- 1Center for Neurodegenerative Diseases and the Aging Brain, University of Bari Aldo Moro at Pia Fondazione “Card. G. Panico”, Tricase, Italy
- 2Neurology Unit, Azienda Sanitaria Locale (ASL) Bari, San Paolo Hospital, Bari, Italy
- 3Department of Medical Sciences, Institute of Neurology, Magna Græcia University, Catanzaro, Italy
- 4Department of Translational Biomedicine and Neuroscience (DiBraiN), University of Bari Aldo Moro, Bari, Italy
Amyotrophic lateral sclerosis (ALS) is the most common motor neuron disease and has emerged among the disorders with the largest increasing incidence in Western countries. Although the diagnosis is based on clinical grounds, electromyography (EMG), and nerve conduction studies (NCS) play a crucial role to exclude other potential etiologies of lower motor neuron (LMN) dysfunction. Based on clinical grounds, a peculiar pattern of dissociated atrophy of the intrinsic hand and foot muscles, termed the “split-hand” (SH) and “split-leg” (SL) signs, has been described in a significant proportion of subjects with ALS, even at the early stages of the disease, when symptoms are focal. These signs are rare in neurological and non-neurological diseases other than ALS. In this review, we discussed current evidences concerning SH and SL signs, their pathogenetic hypotheses and neurophysiological findings. We also analyze whether SH and SL signs can be reliable markers in the differential diagnosis and in the prognosis of ALS.
1. Introduction
Amyotrophic lateral sclerosis (ALS) involves upper (UMN) and lower motor neuron (LMN), and is fatal on average within 4 years from onset. The disease onset is often focal, embroiling face or distal segments of arms/legs and steadily advances over time and space (Kiernan et al., 2011). Although the diagnosis is based on clinical grounds, electromyography (EMG) and nerve conduction studies (NCS) help either to rule out LMN dysfunction of different etiologies and to identify LMN signs in muscles not clinically involved (Shefner et al., 2020). Several clinical and neurophysiological studies reported a dissociated pattern of muscle wasting and hypotrophy of intrinsic hand, elbow, and foot muscles in ALS, named respectively, “split-hand” (SH), split-elbow (SE), and “split-leg” (SL) signs (Corcia et al., 2021). In this review, we discuss current evidences, pathogenetic hypotheses and neurophysiological indicators of SH, SE, and SL (Figures 1, 2).
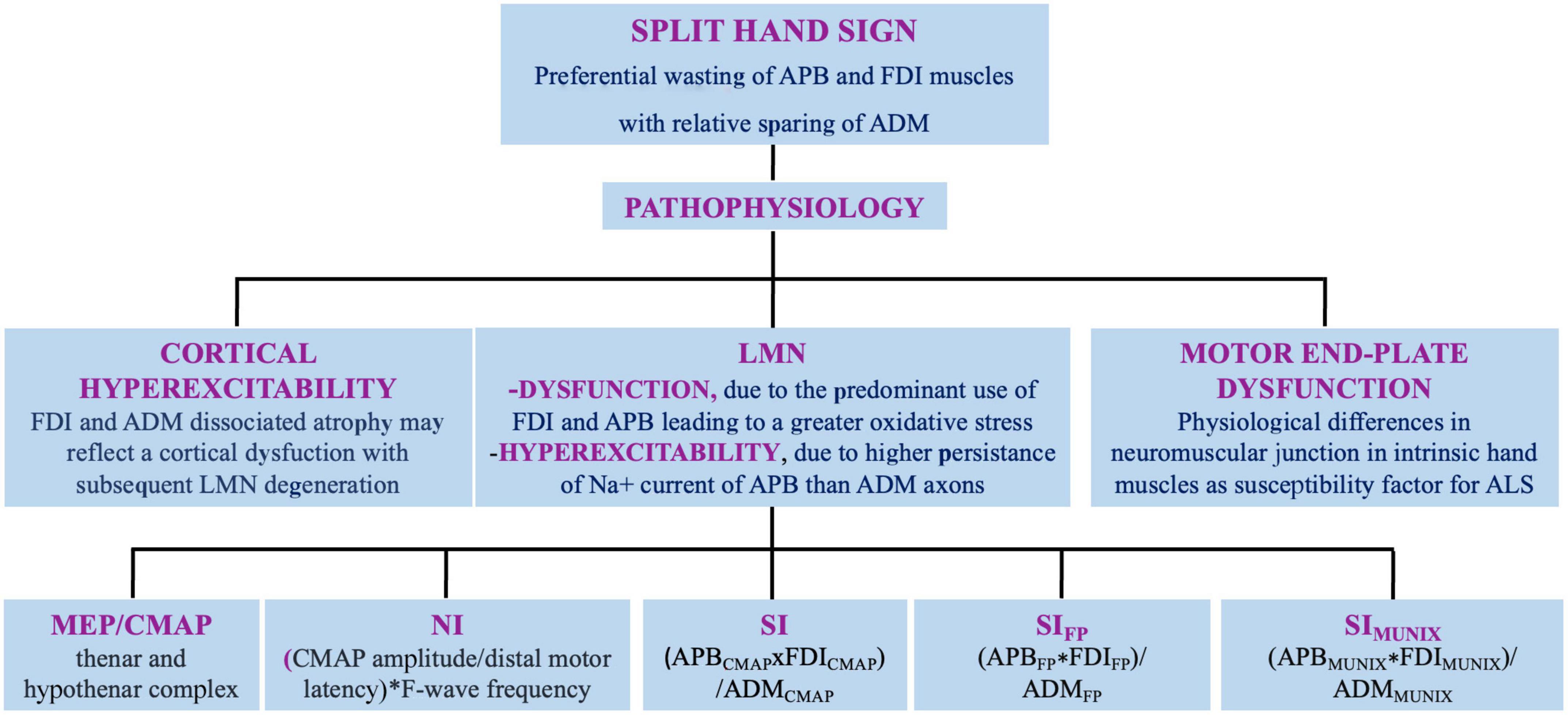
Figure 1. Split hand sign in ALS: definition, pathophysiology, and neurophysiologic indexes. APB, abductor pollicis brevis muscle; FDI, first dorsal interosseus; ADM, abductor digiti minimi muscle; LMN, lower motor neuron; MEP, motor evoked potential; CMAP, compound muscle action potential; NI, neurophysiological index; SI, split hand index; SIFP, SI derived from F-waves; SIMUNIX, SI based on MUNIX software.
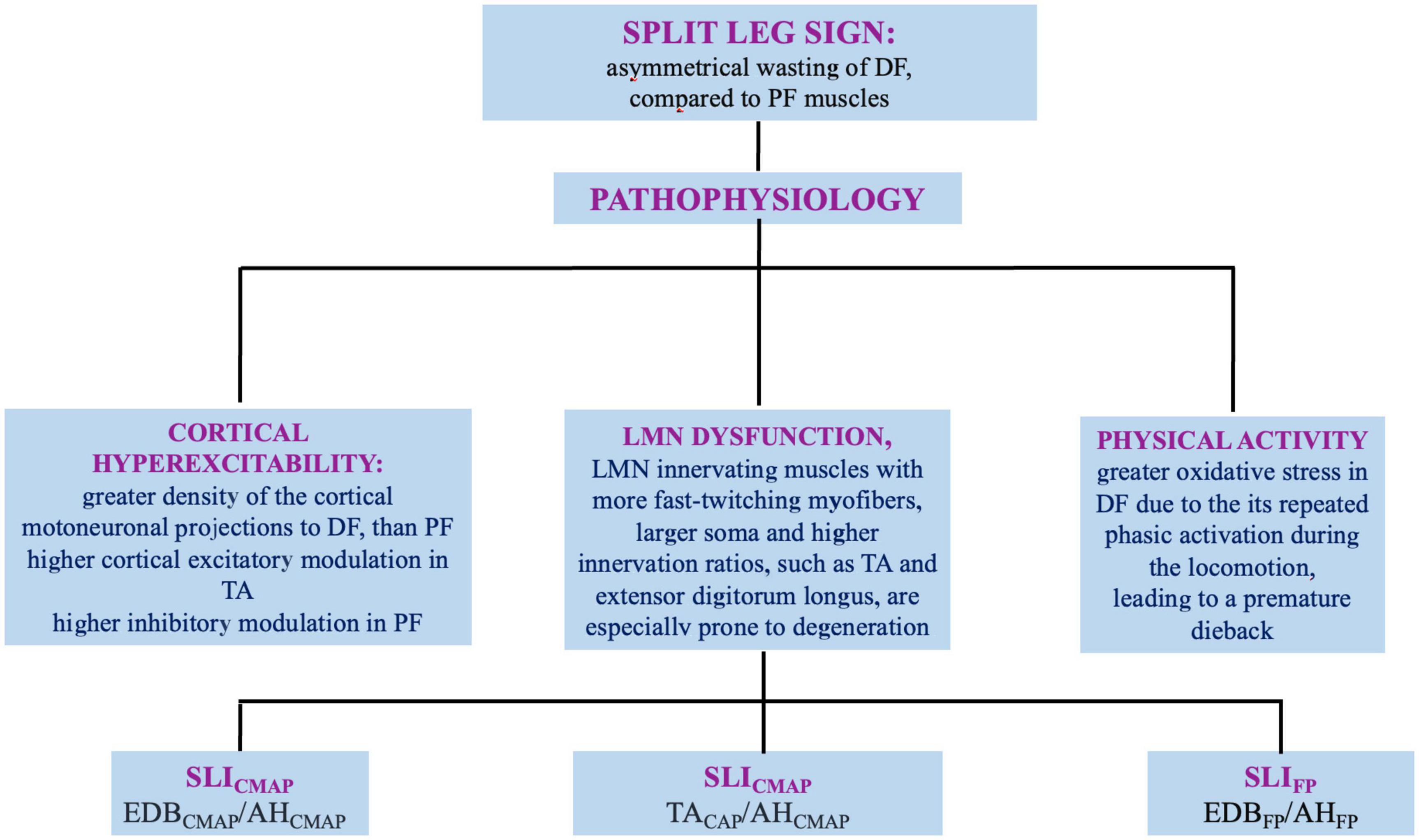
Figure 2. Split leg sign in ALS: definition, pathophysiology, and neurophysiologic indexes. DF, dorsal flexors; PF, plantar flexors; LMN, lower motor neuron; SLI, split leg sign; CMAP, compound muscle action potential; EDB, extensor digitorum brevis; AH, abductor hallucis; TA, tibialis antherior; SLIFP, SLI derived from F-waves.
2. Split-hand sign
The preferential wasting of abductor pollicis brevis (APB) and first dorsal interosseous (FDI), with relative sparing of the abductor digiti minimi (ADM) in ALS was first described in 1994 (Wilbourn and Sweeney, 1994). Further studies defined this phenomenon as the “SH” (Corcia et al., 2021). Independently of the site of onset, approximately 70% of patients with ALS presents SH at diagnosis and almost all cases during the course of the disease (Hu et al., 2021). These findings emphasized a potential role for SH as diagnostic indicator of ALS (Corcia et al., 2021). However, the specificity of SH still remains debated as SH has been reported in other conditions as spinal muscular atrophy, Kennedy’s disease, spinocerebellar ataxia-3 and post-polio syndrome (Schelhaas et al., 2003; Shibuya et al., 2020; Corcia et al., 2021). Moreover, a physiological non-progressive, age-related preferential atrophy of APB and FDI has been described and defined the “senile amyotrophy” (Op de coul, 1970).
2.1. Pathophysiology
The dissociated atrophy of hands muscles does not reflect nerve trunks or root territories. FDI and ADM muscles are both innervated by the ulnar nerve arising from C8–T1 nerve roots. The median nerve innervates APB, and also arises from C8–T1 roots (Corcia et al., 2021). Therefore, four pathophysiologic mechanisms of SH have been proposed (Figure 1).
2.1.1. Corticomotoneuronal dysfunction
The motor cortex may be primarily involved in SH, as although FDI and ADM are innervated by the same myotomes, they depict a dissociated atrophy, that can reflect a cortical mechanism (Weber et al., 2000; Menon et al., 2013b). The intrinsic lateral hand muscles are involved in precision movements that depend on normal corticospinal-tract function (Menon et al., 2013b; Xu and Fan, 2013). APB or FDI cortical motor neurons far outnumber those of ADM (Kuwabara et al., 1999) and their corticospinal connections are more extensive than those of ADM, resulting in greater susceptibility to glutamate excitotoxicity of APB and FDI LMN. The smaller cortical motor evoked potentials (MEPs) obtained from the thenar muscles compared to ADM may indicate a “shrinkage” of cortical representation of the former (Weber et al., 2000). Based on these observations, the dysfunction of corticomotoneuronal network may represent the determinant key of dissociated hand wasting (Weber et al., 2000; Corcia et al., 2021). Other neurophysiological studies, however, argued against this hypothesis, finding no significant difference between FDI and ADM in the cutaneous silent period elicited stimulating a digital sensory nerve in patients with ALS (Cengiz et al., 2018).
2.1.2. LMN dysfunction
SH may reflect the predominant use of thumb and index fingers, that leads to a greater oxidative stress or metabolic demand on APB and FDI LMN (Weber et al., 2000; Corcia et al., 2021). The hypothesized greater resistance to degeneration of ADM LMN may be related to a greater reinnervation capacity of its axons (Murray and Jankelowitz, 2011; de Carvalho and Swash, 2019; Corcia et al., 2021).
2.1.3. LMN hyperexcitability
Experimental studies on peripheral axonal excitability evidenced that in normal subjects the Na+ current of APB motor-axons are more persistent than those of ADM. Consistently, case-control studies demonstrated that the hyperexcitability in ALS is predominant in APB, compared to ADM axons (Shibuya et al., 2013, 2020). However, in other studies excitability changes did not follow a SH pattern, as the increase in axons excitability resulted similar in APB and ADM, arguing against a peripheral contribution (Menon et al., 2013b; Menon and Vucic, 2014).
2.1.4. Motor-end plate dysfunction
A single repetitive nerve stimulation study was conducted in patients with ALS with normal strength hand, normal median, and ulnar compound muscle action potentials (CMAPs) and in normal subjects. In patients with ALS the CMAPs decrement resulted higher in ABD and FDI compared to ADM, whereas the decremental pattern resulted similar in all muscles in controls. Authors hypothesized that differences in neuromuscular junctions in intrinsic hand muscles mirroring SH sign can be a susceptibility factor for ALS (de Carvalho and Swash, 2019).
2.2. Neurophysiology
Several neurophysiological methods have been used through the years to quantify the SH (Figure 1 and Table 1). The first indicator was a cortical/peripheral ratio of the thenar and hypothenar muscles, obtained by peripheral nerve and transcranial magnetic stimulations (TMSs). Compared to controls, the ratio resulted significantly lower in the thenar but not in the hypothenar complex in ALS (Weber et al., 2000). A further approach proposed a “neurophysiological index” (NI) from ulnar nerve:
Neurophysiological index resulted reproducible, sensitive to change, and correlated with ADM weakness, with a sensitivity higher than clinical measures in detecting LMN loss in asymptomatic limbs. NI had a sensitivity at least similar to revised-ALS functional rating scale (ALSFRS-R) in differentiating rapid from slowly progressive ALS (de Carvalho and Swash, 2000, 2019; Swash and de Carvalho, 2004). Another study used a NI deriving from APB, with the same formula with similar results (Xu and Fan, 2013). Several other ratios have been further proposed, using CMAP amplitudes of APB or FDI and ADM in various combinations. The most widely adopted were,
With cut-off values: 0.6 and 0.9, respectively. These indexes resulted useful to differentiate ALS from mimic disorders in some studies (Kuwabara et al., 1999; Menon et al., 2013b; Menon and Vucic, 2014, Corcia et al., 2021), while others found low sensitivity values (Table 1, Wang et al., 2020). Thereafter, a split-hand index (SI) was developed to improve the diagnostic accuracy of SH in ALS (Menon et al., 2013b). The SI is calculated applying the following formula (Menon et al., 2013b; Menon and Vucic, 2014):
The diagnostic accuracy of SI has been confirmed in a large study comparing ALS to mimicking syndromes, with a cut-off value of < 5.2 demonstrating sensitivity of 74% and specificity of 80%, (Menon et al., 2013b). Further studies gave similar results, but using heterogeneous cut-off values (from <5.2 to >10, Table 1) (Kuwabara et al., 1999; Corcia et al., 2021). A recent meta-analysis of available studies identified a cut-off of < 7.4 in patients with a disease duration of 8–20 months (Lu et al., 2022). SI resulted significantly reduced in more than 60% of patients with ALS who did not meet Awaji diagnostic criteria, suggesting a diagnostic role of SI in the earliest stage (Schelhaas et al., 2003; Menon et al., 2013b; Menon and Vucic, 2014). Both NI and SI weakly correlated with strength scores of the corresponding muscles, probably due to the low sensitivity of the manual strength tests, as subjects with reduction of CMAP > 50% still display normal strength (Menon et al., 2013b; Menon and Vucic, 2014). NI and SI significantly correlated with measures of functional status (Menon et al., 2013b; Corcia et al., 2021). The combination of SI and disease duration represented the best predictor of the disease progression. The resulting SI prognostic index significantly correlated with progression and survival in a cohort of patients with ALS (Xu and Fan, 2013; Barp et al., 2021). An adapted version of SI was recently obtained from F-waves persistence (SIFP) (Wang et al., 2020):
SIFP resulted a sensitive diagnostic neurophysiological indicator. SIFP (cut-off value: 73.3) improved diagnostic performances compared to SICMAP, even in the early stage of the disease (sensitivity of 86% vs. 76%) (Wang et al., 2020). However, the need to deliver 100 supramaximal stimuli for each muscle limits its usefulness in clinical practice. Another non-invasive approach to quantify the SH phenomenon is the motor-unit number index (MUNIX) (Higashihara et al., 2020). MUNIX is a simple commercialized software, based on a standardized technique to quantify MUN. MUNIX value is derived from the CMAP area and surface EMG interference pattern. A MUNIX based index (SIMUNIX) obtained as (Higashihara et al., 2020):
SIMUNIX demonstrated high sensitivity (95%) and specificity (84%) and capable to detected SH even when CMAPs were unremarkable (Kim et al., 2016). SIMUNIX appeared more encouraging than SICMAP in distinguishing ALS from cervical spondylotic amyotrophy (Zheng et al., 2019), but further confirmations are required.
All the above-mentioned neurophysiological parameters present, however, several limitations. The most important is the confounding contribute of aging to the intrinsic hand amyotrophy. In a recent study on patients without neuromuscular disorders, the authors found that APB, FDI, and ADM CMAPs decreased with age, approximately of 0.8/0.7/0.3 mV/year, respectively. Consistently, the SI decreased of about 0.15/year (Pechirra et al., 2022). Another potential confounding factor is the coexistence of median or ulnar entrapment neuropathies. Therefore, these indexes should be evaluated just in an extensive neurophysiological and clinical investigation (Corcia et al., 2021). Overall, SH seems a valuable tool in the diagnosis of ALS, but larger and longitudinal studies are required to identify a proper age-related neurophysiologic index (Lu et al., 2022).
3. Split-hand plus sign
In addition to the above mentioned observations, another intrinsic hand muscle seems to be relatively spared in ALS, the flexor pollicis longus (FPL). FLP although share the same innervation of APB from C8–T1 and median nerve, seems to be less involved in ALS (Menon et al., 2013a). This distinctive clinical feature has been called the “SH plus sign” (SHPS). However, the SHPS usefulness in clinical practice remains still poor characterized (Bae et al., 2014).
3.1. Pathophysiology
Although the mechanisms underlying SHPS in ALS remains to be elucidated, a cortical mechanism has been proposed. The thenar muscles play a critical role in fine movements, due to their wider cortical representation (Bae et al., 2013). Therefore, they are more susceptible than FPL to degeneration mediated by corticomotor neurons. Supporting this hypothesis is the significantly reduced cortical excitability from FPL rather than from APB, that gives evidence that FPL muscle receive a lower cortical output (Bae et al., 2013).
3.2. Neurophysiology
To date SHPS has been quantified only clinically, using the score at Medical Research Council (MRC) of APB divided by the score at MRC of FPL muscles, as median nerve strength index (MSI):
A cut-off of 0.9 exhibited a good diagnostic accuracy (sensitivity: 85%; specificity: 86%), differentiating ALS from ALS mimic disorders. However, its preferential reduction in limb-onset ALS potentially limits its utility (Menon et al., 2013b). A subsequent study investigated the pathophysiology underlying SHPS. The authors calculated the corresponding NI as follows:
The SHPI was significantly reduced in ALS patients compared to controls. Interestingly, SHPI also demonstrated an inverse correlation with MEP amplitudes recorded over thenar muscle, supporting the cortical origin of SHPS in ALS (Bae et al., 2014). However, a clear cut-off of SHPI is still not available.
4. Split-elbow sign
Among the dissociated patterns of atrophy, Khalaf et al. (2019) proposed the “SE sign” (SES) to indicate the predominant weakness in ALS of biceps brachii (BB) compared to triceps brachii (TB) muscle. The SES is calculated as follows:
Khalaf demonstrated that BB exhibited a smaller MRC score than TB muscle in a cohort of ALS patients (Khalaf et al., 2019). However, a subsequent study found opposite results, with a relative sparing of elbow flexion (Liu et al., 2021) with a predominant involvement of TB.
4.1. Pathophysiology
The SES further highlights the cortical hyperexcitability as potential mechanism underlying ALS, due to the wider cortical representation of BB than TB and its higher susceptibility to wasting and weakness in ALS (Khalaf et al., 2019; Pavey et al., 2021). Nonetheless, other pathophysiologic hypotheses, as the axonal hyperexcitability or the dysfunction of neuromuscular junction cannot be disclosed (Khalaf et al., 2019; Pavey et al., 2021).
4.2. Neurophysiology
The SES-related neurophysiologic index, the “SE index (SEI)” has been recently developed and is calculated, dividing the CMAP amplitude of BB by the CMAP of TB muscle, as follows:
The SEI, with a cut-off of 0.65 (sensitivity of 71%; specificity of 61%) was significantly reduced in subjects with ALS compared to ALS mimicking disorders, regardless of the hand dominance. SEI may represent an early marker of disease, as it is more frequent in subjects with mild reduction in ALSFRS-R score. However, its usefulness in distinguishing ALS from mimic disorders is lower when the ALSFRS-R score is higher than 38. Larger studies may further contribute to define its utility in clinical settings and broaden ALS pathophysiology (Pavey et al., 2021).
5. Split-leg sign
In ALS, several studies reported an unbalanced muscular involvement in the lower-limbs during the course of the illness (Simon et al., 2015; Hu et al., 2019; Min et al., 2020). Simon et al. (2015) first found a significant asymmetrical wasting of plantar flexor (PF) compared to ankle dorsiflexion muscles (DF). Subsequent studies did not confirm such observation, but identified a preferential degeneration in extensor digitorum brevis (EDB) and tibialis anterior (TA) muscles, compared to abductor hallucis (AH) (Hu et al., 2019; Min et al., 2020), defining it as the “split-leg sign” (SL). Consistent with the SL sign, the foot-drop due to DF weakness is a common clinical manifestation and may be the prominent feature of lower-limbs involvement in ALS (Hu et al., 2019). On the other hand, several disorders may produce a foot-drop, including peroneal neuropathy at the fibular-head, lumbar spondylosis disease, and peripheral neuropathies (Stewart, 2008; Hu et al., 2019).
5.1. Pathophysiology
The mechanisms underlying the predominant involvement of EDB than AH are still not fully elucidated and a multifactorial origin has been assumed (Figure 2).
5.1.1. Corticomotoneuronal dysfunction
The foot-drop represents a prominent feature of corticospinal tract injury. The greater density of the cortical motoneuronal projections to DF rather than PF muscles, may contribute to the pathogenesis of the SL (Brouwer and Ashby, 1992). Experimental studies evidenced higher cortical excitatory modulation in TA and higher inhibitory modulation in PF (Hudson et al., 2013). Although available human data are limited, TMS identified short-latency facilitation in motoneurons innervating TA and other small muscles of the foot, but rarely in soleus and gastrocnemius UMN (Hudson et al., 2013). Functional MRI during ankle DF/PF revealed a broader cortical representation for DF (Jiang et al., 2012). Thus, the corticomotoneuronal hyperexcitability is a key mechanism in degeneration of LMN involved in ankle dorsiflexors, that receive a greater innervation.
5.1.2. LMN dysfunction
Lower motor neuron innervating muscles with more fast-twitching myofibers, larger soma and higher innervation ratios, such as TA and extensor digitorum longus, are especially prone to degeneration (Nijssen et al., 2017). Supporting this hypothesis are: the greater reduction of SL index (SLI) in patients with pure LMN diseases than in patients with ALS (Min et al., 2020); the predominant F-wave dysfunction in EDB compared to AH observed in patients with ALS. This finding could reflect a more severe hyperexcitability and neuronal loss among the EDB LMN, even in asymptomatic legs (Wang et al., 2019). Therefore, the LMN innervating the EDB may be involved in ALS earlier and deeper than those innervating the AH.
5.1.3. The influence of physical activity
Although lower-limbs activity generally involves tonic activation of the glutei, vasti, and PF muscles during standing and walking, some studies observed a significant correlation between ALSFRS-R lower-limbs subscores and ankle DF strength rather than PF strength. The DF muscles may suffer from a greater oxidative stress (Liu et al., 2006). During the locomotion, the repeated phasic activation of DF might produce a greater oxidative stress in these antigravity muscles, leading to a premature dieback (Spiller et al., 2016). Further research should identify whether SL reflect a spinal mechanism or a down-stream process that secondarily involves LMN from cortical pathophysiology.
5.2. Neurophysiology
The SL has been quantified by several neurophysiological methods (Figure 2). Overall, the SLI corresponds to CMAP ratio of peroneal and tibial nerves (Simon et al., 2015; Hu et al., 2019; Min et al., 2020):
Initially, the CMAP of peroneal and tibial nerves were, respectively recorded from TA and gastrocnemius. The higher mean SLI observed in ALS than controls suggested a dissociated involvement of lower-limbs with a greater reduction of PFCMAP than DFCMAP (Simon et al., 2015). However, due to the deepness of tibial nerve in the popliteal fossa, the nerve stimulation in this site may not excite all nerve fibers (Min et al., 2020). This technical issue produces an unusual variability of SLI, that collides with the common observation of foot-drop in patients with ALS (Hu et al., 2019; Min et al., 2020). Other authors therefore calculated the SLI recording peroneal and tibial nerve CMAPs from the belly of EDB and AH muscles (Hu et al., 2019; Min et al., 2020):
This SLI resulted lower in patients with ALS than in subjects with lumbar spondylosis, normal and neurological controls (Hu et al., 2019; Wang et al., 2019; Min et al., 2020), whereas no difference in SLI was observed between subjects with lumbar spondylosis, normal and disease controls (Min et al., 2020). The greater DFCMAP reduction mainly occurs in ALS, whilst in lumbar spondylosis and in neurological controls, tibial and peroneal innervated muscles are affected simultaneously and symmetrically (Min et al., 2020). In a receiver operating characteristic (ROC) analysis, SLI (cut-off: 0.52) demonstrated a sensitivity of 80% and a specificity of 72% for ALS, resulting very useful for the differential diagnosis (Min et al., 2020). Indeed, in clinical practice lower limb-onset ALS can be easily misdiagnosed as lumbar spondylosis, especially for non-neuromuscular neurologists. In another study, SLI (cut-off: 0.61) failed in differentiating ALS from controls: sensitivity and specificity resulted of 67 and 54%, respectively (Wang et al., 2019). In a case/control study, the F-wave derived SLI (EDB/AH) demonstrated a higher sensitivity than the corresponding SLICMAP (Wang et al., 2019). Moreover, data about the clinical and prognostic implications of SLI are limited and conflicting. SLI inversely correlated with the duration of lower-limbs involvement (Hu et al., 2019), but did not correlate with muscle strength and functional outcomes (ALSFRS total score and lower-limbs sub-scores), suggesting that SLI does not link with lower-limbs disability (Menon and Vucic, 2014; Simon et al., 2015; Hu et al., 2019). Other studies observed instead a positive correlation between SLI and functional scales (Menon and Vucic, 2014; Corcia et al., 2021). The weak correlation between SLI and strength scores of the corresponding muscles could be due to the low sensitivity of the manual strength tests (Menon and Vucic, 2014), as for SI. Based on all the above-mentioned observations, the usefulness of SLI for an earlier diagnosis of ALS and its clinical correlations need to be further elucidated.
6. Discussion
This review indicates that the dissociated wasting of intrinsic hand and foot muscles is common in ALS. SH and SL represent typical manifestations of ALS, particularly in the earliest stages, when clinical signs are focal. The highest amount of data belongs to the SH, while the SL (as well as SE and SHP signs) is actually poorly characterized. Although several NIces have tried to assess the SH, the SICMAP represents the most reliable marker and may help to differentiate ALS from mimic syndromes (Hu et al., 2019; Corcia et al., 2021).
On the other hand, the diagnostic usefulness of SLICMAP still remains debated (Simon et al., 2015; Liu et al., 2021; Wang et al., 2019; Min et al., 2020). In the largest case-control studies SLI was calculated by recording CMAPs from EDB and AH muscles, resulting useful for the differential diagnosis of ALS in most, but not all the studies (Simon et al., 2015; Hu et al., 2019; Wang et al., 2019; Min et al., 2020). The application of SLI in the clinical practice could be relevant, as the lower-limbs ALS can be misdiagnosed as lumbar spondylosis. Several observations suggested also a prognostic role for SI, rather than SLI, as it correlates with disease progression and prognosis (Corcia et al., 2021). Finally, just preliminary observations on SE and SHPS are available and no firm conclusion can be actually drawn.
The diagnostic role of CMAP driven SI and SLI is supported by several studies and do not need a sophisticated neurophysiological equipment. Other neurophysiological methods, although potentially more sensitive, requires additional software (e.g., MUNIX) or are less likely to be performed in the routine neurophysiological assessment (F-waves persistence driven). Nevertheless, some possible confounding factors, such as the mononeuropathies of median nerve at wrist and the peroneal neuropathy at the fibular head, should be addressed in the evaluation of SI and SLI. Normative values of SI and SLI are also uncertain, as available data are extremely variable and the contribute of aging is still unclear (Corcia et al., 2021; Pechirra et al., 2022). Finally, the underlying mechanisms of SLI and SLI are also undetermined, and current evidences indicate an involvement of both cortical and spinal/peripheral pathways. The higher use in humans of APB, FDI, TA, and EDB muscles, compared to ADM, AH, gastrocnemius, leads to a greater oxidative stress and metabolic demand for both UMN and LMN innervating such muscles. This could explain the typical topography of intrinsic hand and foot muscle hypotrophies and the presence of SH in MND without UMN involvement, as Kennedy’s disease. Overall, SI and SLI are reliable neurophysiological markers. Although diagnostic cut-offs are uncertain, their diffuse use in clinical settings may provide new insight into the pathophysiology of ALS. Further studies are, however, needed to clarify their pathophysiology and clinical correlations.
Author contributions
All authors listed have made a substantial, direct, and intellectual contribution to the work, and approved it for publication.
Funding
This work was supported with the founding of Regione Puglia and CNR for Tecnopolo per la Medicina di Precisione. D.G.R. n. 2117 of 21.11.2018 (CUPB84I18000540002)–C.I.R.E.M.I.C. (Research Center of Excellence for Neurodegenerative Diseases and Brain Aging)–University of Bari “Aldo Moro.”
Conflict of interest
The authors declare that the research was conducted in the absence of any commercial or financial relationships that could be construed as a potential conflict of interest.
Publisher’s note
All claims expressed in this article are solely those of the authors and do not necessarily represent those of their affiliated organizations, or those of the publisher, the editors and the reviewers. Any product that may be evaluated in this article, or claim that may be made by its manufacturer, is not guaranteed or endorsed by the publisher.
Abbreviations
ADM, abductor digiti minimi muscle; AH, abductor hallucis; ALS, amyotrophic lateral sclerosis; ALSFRS-R, revised-ALS functional rating scale; APB, abductor pollicis brevis muscle; BB, biceps brachii; CMAP, compound muscle action potential; DF, ankle dorsiflexion muscles; EDB, extensor digitorum brevis; EMG, electromyography; FDI, first dorsal interosseous; FPL, flexor pollicis longus; LMN, lower motor neuron; MEP, motor evoked potential; MRC, Medical Research Council; MSI, median nerve strength index; MUNIX, motor-unit number index; NCS, nerve conduction studies; NI, neurophysiological index; PF, plantar flexor muscles; SES, split-elbow sign; SEI, split-elbow index; SH, split-hand sign; SHPS, split-hand plus sign; SHPI, split-hand plus index; SI, split-hand index; SIFP, SI recorded from F-waves persistence; SIMUNIX, SI based on MUNIX software; SL, split-leg sign; SLI, split-leg index; TA, tibialis anterior; TB, triceps brachii; UMN, upper motor neuron.
References
Bae, J. S., Menon, P., Mioshi, E., Kiernan, M. C., and Vucic, S. (2013). Cortical excitability differences between flexor pollicis longus and APB. Neurosci. Lett. 541, 150–154. doi: 10.1016/j.neulet.2013.03.003
Bae, J. S., Menon, P., Mioshi, E., Kiernan, M. C., and Vucic, S. (2014). Cortical hyperexcitability and the split-hand plus phenomenon: Pathophysiological insights in ALS. Amyotroph. Lateral Scler. Frontotemporal Degener. 15, 250–256. doi: 10.3109/21678421.2013.872150
Barp, A., Lizio, A., Gerardi, F., Tarlarini, C., Mauro, L., Sansone, V. A., et al. (2021). Neurophysiological indices in amyotrophic lateral sclerosis correlate with functional outcome measures, staging and disease progression. Clin. Neurophysiol. 132, 1564–1571. doi: 10.1016/j.clinph.2021.02.394
Brouwer, B., and Ashby, P. (1992). Corticospinal projections to lower limb motoneurons in man. Exp. Brain Res. 89, 649–654. doi: 10.1007/BF00229889
Cengiz, B., Mercan, M., and Kuruoğlu, R. (2018). Spinal excitability changes do not influence the mechanisms of split-hand syndrome in amyotrophic lateral sclerosis. Muscle Nerve 58, 503–508. doi: 10.1002/mus.26123
Corcia, P., Bede, P., Pradat, P. F., Couratier, P., Vucic, S., and de Carvalho, M. (2021). Split-hand and split-limb phenomena in amyotrophic lateral sclerosis: Pathophysiology, electrophysiology and clinical manifestations. J. Neurol. Neurosurg. Psychiatry 92, 1126–1130. doi: 10.1136/jnnp-2021-326266
de Carvalho, M., and Swash, M. (2000). Nerve conduction studies in amyotrophic lateral sclerosis. Muscle Nerve 23, 344–352. doi: 10.1002/(sici)1097-4598(200003)23:3<344::aid-mus5<3.0.co;2-n
de Carvalho, M., and Swash, M. (2019). The split hand in amyotrophic lateral sclerosis: A possible role for the neuromuscular junction. Amyotroph. Lateral Scler. Frontotemporal Degener. 20, 368–375. doi: 10.1080/21678421.2019.1606245
Higashihara, M., Menon, P., van den Bos, M., Pavey, N., and Vucic, S. (2020). Reproducibility of motor unit number index and MScanFit motor unit number estimation across intrinsic hand muscles. Muscle Nerve 62, 192–200. doi: 10.1002/mus.26839
Hu, F., Jin, J., Chen, Q., Kang, L., Jia, R., Qin, X., et al. (2019). Dissociated lower limb muscle involvement in amyotrophic lateral sclerosis and its differential diagnosis value. Sci. Rep. 9:17786. doi: 10.1038/s41598-019-54372-y
Hu, N., Wang, J., and Liu, M. (2021). Split hand in amyotrophic lateral sclerosis: A systematic review and meta-analysis. J. Clin. Neurosci. 90, 293–301. doi: 10.1016/j.jocn.2021.06.015
Hudson, H. M., Griffin, D. M., Belhaj-Saïf, A., and Cheney, P. D. (2013). Cortical output to fast and slow muscles of the ankle in the rhesus macaque. Front. Neural Circuits 7:33. doi: 10.3389/fncir.2013.00033
Jiang, T., Wu, W., Wang, X., Weng, C., Wang, Q., and Guo, Y. (2012). Activation of brain areas following ankle dorsiflexion versus plantar flexion: Functional magnetic resonance imaging verification. Neural Regener. Res. 7, 501–505. doi: 10.3969/j.issn.1673-5374.2012.07.004
Khalaf, R., Martin, S., Ellis, C., Burman, R., Sreedharan, J., Shaw, C., et al. (2019). Relative preservation of triceps over biceps strength in upper limb-onset ALS: The ‘split elbow’. J. Neurol. Neurosurg. Psychiatry 90, 730–733. doi: 10.1136/jnnp-2018-319894
Kiernan, M. C., Vucic, S., Cheah, B. C., Turner, M. R., Eisen, A., Hardiman, O., et al. (2011). Amyotrophic lateral sclerosis. Lancet 377, 942–955. doi: 10.1016/S0140-6736(10)61156-7
Kim, D. G., Hong, Y. H., Shin, J. Y., Park, K. H., Sohn, S. Y., Lee, K. W., et al. (2016). Split-hand phenomenon in amyotrophic lateral sclerosis: A motor unit number index study. Muscle Nerve 53, 885–888. doi: 10.1002/mus.24958
Kim, J. E., Hong, Y. H., Lee, J. H., Ahn, S. W., Kim, S. M., Park, K. S., et al. (2015). Pattern difference of dissociated hand muscle atrophy in amyotrophic lateral sclerosis and variants. Muscle Nerve 51, 333–337. doi: 10.1002/mus.24323
Kuwabara, S., Mizobuchi, K., Ogawara, K., and Hattori, T. (1999). Dissociated small hand muscle involvement in amyotrophic lateral sclerosis detected by motor unit number estimates. Muscle Nerve 22, 870–873. doi: 10.1002/(sici)1097-4598(199907)22:7<870::aid-mus9<3.0.co;2-o
Liu, J., Wang, Z., Shen, D., Yang, X., Liu, M., and Cui, L. (2021). Split phenomenon of antagonistic muscle groups in amyotrophic lateral sclerosis: Relative preservation of flexor muscles. Neurol. Res. 43, 372–380. doi: 10.1080/01616412.2020.1866354
Liu, M. Q., Anderson, F. C., Pandy, M. G., and Delp, S. L. (2006). Muscles that support the body also modulate forward progression during walking. J. Biomech. 39, 2623–2630. doi: 10.1016/j.jbiomech.2005.08.017
Lu, W. Z., Lin, H. A., Hou, S. K., Lee, C. F., Bai, C. H., and Lin, S. F. (2022). Split-hand index for amyotrophic lateral sclerosis diagnosis: A frequentist and Bayesian meta-analysis. Clin. Neurophysiol. 143, 56–66. doi: 10.1016/j.clinph.2022.08.020
Menon, P., Kiernan, M. C., Yiannikas, C., Stroud, J., and Vucic, S. (2013b). Split-hand index for the diagnosis of amyotrophic lateral sclerosis. Clin. Neurophysiol. 124, 410–416. doi: 10.1016/j.clinph.2012.07.025
Menon, P., Bae, J. S., Mioshi, E., Kiernan, M. C., and Vucic, S. (2013a). Split-hand plus sign in ALS: Differential involvement of the flexor pollicis longus and intrinsic hand muscles. Amyotroph. Lateral Scler. Frontotemporal Degener. 14, 315–318. doi: 10.3109/21678421.2012.734521
Menon, P., and Vucic, S. (2014). Utility of dissociated intrinsic hand muscle atrophy in the diagnosis of amyotrophic lateral sclerosis. J. Vis. Exp. 51056. doi: 10.3791/51056
Min, Y. G., Choi, S. J., Hong, Y. H., Kim, S. M., Shin, J. Y., and Sung, J. J. (2020). Dissociated leg muscle atrophy in amyotrophic lateral sclerosis/motor neuron disease: The ‘split-leg’ sign. Sci. Rep. 10:15661. doi: 10.1038/s41598-020-72887-7
Murray, J. E., and Jankelowitz, S. K. (2011). A comparison of the excitability of motor axons innervating the APB and ADM muscles. Clin. Neurophysiol. 122, 2290–2293. doi: 10.1016/j.clinph.2011.04.007
Nijssen, J., Comley, L. H., and Hedlund, E. (2017). Motor neuron vulnerability and resistance in amyotrophic lateral sclerosis. Acta Neuropathol. 133, 863–885. doi: 10.1007/s00401-017-1708-8
Op de coul, A. A. W. (1970). The atrophy of small hand muscles. Ph.D. thesis. Asten: Schriks drukkerji.
Pavey, N., Higashihara, M., van den Bos, M. A. J., Menon, P., and Vucic, S. (2021). The split-elbow index: A biomarker of the split elbow sign in ALS. Clin. Neurophysiol. Pract. 7, 16–20. doi: 10.1016/j.cnp.2021.11.002
Pechirra, G., Swash, M., and de Carvalho, M. (2022). The senile hand: Age effects on intrinsic hand muscle CMAP amplitudes influence split-hand index calculations. Muscle Nerve 65, 463–467. doi: 10.1002/mus.27489
Schelhaas, H. J., van de Warrenburg, B. P., Kremer, H. P., and Zwarts, M. J. (2003). The “split hand” phenomenon: Evidence of a spinal origin. Neurology 61, 1619–1620. doi: 10.1212/01.wnl.0000096009.50213.6c
Shefner, J. M., Al-Chalabi, A., Baker, M. R., Cui, L. Y., de Carvalho, M., Eisen, A., et al. (2020). A proposal for new diagnostic criteria for ALS. Clin. Neurophysiol. 131, 1975–1978. doi: 10.1016/j.clinph.2020.04.005
Shibuya, K., Misawa, S., Nasu, S., Sekiguchi, Y., Mitsuma, S., Beppu, M., et al. (2013). Split hand syndrome in amyotrophic lateral sclerosis: Different excitability changes in the thenar and hypothenar motor axons. J. Neurol. Neurosurg. Psychiatry 84, 969–972. doi: 10.1136/jnnp-2012-304109
Shibuya, K., Misawa, S., Uzawa, A., Sawai, S., Tsuneyama, A., Suzuki, Y. I., et al. (2020). Split hand and motor axonal hyperexcitability in spinal and bulbar muscular atrophy. J. Neurol. Neurosurg. Psychiatry 91, 1189–1194. doi: 10.1136/jnnp-2020-324026
Simon, N. G., Lee, M., Bae, J. S., Mioshi, E., Lin, C. S., Pfluger, C. M., et al. (2015). Dissociated lower limb muscle involvement in amyotrophic lateral sclerosis. J. Neurol. 262, 1424–1432. doi: 10.1007/s00415-015-7721-8
Spiller, K. J., Cheung, C. J., Restrepo, C. R., Kwong, L. K., Stieber, A. M., Trojanowski, J. Q., et al. (2016). Selective motor neuron resistance and recovery in a new inducible mouse model of TDP-43 proteinopathy. J. Neurosci. 36, 7707–7717. doi: 10.1523/JNEUROSCI.1457-16.2016
Stewart, J. D. (2008). Foot drop: Where, why and what to do? Pract. Neurol. 8, 158–169. doi: 10.1136/jnnp.2008.149393
Swash, M., and de Carvalho, M. (2004). The neurophysiological index in ALS. Amyotroph. Lateral Scler. Other Motor Neuron Disord. 5(Suppl. 1) 108–110. doi: 10.1080/17434470410020067
Wang, Z. L., Cui, L., Liu, M., Zhang, K., Liu, S., Ding, Q., et al. (2019). Reassessment of split-leg signs in amyotrophic lateral sclerosis: Differential involvement of the extensor digitorum brevis and abductor hallucis muscles. Front. Neurol. 10:565. doi: 10.3389/fneur.2019.00565
Wang, Z. L., Liu, M., Cai, Z., Ding, Q., Hu, Y., and Cui, L. (2020). A prospective study on split-hand index as a biomarker for the diagnosis of amyotrophic lateral sclerosis. Amyotroph. Lateral Scler. Frontotemporal Degener. 21, 574–583. doi: 10.1080/21678421.2020.1779299
Weber, M., Eisen, A., Stewart, H., and Hirota, N. (2000). The split hand in ALS has a cortical basis. J. Neurol. Sci. 180, 66–70. doi: 10.1016/s0022-510x(00)00430-5
Wilbourn, A. J., and Sweeney, P. J. (1994). Dissociated wasting of medial and lateral hand muscles with motor neuron disease. Can. J. Neurol. Sci. 21, (Suppl. 2):59.
Xu, L. Q., Hu, W., Guo, Q. F., Lai, L. L., Xu, G. R., Chen, W. J., et al. (2020). Median nerve-neurophysiological index correlates with the survival of patients with amyotrophic lateral sclerosis. Front. Neurol. 11:570227. doi: 10.3389/fneur.2020.570227
Xu, Y. S., and Fan, D. S. (2013). [Clinical and neuro electrophysiologic study of flail arm syndrome] Chinese. Zhonghua Yi Xue Za Zhi 93, 23–25.
Keywords: amyotrophic lateral sclerosis, motor neuron, split-hand sign, split-leg sign, neurophysiology, split-hand index, split-leg index, split-elbow index
Citation: Zoccolella S, Giugno A and Logroscino G (2023) Split phenomena in amyotrophic lateral sclerosis: Current evidences, pathogenetic hypotheses and diagnostic implications. Front. Neurosci. 16:1100040. doi: 10.3389/fnins.2022.1100040
Received: 16 November 2022; Accepted: 16 December 2022;
Published: 09 January 2023.
Edited by:
Fabiola De Marchi, Azienda Ospedaliero Universitaria Maggiore della Carità, ItalyReviewed by:
Kazumoto Shibuya, Chiba University, JapanJung-Joon Sung, Seoul National University Hospital, Republic of Korea
Steve Vucic, The University of Sydney, Australia
Copyright © 2023 Zoccolella, Giugno and Logroscino. This is an open-access article distributed under the terms of the Creative Commons Attribution License (CC BY). The use, distribution or reproduction in other forums is permitted, provided the original author(s) and the copyright owner(s) are credited and that the original publication in this journal is cited, in accordance with accepted academic practice. No use, distribution or reproduction is permitted which does not comply with these terms.
*Correspondence: Stefano Zoccolella, stefzoc@hotmail.it