adrenal (HPA) axis and disrupting brain functions.
Neuronal Pathways: The Mysterious Vagus
A growing number of studies suggest there may be a “potential” link between the brain and the gut. For example, Sherwin et al. (2019) have proposed that brain development is subject to microbiome changes – disturbances in the local microenvironment during the early maturation of the gut microbiome may lead to defects in neurogenesis, axon and dendrite growth, which may have an impact on early brain development, and even the entire life cycle of the body. What we need to know is that the gut microbiome, even though it can be thought of as a microbiome, is not uniform in time and space (Fulling et al., 2019). This results in differences in the composition of microbes in different parts of the gut, as well as metabolites, including sugars, short-chain fatty acids (SCFAs), and neuroactive substances such as serotonin (5-HT) and γ-aminobutyric acid (GABA) (Fulling et al., 2019). These metabolites can act on the local nervous system of the intestinal tract, of which the vagus nerve is the most widely studied.
Vagus nerve is the most important plant nerve controlling gastrointestinal function. Vagovagal reflex can inhibit gastric empty and promote the secretion of gastrointestinal glands, thus regulating the digestion and absorption function of the gastrointestinal tract. There are a large number of vagus nerve endings in the submucosa and muscularis of the intestinal mucosa, and these nerve endings account for about 90% of the total vagus nerve endings (Fulling et al., 2019). Meanwhile, the vagus nerve can also transmit information from the intestinal tract and other peripheral areas to the cerebral cortex, amygdala and hippocampus, thus playing a role in memory, emotion and cognition regulation (Wang et al., 2021). Bonaz et al. (2018) made an analysis on the vagus nerve and brain function, and they found that this plant nerve was capable of directly sensing neural activity signals from intestinal flora metabolites through the endosensory function of the afferent nerve and 5-HT receptors, toll-like receptors (TLR) 4, and free fatty acid receptors distributed on the surface of the vagus nerve, after which it sent afferent signals to the brain. After cutting the vagus nerve of the experimental animals, the researchers found that the effects of Lactobacillus rhamnosus JB-1 against anxious behavior and elevated GABA receptor expression were reduced (Bravo et al., 2011). Additionally, nerve fiber connections between the gut vagus nerve and dopamine reward pathways in the striatum of the basal ganglia have been demonstrated in previous experiments (Han et al., 2018). Functional neuroimaging studies have shown that functional changes in the dopamine reward pathway may be the cause of some mental illnesses, such as substance addiction (Lindgren et al., 2018), mood disorders (Serafini et al., 2020), and eating disorders (Lindgren et al., 2018). We speculate that stimulation of the vagus nerve may be able to “awaken” the dopamine reward pathway to achieve intervention in these illnesses (Han et al., 2018).
Immune System: Neuroinflammation and Immuno-Response
Neuroinflammation and immune responses have been observed in a variety of mental illnesses, such as depression (Troubat et al., 2021), BD (Benedetti et al., 2020), and schizophrenia (Buckley, 2019). In these common psychiatric disorders, the inflammatory response is mainly reflected in the activation of immune cells in the nervous system dominated by microglia, which leads to the release of a series of pro-inflammatory and anti-inflammatory cytokines (Kim and de Vellis, 2005; Beurel et al., 2020). Long-term chronic inflammation will change the microenvironment around neurons, leading to a series of adverse consequences, such as neuron damage, impaired cell signal transduction mechanism and neurotransmitter reuptake dysfunction (Stephan et al., 2012). These subtle changes exacerbated brain dysfunction. In short, inflammation is a double-edged sword. Failure to achieve appropriate balance can lead to unpredictable damage.
Neuroimmune response can be modulated by many variables, among which the role of intestinal microbes cannot be ignored. The role of intestinal microbes in immune regulation can be roughly divided into immune cells and cytokines. Intestinal microorganisms mainly regulate T-line immune cells, including Th-1, Th-2, Th-17, and Treg, etc. In an animal study of symbiotic regulation, Ivanov et al. (2009) found that mice colonized by segmented filamentous bacterium in the lamella propria of terminal ileum mucosa produced immune effector TH-17 cells and CD4 (+) helper T cells. The same results were found in the experiments of Lee et al. (2020). Meanwhile, mice fed with Bacteroidetes fragilis polysaccharides elicited a specific Th-1 cell response (Jakobsson et al., 2014), while Bifidobacterium inhibited the action of Th-2 cells (Fang et al., 2020). Other studies have found that the differentiation process of Treg cells is affected by a variety of factors. Differentiation of Treg cells was inhibited in the mesenteric lymph nodes of mice that carried Akkermansia muciniphila and Acinetobacter calcoaceticus (intestinal flora transplants from multiple sclerosis patients) in their intestines (Berer et al., 2017).
In the central nervous system (CNS), microglia play a similar role to that of macrophages in peripheral tissues, namely non-specific immune recognition and phagocytosis. Microglia have been found to make up about 5–10% of all brain cells (Kim and de Vellis, 2005). These cells can proliferate and differentiate under the action of various stimulus factors, and produce cytokines and chemokines, thus mediating neuroinflammation and immune response, participating in maintaining synaptic plasticity (Yirmiya et al., 2015), and playing a role in neuronal network regulation and damage repair (Lenz and Nelson, 2018). A variety of factors can affect microglia homeostasis. Erny et al. (2015) treated germ-free (GF) mice with SCFAs produced by microbial fermentation, and individuals originally deficient in microglia function showed partial restoration of microglia function, whereas those changes were not observed in individuals with SCFAs receptor gene (FFAR2) defects. It suggests that the symbiotic microbiome can play a regulatory role in the function of microglia, that is, the complexity of the effective microbiome can indirectly affect the immune status of CNS. This conclusion provides a scientific basis for establishing the GBA functional model.
The TLR is a series of cell receptors expressed in neurons, glial cells and non-specific immune cells, and plays an important role in the immune system’s recognition of bacterial lipopolysaccharides. Intestinal microbes activate TLR, which in turn promotes the release of a series of cytokines (e.g., IL-1, IL-6, TNF-α, etc.) that can cross the blood–brain barrier (BBB), thus affecting the function of CNS (Lucidi et al., 2021). BBB originally had a certain permeability to cytokines such as TNF-α. While in GF mice, this permeability is significantly increased, resulting in significantly elevated levels of cytokines in the cerebrospinal fluid (CSF), leading to a series of CNS inflammation, and ultimately lead to dysfunction of neural pathways (Braniste et al., 2014). In the peripheral immune system, various circulating cytokines can also be regulated by intestinal flora and its metabolites. The relative abundance of Blautia could affect plasma IFN-γ levels, and there was a negative correlation between them. With the increase of Pseudomonas, Streptomyces, Clostridium and Bacillus abundance, IFN-γ levels also increased (Cao et al., 2021).
Neuroendocrine and Metabolic Pathways: Associated With Emotion
The regulation of emotion is influenced by many factors. Among these factors, neurotransmitters are the most widely studied. The role of GABA is mainly inhibitory, while glutamate and dopamine can promote the production of positive emotions, which has almost become a consensus in psychiatry. In previous studies of brain neurotransmitters, high concentrations of GABA in the synaptic cleft and decreased sensitivity of 5-HT receptor was associated with depression (depressive symptoms of BD, and other mood disorders as well) (Mahar et al., 2014). The abnormal release of dopamine can lead to mental disorders characterized by emotional overactivity, such as schizophrenia (Howes et al., 2017) and anxiety disorder (Sun et al., 2019). In addition, the excitatory toxicity mechanism of quinolinic acid which is reasonable for affecting synaptic plasticity has been found (Potter et al., 2010). Kynurenine can be further metabolized through the kynurenic acid and quinolinic acid pathways. Kynurenic acid has a neuroprotective effect against N-methyl-D-aspartate receptor antagonists, but high concentrations of quinolinic acid can lead to synaptic dysfunction, which is associated with cognitive deficits (Kadriu et al., 2019).
Gut microbes produce some neurotransmitters directly or indirectly. For example, Bifidobacterium and Lactobacillus are able to produce GABA (Patterson et al., 2019), which contributes to inhibitory changes in brain function. While Lactobacillus, Oscillibacter, and Blautia can promote the synthesis of 5-HT by increasing the expression level of tryptophan synthase gene (Busnelli et al., 2019; Chen et al., 2019). In fact, about 95% of 5-HT is derived from this synthetic pathway (Yano et al., 2015). Although we present the neurotransmitter here as a separate component, its changes are in fact the result of a combination of factors, including vagus nerve signaling stimulation, as well as inflammation and immune responses. Meanwhile, gut microbes can interfere with the body’s immune function. Some gut microbes (usually named “probiotics”) produce SCFAs (LeBlanc et al., 2017), which affect the immune function of the intestinal mucosa (Correa-Oliveira et al., 2016). Alternatively, activation of afferent signals in the vagus nerve via G-protein-coupled receptors (Nohr et al., 2015) or histone deacetylases (Waldecker et al., 2008) may affect the functions of macrophages, dendritic cells, monocytes and neutrophils, and affects the recruitment and differentiation of T cells (Correa-Oliveira et al., 2016). This pathway is mediated by immune cells and therefore ultimately comes down to changes in the body’s immune function. When the body’s immune function is impaired, the metabolism of kynurenine is accelerated, which changes the efficiency of GABA and dopamine secretion (Schwarcz and Stone, 2017). This is another way that the intestinal flora affects the levels of neurotransmitters in the brain.
Gut-Brain Axis and Bipolar Disorder: Potential Connections
Alterations of Gut Microbiota in Bipolar Disorder
As an important part of affective disorders, the relationship between BD and GBA has always been the focus of research. We summarized the existing studies and showed relevant research results in Table 1. The searched strategy in this review was consistent with the Preferred Reporting Items for Systematic Reviews and Meta-analyses (PRISMA). Relevant studies were searched via PubMed, EMBASE and Cochrane databases before December 01, 2021. The selected keywords were (“bipolar disorder” AND “gut microbiota”). We also searched the relevant articles, manually. Studies eligible for inclusion needed to be further filtered by human studies and original research papers, rather than reviews, letters, or meeting abstracts. Duplicate records were removed followed by titles and abstracts screening, as well as the full article. The process of this study selection is shown in Figure 2. Eventually, 16 articles on the gut microbiota in BD were included.
Alpha diversity is a comprehensive measure that assesses the richness and evenness of species. In general, the alpha diversity of intestinal flora in BD patients showed a decreasing trend (Hu et al., 2019). The alpha diversity of intestinal flora decreased with the increase of the methylation ratio of a clock gene, ARNTL (Bengesser et al., 2019). A longitudinal case study of twins with BD suggests that increased alpha diversity of gut flora may be associated with improved depressive symptoms (Vinberg et al., 2019). Generally speaking, changes in the composition of intestinal flora or metabolic capacity are associated with the severity of BD symptoms, the selection of therapeutic agents, and the evaluation of therapeutic effects. More specifically, changes in gut microbiota composition were found in patients with BD, and it is believed to be associated with the development of BD. A number of studies on BD and intestinal flora have found that the abundance of one of the butyrate-producing bacteria – Faecalibacterium – is observed to decrease in BD patients with acute mood changes, while the above indicators increase again when the mood is softened (Hu et al., 2019; Painold et al., 2019). Other butyric acid producing bacteria, such as Roseburia and Coprococcus, were also at low levels in BD patients, suggesting that the reduction of this beneficial bacteria may be the cause of the disorder (Hu et al., 2019). Lu et al. (2019) sampled and analyzed the intestinal flora of BD patients before and after quetiapine treatment, and found that the proportion of Bifidobacteria and Enterobacteriaceae increased. BD patients treated with probiotics showed a significant reduction in symptoms and improvement in cognitive function (Aizawa et al., 2018; Reininghaus et al., 2018).
Pathways That the Gut-Brain Axis Mediates in Bipolar Disorder
Previous research results show that the pathogenesis of BD is complicated. Under the combined action of a variety of internal and external factors (such as genetic susceptibility, external environmental stimulation and homeostasis disorders, etc.), the characteristic clinical manifestations and auxiliary examination abnormalities of BD are finally formed. Among different proposed mechanisms for BD, the role of GBA is gradually recognized by many researchers, who believe that studies on the GBA-related pathways may help to clarify the pathogenesis of BD.
The BD is genetically hereditable. A paper published in 2018 showed that 85% of BD cases can be inherited in families (Vieta et al., 2018). Goodrich et al. (2014) suggested that this genetic trait might be related to the interaction between genetic variations and gut microbes. Genetic phenotypes cannot only affect the composition of intestinal microbes, but also affect the metabolic pattern of the body through microbes. This raises a whole new question – can genetic traits link gut microbes to BD? A genome-wide association study was conducted to evaluate the gene-microbiome correlation in BD patients, and the results showed that the increased level of Desulfovibrio community was associated with the genetic characteristics of the host, and both showed a significant correlation with BD (Cheng et al., 2020). In addition, in a study of BD patients with identical twins, Jiang et al. (2019) found that compared with healthy partners, the intestinal microbiota spectrum of BD patients with identical twins was more different. After complete remission of BD, this difference was significantly reduced. Thus, we can speculate that gut microbes play a role in the formation of BD phenotypes.
The conjecture about the connection between vagus nerve and brain function has been explained above. Generally speaking, we need to pay attention to the relationship between the vagus nerve and the intestinal flora, and the effects of the vagus nerve on the onset of BD. There is increasing evidence that the vagus nerve may be a “bridge” between GBA and brain function. Based on this theory, a new type of physical therapy has emerged in recent years. Vagus nerve stimulation – the use of electrodes to stimulate the vagus nerve in the neck – was initially used to control acute seizures and shorten the duration of drug treatment. Now, this surgery has achieved some remarkable results in improving the clinical symptoms of BD patients (McAllister-Williams et al., 2020). In addition, a preclinical study showed that the depressive-like phenotype in experimental mice was related to an overactivation of the vagus nerve (Wang et al., 2020). The gut microbiota of the mice was artificially altered by a diet containing Lactobacillus intestinalis and Lactobacillus reuteri. However, despite these evidences, the specific relationship between vagus nerve and GBA remains to be confirmed by further experiments.
As for the pathophysiological mechanism of BD, existing studies mainly focus on the neurotransmitter imbalance (Newberg et al., 2008). The formation and regulation of emotion and cognitive function is complicated and tedious. The homeostasis of excitatory and inhibitory neurotransmitters and their interactions all play an important role. Previous studies have suggested that the dopamine system (mesencephalon-limbic system, mesencephalon-cortical system, substantia nigra-striatum system, and nodular-funnel system) and reward circuits may be involved in the manic symptoms of schizophrenia and BD (Berk et al., 2007), while executive function relies on the GABA neurotransmitter (Huber et al., 2018). Meanwhile, studies on BD also show that an improvement of the efficiency in 5-HT transmission is beneficial to alleviate the severity and duration of BD depressive symptoms (Ananth et al., 2020). As mentioned above, the production of these neurotransmitters also depends on intestinal microbes. Therefore, intervention in the composition or metabolic function of intestinal microbes may become a new approach for the treatment of mental diseases represented by BD. In addition to the common neurotransmitters, some neuroactive substances produced by gut microbes can influence the concentration or delivery efficiency of neurotransmitters. For example, kynurenine is produced by the fermentation of tryptophan, a neuroactive substance that inhibits 5-HT synthesis (O’Mahony et al., 2015). Hydroxykynurenine is neurotoxic, and accumulation of this substance may lead to neurotoxic reactions that intervene neurotransmitter function (Bartoli et al., 2020).
The GBA contains multiple pathways with potential for interaction. For example, short-chain fatty acids can alter neurotransmitter levels, activate the vagus nerve, and trigger an immune response via activation of T cells and macrophages (Dalile et al., 2019). The metabolism of kynurenine is influenced by immune function, and abnormal metabolism can affect the secretion of neurotransmitters such as dopamine and GABA (Schwarcz and Stone, 2017). In summary, the occurrence of BD is closely related to the GBA, which is in line with the multi-factor hypothesis of the pathogenesis of BD, namely, functional abnormalities of the monoamine neurotransmitter system and immune system, and changes in connections between neurons (Vieta E). Given the lack of current research results, it is still a new way to clarify the pathogenesis of BD from the GBA perspective and develop new anti-BD drugs that interfere with intestinal microorganisms.
Therapies Connecting Gut Microbiata and Bipolar Disorder
In view of the high prevalence of BD, traditional treatment methods for BD include mood stabilizers (carbamazepine, lithium carbonate, etc.), atypical antipsychotics (quetiapine, olanzapine, etc.), traditional antidepressants (SSRIs, SNRIs, etc.), psychotherapy, and peer support (McCormick et al., 2015). Although existing treatment methods and clinical care are more or less effective for not a few patients, the prognosis and the social burden caused by BD still should not be underestimated (Dilsaver, 2011). Management of BD patients is a major challenge for healthcare professionals. One reason for this result is that there is still a significant proportion of patients who have not achieved complete remission and functional recovery. These patients may experience relapse or aggravation of the disease, lack of social support, adverse effects of medications and other adverse outcomes directly or indirectly caused by BD. Given the association between the GBA and BD, we speculate that regulation of gut microbiome may be an attractive and promising treatment for BD.
Probiotics and Prebiotics
Probiotics are defined as beneficial living bacteria, and prebiotics contain non-digestible fibers which can enhance the probiotics’ function. Particularly, some probiotics are called “psychobiotics,” and the term refers to their effects on mental illnesses (Barbosa and Vieira-Coelho, 2020). Animal studies indicated that administration of probiotics could upregulate neuronal and synaptic connections and exert anti-depressive effects (Capuco et al., 2020). A clinical study showed that the severity of both depression and mania was reduced after 8 weeks’ probiotic supplement in patients with type I BD, which proved the benefits showed by that kind of “psychobiotics” (Eslami Shahrbabaki et al., 2020). Dickerson et al. (2018) reported remission in manic symptoms and reduction in rehospitalization rate after treatment with Bifidobacterium and Lactobacillus, especially in BD patients with manic episodes and high baseline levels of systemic inflammation. Cognitive impairments may persist in euthymic periods of BD and thus result in low quality of life. Reininghaus et al. (2018) found that the attention and executive functions were enhanced after 3-month treatment with probiotic in 20 individuals with euthymic BD, indicating that probiotics supplement could improve the cognition dysfunctions. These preliminary data support that prebiotics and probiotics were beneficial to regulate the mood and behaviors and may be promising treatment strategies for BD. In spite of these findings, more efforts are needed before the clinical application of psychobiotics because the proper dosages, duration and adverse effects are still needing of robust evidence.
Diet
As one of the bodily systems interacting with foods, manipulation of gut microbiota via dietary adjustment has garnered much attention. Mediterranean diet (MD) emphasizes a balanced consumption of fruits, vegetables, legumes, unsaturated fats with limited red meat intake rather than excluding any particular or specific food. MD could enhance the abundance of Prevotella and SCFAs producing bacteria such as Faecalibacterium and Clostridium whereas decrease the phylum Bacteroides (Jin et al., 2019). Researches have shown that MD was correlated with improvement of cognitive functions and emerged as an accessible strategy to manage depressive syndromes (Nagpal et al., 2019). Dietary interventions enriched in omega-3 polyunsaturated fatty acids can improve the abundance of beneficial bacteria, such as Bifidobacterium and Lactobacillus, and dampen the HPA-axis activity in mice (Robertson et al., 2017). Mice fed with a high-fat diet elevated the Firmicutes-Bacteroidetes ratio and displayed anxiety-like behaviors (Marx et al., 2021). In general, dietary patterns are considered as influential factors in shaping gut microbiota and present as potential treatment targets in mediating brain health.
Exercise
Regular exercise offers a strategy to regulate physiological responses including the inhabiting of gut microbiota. Compared to individuals who always keep sedentary, the diversity of microbiota in professional athletes was elevated and the profile of beneficial metabolites especially SCFAs was enriched (Barton et al., 2018). In addition to the altered β diversity of gut microbiota, Allen et al. (2018) also found that SCFAs were increased through exercise intervention, especially in individuals with a lean body. In marathon athletes, increased abundance was observed in Veillonella atypica, which is a genus that can metabolize lactate into propionate, a sort of SCFAs (Scheiman et al., 2019). At present, SCFAs have been regarded as a mediator in the gut and brain due to their neuroactive, anti-inflammatory role and beneficial effects on brain health. Therefore, we can speculate that exercise can also be an approach to modulate the composition and metabolic capacity of gut microbiota in BD individuals.
Fecal Microbiota Transplantation
Fecal microbiota transplantation (FMT), a direct method to manipulate the microbiota, refers to transfer the stool samples from a donor to a recipient, in order to observe effects caused by the factors lying in the stool of the donor (Dabke et al., 2019). In patients with Clostridium difficile infection, FMT has been proved to exert therapeutic effects through shaping the gut microbiota (Hvas et al., 2019). Up to now, there has been one clinical study depicting FMT in BD. A woman diagnosed with BD went through FMT from her healthy husband at least nine times. Interestingly, she became free from manic or depressive symptoms in the sixth month and had a weight loss of nearly 33 kg (Hinton, 2020). These positive results provide hope that FMT would be an emerging therapy for abnormal neurologic and behavioral conditions, though many difficulties and issues affecting donors and recipients still exist.
In summary, preliminary progress has been made in the treatment of BD based on intestinal microbiome and the GBA. However, due to the uncertainty of the specific mechanism of the axis, new therapeutic methods are still in the stage of exploration. Encouragingly, a growing number of clinical experimental therapies are showing advantages over traditional BD therapies, such as the avoidance of adverse drug reactions, significantly shorter response times, and improved patient compliance. It suggests that with the continuous clarification of various mechanisms in the GBA pathway, the treatment based on intestinal microbiome may replace the traditional treatments in the future, becoming an alternative choice for BD patients.
Discussion
There are many causes leading to BD via a complex interaction of various factors. Abundant experimental evidence suggests that elucidating the mechanism of GBA will help to explain the etiology of BD. There is an obvious correlation between the changes of intestinal microbiome and the pathogenesis and clinical manifestations of BD – truly a breakthrough, since it may open up a new idea for the diagnosis and management of BD.
This review focuses on the possible mechanism of GBA, etiology and susceptibility factors of BD, and summarizes recent research conclusions on the relationship between the GBA and BD. Through the combination of sequencing technology and bioinformatics analysis, it is very important to identify the functional genes of specific microorganisms and their encoding enzymes involved in metabolic pathways, which is the fundamental work in this field. At present, the most surprising discovery lies in that, on the one hand, the composition of intestinal microbe or metabolic function of BD patients is different from that of healthy individuals, and these changes are more obvious in acute mood episodes (Painold et al., 2019; Vinberg et al., 2019). On the other hand, artificially altering the gut microbiome (e.g., using probiotics, or changing the composition of the feed) of subjects or laboratory animals can lead to secondary changes in brain functions (Wang et al., 2020). However, these observational results do not determine any change that is specially linked to certain microbe, since individual heterogeneity cannot be ruled out. Under different living habits and demographic background, the composition ratio of intestinal flora varies among different individuals. In addition, there are still many microorganisms whose metabolic mechanisms have not been thoroughly studied, so conclusions cannot be drawn arbitrarily.
Based on the above conclusions, some treatments for BD specific to the GBA have been found. Compared to traditional treatments, these new treatments focus on gut microbes, which are treated in a gentler and more patient-friendly way, through probiotics, diet, exercise and FMT. This, to some extent, alleviates the problem of reduced patient compliance caused by adverse reactions to psychotropic drugs. In fact, traditional BD medications such as mood stabilizers, antidepressants, and antipsychotics are “symptomatic treatment” rather than “causal treatment,” This is because most of the mechanisms of action of these drugs involve direct intervention of brain function via the neurotransmitter system – which is why these drugs (especially mood stabilizers and antipsychotics) are commonly used for the treatment of acute mood episodes. The treatment based on intestinal microbiome is worthy of explorations, because there has been more and more evidence suggesting that the disorder of intestinal microenvironment is one of the causes of BD.
Certainly, studies of the relationship between gut microbiota in BD patients are still at a juvenile stage up to now. Considering the cross-sectional research shortcoming, the knowledge gap about the dynamic alterations of gut microbiota in BD patients need to be filled through longitudinal cohorts. When it comes to the impact factors on gut microbiota, and medication is not able to be neglected. However, most individuals are currently treated with medication prior to recruitment. Thus, the randomized controlled trials would be undertaken to eliminate confounding factors. At the same time, the complex of gut microbiota is obvious. In addition to bacteria, the intestinal microbiome also contains fungi, virus, and bacteriophages. The relationship among gut microbiota and metabolomes, peptidomes, transcriptomics and brain function, etc., is yet unknown. Therefore, the combined analysis of multi-omics provides greater insight into gut microbiota framework. Despite lack of animal models close to humans, pre-clinical experiments are still crucial to identify the specific mechanism of gut microbiota in BD.
Conclusion
The BD is a mental illness caused by a variety of factors. It is characterized by hidden early symptoms, long course of disease and poor prognosis in severe cases. Research on the etiology of BD has always been a hot issue in psychiatry. In recent years, important advances have been made in the biological role of gut microbiota in BD. Existing experiments provide evidence that one of the etiologies of BD is the disturbance of intestinal ecosystem, and the structural basis of the association between BD and the GBA. The periodic fluctuation of BD patients’ mood is believed to be related to gut microbiota, which produces a series of metabolites that can affect brain function through vagus nerve, immune system and other pathways. Therefore, treatment targeting gut microbiota may be a potential adjunctive therapy for BD. But the research is still in its infancy, and the available evidence is still insufficient for clinical application. It is necessary to further determine the types of gut microbiota and the specific mechanism of action in BD, and develop more feasible treatment methods in the future.
Author Contributions
PZ and SH: conceptualization. PZ and LK: writing—original draft preparation. LK: visualization. HH, YP, DZ, JJ, CX, and YS: literature. JL, CN, and SH: writing—review and editing. JL and SH: funding acquisition. All authors have read and agreed to the published version of the manuscript.
Funding
This work was supported by the National Natural Science Foundation of China (No. 81971271), the Zhejiang Provincial Key Research and Development Program (No. 2021C03107), the Zhejiang Provincial Natural Science Foundation (No. LQ20H090013), and the Program from the Health and Family Planning Commission of Zhejiang Province (No. 2020KY548).
Conflict of Interest
The authors declare that the research was conducted in the absence of any commercial or financial relationships that could be construed as a potential conflict of interest.
Publisher’s Note
All claims expressed in this article are solely those of the authors and do not necessarily represent those of their affiliated organizations, or those of the publisher, the editors and the reviewers. Any product that may be evaluated in this article, or claim that may be made by its manufacturer, is not guaranteed or endorsed by the publisher.
References
Aizawa, E., Tsuji, H., Asahara, T., Takahashi, T., Teraishi, T., Yoshida, S., et al. (2018). Bifidobacterium and Lactobacillus Counts in the Gut Microbiota of Patients With Bipolar Disorder and Healthy Controls. Front. Psychiatry 9:730. doi: 10.3389/fpsyt.2018.00730
Allen, J. M., Mailing, L. J., Niemiro, G. M., Moore, R., Cook, M. D., White, B. A., et al. (2018). Exercise Alters Gut Microbiota Composition and Function in Lean and Obese Humans. Med. Sci. Sports Exerc. 50, 747–757. doi: 10.1249/MSS.0000000000001495
Ananth, M., Bartlett, E. A., DeLorenzo, C., Lin, X., Kunkel, L., Vadhan, N. P., et al. (2020). Prediction of lithium treatment response in bipolar depression using 5-HTT and 5-HT1A PET. Eur. J. Nucl. Med. Mol. Imaging 47, 2417–2428. doi: 10.1007/s00259-020-04681-6
Barbosa, R. S. D., and Vieira-Coelho, M. A. (2020). Probiotics and prebiotics: focus on psychiatric disorders - a systematic review. Nutr. Rev. 78, 437–450. doi: 10.1093/nutrit/nuz080
Bartoli, F., Misiak, B., Callovini, T., Cavaleri, D., Cioni, R. M., Crocamo, C., et al. (2020). The kynurenine pathway in bipolar disorder: a meta-analysis on the peripheral blood levels of tryptophan and related metabolites. Mol. Psychiatry 2020:1. doi: 10.1038/s41380-020-00913-1
Barton, W., Penney, N. C., Cronin, O., Garcia-Perez, I., Molloy, M. G., Holmes, E., et al. (2018). The microbiome of professional athletes differs from that of more sedentary subjects in composition and particularly at the functional metabolic level. Gut 67, 625–633. doi: 10.1136/gutjnl-2016-313627
Bauer, M., Andreassen, O. A., Geddes, J. R., Vedel Kessing, L., Lewitzka, U., Schulze, T. G., et al. (2018). Areas of uncertainties and unmet needs in bipolar disorders: clinical and research perspectives. Lancet Psychiat. 5, 930–939. doi: 10.1016/S2215-0366(18)30253-0
Benedetti, F., Aggio, V., Pratesi, M. L., Greco, G., and Furlan, R. (2020). Neuroinflammation in Bipolar Depression. Front. Psychiat. 11:71. doi: 10.3389/fpsyt.2020.00071
Bengesser, S. A., Morkl, S., Painold, A., Dalkner, N., Birner, A., Fellendorf, F. T., et al. (2019). Epigenetics of the molecular clock and bacterial diversity in bipolar disorder. Psychoneuroendocrinology 101, 160–166. doi: 10.1016/j.psyneuen.2018.11.009
Berer, K., Gerdes, L. A., Cekanaviciute, E., Jia, X., Xiao, L., Xia, Z., et al. (2017). Gut microbiota from multiple sclerosis patients enables spontaneous autoimmune encephalomyelitis in mice. Proc. Natl. Acad. Sci. U S A. 114, 10719–10724. doi: 10.1073/pnas.1711233114
Berk, M., Dodd, S., Kauer-Sant’anna, M., Malhi, G. S., Bourin, M., Kapczinski, F., et al. (2007). Dopamine dysregulation syndrome: implications for a dopamine hypothesis of bipolar disorder. Acta Psychiatr. Scand. Suppl. 2007, 41–49. doi: 10.1111/j.1600-0447.2007.01058.x
Beurel, E., Toups, M., and Nemeroff, C. B. (2020). The Bidirectional Relationship of Depression and Inflammation: Double Trouble. Neuron 107, 234–256. doi: 10.1016/j.neuron.2020.06.002
Bonaz, B., Bazin, T., and Pellissier, S. (2018). The Vagus Nerve at the Interface of the Microbiota-Gut-Brain Axis. Front. Neurosci. 12:49. doi: 10.3389/fnins.2018.00049
Braniste, V., Al-Asmakh, M., Kowal, C., Anuar, F., Abbaspour, A., Toth, M., et al. (2014). The gut microbiota influences blood-brain barrier permeability in mice. Sci. Transl. Med. 6:263ra158. doi: 10.1126/scitranslmed.3009759
Bravo, J. A., Forsythe, P., Chew, M. V., Escaravage, E., Savignac, H. M., Dinan, T. G., et al. (2011). Ingestion of Lactobacillus strain regulates emotional behavior and central GABA receptor expression in a mouse via the vagus nerve. Proc. Natl. Acad. Sci. U S A. 108, 16050–16055. doi: 10.1073/pnas.1102999108
Buckley, P. F. (2019). Neuroinflammation and Schizophrenia. Curr. Psychiatry Rep. 21:72. doi: 10.1007/s11920-019-1050-z
Busnelli, M., Manzini, S., and Chiesa, G. (2019). The Gut Microbiota Affects Host Pathophysiology as an Endocrine Organ: A Focus on Cardiovascular Disease. Nutrients 12:nu12010079. doi: 10.3390/nu12010079
Cao, X., Liu, K., Liu, J., Liu, Y. W., Xu, L., Wang, H., et al. (2021). Dysbiotic Gut Microbiota and Dysregulation of Cytokine Profile in Children and Teens With Autism Spectrum Disorder. Front. Neurosci. 15:635925. doi: 10.3389/fnins.2021.635925
Capuco, A., Urits, I., Hasoon, J., Chun, R., Gerald, B., Wang, J. K., et al. (2020). Current Perspectives on Gut Microbiome Dysbiosis and Depression. Adv. Ther. 37, 1328–1346. doi: 10.1007/s12325-020-01272-7
Chen, L., Chen, D. Q., Liu, J. R., Zhang, J., Vaziri, N. D., Zhuang, S., et al. (2019). Unilateral ureteral obstruction causes gut microbial dysbiosis and metabolome disorders contributing to tubulointerstitial fibrosis. Exp. Mol. Med. 51, 1–18. doi: 10.1038/s12276-019-0234-2
Cheng, S., Han, B., Ding, M., Wen, Y., Ma, M., Zhang, L., et al. (2020). Identifying psychiatric disorder-associated gut microbiota using microbiota-related gene set enrichment analysis. Brief Bioinform. 21, 1016–1022. doi: 10.1093/bib/bbz034
Coello, K., Hansen, T. H., Sorensen, N., Munkholm, K., Kessing, L. V., Pedersen, O., et al. (2019). Gut microbiota composition in patients with newly diagnosed bipolar disorder and their unaffected first-degree relatives. Brain Behav. Immun. 75, 112–118. doi: 10.1016/j.bbi.2018.09.026
Correa-Oliveira, R., Fachi, J. L., Vieira, A., Sato, F. T., and Vinolo, M. A. (2016). Regulation of immune cell function by short-chain fatty acids. Clin. Transl. Immunol. 5:e73. doi: 10.1038/cti.2016.17
Costello, E. K., Lauber, C. L., Hamady, M., Fierer, N., Gordon, J. I., and Knight, R. (2009). Bacterial community variation in human body habitats across space and time. Science 326, 1694–1697. doi: 10.1126/science.1177486
Dabke, K., Hendrick, G., and Devkota, S. (2019). The gut microbiome and metabolic syndrome. J. Clin. Invest. 129, 4050–4057. doi: 10.1172/JCI129194
Dalile, B., Van Oudenhove, L., Vervliet, B., and Verbeke, K. (2019). The role of short-chain fatty acids in microbiota-gut-brain communication. Nat. Rev. Gastroenterol. Hepatol. 16, 461–478. doi: 10.1038/s41575-019-0157-3
De Berardis, D., Vellante, F., Pettorruso, M., Lucidi, L., Tambelli, A., Di Muzio, I., et al. (2021). Suicide and Genetic Biomarkers: Toward Personalized Tailored-treatment with Lithium and Clozapine. Curr. Pharmaceut. Design 27, 3293–3304. doi: 10.2174/1381612827666210603143353
Dickerson, F., Adamos, M., Katsafanas, E., Khushalani, S., Origoni, A., Savage, C., et al. (2018). Adjunctive probiotic microorganisms to prevent rehospitalization in patients with acute mania: A randomized controlled trial. Bipolar Disord. 20, 614–621. doi: 10.1111/bdi.12652
Dilsaver, S. C. (2011). An estimate of the minimum economic burden of bipolar I and II disorders in the United States: 2009. J. Affect. Disord. 129, 79–83. doi: 10.1016/j.jad.2010.08.030
Dominguez-Bello, M. G., Costello, E. K., Contreras, M., Magris, M., Hidalgo, G., Fierer, N., et al. (2010). Delivery mode shapes the acquisition and structure of the initial microbiota across multiple body habitats in newborns. Proc. Natl. Acad. Sci. U S A. 107, 11971–11975. doi: 10.1073/pnas.1002601107
Erny, D., Hrabe de Angelis, A. L., Jaitin, D., Wieghofer, P., Staszewski, O., David, E., et al. (2015). Host microbiota constantly control maturation and function of microglia in the CNS. Nat. Neurosci. 18, 965–977. doi: 10.1038/nn.4030
Eslami Shahrbabaki, M., Sabouri, S., Sabahi, A., Barfeh, D., Divsalar, P., Esmailzadeh, M., et al. (2020). The Efficacy of Probiotics for Treatment of Bipolar Disorder-Type 1: A Randomized, Double-Blind, Placebo Controlled Trial. Iran. J. Psychiat. 15, 10–16.
Evans, S. J., Bassis, C. M., Hein, R., Assari, S., Flowers, S. A., Kelly, M. B., et al. (2017). The gut microbiome composition associates with bipolar disorder and illness severity. J. Psychiatr. Res. 87, 23–29. doi: 10.1016/j.jpsychires.2016.12.007
Fang, Z., Li, L., Zhao, J., Zhang, H., Lee, Y. K., Lu, W., et al. (2020). Bifidobacteria adolescentis regulated immune responses and gut microbial composition to alleviate DNFB-induced atopic dermatitis in mice. Eur. J. Nutr. 59, 3069–3081. doi: 10.1007/s00394-019-02145-8
Flowers, S. A., Baxter, N. T., Ward, K. M., Kraal, A. Z., McInnis, M. G., Schmidt, T. M., et al. (2019). Effects of Atypical Antipsychotic Treatment and Resistant Starch Supplementation on Gut Microbiome Composition in a Cohort of Patients with Bipolar Disorder or Schizophrenia. Pharmacotherapy 39, 161–170. doi: 10.1002/phar.2214
Flowers, S. A., Evans, S. J., Ward, K. M., McInnis, M. G., and Ellingrod, V. L. (2017). Interaction Between Atypical Antipsychotics and the Gut Microbiome in a Bipolar Disease Cohort. Pharmacotherapy 37, 261–267. doi: 10.1002/phar.1890
Fornaro, M., Anastasia, A., Monaco, F., Novello, S., Fusco, A., Iasevoli, F., et al. (2018). Clinical and psychopathological features associated with treatment-emergent mania in bipolar-II depressed outpatients exposed to antidepressants. J. Affect. Disord. 234, 131–138. doi: 10.1016/j.jad.2018.02.085
Fortinguerra, S., Sorrenti, V., Giusti, P., Zusso, M., and Buriani, A. (2019). Pharmacogenomic Characterization in Bipolar Spectrum Disorders. Pharmaceutics 12:harmaceutics12010013. doi: 10.3390/pharmaceutics12010013
Fulling, C., Dinan, T. G., and Cryan, J. F. (2019). Gut Microbe to Brain Signaling: What Happens in Vagus. Neuron 101, 998–1002. doi: 10.1016/j.neuron.2019.02.008
Goodrich, J. K., Waters, J. L., Poole, A. C., Sutter, J. L., Koren, O., Blekhman, R., et al. (2014). Human genetics shape the gut microbiome. Cell 159, 789–799. doi: 10.1016/j.cell.2014.09.053
Haack, M., Sanchez, E., and Mullington, J. M. (2007). Elevated inflammatory markers in response to prolonged sleep restriction are associated with increased pain experience in healthy volunteers. Sleep 30, 1145–1152. doi: 10.1093/sleep/30.9.1145
Han, W., Tellez, L. A., Perkins, M. H., Perez, I. O., Qu, T., Ferreira, J., et al. (2018). A Neural Circuit for Gut-Induced Reward. Cell 175, 665–678e623. doi: 10.1016/j.cell.2018.08.049
Hinton, R. (2020). A case report looking at the effects of faecal microbiota transplantation in a patient with bipolar disorder. Aust. N Z. J. Psychiat. 54, 649–650. doi: 10.1177/0004867420912834
Howes, O. D., McCutcheon, R., Owen, M. J., and Murray, R. M. (2017). The Role of Genes, Stress, and Dopamine in the Development of Schizophrenia. Biol. Psychiat. 81, 9–20. doi: 10.1016/j.biopsych.2016.07.014
Hu, S., Li, A., Huang, T., Lai, J., Li, J., Sublette, M. E., et al. (2019). Gut Microbiota Changes in Patients with Bipolar Depression. Adv. Sci. 6:1900752. doi: 10.1002/advs.201900752
Huber, R. S., Kondo, D. G., Shi, X. F., Prescot, A. P., Clark, E., Renshaw, P. F., et al. (2018). Relationship of executive functioning deficits to N-acetyl aspartate (NAA) and gamma-aminobutyric acid (GABA) in youth with bipolar disorder. J. Affect. Disord. 225, 71–78. doi: 10.1016/j.jad.2017.07.052
Hvas, C. L., Dahl Jorgensen, S. M., Jorgensen, S. P., Storgaard, M., Lemming, L., Hansen, M. M., et al. (2019). Fecal Microbiota Transplantation Is Superior to Fidaxomicin for Treatment of Recurrent Clostridium difficile Infection. Gastroenterology 156, 1324–1332e1323. doi: 10.1053/j.gastro.2018.12.019
Ivanov, I. I., Atarashi, K., Manel, N., Brodie, E. L., Shima, T., Karaoz, U., et al. (2009). Induction of intestinal Th17 cells by segmented filamentous bacteria. Cell 139, 485–498. doi: 10.1016/j.cell.2009.09.033
Jakobsson, H. E., Abrahamsson, T. R., Jenmalm, M. C., Harris, K., Quince, C., Jernberg, C., et al. (2014). Decreased gut microbiota diversity, delayed Bacteroidetes colonisation and reduced Th1 responses in infants delivered by caesarean section. Gut 63, 559–566. doi: 10.1136/gutjnl-2012-303249
Jiang, H. Y., Xu, L. L., Zhang, X., Zhang, Z., and Ruan, B. (2019). The microbiome in bipolar depression: A longitudinal study of one pair of monozygotic twins. Bipol. Disord. 21, 93–97. doi: 10.1111/bdi.12736
Jin, Q., Black, A., Kales, S. N., Vattem, D., Ruiz-Canela, M., and Sotos-Prieto, M. (2019). Metabolomics and Microbiomes as Potential Tools to Evaluate the Effects of the Mediterranean Diet. Nutrients 11:nu11010207. doi: 10.3390/nu11010207
Kadriu, B., Farmer, C. A., Yuan, P., Park, L. T., Deng, Z. D., Moaddel, R., et al. (2019). The kynurenine pathway and bipolar disorder: intersection of the monoaminergic and glutamatergic systems and immune response. Mol. Psychiatry 2019:8. doi: 10.1038/s41380-019-0589-8
Kamada, N., Seo, S. U., Chen, G. Y., and Nunez, G. (2013). Role of the gut microbiota in immunity and inflammatory disease. Nat. Rev. Immunol. 13, 321–335. doi: 10.1038/nri3430
Kao, L. T., Lin, H. C., and Lee, H. C. (2019). Inflammatory bowel disease and bipolar disorder: A population-based cross-sectional study. J. Affect. Disord. 247, 120–124. doi: 10.1016/j.jad.2019.01.014
Karling, P., Maripuu, M., Wikgren, M., Adolfsson, R., and Norrback, K. F. (2016). Association between gastrointestinal symptoms and affectivity in patients with bipolar disorder. World J. Gastroenterol. 22, 8540–8548. doi: 10.3748/wjg.v22.i38.8540
Kim, S. U., and de Vellis, J. (2005). Microglia in health and disease. J. Neurosci. Res. 81, 302–313. doi: 10.1002/jnr.20562
Kirkpatrick, B., and Miller, B. J. (2013). Inflammation and schizophrenia. Schizophr. Bull. 39, 1174–1179. doi: 10.1093/schbul/sbt141
Knight, R., Callewaert, C., Marotz, C., Hyde, E. R., Debelius, J. W., McDonald, D., et al. (2017). The Microbiome and Human Biology. Annu. Rev. Genomics Hum. Genet. 18, 65–86. doi: 10.1146/annurev-genom-083115-022438
Kostic, A. D., Xavier, R. J., and Gevers, D. (2014). The microbiome in inflammatory bowel disease: current status and the future ahead. Gastroenterology 146, 1489–1499. doi: 10.1053/j.gastro.2014.02.009
Lai, W., Zhao, J., Xu, S., Deng, W., Xu, D., Wang, M., et al. (2021). Shotgun metagenomics reveals both taxonomic and tryptophan pathway differences of gut microbiota in bipolar disorder with current major depressive episode patients. J. Affect. Disord. 278, 311–319. doi: 10.1016/j.jad.2020.09.010
LeBlanc, J. G., Chain, F., Martin, R., Bermudez-Humaran, L. G., Courau, S., and Langella, P. (2017). Beneficial effects on host energy metabolism of short-chain fatty acids and vitamins produced by commensal and probiotic bacteria. Microb. Cell Fact. 16:79. doi: 10.1186/s12934-017-0691-z
Lee, J. Y., Hall, J. A., Kroehling, L., Wu, L., Najar, T., Nguyen, H. H., et al. (2020). Serum Amyloid A Proteins Induce Pathogenic Th17 Cells and Promote Inflammatory Disease. Cell 183, 2036–2039. doi: 10.1016/j.cell.2020.12.008
Lenz, K. M., and Nelson, L. H. (2018). Microglia and Beyond: Innate Immune Cells As Regulators of Brain Development and Behavioral Function. Front. Immunol. 9:698. doi: 10.3389/fimmu.2018.00698
Lindgren, E., Gray, K., Miller, G., Tyler, R., Wiers, C. E., Volkow, N. D., et al. (2018). Food addiction: A common neurobiological mechanism with drug abuse. Front. Biosci. 23:811–836. doi: 10.2741/4618
Lu, Q., Lai, J., Lu, H., Ng, C., Huang, T., Zhang, H., et al. (2019). Gut Microbiota in Bipolar Depression and Its Relationship to Brain Function: An Advanced Exploration. Front. Psychiat. 10:784. doi: 10.3389/fpsyt.2019.00784
Lucidi, L., Pettorruso, M., Vellante, F., Di Carlo, F., Ceci, F., Santovito, M. C., et al. (2021). Gut Microbiota and Bipolar Disorder: An Overview on a Novel Biomarker for Diagnosis and Treatment. Int. J. Mol. Sci. 22:ijms22073723. doi: 10.3390/ijms22073723
Mahar, I., Bambico, F. R., Mechawar, N., and Nobrega, J. N. (2014). Stress, serotonin, and hippocampal neurogenesis in relation to depression and antidepressant effects. Neurosci. Biobehav. Rev. 38, 173–192. doi: 10.1016/j.neubiorev.2013.11.009
Marotta, A., Sarno, E., Del Casale, A., Pane, M., Mogna, L., Amoruso, A., et al. (2019). Effects of Probiotics on Cognitive Reactivity, Mood, and Sleep Quality. Front. Psychiatry 10:164. doi: 10.3389/fpsyt.2019.00164
Marx, W., Lane, M., Hockey, M., Aslam, H., Berk, M., Walder, K., et al. (2021). Diet and depression: exploring the biological mechanisms of action. Mol. Psychiatry 26, 134–150. doi: 10.1038/s41380-020-00925-x
McAllister-Williams, R. H., Sousa, S., Kumar, A., Greco, T., Bunker, M. T., Aaronson, S. T., et al. (2020). The effects of vagus nerve stimulation on the course and outcomes of patients with bipolar disorder in a treatment-resistant depressive episode: a 5-year prospective registry. Int. J. Bipolar Disord. 8:13. doi: 10.1186/s40345-020-0178-4
McCormick, U., Murray, B., and McNew, B. (2015). Diagnosis and treatment of patients with bipolar disorder: A review for advanced practice nurses. J. Am. Assoc. Nurse Pract. 27, 530–542. doi: 10.1002/2327-6924.12275
McIntyre, R. S., Subramaniapillai, M., Shekotikhina, M., Carmona, N. E., Lee, Y., Mansur, R. B., et al. (2021). Characterizing the gut microbiota in adults with bipolar disorder: a pilot study. Nutr. Neurosci. 24, 173–180. doi: 10.1080/1028415x.2019.1612555
Merikangas, K. R., Jin, R., He, J. P., Kessler, R. C., Lee, S., Sampson, N. A., et al. (2011). Prevalence and correlates of bipolar spectrum disorder in the world mental health survey initiative. Arch. Gen. Psychiatry 68, 241–251. doi: 10.1001/archgenpsychiatry.2011.12
Nagpal, R., Neth, B. J., Wang, S., Craft, S., and Yadav, H. (2019). Modified Mediterranean-ketogenic diet modulates gut microbiome and short-chain fatty acids in association with Alzheimer’s disease markers in subjects with mild cognitive impairment. EBioMedicine 47, 529–542. doi: 10.1016/j.ebiom.2019.08.032
Newberg, A. R., Catapano, L. A., Zarate, C. A., and Manji, H. K. (2008). Neurobiology of bipolar disorder. Expert Rev. Neurother. 8, 93–110. doi: 10.1586/14737175.8.1.93
Nguyen, T. T., Kosciolek, T., Eyler, L. T., Knight, R., and Jeste, D. V. (2018). Overview and systematic review of studies of microbiome in schizophrenia and bipolar disorder. J. Psychiatr. Res. 99, 50–61. doi: 10.1016/j.jpsychires.2018.01.013
Nohr, M. K., Egerod, K. L., Christiansen, S. H., Gille, A., Offermanns, S., Schwartz, T. W., et al. (2015). Expression of the short chain fatty acid receptor GPR41/FFAR3 in autonomic and somatic sensory ganglia. Neuroscience 290, 126–137. doi: 10.1016/j.neuroscience.2015.01.040
Ogawa, Y., Miyoshi, C., Obana, N., Yajima, K., Hotta-Hirashima, N., Ikkyu, A., et al. (2020). Gut microbiota depletion by chronic antibiotic treatment alters the sleep/wake architecture and sleep EEG power spectra in mice. Sci. Rep. 10:19554. doi: 10.1038/s41598-020-76562-9
O’Mahony, S. M., Clarke, G., Borre, Y. E., Dinan, T. G., and Cryan, J. F. (2015). Serotonin, tryptophan metabolism and the brain-gut-microbiome axis. Behav. Brain Res. 277, 32–48. doi: 10.1016/j.bbr.2014.07.027
Orsolini, L., Valchera, A., Vecchiotti, R., Tomasetti, C., Iasevoli, F., Fornaro, M., et al. (2016). Suicide during Perinatal Period: Epidemiology, Risk Factors, and Clinical Correlates. Front. Psychiat. 7:138. doi: 10.3389/fpsyt.2016.00138
Painold, A., Morkl, S., Kashofer, K., Halwachs, B., Dalkner, N., Bengesser, S., et al. (2019). A step ahead: Exploring the gut microbiota in inpatients with bipolar disorder during a depressive episode. Bipolar Disord. 21, 40–49. doi: 10.1111/bdi.12682
Passos, I. C., Mwangi, B., Vieta, E., Berk, M., and Kapczinski, F. (2016). Areas of controversy in neuroprogression in bipolar disorder. Acta Psychiatr. Scand. 134, 91–103. doi: 10.1111/acps.12581
Patterson, E., Ryan, P. M., Wiley, N., Carafa, I., Sherwin, E., Moloney, G., et al. (2019). Gamma-aminobutyric acid-producing lactobacilli positively affect metabolism and depressive-like behaviour in a mouse model of metabolic syndrome. Sci. Rep. 9:16323. doi: 10.1038/s41598-019-51781-x
Pompili, M., Gonda, X., Serafini, G., Innamorati, M., Sher, L., Amore, M., et al. (2013). Epidemiology of suicide in bipolar disorders: a systematic review of the literature. Bipolar Disord. 15, 457–490. doi: 10.1111/bdi.12087
Potter, M. C., Elmer, G. I., Bergeron, R., Albuquerque, E. X., Guidetti, P., Wu, H. Q., et al. (2010). Reduction of endogenous kynurenic acid formation enhances extracellular glutamate, hippocampal plasticity, and cognitive behavior. Neuropsychopharmacology 35, 1734–1742. doi: 10.1038/npp.2010.39
Qin, J., Li, R., Raes, J., Arumugam, M., Burgdorf, K. S., Manichanh, C., et al. (2010). A human gut microbial gene catalogue established by metagenomic sequencing. Nature 464, 59–65. doi: 10.1038/nature08821
Reininghaus, E. Z., Wetzlmair, L. C., Fellendorf, F. T., Platzer, M., Queissner, R., Birner, A., et al. (2018). The Impact of Probiotic Supplements on Cognitive Parameters in Euthymic Individuals with Bipolar Disorder: A Pilot Study. Neuropsychobiology 2018, 1–8. doi: 10.1159/000492537
Robertson, R. C., Seira Oriach, C., Murphy, K., Moloney, G. M., Cryan, J. F., Dinan, T. G., et al. (2017). Omega-3 polyunsaturated fatty acids critically regulate behaviour and gut microbiota development in adolescence and adulthood. Brain Behav. Immun. 59, 21–37. doi: 10.1016/j.bbi.2016.07.145
Rong, H., Xie, X., Zhao, J., Lai, W., Wang, M., Xu, D., et al. (2019). Similarly in depression, nuances of gut microbiota: Evidences from a shotgun metagenomics sequencing study on major depressive disorder versus bipolar disorder with current major depressive episode patients. J. Psychiat. Res. 113, 90–99. doi: 10.1016/j.jpsychires.2019.03.017
Round, J. L., and Mazmanian, S. K. (2009). The gut microbiota shapes intestinal immune responses during health and disease. Nat. Rev. Immunol. 9, 313–323. doi: 10.1038/nri2515
Scheiman, J., Luber, J. M., Chavkin, T. A., MacDonald, T., Tung, A., Pham, L. D., et al. (2019). Meta-omics analysis of elite athletes identifies a performance-enhancing microbe that functions via lactate metabolism. Nat. Med. 25, 1104–1109. doi: 10.1038/s41591-019-0485-4
Schwarcz, R., and Stone, T. W. (2017). The kynurenine pathway and the brain: Challenges, controversies and promises. Neuropharmacology 112, 237–247. doi: 10.1016/j.neuropharm.2016.08.003
Sen, P., Molinero-Perez, A., O’Riordan, K. J., McCafferty, C. P., O’Halloran, K. D., and Cryan, J. F. (2021). Microbiota and sleep: awakening the gut feeling. Trends Mol. Med. 2021:4. doi: 10.1016/j.molmed.2021.07.004
Serafini, R. A., Pryce, K. D., and Zachariou, V. (2020). The Mesolimbic Dopamine System in Chronic Pain and Associated Affective Comorbidities. Biol. Psychiatry 87, 64–73. doi: 10.1016/j.biopsych.2019.10.018
Sherwin, E., Bordenstein, S. R., Quinn, J. L., Dinan, T. G., and Cryan, J. F. (2019). Microbiota and the social brain. Science 366:2016. doi: 10.1126/science.aar2016
Stephan, A. H., Barres, B. A., and Stevens, B. (2012). The complement system: an unexpected role in synaptic pruning during development and disease. Annu. Rev. Neurosci. 35, 369–389. doi: 10.1146/annurev-neuro-061010-113810
Sun, F., Lei, Y., You, J., Li, C., Sun, L., Garza, J., et al. (2019). Adiponectin modulates ventral tegmental area dopamine neuron activity and anxiety-related behavior through AdipoR1. Mol. Psychiatry 24, 126–144. doi: 10.1038/s41380-018-0102-9
Troubat, R., Barone, P., Leman, S., Desmidt, T., Cressant, A., Atanasova, B., et al. (2021). Neuroinflammation and depression: A review. Eur. J. Neurosci. 53, 151–171. doi: 10.1111/ejn.14720
Vieta, E., Berk, M., Schulze, T., Carvalho, A., Suppes, T., Calabrese, J., et al. (2018). Bipolar disorders. Nat. Rev. Dis. Primers 4:18008. doi: 10.1038/nrdp.2018.8
Vinberg, M., Ottesen, N. M., Meluken, I., Sorensen, N., Pedersen, O., Kessing, L. V., et al. (2019). Remitted affective disorders and high familial risk of affective disorders associate with aberrant intestinal microbiota. Acta Psychiatr. Scand. 139, 174–184. doi: 10.1111/acps.12976
Waldecker, M., Kautenburger, T., Daumann, H., Busch, C., and Schrenk, D. (2008). Inhibition of histone-deacetylase activity by short-chain fatty acids and some polyphenol metabolites formed in the colon. J. Nutr. Biochem. 19, 587–593. doi: 10.1016/j.jnutbio.2007.08.002
Wang, S., Ishima, T., Zhang, J., Qu, Y., Chang, L., Pu, Y., et al. (2020). Ingestion of Lactobacillus intestinalis and Lactobacillus reuteri causes depression- and anhedonia-like phenotypes in antibiotic-treated mice via the vagus nerve. J. Neuroinflamm. 17:241. doi: 10.1186/s12974-020-01916-z
Wang, Y., Zhan, G., Cai, Z., Jiao, B., Zhao, Y., Li, S., et al. (2021). Vagus nerve stimulation in brain diseases: Therapeutic applications and biological mechanisms. Neurosci. Biobehav. Rev. 127, 37–53. doi: 10.1016/j.neubiorev.2021.04.018
Yano, J. M., Yu, K., Donaldson, G. P., Shastri, G. G., Ann, P., Ma, L., et al. (2015). Indigenous bacteria from the gut microbiota regulate host serotonin biosynthesis. Cell 161, 264–276. doi: 10.1016/j.cell.2015.02.047
Yirmiya, R., Rimmerman, N., and Reshef, R. (2015). Depression as a microglial disease. Trends Neurosci. 38, 637–658. doi: 10.1016/j.tins.2015.08.001
Zhang, Y., Xie, B., Chen, X., Zhang, J., and Yuan, S. (2021). A key role of gut microbiota-vagus nerve/spleen axis in sleep deprivation-mediated aggravation of systemic inflammation after LPS administration. Life Sci. 265:118736. doi: 10.1016/j.lfs.2020.118736
Zhang, Z., Tang, H., Chen, P., Xie, H., and Tao, Y. (2019). Demystifying the manipulation of host immunity, metabolism, and extraintestinal tumors by the gut microbiome. Signal Transduct. Target Ther. 4:41. doi: 10.1038/s41392-019-0074-5
Keywords: bipolar disorder, gut microbiota, gut-brain axis, pathogenesis, treatment
Citation: Zhang P, Kong L, Huang H, Pan Y, Zhang D, Jiang J, Shen Y, Xi C, Lai J, Ng CH and Hu S (2022) Gut Microbiota – A Potential Contributor in the Pathogenesis of Bipolar Disorder. Front. Neurosci. 16:830748. doi: 10.3389/fnins.2022.830748
Received: 07 December 2021; Accepted: 28 February 2022;
Published: 23 March 2022.
Edited by:
Zoltan Sarnyai, James Cook University, AustraliaReviewed by:
Domenico De Berardis, Mental Health Center (CSM) and Psychiatric Service of Diagnosis and Treatment (SPDC), ItalyLu Cheng, Rutgers, The State University of New Jersey, United States
Copyright © 2022 Zhang, Kong, Huang, Pan, Zhang, Jiang, Shen, Xi, Lai, Ng and Hu. This is an open-access article distributed under the terms of the Creative Commons Attribution License (CC BY). The use, distribution or reproduction in other forums is permitted, provided the original author(s) and the copyright owner(s) are credited and that the original publication in this journal is cited, in accordance with accepted academic practice. No use, distribution or reproduction is permitted which does not comply with these terms.
*Correspondence: Chee H. Ng, Y25nQHVuaW1lbGIuZWR1LmF1; Shaohua Hu, ZG9yaHVzaGFvaHVhQHpqdS5lZHUuY24=
†These authors have contributed equally to this work