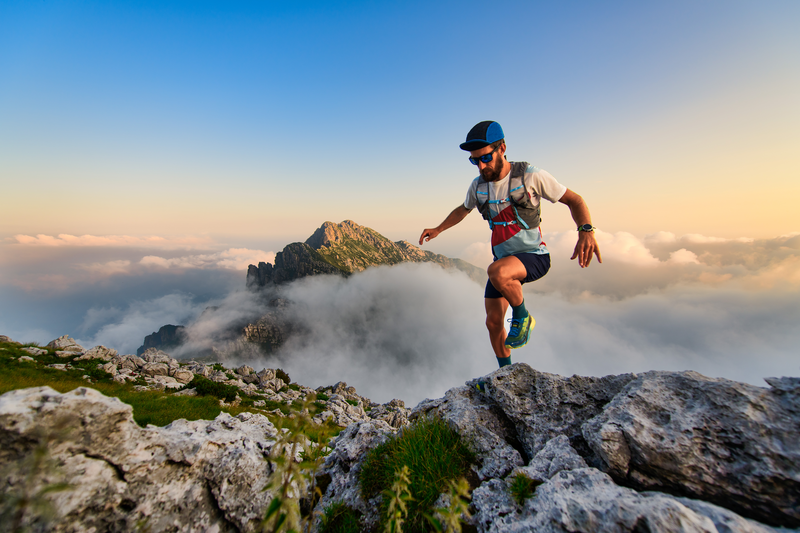
95% of researchers rate our articles as excellent or good
Learn more about the work of our research integrity team to safeguard the quality of each article we publish.
Find out more
SYSTEMATIC REVIEW article
Front. Neurosci. , 10 February 2022
Sec. Neurodegeneration
Volume 16 - 2022 | https://doi.org/10.3389/fnins.2022.811335
This article is part of the Research Topic Key Molecules Linking Organelles in Neurodegenerative Diseases View all 5 articles
Background: There is a dose-response relationship between tooth loss and cognitive impairment, while tooth loss can be an independent risk factor for Alzheimer's disease (AD) and vascular dementia (VaD). Tooth loss can also accelerate nerve damage and neurodegeneration. However, the associated mechanisms remain poorly understood.
Objective: To conduct a systematic review of animal experiments on cognitive decline caused by the loss of occlusal support performed over the past 10 years and summarize the possible underlying mechanisms.
Methods: “Tooth Loss,” “Edentulous,” “Tooth Extraction and Memory Loss,” “Cognition Impairment,” and “Dementia” were used as keywords to search PubMed, Embase, SCI, ScienceDirect, and OpenGrey. A total of 1,317 related articles from 2010 to 2021 were retrieved, 26 of which were included in the review after screening according to predetermined inclusion and exclusion criteria. Comprehensiveness was evaluated using ARRIVE guidelines and the risk of bias was assessed using SYCLE'S risk of bias tool.
Results: The putative mechanisms underlying the cognitive impairment resulting from the loss of occlusal support are as follows: (1) The mechanical pathway, whereby tooth loss leads to masticatory motor system functional disorders. Masticatory organ activity and cerebral blood flow decrease. With reduced afferent stimulation of peripheral receptors (such as in the periodontal membrane) the strength of the connections between neural pathways is decreased, and the corresponding brain regions degenerate; (2) the aggravation pathway, in which tooth loss aggravates existing neurodegenerative changes. Tooth loss can accelerates nerve damage through apoptosis and mitochondrial autophagy, increases amyloid deposition in the brain; and (3) the long-term inflammatory stress pathway, which involves metabolic disorders, microbial-gut-brain axis, the activation of microglia and astrocytes, and inflammatory cascade effect in central nervous system.
Conclusion: The loss of occlusal support may lead to cognitive dysfunction through the reduction of chewing-related stimuli, aggravation of nerve damage, and long-term inflammatory stress.
There are many risk factors for cognitive disorders such as Alzheimer's disease (AD) and vascular dementia (VaD). Included psychosocial factors such as depressive symptoms, limited interests, major traumatic life events, lack of education, poor economic status, smoking, and alcohol abuse. And biological risk factors, such as advanced age, sex, apolipoprotein E4 (APOE4) allele, hypertension, hyperlipidemia, diabetes, and heart disease. In decades, an increasing number of studies have shown that a bidirectional relationship exists between the loss of occlusal support and cognitive dysfunction. Patients with cognitive impairment tend to neglect their oral hygiene, and are more likely to develop periodontitis (Chu et al., 2015; Campos et al., 2017; Ma et al., 2021) and to experience tooth loss at an earlier age. However, the loss of occlusal support can significantly increase the risk of dementia (Del Brutto et al., 2014; Dintica et al., 2018), accelerate neurodegeneration, and directly lead to cognitive impairment. Although this connection has been confirmed at the epidemiological level, the associated mechanisms remain unclear. In this review, we systematically discuss and summarize three putative underlying pathways identified through the screening of relevant animal studies undertaken over the last 10 years.
Older patients with degenerative dementia (such as AD, Parkinson's disease, and Lewy body dementia) account for the largest proportion of people with cognitive impairment, followed by patients with vascular dementia, and then other types of dementia. Tooth loss can lead to cognitive dysfunction, while a thorough understanding of the associated pathogenesis can benefit the treatment and/or prevention of this condition. Animal models are conducive to determining the mechanisms that might link the loss of occlusal support and cognitive impairment at different ages.
Food quantity and texture can be strictly controlled in animal experiments. Because powder feeding is also considered to reduce chewing-related stimuli and mimic tooth loss, it was also included as an intervention. Memory loss is often used as a predictor of mild cognitive impairment and dementia. Thus, in addition to “cognitive impairment,” keywords related to memory loss were also used as search strings.
A literature search was performed in PubMed, Embase, SCI, ScienceDirect, and OpenGrey for related articles published between 2010 and 2021 (the search string is shown in Table 1). Article selection was carried out by two researchers (the scanning path is shown in Figure 1). Any disagreement was resolved by a third researcher from the same group. A total of 1,317 records were identified, 526 of which were excluded due to duplication. After screening the titles and abstracts, 467 articles were excluded as they did not meet the inclusion criteria. Of the remaining 324, 128 (40%) met the inclusion criteria, most of which were clinical trials and reviews. Finally, 26 articles involving animal experiments were included for analysis.
(i) Type of experiment: in vivo animal experiment.
(ii) Subjects: experimental animals.
(iii) Publication time: January 2010–September 2021.
(iv) Control group: present.
(v) Experimental intervention: tooth extraction/soft food provision.
(vi) Results: behavioral and central nervous system-related changes.
(vii) Following damage to the inferior alveolar nerve, the neural pathways of the corresponding teeth were damaged, which was consistent with the hypothesis that “tooth loss leads to reduced afferent nerve stimulation.” Thus, damage to the inferior alveolar nerve was included in the study.
(i) Articles reporting the grinding of short teeth were excluded as the influence of residual periodontal membrane in mastication cannot be ruled out (Pei et al., 2018).
(ii) Articles reporting experiments using implants/prosthodontics for missing teeth were also excluded.
(iii) The association between periodontal inflammation and cognition has been widely confirmed (Ishida et al., 2017; Iwasaki et al., 2019; Xue et al., 2020); however, the underlying mechanism remains unclear. The experiment of periodontal disease vs. cognitive impairment is excluded.
Selection bias can be analyzed from three aspects: sequence generation, baseline characteristics, and allocation hiding. Only one article reported dividing the animals into groups according to whether there was cognitive decline based on the results of a passive avoidance test (Oue et al., 2013); none of the other articles assessed the baseline levels of individual cognition. Therefore, it was assessed as “uncertain.” Implementation bias was judged as “uncertain” because none of the experiments clarified whether the animal breeder also performed the experiment. Measurement bias was not clarified. The effect of other biases was low in the 26 included studies.
A total of 26 articles met the inclusion criteria and could be divided into the following three categories according to the associated mechanism: (1) Reduction in mechanical masticatory stimulation and weakening of afferent nerve stimulation; (2) aggravate degeneration; and (3) chronic inflammatory stress. Compared with clinical trials, animal experiments are easier to “randomize” and perform “blind.” However, for subsequent clinical studies, the risk of bias in animal experiments needs to be correctly assessed. Table 2 shows the internal authenticity assessment of the included reports based on the SYRCLE risk of bias assessment tool for animal experiments. A detailed evaluation indicated that the comprehensive bias risk of the included studies was low and that the comprehensiveness and authenticity were reliable. The included literature is summarized in Table 3 (mechanical pathway), Table 4 (aggravation pathway), and Table 5 (inflammatory stress pathway).
Rodents were used in all 26 studies assessed. Twenty-one studies entailed the extraction of molars, most of which involved bilateral maxillary molar extraction, and the remainder unilateral molar extraction. Notably, no anterior teeth were extracted in any of the included studies because the effect on animal nutrition intake is believed to be too severe. The age of the animals ranged from 3 weeks to 12 months. In two studies, food hardness was altered to adjust the masticatory intensity to simulate masticatory disorders following the loss of occlusal support. He et al. (2014) exposed and truncated the inferior alveolar nerve of middle-aged and elderly mice and assessed the distribution of hippocampal cells and the behavior of the animals after 4 months. The results showed that injury to the inferior alveolar nerve can damage the hippocampus and affect cognition.
Six of the studies did not perform behavioral experiments. The Morris water maze test was the most frequently used behavioral test, followed by the passive avoidance, novel object recognition, and open field tests.
Mastication is an autonomous rhythmic movement generated by a central pattern generator (CPG) located in the pons and medulla. Receptors in the cerebral cortex and peripheral receptors together help regulate the formation of a unique chewing pattern. Anatomically, the upper craniofacial and maxillofacial areas are closely related. The loss of occlusal support can change the blood supply in the brain and affect cognitive ability through vascular-related factors. Hasegawa et al. (2007) showed that mastication can increase cerebrovascular blood flow, while Miyake et al. (2012) reported that chewing exercise can help prevent Alzheimer's disease by maintaining a good blood supply to the brain. The above relationship was further confirmed in animal experiments. Using arterial spin labeling–magnetic resonance imaging (ASL-MRI), Luo et al. (2019) found that blood flow in cognition-related brain areas was significantly decreased 12 weeks after tooth extraction in rats. Concomitantly, the glutamate content and the expression levels of Bax/Bcl-2, caspase-3, and other apoptosis-related genes in the hippocampal CA1 region were increased, whereas the number of pyramidal cells was decreased. Meanwhile, in the behavioral experiment, rats that underwent tooth extraction showed memory impairment.
Studies have shown that removing molars at a young age leads to a reduction in the number of new cells in the mouse hippocampus and cognitive decline in young mice. Jiang et al. (2011) suggested that afferent stimulation might be weakened after tooth loss, thereby resulting in the down regulation of the BDNF/TrkB/CREB signaling pathway and synaptic plasticity in the hippocampal CA1 region. The loss of occlusal support affects the entire cognition-related network, not only one brain region. Xu et al. (2015) compared the electrophysiological characteristics of the anterior cingulate cortex (ACC) and basolateral amygdala (BLA) between controls and rats subjected to molar extraction after electrical stimulation and found that theta frequency, the characteristic signal of cognition, was asynchronous between the ACC and BLA after tooth extraction. This indicated a weakening of the connection between the ACC and BLA and that tooth extraction exerted a negative effect on the strength of neural networks.
Masticatory organs produce nutritional factors that are retrogradely transported through nerves. Kobayashi et al. (2019) performed electrical stimulation of masticatory muscles in vitro and in vivo to simulate muscle contraction and then tracked labeled neprilysin (NEP) produced by masticatory muscles. The results showed that NEP was transmitted from masticatory muscles to the hippocampus via the trigeminal nucleus in C57BL/6J mice. The authors suggested that this enzyme, which facilitates the clearance of the amyloid-beta (Aβ) peptide, can be retrogradely transported to the brain during masticatory muscle contraction.
Tooth loss has been identified as a risk factor for AD. Tooth loss may accelerate neurodegeneration by increasing Aβ deposition in the brain. The earlier tooth loss occurs, the more pronounced the neurological effects seem to be. Goto et al. (2020) undertook an immunohistochemical analysis and a Barnes Maze spatial learning/memory assessment at different time points after the bilateral extraction of the maxillary molars in triple transgenic AD (3 × TG-AD) mice and found that cytotoxic Aβ42 was released in the trigeminal nucleus and locus coeruleus after 4 months. This resulted in a significant reduction in the number of neurons in the hippocampal CA1/CA3 regions that receive projections from the locus coeruleus. Similarly, Oue et al. (2013) analyzed amyloid deposition and neuron numbers in the hippocampi of 6-month-old J20 mice 4 months after tooth extraction and found no difference in Aβ, Aβ40, and Aβ42 contents between the experimental and control groups. However, differences in behavioral performance and hippocampal nerve number were detected.
Tooth loss induces the activation of inflammatory cells. Taslima et al. (2021) reported that, after tooth extraction in mice with amyloid precursor protein (App) gene knock-in (a mouse model of AD), the numbers of microglia and astrocytes in the hippocampus increased, and a large number of inflammatory cytokines (such as tumor necrosis factor alpha [TNF-α], interleukin-1 [IL-1], and IL-6) were produced, thereby promoting an inflammatory state in neurons in the hippocampus and prefrontal cortex. Early tooth loss can also lead to malnutrition and chronic stress. Kubo et al. (2021) extracted maxillary molars from SAMP8 mice soon after tooth eruption (at 1 month of age) and found that the plasma corticosterone content was significantly higher in the experimental group than in the control group, whereas synaptophysin expression was inhibited. Most researchers in the 26 studies measured plasma corticosterone levels and correlated plasma corticosterone content with chronic stress, thereby quantifying the negative effects of tooth loss on animals, at least partially.
Based on a total of 26 animal-based studies from 2010 to 2021, the objective of this review was to summarize the putative mechanisms underlying the cognitive dysfunction caused by tooth loss. Clinically, this condition primarily affects patients with AD, and treating such patients is always complicated. The existing therapeutic regimens can only reduce memory loss and delay disease deterioration, they cannot cure the disease. Therefore, intervention in the early stages of memory loss is expected to yield the most effective results. A small number of epidemiological studies found no association after confounding factors were removed between tooth loss and cognitive impairment (Naorungroj et al., 2015; Stewart et al., 2015), not animal-based studies. A larger number of epidemiological studies have shown that tooth loss can be a risk factor for Alzheimer's disease and other cognitive disorders (Cerutti-Kopplin et al., 2016; Tsai et al., 2020; Wang and Ge, 2020), and there is a dose-response relationship between the number of missing teeth and cognitive impairment (Chen et al., 2018).
If the link between tooth loss and cognitive impairment is confirmed, it will provide a novel means of targeting the prevention of cognitive impairment. Many clinical studies have shown that chewing exercise can be considered as a preventive method for cognitive impairment (Ono et al., 2010; Tada and Miura, 2017; Chuhuaicura et al., 2019). Corresponding results appeared after the use of implants or dentures to repair the missing teeth, patients' cognitive level can be improved (Ki et al., 2019; Tan et al., 2020). Avivi-Arber et al. (2015) have proved that the neural plasticity of the facial primary motor cortex (FACE-M1) and the adjacent primary somatosensory cortex (FACE-S1) of rats was enhanced after the implantation. More animal-based experiments on observation of cognitive performance after restoration are needed.
Here, we conducted a comprehensive evaluation of the literature according to the ARRIVE checklist and SYRCLE risk of bias assessment tool. The network connecting tooth loss and cognitive impairment is complex, and the analysis of a single pathway often leads to vague and confusing results. Therefore, most of the studies evaluated in this study included multiple mechanisms of action simultaneously.
Most of the articles reported that changes in cognition-related brain regions after tooth loss resulted in impaired masticatory movement and reduced oral stimulation. Cerebral blood flow is stable in general. However, studies have confirmed that mastication has positive effect on cerebral blood flow (Sesay et al., 2000; Luo et al., 2019), increasing blood flow in the motor area, somatosensory area, thalamus and cerebellum, and the number of pyramidal cells in the hippocampus (Momose et al., 1997; Stratulat et al., 2014). And it leads to changes in cerebral metabolism through glial cells. For example, astrocytes wrap around vascular walls and nerves and are activated after cerebral ischemia (Koizumi et al., 2018). It plays a neuroprotective role by ingesting glutamate, releasing glutamine to reduce oxidative stress damage, repairing blood brain barrier (BBB) (Neuhaus et al., 2014), and so on. Activation of the glial cell network accompanied by release of a large number of cytokines and neurotoxic reactive oxygen species (ROS), which can lead to cognitive decline through PI3K/AKT/mTOR and many other pathways (Yang et al., 2021).
The teeth and periodontal membrane are supplied by various branches of the trigeminal nerve. After unilateral damage on different branches of trigeminal nerve in rats, the amount of ganglion neuron on both side was counted. Eight percent associated with teeth (Gregg and Dixon, 1973). Reduction of dental pulp- and periodontal membrane-derived neural signals may have an important negative impact on cognition-related brain regions (Taylor et al., 2009; Osborne et al., 2018). The regulation of mastication and sensation involves multiple brain regions, including the brain stem, prefrontal cortex, thalamus, and limbic system, as well as communication among neural networks related to cognition (Lin et al., 2017). After extraction of incisors and fangs in cat, afferent nerve fibers in the trigeminal nucleus reduced (Linden and Scott, 1989), the number of neurons and the postsynaptic density decreased in the hippocampal CA1 region (Katano et al., 2020). Brain-derived neurotrophic factor (BDNF) and its related pathways may play a certain role in this process (Huang et al., 2021). BDNF is one of the most widely studied neurotrophic factors, produced by nervous system and dominant target organs [such as muscle, dental pulp, and salivary gland (Saruta et al., 2020)]. It is mainly expressed in the hippocampus, cerebral cortex and other cognition-related brain regions, assisting development and repairing of the nervous system. Tyrosine Kinase receptor B (TrkB) is a specific receptor for BDNF. BDNF/TrkB participate in autophagy or neuroplasticity through PI3K/Akt, MAPK/Ras and other pathways (Bramham and Messaoudi, 2005), and eventually have influence on learning and memory. The essential role of BDNF in exercise-memory link may inspire us on the mechanism research between mastication and cognition (Loprinzi and Frith, 2019).
Mitochondrial damage and oxidative stress are the two most reliable hypotheses about aging. Occlusal support loss induces cognitive dysfunction by aggravating degeneration in brain. Early tooth loss leads to mitochondrial damage in hippocampus (Katano et al., 2020). Tooth loss causes excessive releasing ROS (Zhao et al., 2021) and reactive nitrogen free radicals (RNS), let oxidation exceeds the removal of oxides, resulting in tissue damage. Tooth loss can also activate the immune-related glial cells that produce a large number of bioactive factors, such as TNF-α, IL-1, IL-3, IL-6, colony-stimulating factor (CSF), and Interferon-γ (IFN-γ) in brain. Tooth loss leads to neuroplasticity changes and apoptosis (Del et al., 2013; Sofroniew, 2013). Clinical (Okamoto et al., 2010; Goto and Leung, 2019) and animal studies (Oue et al., 2013; Goto et al., 2020) related to Alzheimer's disease, have confirmed the aggravating effect of missing teeth on brain degeneration; however, when it comes to animal modeling, the analyzed genes are mostly related to familial Alzheimer's disease, which accounts for <5% of the total number of these patients (Cannon-Albright et al., 2019), highlighting the need for the development of a more appropriate model to investigate this condition.
Tooth extraction and local anesthesia are strong traumatic stimuli (Gasparini et al., 2002), and the long-term loss of teeth puts the body in a state of chronic stress (Singhrao et al., 2014). Chronic stress is accompanied by changes in glucose, fat and protein metabolism. With further research on cognitive disorders in the last decades, metabolic disorders are gradually regarded as the pathogenic mechanism behind dementia represented by AD (Manyevitch et al., 2018; Ryu et al., 2019). Fasting blood glucose rises sharply under chronic stress, even meets the diagnostic criteria of stress diabetes (Halim, 2019). Elderly patients with diabetes risk from dementia (Ahtiluoto et al., 2010; Biessels and Despa, 2018). This may be related to insulin resistance or dysglycemia in brain. Dysglycemia of brain observed with fluorodeoxyglucose positron emission tomography (FDG-PET) can be one of the valuable indicators long before AD. Besides, enhanced lipolysis brings more free fatty acids and ketone bodies into blood, Ketone bodies can go through the BBB and provide energy to brain. Though several human studies have shown that ketogenic diet had a cognitive protective effect (Neth et al., 2020; Davis and Fournakis, 2021), the relationship between cerebral ketone body and cognitive disorders remains to be studied. From another point of view, enhanced mastication can inhibit the hypothalamic-pituitary-adrenal (HPA) axis (Azuma et al., 2017) and attenuate stress-induced increases in the plasma concentrations of glucocorticoid, catechol, and nitric oxide (NO) (Sunariani et al., 2019). They can cross the BBB and lead to depression, anxiety, and cognitive disorders.
Chronic stress is closely related to inflammation. After teeth extraction, chronic stress activates glial cells and release excess ROS, causing an inflammatory cascade effect in the central nervous system (Taslima et al., 2021). Chronic inflammation will also interact with chronic stress and oxidative stress, thereby aggravating nerve damage. In clinical practice, tooth loss is mostly caused by periodontitis. Large number of periodontal pathogens (such as Klebsiella) can be ingested and disturb the intestinal flora (Olsen and Yamazaki, 2019; Xue et al., 2020). Through the microbial-gut-brain axis, short-chain fatty acids (SCFAs) produced by intestinal flora participate in recruiting neutrophils, dendritic cells (DCs), macrophages, T cells and other immune cells, and arise systemic immune responses (Silva et al., 2020; Mirzaei et al., 2021). SCFAs activate glial cells network and cause central nervous inflammation. The relationship between tooth loss caused by severe periodontitis and cognitive impairment along the microbial-gut-brain axis remains to be further studied.
In conclusion, the loss of occlusal support can lead to cognitive impairment through three mechanisms: impaired masticatory movement, the aggravation of neurodegenerative changes, and long-term inflammatory stress (The possible mechanisms are shown in Figure 2). The pathogenesis, even the most commonly studied cognitive disorders such as AD, remains unclear at present. In the future, mechanism of tooth loss leading to cognitive impairment needs to catch up with the evolution of brain science. Specific cytological changes after tooth loss or screening of sensitive indicators before cognitive impairment require cohort study. Further studies are needed on the cognitive changes before and after implantation or restoration of losing teeth in vivo.
The datasets presented in this study can be found in online repositories. The names of the repository/repositories and accession number(s) can be found in the article/supplementary material.
XW: literature searching—screening and manuscript writing. JH: design searching strategy and literature screening. QJ: evolution of searching aims. All authors have given approval to the final version of the manuscript.
This work was supported by the National Natural Science Foundation of China (Grant numbers 81771094 and 82170980).
The authors declare that the research was conducted in the absence of any commercial or financial relationships that could be construed as a potential conflict of interest.
All claims expressed in this article are solely those of the authors and do not necessarily represent those of their affiliated organizations, or those of the publisher, the editors and the reviewers. Any product that may be evaluated in this article, or claim that may be made by its manufacturer, is not guaranteed or endorsed by the publisher.
Ahtiluoto, S., Polvikoski, T., Peltonen, M., Solomon, A., Tuomilehto, J., Winblad, B., et al. (2010). Diabetes, Alzheimer disease, and vascular dementia: a population-based neuropathologic study. Neurology 75, 1195–1202. doi: 10.1212/WNL.0b013e3181f4d7f8
Avivi-Arber, L., Lee, J. C., Sood, M., Lakschevitz, F., Fung, M., and Barashi-Gozal, M. S. (2015). Long-term neuroplasticity of the face primary motor cortex and adjacent somatosensory cortex induced by tooth loss can be reversed following dental implant replacement in rats. J. Comp. Neurol. 523, 2372–2389. doi: 10.1002/cne.23793
Azuma, K., Zhou, Q., Niwa, M., and Kubo, K. Y. (2017). Association between mastication, the hippocampus, and the HPA Axis: a comprehensive review. Int. J. Mol. Sci. 18, 1687. doi: 10.3390/ijms18081687
Biessels, G. J., and Despa, F. (2018). Cognitive decline and dementia in diabetes mellitus: mechanisms and clinical implications. Nat. Rev. Endocrinol. 14, 591–604. doi: 10.1038/s41574-018-0048-7
Bramham, C. R., and Messaoudi, E. (2005). BDNF function in adult synaptic plasticity: the synaptic consolidation hypothesis. Prog. Neurobiol. 76, 99–125. doi: 10.1016/j.pneurobio.2005.06.003
Campos, C. H., Ribeiro, G. R., Costa, J. L., and Rodrigues Garcia, R. C. (2017). Correlation of cognitive and masticatory function in Alzheimer's disease. Clin. Oral Investig. 21, 573–578. doi: 10.1007/s00784-016-1923-z
Cannon-Albright, L. A., Foster, N. L., Schliep, K., Farnham, J. M., Teerlink, C. C., and Kaddas, H. (2019). Relative risk for Alzheimer disease based on complete family history. Neurology 92, e1745–e1753. doi: 10.1212/WNL.0000000000007231
Cerutti-Kopplin, D., Feine, J., Padilha, D. M., de Souza, R. F., Ahmadi, M., Rompre, P., et al. (2016). Tooth loss increases the risk of diminished cognitive function: a systematic review and meta-analysis. JDR Clin. Trans. Res. 1, 10–19. doi: 10.1177/2380084416633102
Chen, J., Ren, C. J., Wu, L., Xia, L. Y., Shao, J., and Leng, W. D. (2018). Tooth loss is associated with increased risk of dementia and with a dose-response relationship. Front. Aging Neurosci. 10, 415. doi: 10.3389/fnagi.2018.00415
Chu, C. H., Ng, A., Chau, A. M. H., and Lo, E. C. M. (2015). Oral health status of elderly Chinese with dementia in Hong Kong. Oral Health Prev Dent 13, 51–57. doi: 10.3290/j.ohpd.a32343
Chuhuaicura, P., Dias, F. J., Arias, A., Lezcano, M. F., and Fuentes, R. (2019). Mastication as a protective factor of the cognitive decline in adults: a qualitative systematic review. Int. Dent. J. 69, 334–340. doi: 10.1111/idj.12486
Davis, J. J., and Fournakis, N. (2021). Ketogenic diet for the treatment and prevention of dementia: a review. J. Geriatr. Psychiatry Neurol. 34, 3–10. doi: 10.1177/0891988720901785
Del Brutto, O. H., Gardener, H., Del Brutto, V. J., Maestre, G. E., Zambrano, M., and Montenegro, J. E. (2014). Edentulism associates with worse cognitive performance in community-dwelling elders in rural Ecuador: results of the Atahualpa project. J Community Health 39, 1097–1100. doi: 10.1007/s10900-014-9857-3
Del, R. A., Balschun, D., Wetzel, W., Randolf, A., and Besedovsky, H. O. A. (2013). cytokine network involving brain-borne IL-1β, IL-1ra, IL-18, IL-6, and TNFα operates during long-term potentiation and learning. Brain Behav. Immun. 33, 15–23. doi: 10.1016/j.bbi.2013.05.011
Dintica, C. S., Rizzuto, D., Marseglia, A., Kalpouzos, G., Welmer, A.-. K., et al. (2018). Tooth loss is associated with accelerated cognitive decline and volumetric brain differences: a population-based study. Neurobiol Aging 67:23–30. doi: 10.1016/j.neurobiolaging.2018.03.003
Fukushima-Nakayama, Y., Ono, T., Hayashi, M., Inoue, M., Wake, H., and Ono, T. Reduced mastication impairs memory function. J. Dent. Res. (2017) 96, 1058–1066. doi: 10.1177/0022034517708771.
Gasparini, M., Vanacore, N., Schiaffini, C., Brusa, L., Panella, M., and Talarico, G. (2002). A case-control study on Alzheimer's disease and exposure to anesthesia. Neurol. Sci. 23, 11–14. doi: 10.1007/s100720200017
Goto, T., Kuramoto, E., Dhar, A., Wang, R. P., Seki, H., and Iwai, H. (2020). Neurodegeneration of trigeminal mesencephalic neurons by the tooth loss triggers the progression of Alzheimer's disease in 3 × Tg-AD model mice. J. Alzheimers Dis. 76, 1443–1459. doi: 10.3233/JAD-200257
Goto, T., and Leung, W. K. (2019). Tooth loss and Alzheimer's disease. Curr. Oral Health Rep. 6, 82–88. doi: 10.1007/s40496-019-0219-1
Gregg, J. M., and Dixon, A. D. (1973). Somatotopic organization of the trigeminal ganglion in the rat. Arch. Oral Biol. 18, 487–498. doi: 10.1016/0003-9969(73)90069-1
Halim, M. (2019). The effects of inflammation, aging and oxidative stress on the pathogenesis of diabetes mellitus (type 2 diabetes). Diabetes Metab. Syndr. 13, 1165–1172. doi: 10.1016/j.dsx.2019.01.040
Hasegawa, Y., Ono, T., Hori, K., and Nokubi, T. (2007). Influence of human jaw movement on cerebral blood flow. J. Dent. Res. 86, 64–68. doi: 10.1177/154405910708600110
He, Y., Zhu, J., Huang, F., Qin, L., Fan, W., and He, H. (2014). Age-dependent loss of cholinergic neurons in learning and memory-related brain regions and impaired learning in SAMP8 mice with trigeminal nerve damage. Neural Regen. Res. 9, 1985. doi: 10.4103/1673-5374.145380
Huang, L., Jin, J., Chen, K., You, S., Zhang, H., Sideris, A., et al. (2021). BDNF produced by cerebral microglia promotes cortical plasticity and pain hypersensitivity after peripheral nerve injury. PLoS Biol. 19, e3001337. doi: 10.1371/journal.pbio.3001337
Iinuma, M., Yasui, S., Oonishi, M., Kurata, C., Ichihashi, Y., Tamura, Y., et al. (2011). Relationship between light and dark period activity cycles and oral condition in senescence-accelerated mice. Okajimas Folia Anat. Jpn. 88, 29–36. doi: 10.2535/ofaj.88.29
Ishida, N., Ishihara, Y., Ishida, K., Tada, H., Funaki-Kato, Y., and Hagiwara, M. (2017). Periodontitis induced by bacterial infection exacerbates features of Alzheimer's disease in transgenic mice. NPJ Aging Mech. Dis. 3, 1–7. doi: 10.1038/s41514-017-0015-x
Iwasaki, M., Kimura, Y., Ogawa, H., Yamaga, T., Ansai, T., and Wada, T. (2019). Periodontitis, periodontal inflammation, and mild cognitive impairment: a 5-year cohort study. J. Periodontal Res. 54, 233–240. doi: 10.1111/jre.12623
Jiang, H., Yin, H., Wang, L., Feng, C., Bai, Y., Huang, D., et al. (2021). Memory impairment of chewing-side preference mice is associated with 5-HT-BDNF signal pathway. Mol. Cell. Biochem. 476,303–310. doi: 10.1007/s11010-020-03907-3
Jiang, Q. S., Liang, Z. L., Wu, M. J., Feng, L., Liu, L. L., and Zhang, J. J. (2011). Reduced brain-derived neurotrophic factor expression in cortex and hippocampus involved in the learning and memory deficit in molarless SAMP8 mice. Chin. Med. J. 124, 1540–1544.
Katano, M., Kajimoto, K., Iinuma, M., Azuma, K., and Kubo, K. Y. (2020). Tooth loss early in life induces hippocampal morphology remodeling in senescence-accelerated mouse prone 8 (SAMP8) mice. Int. J. Med. Sci. 17, 517. doi: 10.7150/ijms.40241
Kawahata, M., Ono, Y., Ohno, A., Kawamoto, S., Kimoto, K., and Onozuka, M. (2014). Loss of molars early in life develops behavioral lateralization and impairs hippocampus-dependent recognition memory. BMC Neurosci. 15, 4. doi: 10.1186/1471-2202-15-4
Ki, S., Yun, J., Kim, J., and Lee, Y. (2019). Association between dental implants and cognitive function in community-dwelling older adults in Korea. J. Prev. Med. Public Health 52, 333. doi: 10.3961/jpmph.19.163
Kida, K., Tsuji, T., Tanaka, S., and Kogo, M. (2015). Zinc deficiency with reduced mastication impairs spatial memory in young adult mice. Physiol. Behav. 152, 231–237. doi: 10.1016/j.physbeh.2015.10.005
Kobayashi, T., Nagai, M., Da Silva, J. D., Galaburda, A. M., Rosenberg, S. H., and Hatakeyama, W. (2019). Retrograde transport of masseter muscle-derived neprilysin to hippocampus. Neurosci. Lett. 698:180–185. doi: 10.1016/j.neulet.2019.01.021
Koizumi, S., Hirayama, Y., and Morizawa, Y. M. (2018). New roles of reactive astrocytes in the brain; an organizer of cerebral ischemia. Neurochem. Int. 119, 107–114. doi: 10.1016/j.neuint.2018.01.007
Kubo, K. Y., Murabayashi, C., Kotachi, M., Suzuki, A., Mori, D., Sato, Y., et al. (2017). Tooth loss early in life suppresses neurogenesis and synaptophysin expression in the hippocampus and impairs learning in mice. Arch. Oral Biol. 74, 21–27. doi: 10.1016/j.archoralbio.2016.11.005
Kubo, K. Y., Ogasawara, A., Tsugane, H., Iinuma, M., Takahashi, T., and Azuma, K. (2021). Environmental enrichment improves hypomyelination, synaptic alterations, and memory deficits caused by tooth loss in aged SAMP8 mice. Arch. Oral Biol. 123, 105039. doi: 10.1016/j.archoralbio.2021.105039
Kurozumi, A., Hara, T., Sakamoto, S., Araki, D., Iida-Tamada, S., Kuroda-Ishimine, C., et al. (2019). Effects of the loss and reconstruction of molar occlusal support on memory retrieval and hippocampal neuron density in rats. J. Prosthodont. Res. 63, 283–287. doi: 10.1016/j.jpor.2018.12.009
Zhao, Y., Gao, J., Zhang, Y., Gan, X., and Yu, H. (2021). Cyclosporine a promotes bone remodeling in lps-related inflammation via inhibiting ros/erk signaling: studies in vivo and in vitro. Oxid. Med. Cell. Longev. 2021, 1–21. doi: 10.1155/2021/8836599
Lin, C. S., Wu, C. Y., Wu, S. Y., Lin, H. H., Cheng, D. H., and Lo, W. L. (2017). Age-related difference in functional brain connectivity of mastication. Front. Aging Neurosci. 9, 82. doi: 10.3389/fnagi.2017.00082
Linden, R. W. A., and Scott, B. J. J. (1989). The effect of tooth extraction on periodontal ligament mechanoreceptors represented in the Mesencephalic nucleus of the CAT. Arch. Oral Biol. 34, 937–941. doi: 10.1016/0003-9969(89)90049-6
Loprinzi, P. D., and Frith, E. (2019). A brief primer on the mediational role of BDNF in the exercise-memory link. Clin. Physiol. Funct. Imaging 39, 9–14. doi: 10.1111/cpf.12522
Luo, B., Pang, Q., and Jiang, Q. (2019). Tooth loss causes spatial cognitive impairment in rats through decreased cerebral blood flow and increased glutamate. Arch. Oral Biol. 102, 225–230. doi: 10.1016/j.archoralbio.2019.05.004
Ma, K. S., Hasturk, H., Carreras, I., Dedeoglu, A., Veeravalli, J. J., Huang, J. Y., et al. (2021). Dementia and the risk of periodontitis: a population-based cohort study. J. Dental Res. 13, 220345211037220. doi: 10.1177/00220345211037220
Manyevitch, R., Protas, M., Scarpiello, S., Deliso, M., Bass, B., Nanajian, A., et al. (2018). Evaluation of metabolic and synaptic dysfunction hypotheses of Alzheimer's disease (AD): a meta-analysis of CSF markers. Curr. Alzheimer Res. 15, 164–181. doi: 10.2174/1567205014666170921122458
Mirzaei, R., Bouzari, B., Hosseini-Fard, S. R., Mazaheri, M., Ahmadyousefi, Y., Abdi, M., et al. (2021). Role of microbiota-derived short-chain fatty acids in nervous system disorders. Biomed. Pharmacother. 139, 111661. doi: 10.1016/j.biopha.2021.111661
Miyake, S., Wada-Takahashi, S., Honda, H., Takahashi, S. S., Sasaguri, K., and Sato, S. (2012). Stress and chewing affect blood flow and oxygen levels in the rat brain. Arch. Oral Biol. 57, 1491–1497. doi: 10.1016/j.archoralbio.2012.06.008
Momose, T., Nishikawa, J., and Watanabe, T. (1997). Effect of mastication on regional cerebral blood flow in humans examined by positron-emission tomography with 15O-labelled water and magnetic resonance imaging. Arch. Oral Biol. 42, 57–61. doi: 10.1016/S0003-9969(96)00081-7
Murakami, A., Hara, T., Yamada-Kubota, C., Kuwahara, M., Ichikawa, T., and Minagi, S. (2021). Lack of occlusal support did not impact amyloid β deposition in APP knock-in mice. J. Prosthodont. Res. 66, 161–166. doi: 10.2186/jpr.JPR_D_20_00205
Naorungroj, S., Schoenbach, V. J., and Wruck, L. (2015). Tooth loss, periodontal disease, and cognitive decline in the Atherosclerosis Risk in Communities (ARIC) study. Commun. Dent. Oral Epidemiol. 43, 47–57. doi: 10.1111/cdoe.12128
Neth, B. J., Mintz, A., Whitlow, C., Jung, Y., Solingapuram Sai, K., Register, T. C., et al. (2020). Modified ketogenic diet is associated with improved cerebrospinal fluid biomarker profile, cerebral perfusion, and cerebral ketone body uptake in older adults at risk for Alzheimer's disease: a pilot study. Neurobiol. Aging 86:54–63. doi: 10.1016/j.neurobiolaging.2019.09.015
Neuhaus, W., Gaiser, F., Mahringer, A., Franz, J., Riethmüller, C., and Förster, C. (2014). The pivotal role of astrocytes in an in vitro stroke model of the blood-brain barrier. Front. Cell. Neurosci. 8, 352. doi: 10.3389/fncel.2014.00352
Noguchi, T., Utsugi, C., and Kashiwayanagi, M. (2017). Soft-diet feeding impairs neural transmission between mitral cells and interneurons in the mouse olfactory bulb. Arch. Oral Biol. 83, 209–213. doi: 10.1016/j.archoralbio.2017.07.015
Okamoto, N., Morikawa, M., Okamoto, K., Habu, N., Hazaki, K., Harano, A., et al. (2010). Tooth loss is associated with mild memory impairment in the elderly: the Fujiwara-kyo study. Brain Res. 1349, 68–75. doi: 10.1016/j.brainres.2010.06.054
Olsen, I., and Yamazaki, K. (2019). Can oral bacteria affect the microbiome of the gut? J. Oral Microbiol. 11, 1586422. doi: 10.1080/20002297.2019.1586422
Ono, Y., Yamamoto, T., Kubo, K., and Onozuka, M. (2010). Occlusion and brain function: mastication as a prevention of cognitive dysfunction. J. Oral Rehabil. 37, 624–640. doi: 10.1111/j.1365-2842.2010.02079.x
Osborne, N. R., Anastakis, D. J., and Davis, K. D. (2018). Peripheral nerve injuries, pain, and neuroplasticity. J. Hand Ther. 31:184–194. doi: 10.1016/j.jht.2018.01.011
Oue, H., Miyamoto, Y., Koretake, K., Okada, S., Doi, K., Jung, C. G., et al. (2016). Tooth loss might not alter molecular pathogenesis in an aged transgenic Alzheimer's disease model mouse. Gerodontology 33, 308–314. doi: 10.1111/ger.12153
Oue, H., Miyamoto, Y., Okada, S., Koretake, K., Jung, C. G., et al. (2013). Tooth loss induces memory impairment and neuronal cell loss in APP transgenic mice. Behav. Brain Res. 252, 318–325. doi: 10.1016/j.bbr.2013.06.015
Pang, Q., Hu, X., Li, X., Zhang, J., and Jiang, Q. (2015). Behavioral impairments and changes of nitric oxide and inducible nitric oxide synthase in the brains of molarless KM mice. Behav. Brain Res. 278, 411–416. doi: 10.1016/j.bbr.2014.10.020
Pang, Q., Wu, Q., Hu, X., Zhang, J., and Jang, Q. (2020). Tooth loss, cognitive impairment and chronic cerebral ischemia. J. Dent. Sci. 15, 84–91. doi: 10.1016/j.jds.2019.09.001
Pei, D., Hu, X., Jin, C., Lu, Y., and Liu, S. (2018). Energy storage and dissipation of human periodontal ligament during mastication movement. ACS Biomater. Sci. Eng. 4, 4028–4035. doi: 10.1021/acsbiomaterials.8b00667
Ryu, J. C., Zimmer, E. R., Rosa-Neto, P., and Yoon, S. O. (2019). Consequences of metabolic disruption in Alzheimer's disease pathology. Neurotherapeutics 16, 600–610. doi: 10.1007/s13311-019-00755-y
Sakamoto, S, Hara, T., Kurozumi, A., Oka, M., Kuroda-Ishimine, C., Araki, D., et al. (2014). Effect of occlusal rehabilitation on spatial memory and hippocampal neurons after long-term loss of molars in rats. J. Oral Rehabil. 41, 715–722. doi: 10.1111/joor.12198
Saruta, J., To, M., Sakaguchi, W., Kondo, Y., and Tsukinoki, K. (2020). Brain-derived neurotrophic factor is related to stress and chewing in saliva and salivary glands. Jpn. Dent. Sci. Rev. 56, 43–49. doi: 10.1016/j.jdsr.2019.11.001
Sesay, M., Tanaka, A., Ueno, Y., Lecaroz, P., and De Beaufort, D. G. (2000). Assessment of regional cerebral blood flow by Xenon-enhanced computed tomography during mastication in humans. Keio J. Med. 49, A125–A128.
Silva, Y. P., Bernardi, A., and Frozza, R. L. (2020). The role of short-chain fatty acids from gut microbiota in gut-brain communication. Front. Endocrinol. 11:25. doi: 10.3389/fendo.2020.00025
Singhrao, S. K., Harding, A., Simmons, T., Robinson, S., Kesavalu, L., and Crean, S. (2014). Oral inflammation, tooth loss, risk factors, and association with progression of Alzheimer's disease. J. Alzheimer's Dis. 42, 723–737. doi: 10.3233/JAD-140387
Sofroniew, M. V. (2013). Multiple roles for astrocytes as effectors of cytokines and inflammatory mediators. Neuroscientist 20, 160–172. doi: 10.1177/1073858413504466
Stewart, R., Stenman, U., Hakeberg, M., Hagglin, C., Gustafson, D., and Skoog, I. (2015). Associations between oral health and risk of dementia in a 37- year follow-up study: the prospective population study of women in Gothenburg. J. Am. Geriatr. Soc. 15, 100–105. doi: 10.1111/jgs.13194
Stratulat, I. S., Cuciureanu, D., and Eva, L. (2014). No. 99 cerebral blood flow in patients with central nervous pathologies associated with an adequate edentation treatment. PM&R 8, S115. doi: 10.1016/j.pmrj.2014.08.231
Su, S., Qi, T., Su, B., Gu, H., Wang, J., and Yang, L. (2014). Tooth loss inhibits neurogenesis in the dentate gyrus of adult mice. Neural Regen. Res. 9, 1606. doi: 10.4103/1673-5374.141786
Sunariani, J., Khoswanto, C., and Irmalia, W. R. (2019). Difference of brain-derived neurotrophic factor expression and pyramid cell count during mastication of food with varying hardness. J. Appl. Oral Sci. 27, e20180182. doi: 10.1590/1678-7757-2018-0182
Tada, A., and Miura, H. (2017). Association between mastication and cognitive status: a systematic review. Arch. Gerontol. Geriatr. 70, 44–53. doi: 10.1016/j.archger.2016.12.006
Takeda, Y., Oue, H., Okada, S., Kawano, A., Koretake, K., Michikawa, M., et al. (2016). Molar loss and powder diet leads to memory deficit and modifies the mRNA expression of brain-derived neurotrophic factor in the hippocampus of adult mice. BMC Neurosci. 17, 81. doi: 10.1186/s12868-016-0319-y
Tan, D., Foster, S., Korgaonkar, M. S., Oxenham, V., Whittle, T., and Klineberg, I. (2020). The role of progressive oral implant rehabilitation in mastication, cognition and oral health-related quality of life outcomes—A pilot to define the protocol. J. Oral Rehabil. 47, 1368–1381. doi: 10.1111/joor.13085
Taslima, F., Jung, C.-. G., Zhou, C., Abdelhamid, M., Abdullah, M., et al. (2021). Tooth loss induces memory impairment and gliosis in app knock-in mouse models of Alzheimer's disease. J. Alzheimers Dis. 80, 1687–1704. doi: 10.3233/JAD-201055
Taylor, K. S., Anastakis, D. J., and Davis, K. D. (2009). Cutting your nerve changes your brain. Brain 132, 3122–3133. doi: 10.1093/brain/awp231
Tsai, C. Y., Lee, H. P., Chang, H. M., and Wu, F. C. (2018). Masticatory hypofunction effects induced by BTXA injection of hippocampal neurons in developing rats. Arch. Oral Biol. 96, 122–129. doi: 10.1016/j.archoralbio.2018.09.005
Tsai, Y. C., Wang, H. J., Wang, L. Y., Shaw, C. K., Lee, Y. P., and Lin, M. C. (2020). Retrospective analysis of the association between tooth loss and dementia: a population-based matched case-control study. Community Dent. Health 37, 59–64.
Wang, K. R., and Ge, S. (2020). Research progress in relationship between tooth loss and Alzheimer's disease. Zhonghua Kou Qiang Yi Xue Za Zhi 55, 586–590. doi: 10.3760/cma.j.cn112144-20191110-00400
Xu, X., Cao, B., Wang, J., Yu, T., and Li, Y. (2015). Decision-making deficits associated with disrupted synchronization between basolateral amygdala and anterior cingulate cortex in rats after tooth loss. Prog. Neuropsychopharmacol. Biol. Psychiatry 60, 26–35. doi: 10.1016/j.pnpbp.2015.02.002
Xue, L., Zou, X., Yang, X. Q., Peng, F., Yu, D. K., and Du, J. R. (2020). Chronic periodontitis induces microbiota-gut-brain axis disorders and cognitive impairment in mice. Exp. Neurol. 326, 113176. doi: 10.1016/j.expneurol.2020.113176
Keywords: tooth loss, cognitive dysfunction, Alzheimer's disease, vascular dementia, neurodegenerative diseases, oxidative stress, mitochondrial autophagy, nerve damage
Citation: Wang X, Hu J and Jiang Q (2022) Tooth Loss-Associated Mechanisms That Negatively Affect Cognitive Function: A Systematic Review of Animal Experiments Based on Occlusal Support Loss and Cognitive Impairment. Front. Neurosci. 16:811335. doi: 10.3389/fnins.2022.811335
Received: 08 November 2021; Accepted: 18 January 2022;
Published: 10 February 2022.
Edited by:
Xinwen Zhang, China Medical University, ChinaReviewed by:
Junjun Ni, Beijing Institute of Technology, ChinaCopyright © 2022 Wang, Hu and Jiang. This is an open-access article distributed under the terms of the Creative Commons Attribution License (CC BY). The use, distribution or reproduction in other forums is permitted, provided the original author(s) and the copyright owner(s) are credited and that the original publication in this journal is cited, in accordance with accepted academic practice. No use, distribution or reproduction is permitted which does not comply with these terms.
*Correspondence: Qingsong Jiang, cXNqaWFuZzc0QGhvdG1haWwuY29t
Disclaimer: All claims expressed in this article are solely those of the authors and do not necessarily represent those of their affiliated organizations, or those of the publisher, the editors and the reviewers. Any product that may be evaluated in this article or claim that may be made by its manufacturer is not guaranteed or endorsed by the publisher.
Research integrity at Frontiers
Learn more about the work of our research integrity team to safeguard the quality of each article we publish.