- 1Department of Neurosurgery, The Third Affiliated Hospital, Sun Yat-sen University, Guangzhou, Guangdong, China
- 2Department of Rehabilitation Medicine, The Third Affiliated Hospital, Sun Yat-sen University, Guangzhou, Guangdong, China
Long-chain acyl-coenzyme A synthetases (ACSLs) are a family of CoA synthetases that activate fatty acid (FA) with chain lengths of 12–20 carbon atoms by forming the acyl-AMP derivative in an isozyme-specific manner. This family mainly includes five members (ACSL1, ACSL3, ACSL4, ACSL5, and ACSL6), which are thought to have specific and different functions in FA metabolism and oxidative stress of mammals. Accumulating evidence shows that the dysfunction of ACSLs is likely to affect cell proliferation and lead to metabolic diseases in multiple organs and systems through different signaling pathways and molecular mechanisms. Hence, a central theme of this review is to emphasize the therapeutic implications of ACSLs in nervous system disorders.
Introduction
Long-chain acyl-coenzyme A synthetases (ACSLs) are composed of a CoA synthetases family that activates fatty acid (FA) with chain lengths of 12–20 carbon atoms by forming the acyl-AMP derivative in an isozyme-specific manner. ACSLs identified in mammals mainly include five members—ACSL1, ACSL3, ACSL4, ACSL5, and ACSL6 (ACSL2 has been deleted because its cDNAs correspond to the same gene as ACSL1)—which encode the corresponding proteins and are essential for FA catabolism, de novo lipid synthesis, and remodeling of membranes (Soupene and Kuypers, 2008; Tang et al., 2018; Kuwata and Hara, 2019; Quan et al., 2021).
It is well-known that FA metabolism is the major source of energy in mammals since it can release large quantities of adenosine triphosphate (Yan et al., 2015). The key step of long chain FA metabolism depends on its activation, which requires specific ACSLs (Figure 1). ACSLs have individual functions in FA metabolism among different types of cells, thus their dysregulation will contribute to a variety of metabolic diseases, such as fatty liver disease, obesity, atherosclerosis, diabetes, tumor, etc. (Yan et al., 2015). Several ACSLs-related reviews have summarized the mechanisms and function of the ACSLs in cancer: ACSL1 and ACSL3 may lead to cancer progression and worse prognosis, while ASCL5 and ACSL6 act as key suppressor with the opposite effect (Yan et al., 2015; Kuwata and Hara, 2019; Quan et al., 2021).
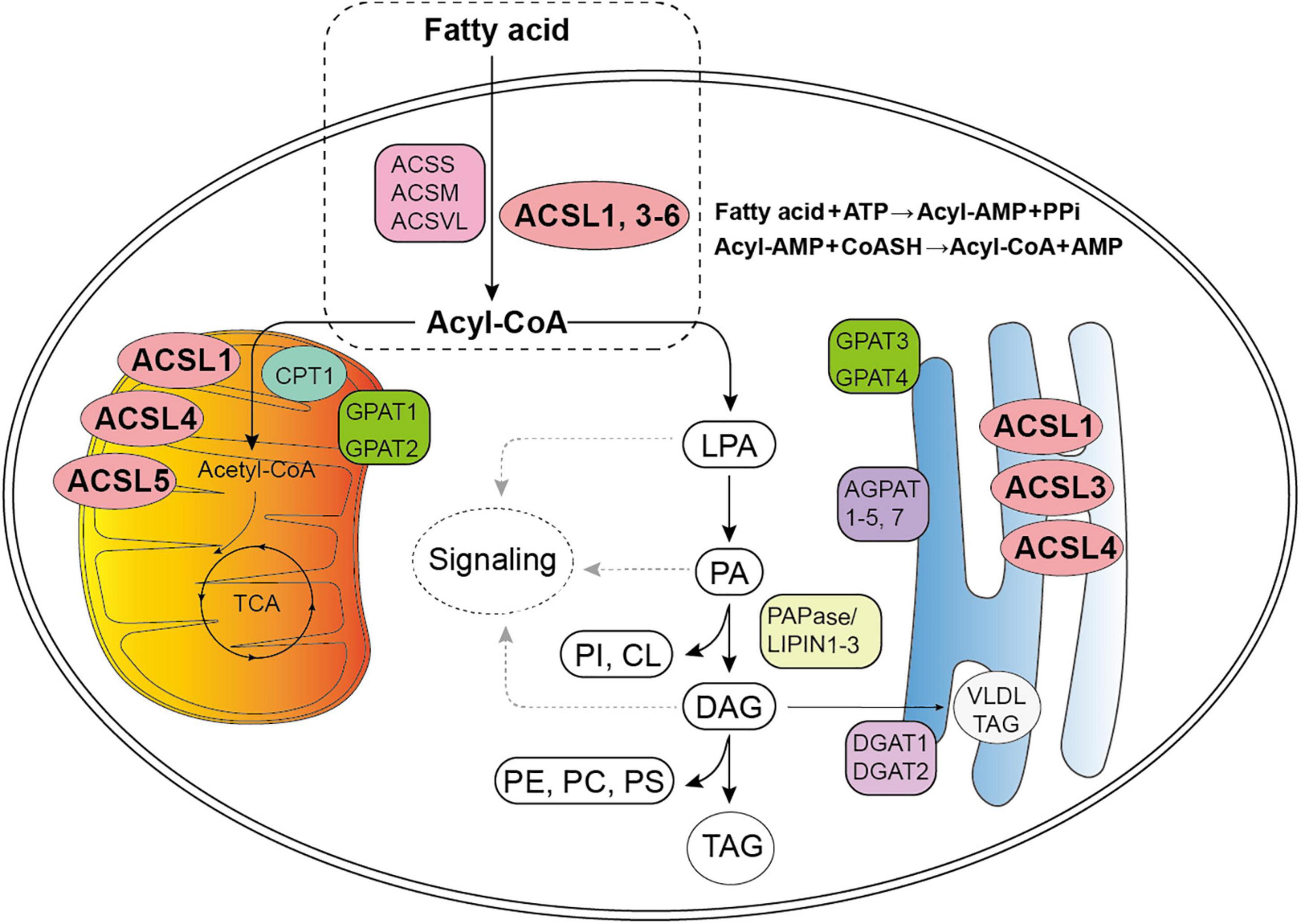
Figure 1. Diagram for fatty acid (FA) metabolism. The first stage of FA metabolism is the activation of FA, which requires a two-step reaction catalyzed by Acyl-CoA synthetases: an acyl-AMP intermediate is first formed from ATP, and then exchanged with CoA to yield the activated acyl-CoA. (Gassler et al., 2007; Soupene and Kuypers, 2008; Golej et al., 2011; Nakahara et al., 2012; Rossi Sebastiano and Konstantinidou, 2019). Subsequently, Acyl-CoA participates in the synthesis of lysophosphatidic acid (LPA), phosphatidic acid (PA), glycerol diester (DAG), and triacylglycerol (TAG) (Coleman, 2019). Acyl-CoAs can also be converted to acyl-carnitines by carnitine palmitoyltransferase (CPT1) to enter the mitochondria for β-oxidation and tricarboxylic acid cycle (TCA). The LPA, PA, and DAG intermediates may initiate signaling cascades, and PA and DAG are also precursors of all the glycerophospholipids: phosphatidylinositol (PI), phosphatidylcholine (PC), phosphatidylethanolamine (PE), phosphatidylserine (PS), and cardiolipin (CL) (Coleman, 2019). The TAG may remain in the cytosol within a lipid droplet or, in liver, be secreted a part of a very-low-density lipoprotein particle (VLDL) (Coleman, 2019). ACSS, short–chain acyl–CoA synthetase; ACSM, medium–chain acyl–CoA synthetase; ACSL, long–chain acyl–CoA synthetase; ACSVL, very long–chain acyl–CoA synthetase; AMP, adenosine monophosphate; PPi, pyrophosphoric acid; CoASH, coenzyme A; GPAT, glycerol-3-P acyltransferases; AGPAT, 1-acylglycerol-3-phosphate acyltransferases (also known as LPA acyltransferase); PAPase/Lipin, PA hosphohydrolases; DGAT, diacylglycerol acyltransferases.
For the past decade, ACSLs have drawn the researchers’ attention to the areas of brain tumor (Yee et al., 2020), stroke (Chang et al., 2019; Chen J. et al., 2021; Cui et al., 2021), injury (Qu et al., 2021; Pang et al., 2022; Yuan et al., 2022), neurodegenerative disease (Yao et al., 2021; Ben-Zaken et al., 2022) etc. (Table 1). However, there has been no special review on the functions of ACSLs in nervous system diseases (NSDs) up to now. Here, we aim to highlight the important roles of ACSLs in NSDs.
ACSL1
ACSL1 has a marked preference for oleate and linoleate (Kanter et al., 2012), and can promote ungoverned cell growth, facilitate tumor invasion and evade programmed cell death (Zhou et al., 2019; Xu et al., 2022). Several studies revealed expression of ACSL1 is related to the progression and prognosis of glioma and amyotrophic lateral sclerosis (ALS).
Glioma
A previous analysis based on The Cancer Genome Atlas (TCGA) RNA sequencing data suggested lower expression of ACSL1 influences metabolic reprogramming and contributes to the better survival of patients with isocitrate dehydrogenase 1 (IDH1) mutant glioma (Zhou et al., 2019). In a recent study on glioma, lower expression of ACSL1 was also found to reverse the accelerated cellular metabolism and tumor growth induced by PRADX (a novel lncRNA ENST00000449248.1 identified by Xu et al., 2022) overexpression in vivo and in vitro. A total of six genes including ACSL1 (the other five were TGFBR2, RUNX1, PPARG, GIT2, and RAP1B) have been characterized in glioma, which can interact with each other in both a competitive endogenous RNA-related manner and were predicted as markers of the mesenchymal subtype in terms of their protein functions (Wang et al., 2018). These findings provide some potential therapeutic targets for the treatment of human glioma.
Amyotrophic lateral sclerosis
ALS is a devastating progressive motor neuron disease that affects people of all ethnicities. A recent study on ACSL1 found that A/G rs6552828 polymorphism may be associated with ALS, in which A-allele may be a risk factor for the development of ALS (Ben-Zaken et al., 2022). The authors analyzed at least 350 samples from 178 ALS patients and 172 athletes (including soccer players, middle- and long-distance runners) and found that the ACSL1 AA genotype was more prevalent among ALS patients and soccer players compared to controls, while ACSL1 GG carriers had a higher mortality rate (Ben-Zaken et al., 2022). This result suggests that ALS patients and soccer players may carry a common genetic predisposition, which is related to impaired FA utilization. However, given that little research on the connection between ACSL1 and ALS has been published so far, more studies are needed to determine the regulatory mechanisms and therapeutic implications of ACSL1 on ALS.
ACSL3
The preferred substrates of ACSL3 are myristate, palmitate, arachidonate, and eicosapentaenoate (Grevengoed et al., 2014). As one of two predominant ACSL isoforms in the brain (another is ACSL6) (Van Horn et al., 2005), the expression of ACSL3 reaches a maximum level 15 days after birth, then declines gradually to 10% of the maximum in the adult brain (Fujino et al., 1996). Thus, ACSL3 may be closely related to the development of the brain.
Glioma
Fujino et al. (1997) found ACSL3 existed in rat glioma cell line KEG1 cells two decades ago. Recently, Qiu et al. (2020) observed significant downregulation of ACSL3 in U251 cells (human glioma cells) treated with 1.42 μM CN-3 (a new asterosaponin isolated from the starfish Culcita novaeguineae, which is characterized as exhibiting antitumor activities at low concentrations). It was reported that ACSL3 has relatively complex functions in different types of cancer. For example, its expression may vary in the different stages and types of cancer: ACSL3 was increased in early carcinogenesis to promote lipid anabolism and deposition, but deceased in advanced breast and prostate cancer to increase lipid utilization. Especially in breast cancer, ACSL3 was found to be upregulated in women with ER-negative breast cancer (Wang et al., 2013), while downregulated in triple-negative breast cancer (Wright et al., 2017). This difference and change in expression may be closely related to cancer cell survival and invasiveness (Tang et al., 2018). Although the similar finding has not been found in glioma till now, the ACSL3 gene has been shown to be downregulated in U251 cells (as mentioned above) (Qiu et al., 2020). Future studies may discover more meaningful details about the changes in function of ACSL3 for glioma.
Stroke
A newly published article revealed that ACSL3 may play an important role in ferroptosis after cerebral I/R injury through GPX4/ACSL4/ACSL3 axis (Li et al., 2022). In transient middle cerebral artery occlusion (tMCAO) mice, the authors found that baicalein (an antioxidant from Scuetellaria baicalensis Georgi) could significantly increase the expression level of ACSL3, which suggested that ACSL3 is the negative regulator on ferroptosis (Li et al., 2022). The possible explanation may be that ACSL3 can convert monounsaturated fatty acids (MUFA) into acyl coenzyme A that binds to membrane phospholipids to protect cells from ferroptosis (Magtanong et al., 2019).
ACSL4
ACSL4 has a marked preference for arachidonate and plays an important role in neuronal differentiation in the brain (Cho, 2012; Klett et al., 2017). Knockout of ACSL4 in embryonic stem cells markedly attenuated neuronal differentiation induced by all-trans retinoic acids and nerve growth factors (Cho, 2012). In the past decade, this enzyme has also been demonstrated essential for the induction of ferroptosis (a newly found form of programmed cell death) by facilitating arachidonic acid (AA) oxidation, which makes it a desirable target of some NSDs-related ferroptosis (Figure 2).
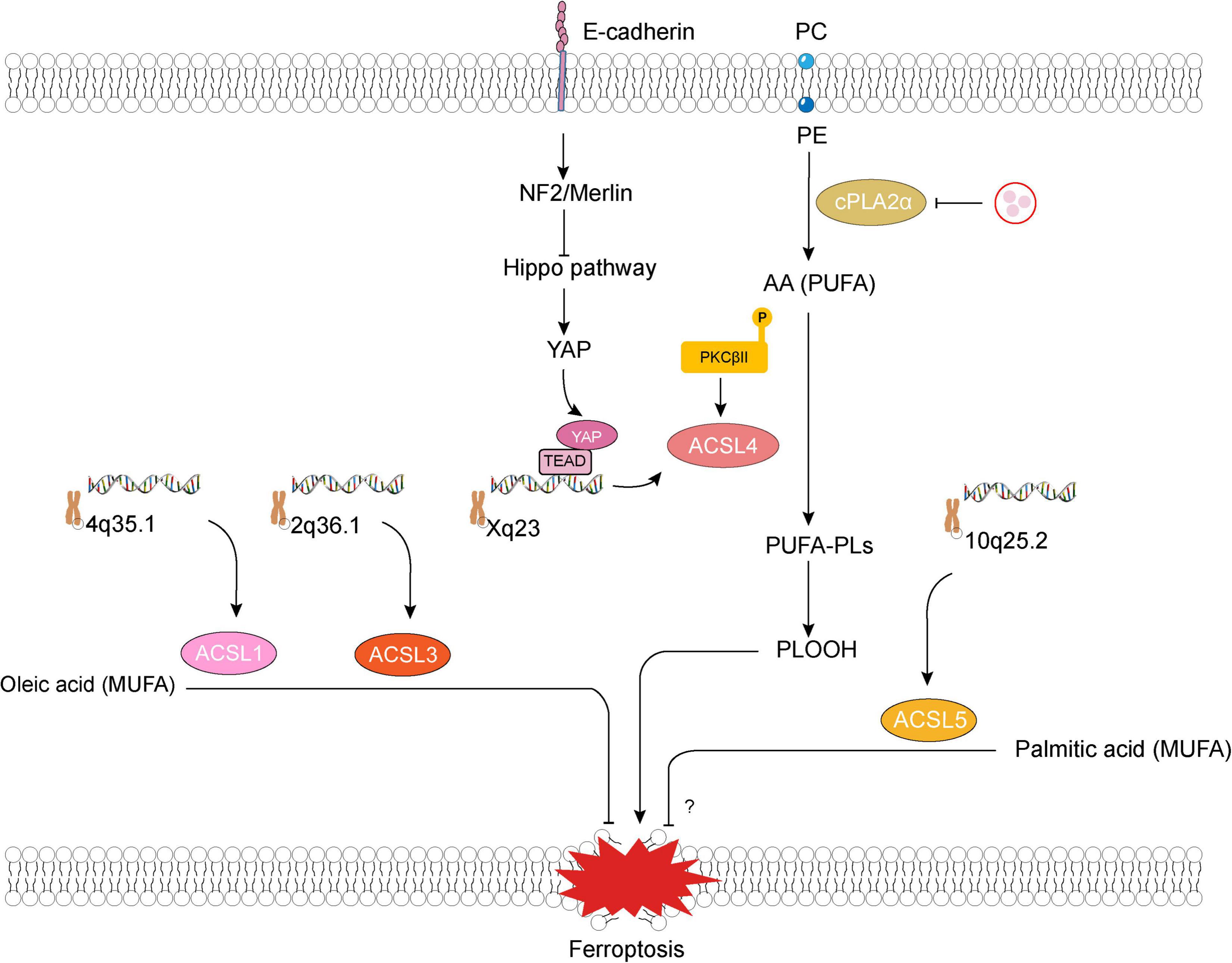
Figure 2. Long-chain acyl-coenzyme A synthetases family member 1, 3–5 (ACSL1, 3–5) and ferroptosis. E-cadherin–NF2–Hippo–YAP pathway suppresses ferroptosis by attenuating YAP-mediated transcription and translation of ACSL4. Lipogenesis involving production of phospholipids containing polyunsaturated fatty acid chains (PUFA-PLs) that are mediated by ACSL4 and multiple other enzymes is required for phospholipid peroxidation and ferroptosis (Jiang et al., 2021). Some pathological states (such as cerebral ischemia) would lead to an unexpected increase in thrombin within neurons, promote the mobilization of phosphatidylcholine (PC) and phosphatidylethanolamine (PE) in the phospholipid membrane of neuronal cells through cytosolic phospholipase A2alpha (cPLA2α), and accelerate the production of polyunsaturated fatty acid, such as arachidonic acid (AA) (Tuo et al., 2022). Besides, ferroptosis inducers promote a slight accumulation of lipid peroxide, which induces the activation of protein kinase C βII (PKCβII). Subsequently, activated PKCβII interacts directly with and phosphorylates ACSL4 at Thr328, which activates ACSL4, triggering the biosynthesis of PUFA-containing lipids and then promoting the generation of lipid-peroxidation products (Zhang H. L. et al., 2022). NF2, neurofibromin 2; YAP, Yes-associated protein; PLOOH, phospholipid hydroperoxide.
Glioma
Numerous glioma-associated studies have focused on the determination of ferroptosis-related protein expression, such as ACSL4, glutathione peroxidase (GPX4), system Xc–, and ferroptosis suppressor protein 1/AIFM2 (FSP1), etc. Mechanistically, ACSL4 is required for ferroptosis in glioma via the regulation of proliferation, migration of glioblastoma, and self-renewal of glia cells (Cheng et al., 2020; Bao C. et al., 2021; Dattilo et al., 2021). The decrease in the expression level of ACSL4 has been observed in isocitrate dehydrogenase (IDH) 1 wild-type and mutant gliomas (Zhou et al., 2019). The chemical inhibition, stable silencing, or depletion of ACSL4 demonstrated it can diminish necrosis and aggressiveness of glioblastoma (Yee et al., 2020). However, in the recurrent glioma, the expression of ACSL4 was found significantly increased, indicating glioblastoma relapses may be higher vulnerable to ferroptosis (Kram et al., 2022).
Subsequently, some targeting molecular mechanisms had been put up based on ACSL4, which shed light on the treatment of glioma. For example, miR-670-3p was found to suppress ferroptosis of human glioblastoma by inhibiting ACSL4, suggesting that inhibition of miR-670-3p can be an adjuvant strategy to treat glioblastoma (Bao C. et al., 2021). Dihydrotanshinone I (DHI) can inhibit the proliferation of human glioma cells via the induction of ferroptosis (Tan et al., 2020), while capsaicin can induce ferroptosis through the increase in expression of ACSL4 (Hacioglu and Kar, 2022). Besides, in erastin-induced ferroptosis in gliomas, heat shock protein 90 (Hsp90) and dynamin-related protein 1 (Drp1) were reported to actively stabilize and regulate ACSL4 expression. Hsp90 overexpression or calcineurin (CN)-mediated Drp1 dephosphorylation at serine 637 (Ser637) can promote ferroptosis via alteration of mitochondrial morphology and increase of ACSL4-mediated lipid peroxidation (Miao et al., 2022). These mechanisms might be used as potential anticancer agents or targets with ferroptosis-induced anti-proliferative effects.
Ischemic stroke
The increase of ACSL4 expression is frequently observed in the stroke models simulating ischemia/reperfusion neuronal injury (Li C. et al., 2021; Liao et al., 2021). Ischemia-induced ACSL4 activation can exacerbate ischemic stroke and contribute to ferroptosis-mediated tissue injury in ischemia/reperfusion (Cui et al., 2021). The up-regulation of ACSL4 may facilitate or hinder neurological recovery after stroke through the regulation of ferroptosis (Chen J. et al., 2021). Therefore, interventions of ACSL4 expression have been predicted to be the potential therapeutic target (Gubern et al., 2013). For example, a recent ferroptosis study pointed out that thrombin can induce ACSL4-dependent ferroptosis during cerebral ischemia/reperfusion, which suggested thrombin-ACSL4 axis may be an important therapeutic target to ameliorate ferroptotic neuronal injury during ischemic stroke (Tuo et al., 2022). Moreover, transcription factor special protein 1 (Sp1) has been identified as an important factor in promoting ACSL4 expression and exacerbating ferroptosis (Li et al., 2019). Pomelo peel essential oil (PPEO) also has a neuroprotective effect after cerebral ischemia/reperfusion injury by inhibiting the expression of ACSL4 and ferroptosis (Hu et al., 2022). Besides, carthamin yellow (CY) treatment can indirectly reverse ACSL4 expression level in the brain by the inhibition of Fe2+ and reactive oxygen species accumulation (Guo H. et al., 2021).
Intracerebral hemorrhage
Intracerebral hemorrhage (ICH) is one of the most refractory and lethal NSDs. Two ACSL4-associated mechanisms on ICH have been proposed, including lncRNA H19/miR-106b-5p/ACSL4 axis and HOTAIR/UPF1/ACSL4 axis (Chen B. et al., 2021; Jin et al., 2021), which may play a crucial role in ICH. In the first mechanism, miR-106b-5p is a target of H19, while ACSL4 is a target gene of miR-106b-5p. This kind of regulation effect among H19, miR-106b-5p, and ACSL4 implicates ACSL4 may be downstream of this axis. With the use of the ICH model (glucose deprivation hemin-treated, OGD/H-treated), Chen B. et al. (2021) verified the knockdown of H19 can promote cell proliferation and suppress ferroptosis in the brain microvascular endothelial cells by regulating the lncRNA H19/miR-106b-5p/ACSL4 axis. Besides, HOTAIR/UPF1/ACSL4 axis has been also reported to play an important role in the ferroptosis of neuronal cells in the progression of ICH, which can be mediated by Paeonol (2′-hydroxy-4′-methoxyacetophenone), the main active compound of the radix of Paeonia suffruticosa (Jin et al., 2021). Paeonol notably inhibited ferroptosis in hemin-treated neuronal cells via inhibition of ACSL4. In short, the intervention of the axis may be a promising therapeutic strategy for ICH.
Subarachnoid hemorrhage
ACSL4 can exacerbate subarachnoid hemorrhage (SAH)-induced early brain injury (EBI) by mediating ferroptosis (Qu et al., 2021; Yuan et al., 2022). After SAH, the expression of ACSL4 in brain tissue increased significantly. Inhibiting the expression of ACSL4 using small interfering RNAs has been proven to alleviate inflammation, oxidative stress, brain edema, blood-brain barrier impairment, etc., and increase the number of surviving neurons (Qu et al., 2021). Furthermore, activation of SIRT1 (Sirtuin 1, a class III histone deacetylase) was found to suppress SAH-induced ferroptosis by deregulating the expression of ACSL4 (Yuan et al., 2022). Another study also demonstrated puerarin [8-(β-d-glucopyranosyl-daidzein)] can remarkably inhibit the expression of ACSL4 and ferroptosis, which is associated with EBI after SAH (Huang et al., 2022). The above evidence suggested ACSL4 could be a potential therapeutic target for SAH.
Traumatic brain injury
Increased expression of ACSL4 and other biomarkers of ferroptosis have been found in traumatic brain injury (TBI), which indicated ferroptosis is implicated in this pathological state and may contribute to neuronal death and worse functional outcome (Kenny et al., 2019; Xiao et al., 2019; Bao Z. et al., 2021). In a mice-based experimental study, the authors proved that cortical impact injury can result in accumulation of oxidized phosphatidylethanolamine, increased expression of 15-lipoxygenase and ACSL4, and depletion of glutathione in the ipsilateral cortex. These results can be reversed with the use of baicalein (12/15-lipoxygenase inhibitor) (Kenny et al., 2019). Another two mice-based studies also identified the increased expression of ACSL4 and the occurrence of ferroptosis in TBI, in which ferroptotic neuronal death can be attenuated by miR-212-5p and prokineticin-2 (Prok2) (Xiao et al., 2019; Bao Z. et al., 2021). Prokineticin-2 (Prok2) is an important secreted protein likely involved in the pathogenesis of TBI, which can suppress the biosynthesis of lipid peroxidation substrates, AA-phospholipids, via accelerated degradation of ACSL4 (Bao Z. et al., 2021).
Spinal cord injury
Edaravone, a lipid peroxidation scavenger, has been approved by Food and Drug Administration as a neuroprotective effect on spinal cord injury (SCI) and many other central nervous system diseases. It can downregulate pro-ferroptosis proteins ACSL4 and 5-lipoxygenase (5-LOX), and reduce microgliosis and astrogliosis to promote recovery after SCI (Pang et al., 2022). Proanthocyanidins (PACs) treatment has also been shown to mediate ferroptosis by significantly decreasing the levels of ACSL4 and iron in traumatic spinal cords and improving the locomotive function of SCI mice (Zhou et al., 2020). Lipoxin A4 (LXA4) can exert a protective role in SCI by inhibiting the expression of ferroptosis biomarkers including ACSL4 (Wei et al., 2021).
Non-syndromic X-Linked intellectual developmental disorder
Deletions or mutations of ACSL4 are a rare cause of non-syndromic X-linked intellectual disability (Gazou et al., 2013). Zhang et al. (2009) and Jia et al. (2019) demonstrated that Drosophila ACSL-like protein, dAcsl, is highly homologous to human ACSL3 and ACSL4; and the mutation of dAcsl can lead to the dramatical decrease of number of glial cells and neurons, which may further result in developmental defects. Liu et al. (2011) demonstrated that dAcsl can regulate axonal transport of synaptic vesicles and is required for synaptic development and function. Three years later, they further revealed that dAcsl can inhibit synapse growth by attenuating bone morphogenetic protein signaling via endocytic recycling (Liu et al., 2014). Another relevant study revealed that ACSL4 can inhibit neuromuscular junction growth by stimulating C16:1 fatty acyl production and concomitantly suppressing raft-associated lipid levels (Huang et al., 2016). Besides, Meloni et al. (2009) found the reduced levels of ACSL4 in the brain would induce a significant reduction in dendritic spine density and an alteration in spine/filopodia distribution. Chang et al. (2019) reported a very rare childhood stroke with ACSL4 hemizygous intragenic deletion. These findings provide insights into the pathogenesis of ACSL4-related intellectual disability.
Alport syndrome with intellectual disability
Alport syndrome with intellectual disability (ATS-ID) is an X-linked contiguous gene deletion syndrome associated with an Xq22.3 locus, which is mainly characterized by neurodevelopmental disorder (NDD), hearing loss/deafness, hematuria, renal failure, midface retrusion, and elliptocytosis. It is thought that ATS-ID is caused by the loss of function of ACSL4 genes through the interstitial (micro) deletion of chromosomal band Xq22.3 (Smetana et al., 2021). Another research reported a family with two males with this disorder, which is characterized by ID with absent or severely delayed speech, midface hypoplasia, and facial hypotonia (Rodriguez et al., 2010).
Alzheimer’s disease
Alzheimer’s disease (AD) is a progressive, age-related neurodegenerative disease. The expression of ACSL4 in the hippocampus in patients with AD has been shown to be related to dietary AA (Thomas et al., 2017). AA consumption is elevated in patients with AD, indicating an excess of AA in the human diet could constitute a risk factor for AD (Rapoport, 2008). In APP/PS1 mice (APPswe/PSEN1dE9 transgenic mice model of AD), transcriptome analysis identified the enriched ACSL4 (Yan et al., 2022), which can be inhibited by tetrahydroxy stilbene glycoside (TSG) (Gao et al., 2021). Besides, N2L, a novel lipoic acid-niacin dimer regulating lipid metabolism with multifunction, was also proved to exert neuroprotective effects by blocking the increase of ACSL4 protein expression (Peng et al., 2021). Interestingly, ACSL4 was also found to participate in AD-related cardiac contractile dysfunction, which can be rescued by mitochondrial aldehyde dehydrogenase (ALDH2) (Zhu et al., 2022).
Parkinson’s disease
Parkinson’s disease (PD) is another age-related degenerative brain disorder characterized by the loss of dopaminergic neurons in the substantia nigra and striatum. Recently, in 1-methyl-4-phenyl-1,2,3,6-tetrahydropyridine (MPTP)-induced PD mouse models, up-regulation of ACSL4 has been found and could be inhibited by apoferritin, a typical iron storage protein with a diameter of 12.5 nm. Apoferritin can improve motor deficits by preventing ferroptosis (Song et al., 2021). In another experiment stimulating cellular senescence, 1-methyl-4-phenylpyridinium (MPP) induced upregulation of ACSL4 expression and enhanced levels of oxidative stress, which were important characteristics of ferroptosis (Li S. et al., 2021).
Cognitive impairment
Diabetes has been shown to result in neurodegenerative diseases and cognitive decline, which can be alleviated by liraglutide (An et al., 2022). This drug mainly exerts its therapeutic effect by preventing the excessive amount of ACSL4 and inhibiting ferroptosis in the hippocampus (An et al., 2022). ACSL4 was also identified to be implicated in sevoflurane-induced postoperative cognitive dysfunction (POCD). In SH-SY5Y cells, increased ACSL4 expression can lead to ferroptotic neuronal death via the 5′ AMP-activated protein kinase/mammalian target of the rapamycin (AMPK/mTOR) pathway, while its downregulation has the opposite effect, providing a potential therapeutic approach to alleviate sev-induced POCD (Cheng et al., 2021).
Other central nervous system diseases
As ACSL4 dictates ferroptosis sensitivity via shaping cellular lipid composition, its inactivation has been considered a key mechanism for suppressing ferroptosis in diverse contexts (Doll et al., 2017). Ferroptosis has been shown to be involved in the neuronal damage, aberrant electrical brain activity (Shao et al., 2020, 2022; Chen et al., 2022), and the progressive death of motor neurons (Moujalled et al., 2021; Wang T. et al., 2022). Therefore, targeting ferroptosis-related protein ACSL4 may shed new light on the therapy of some other CNS diseases, including epilepsy (Kahn-Kirby et al., 2019; Mao et al., 2019; Shao et al., 2020, 2022; Yang et al., 2020; Chen et al., 2022), ALS (Moujalled et al., 2021; Wang T. et al., 2022), cerebral malaria (Liang et al., 2022), bipolar disorder (Modi et al., 2014, 2017), and sepsis-associated encephalopathy (Wang J. et al., 2022). For example, mood stabilizer valproic acid and chiral isomer valnoctamide have been shown to take effect in the treatment of bipolar disorder by inhibiting recombinant ACSL4, brain AA turnover in brain phospholipids, and AA activation to AA-CoA (Modi et al., 2014, 2017).
Neuropathic pain induced by peripheral nerve injury
Neuropathic pain (NP) induced by peripheral nerve injury has been shown to be associated with the over-expression of ACSL4 and ferroptosis (Guo Y. et al., 2021; Wang et al., 2021; Zhang X. et al., 2022). Here we introduce two identical NP models, including spared nerve injury (SNI) and chronic constriction injury (CCI). In the first rat models of NP, the expression of ACSL4 was found significantly increased in the spinal cord after SNI, which can be reversed by intrathecal injection of sirtuin 2 (SIRT2) overexpressed recombinant adenovirus, indicating that SIRT2 may achieve a neuroprotective effect via the suppression of ferroptosis (Zhang X. et al., 2022). In another CCI model of the sciatic nerve, Guo H. et al. (2021) also observed a similar phenomenon: the level of ACSL4 significantly increased in the corresponding spinal cord segment after injury. They further revealed that spinal ferroptosis-like cell death was involved in the development of NP resulting from injury, and inhibition of ferroptosis could alleviate mechanical and thermal hypersensitivities (Guo Y. et al., 2021). However, they failed to further reveal the inner molecule mechanism that affected ferroptosis, and Schwann cells (SCs), the basic cells of the myelin sheath of the axis cylinder, were not mentioned.
Several SCs-related studies have tried to clarify the mechanism of ferroptosis in peripheral nerve injury (Chang et al., 2021; Gao et al., 2022). Chang et al. (2021) pointed out complex IV subunit 4 isoform 2 (Cox4i2) can trigger an increase in reactive oxygen species, leading to ferroptosis and apoptosis in human herpesvirus 7 (HHV7) infected SCs. Gao et al. (2022) found the overexpression of c-Jun, a key regulator of the response of SCs to peripheral nerve injury, inhibits erastin-induced ferroptosis in SCs and promotes repair of facial nerve function.
Previous analysis of ferroptosis-resistant cell lines and a CRISPR suppression screen independently yielded ACSL4 inactivation as a key mechanism for suppressing ferroptosis in diverse contexts (Doll et al., 2017). ACSL4 may be more akin to caspase-3, the executioner of apoptosis, than to a housekeeping protein (Stockwell, 2022). These results implied there may exist a close relationship between ACSL4 and ferroptosis in the SCs, although changes in the expression of ACSL4 were not detected directly in the above two studies.
ACSL5
ACSL5 is encoded by ACSL5 gene, of which the preferred substrates are palmitate, palmitoleate, oleate, and linoleate (Klett et al., 2017). The current studies mainly reported its correlation with glioma and ALS.
Glioma
Mashima et al. (2009b) reported that ACSL5 is overexpressed in malignant glioma, and can selectively enhance human glioma cell survival through its ACS catalytic activity under extracellular acidosis. cDNA microarray analysis further suggested that ACSL5 was critical to the expression of tumor-related factors including midkine (MDK), and the knockdown of MDK expression significantly attenuated ACSL5-mediated survival under an acidic state (Mashima et al., 2009b). Mashima et al. (2009a) also found that ACSL5 inhibition can synergistically potentiate the glioma cell death induced by etoposide, a well-known activator of apoptosis. In another earlier study, Yamashita et al. (2000) found FA-induced glioma cell growth is mediated by the ACSL5 gene located on chromosome 10q25.1-q25.2, where deletion frequently happened in malignant gliomas. This evidence demonstrated the important role of ACSL5 on glioma cell growth.
Amyotrophic lateral sclerosis
In a study on genome-wide data analysis, ACSL5 was identified as one of six differentially expressed genes through two-sample Mendelian randomization (Saez-Atienzar et al., 2021). Another genome-wide meta-analysis on data from European and Chinese populations (84,694 individuals) also found the ACSL5-ZDHHC6 locus is associated with ALS and links weight loss to the disease genetics—body weight loss is a frequent complication in ALS patients and is reported to be associated with shorter survival (Iacoangeli et al., 2020). This connection between ACSL5 and ALS was also confirmed by Nakamura et al. (2020) in a genome-wide association study using 1,173 sporadic ALS cases and 8,925 controls in a Japanese population.
ACSL6
ACSL6 is reported to have an equal preference for saturated and polyunsaturated FA with a backbone of C16–C20 (Lopes-Marques et al., 2013). ACSL6 is enriched in the brain and highly critical for maintaining brain omega-3 FA docosahexaenoic acid (DHA) levels (Chouinard-Watkins and Bazinet, 2018; Fernandez et al., 2018). DHA is also abundant in the brain and helpful in protection against numerous neurological disorders, and this type of protective effect can be enhanced by ACSL6 (Fernandez et al., 2018). However, it is noteworthy that the neuroprotection effect was confirmed to be confined only to neurons, not in astrocytes (Fernandez et al., 2021). Besides, ACSL6-related NSDs mainly include AD and schizophrenia.
Alzheimer’s disease
As one of the DHA transporters (another known is Fatp4), the alteration in the expression of ACSL6 may be a risk factor for an exacerbation of cognitive and neurological deficits in patients with AD (Pontifex et al., 2021). It was reported that overexpression of ACSL6 in nerve cells can significantly increase DHA and AA internalization within the first 24 h of neuronal differentiation to stimulate and enhance phospholipids synthesis and neurite outgrowth (Marszalek et al., 2005). Silencing ACSL6 inhibits axon outgrowth of mouse neural cells. ACSL6-induced activation of acetylcholinesterase may be involved in this process, as acetylcholinesterase promotes neural differentiation. The insufficiency of ACSL6 can lead to neuronal degeneration, while its over-expression is closely associated with neurite outgrowth (Kim et al., 2009). Lipid profiling of ACSL6–/– (loss of ACSL6) tissues reveals consistent reductions in DHA-containing lipids in tissues highly abundant with ACSL6 (Fernandez et al., 2018), and ACSL6–/– depletes brain membrane phospholipid DHA levels, which is related to motor function, memory, and age-related neuroinflammation (Fernandez et al., 2021).
Schizophrenia
ACSL6 gene is also reported to be associated with schizophrenia (Chen et al., 2006, 2011). Its variation may contribute to the number of cigarettes smoked in patients (Chen et al., 2011). For example, nicotine exposure can stimulate the expression of ACSL6 in the prefrontal cortex and hippocampus of mice (in vivo), which can be suppressed by injection of the nicotinic receptor antagonist mecamylamine (Chen et al., 2011). However, in another candidate gene association analysis, the authors claimed their results did not yield convincing evidence for associations of schizophrenia with ACSL6 (Chowdari et al., 2007).
Conclusion
ACSLs are involved in some biological responses by activating long-chain FAs in the nervous system, such as tumor development, progression and cell death. Several diseases are related to more than one subtype. For example, the progression of glioma is regulated by four members of ACSLs, including ACSL1, 3, 4, and 5; ALS is associated with the dysregulation of at least three subtypes of ACSLs, including ACSL1, 4, and 5.
However, most of the previous studies mainly focused on the central nervous system and presented encouraging results, while little evidence on the relationship between ACSLs and peripheral nervous diseases has been provided. Although some recent studies have proposed the key roles of ACSL4 in the mechanism of neuropathic pain induced by peripheral nerve injury, few studies are involved in the regulation effect of ACSLs in the SCs.
Furthermore, ACSL4 is universal in numerous NSDs because it correlates with ferroptosis and has been predicted to be the potential therapeutic target in some NSDs. It is noteworthy that ferroptosis was not mentioned in studies that suggested ACSL4 gene dysfunction leads to intellectual disability. However, this doesn’t mean ferroptosis is not relevant to these diseases due to its close relationship with ACSL4. Further studies are needed to verify the inner correlation between ferroptosis and these neurological disorders.
What’s more, ACSL1, 3, and 5 are also reported to be ferroptosis-relevant isoforms. For example, ACSL1 can mediate α-eleostearic acid (ESA) -triggered ferroptosis as well as αESA incorporation into specific lipid species including DAGs and TAGs. ACSL3 can activate MUFAs (e.g., oleic acid) and promote a ferroptosis-resistant cell state. ACSL5 has also been identified as the ferroptosis-related gene in cancer. Although they play important roles in non-NSDs, their functions in the nervous system have not been well proven.
In conclusion, plenty of encouraging findings indicated that targeting ACSLs and ferroptosis may be a novel potential therapeutic strategy, especially in the situation of NSDs.
Author contributions
All authors listed have made a substantial, direct, and intellectual contribution to the work, and approved it for publication.
Funding
This work was supported by the Guangdong Basic and Applied Basic Research Foundation (Grant No. 2022A1515012433), the Sun Yat-sen University Clinical Research 5010 Program (Grant No. 2021001), and the Third Affiliated Hospital of Sun Yat-sen University, Clinical Research Program (Grant No. YHJH201902).
Conflict of interest
The authors declare that the research was conducted in the absence of any commercial or financial relationships that could be construed as a potential conflict of interest.
Publisher’s note
All claims expressed in this article are solely those of the authors and do not necessarily represent those of their affiliated organizations, or those of the publisher, the editors and the reviewers. Any product that may be evaluated in this article, or claim that may be made by its manufacturer, is not guaranteed or endorsed by the publisher.
References
An, J. R., Su, J. N., Sun, G. Y., Wang, Q. F., Fan, Y. D., Jiang, N., et al. (2022). Liraglutide alleviates cognitive deficit in db/db Mice: Involvement in oxidative stress, iron overload, and ferroptosis. Neurochem. Res. 47, 279–294.
Bao, C., Zhang, J., Xian, S. Y., and Chen, F. (2021). MicroRNA-670-3p suppresses ferroptosis of human glioblastoma cells through targeting ACSL4. Free Radic. Res. 55, 853–864. doi: 10.1080/10715762.2021.1962009
Bao, Z., Liu, Y., Chen, B., Miao, Z., Tu, Y., Li, C., et al. (2021). Prokineticin-2 prevents neuronal cell deaths in a model of traumatic brain injury. Nat. Commun. 12:4220. doi: 10.1038/s41467-021-24469-y
Ben-Zaken, S., Nefussy, B., Meckel, Y., Eliakim, A., Nemet, D., Gotkine, M., et al. (2022). Common genetic basis of ALS patients and soccer players may contribute to disease risk. Neurol. Sci. 43, 4231–4238. doi: 10.1007/s10072-022-05990-4
Chang, B., Guan, H., Wang, X., Chen, Z., Zhu, W., Wei, X., et al. (2021). Cox4i2 Triggers an increase in reactive oxygen species, leading to ferroptosis and apoptosis in HHV7 infected schwann cells. Front. Mol. Biosci. 8:660072. doi: 10.3389/fmolb.2021.660072
Chang, C. A., Lauzon, J., Kirton, A., and Argiropoulos, B. (2019). An ACSL4 hemizygous intragenic deletion in a patient with childhood stroke. Pediatr. Neurol. 100, 100–101. doi: 10.1016/j.pediatrneurol.2019.06.014
Chen, B., Wang, H., Lv, C., Mao, C., and Cui, Y. (2021). Long non-coding RNA H19 protects against intracerebral hemorrhage injuries via regulating microRNA-106b-5p/acyl-CoA synthetase long chain family member 4 axis. Bioengineered 12, 4004–4015. doi: 10.1080/21655979.2021.1951070
Chen, J., Brunzell, D. H., Jackson, K., van der Vaart, A., Ma, J. Z., Payne, T. J., et al. (2011). ACSL6 is associated with the number of cigarettes smoked and its expression is altered by chronic nicotine exposure. PLoS One 6:e28790. doi: 10.1371/journal.pone.0028790
Chen, J., Yang, L., Geng, L., He, J., Chen, L., Sun, Q., et al. (2021). Inhibition of Acyl-CoA synthetase long-chain family member 4 facilitates neurological recovery after stroke by regulation ferroptosis. Front. Cell. Neurosci. 15:632354. doi: 10.3389/fncel.2021.632354
Chen, K. N., Guan, Q. W., Yin, X. X., Wang, Z. J., Zhou, H. H., and Mao, X. Y. (2022). Ferrostatin-1 obviates seizures and associated cognitive deficits in ferric chloride-induced posttraumatic epilepsy via suppressing ferroptosis. Free Radic. Biol. Med. 179, 109–118. doi: 10.1016/j.freeradbiomed.2021.12.268
Chen, X., Wang, X., Hossain, S., O’Neill, F. A., Walsh, D., Pless, L., et al. (2006). Haplotypes spanning SPEC2, PDZ-GEF2 and ACSL6 genes are associated with schizophrenia. Hum. Mol. Genet. 15, 3329–3342. doi: 10.1093/hmg/ddl409
Cheng, J., Fan, Y. Q., Liu, B. H., Zhou, H., Wang, J. M., and Chen, Q. X. (2020). ACSL4 suppresses glioma cells proliferation via activating ferroptosis. Oncol. Rep. 43, 147–158. doi: 10.3892/or.2019.7419
Cheng, L., Zhu, X., Liu, Y., Zhu, K., Lin, K., and Li, F. (2021). ACSL4 contributes to sevoflurane-induced ferroptotic neuronal death in SH-SY5Y cells via the 5’ AMP-activated protein kinase/mammalian target of rapamycin pathway. Ann. Transl. Med. 9:1454. doi: 10.21037/atm-21-4249
Cho, Y. Y. (2012). A novel role of brain-type ACS4 isotype in neuronal differentiation. Biochem. Biophys. Res. Commun. 419, 505–510. doi: 10.1016/j.bbrc.2012.02.046
Chouinard-Watkins, R., and Bazinet, R. P. (2018). ACSL6 is critical for maintaining brain DHA levels. Proc. Natl. Acad. Sci. U.S.A. 115, 12343–12345. doi: 10.1073/pnas.1817557115
Chowdari, K. V., Northup, A., Pless, L., Wood, J., Joo, Y. H., Mirnics, K., et al. (2007). DNA pooling: A comprehensive, multi-stage association analysis of ACSL6 and SIRT5 polymorphisms in schizophrenia. Genes Brain Behav. 6, 229–239. doi: 10.1111/j.1601-183X.2006.00251.x
Coleman, R. A. (2019). It takes a village: Channeling fatty acid metabolism and triacylglycerol formation via protein interactomes. J. Lipid Res. 60, 490–497. doi: 10.1194/jlr.S091843
Cui, Y., Zhang, Y., Zhao, X., Shao, L., Liu, G., Sun, C., et al. (2021). ACSL4 exacerbates ischemic stroke by promoting ferroptosis-induced brain injury and neuroinflammation. Brain Behav. Immun. 93, 312–321. doi: 10.1016/j.bbi.2021.01.003
Dattilo, M. A., Benzo, Y., Herrera, L. M., Prada, J. G., Lopez, P. F., Caruso, C. M., et al. (2021). Regulation and role of Acyl-CoA synthetase 4 in glial cells. J. Steroid Biochem. Mol. Biol. 208:105792.
Doll, S., Proneth, B., Tyurina, Y. Y., Panzilius, E., Kobayashi, S., Ingold, I., et al. (2017). ACSL4 dictates ferroptosis sensitivity by shaping cellular lipid composition. Nat. Chem. Biol. 13, 91–98. doi: 10.1038/nchembio.2239
Fernandez, R. F., Kim, S. Q., Zhao, Y., Foguth, R. M., Weera, M. M., Counihan, J. L., et al. (2018). Acyl-CoA synthetase 6 enriches the neuroprotective omega-3 fatty acid DHA in the brain. Proc. Natl. Acad. Sci. U.S.A. 115, 12525–12530.
Fernandez, R. F., Pereyra, A. S., Diaz, V., Wilson, E. S., Litwa, K. A., Martínez-Gardeazabal, J., et al. (2021). Acyl-CoA synthetase 6 is required for brain docosahexaenoic acid retention and neuroprotection during aging. JCI Insight 6:e144351. doi: 10.1172/jci.insight.144351
Fujino, T., Kang, M. J., Suzuki, H., Iijima, H., and Yamamoto, T. (1996). Molecular characterization and expression of rat acyl-CoA synthetase 3. J. Biol. Chem. 271, 16748–16752.
Fujino, T., Man-Jong, K., Minekura, H., Suzuki, H., and Yamamoto, T. T. (1997). Alternative translation initiation generates acyl-CoA synthetase 3 isoforms with heterogeneous amino termini. J. Biochem. 122, 212–216. doi: 10.1093/oxfordjournals.jbchem.a021731
Gao, D., Huang, Y., Sun, X., Yang, J., Chen, J., and He, J. (2022). Overexpression of c-Jun inhibits erastin-induced ferroptosis in Schwann cells and promotes repair of facial nerve function. J. Cell. Mol. Med. 26, 2191–2204. doi: 10.1111/jcmm.17241
Gao, Y., Li, J., Wu, Q., Wang, S., Yang, S., Li, X., et al. (2021). Tetrahydroxy stilbene glycoside ameliorates Alzheimer’s disease in APP/PS1 mice via glutathione peroxidase related ferroptosis. Int. Immunopharmacol. 99:108002. doi: 10.1016/j.intimp.2021.108002
Gassler, N., Roth, W., Funke, B., Schneider, A., Herzog, F., Tischendorf, J. J., et al. (2007). Regulation of enterocyte apoptosis by acyl-CoA synthetase 5 splicing. Gastroenterology 133, 587–598. doi: 10.1053/j.gastro.2007.06.005
Gazou, A., Riess, A., Grasshoff, U., Schäferhoff, K., Bonin, M., Jauch, A., et al. (2013). Xq22.3-q23 deletion including ACSL4 in a patient with intellectual disability. Am. J. Med. Genet. A 161A, 860–864. doi: 10.1002/ajmg.a.35778
Golej, D. L., Askari, B., Kramer, F., Barnhart, S., Vivekanandan-Giri, A., Pennathur, S., et al. (2011). Long-chain acyl-CoA synthetase 4 modulates prostaglandin E2 release from human arterial smooth muscle cells. J. Lipid Res. 52, 782–793. doi: 10.1194/jlr.M013292
Grevengoed, T. J., Klett, E. L., and Coleman, R. A. (2014). Acyl-CoA metabolism and partitioning. Annu. Rev. Nutr. 34, 1–30.
Gubern, C., Camós, S., Ballesteros, I., Rodríguez, R., Romera, V. G., Cañadas, R., et al. (2013). miRNA expression is modulated over time after focal ischaemia: Up-regulation of miR-347 promotes neuronal apoptosis. FEBS J. 280, 6233–6246. doi: 10.1111/febs.12546
Guo, H., Zhu, L., Tang, P., Chen, D., Li, Y., Li, J., et al. (2021). Carthamin yellow improves cerebral ischemia-reperfusion injury by attenuating inflammation and ferroptosis in rats. Int. J. Mol. Med. 47:52. doi: 10.3892/ijmm.2021.4885
Guo, Y., Du, J., Xiao, C., Xiang, P., Deng, Y., Hei, Z., et al. (2021). Inhibition of ferroptosis-like cell death attenuates neuropathic pain reactions induced by peripheral nerve injury in rats. Eur. J. Pain 25, 1227–1240. doi: 10.1002/ejp.1737
Hacioglu, C., and Kar, F. (2022). Capsaicin induces redox imbalance and ferroptosis through ACSL4/GPx4 signaling pathways in U87-MG and U251 glioblastoma cells. Metab. Brain Dis. doi: 10.1007/s11011-022-00983-w [Epub ahead of print].
Hu, W., Chen, M., Wang, W., Huang, F., Tian, X., and Xie, L. (2022). Pomelo peel essential oil ameliorates cerebral ischemia-reperfusion injury through regulating redox homeostasis in rats and SH-SY5Y cells. Oxid. Med. Cell. Longev. 2022:8279851. doi: 10.1155/2022/8279851
Huang, Y., Huang, S., Lam, S. M., Liu, Z., Shui, G., and Zhang, Y. Q. (2016). Acsl, the Drosophila ortholog of intellectual-disability-related ACSL4, inhibits synaptic growth by altered lipids. J. Cell Sci. 129, 4034–4045. doi: 10.1242/jcs.195032
Huang, Y., Wu, H., Hu, Y., Zhou, C., Wu, J., Wu, Y., et al. (2022). Puerarin Attenuates oxidative stress and ferroptosis via AMPK/PGC1α/Nrf2 pathway after subarachnoid hemorrhage in rats. Antioxidants (Basel) 11:1259.
Iacoangeli, A., Lin, T., Al Khleifat, A., Jones, A. R., Opie-Martin, S., Coleman, J. R. I., et al. (2020). Genome-wide meta-analysis finds the ACSL5-ZDHHC6 locus is associated with als and links weight loss to the disease genetics. Cell Rep. 33:108323. doi: 10.1016/j.celrep.2020.108323
Jia, M., Meng, D., Chen, M., Li, T., Zhang, Y. Q., and Yao, A. (2019). Drosophila homolog of the intellectual disability-related long-chain acyl-CoA synthetase 4 is required for neuroblast proliferation. J. Genet. Genom. 46, 5–17. doi: 10.1016/j.jgg.2018.10.006
Jiang, X., Stockwell, B. R., and Conrad, M. (2021). Ferroptosis: Mechanisms, biology and role in disease. Nat. Rev. Mol. Cell Biol. 22, 266–282.
Jin, Z. L., Gao, W. Y., Liao, S. J., Yu, T., Shi, Q., Yu, S. Z., et al. (2021). Paeonol inhibits the progression of intracerebral haemorrhage by mediating the HOTAIR/UPF1/ACSL4 axis. ASN Neuro 13:17590914211010647. doi: 10.1177/17590914211010647
Kahn-Kirby, A. H., Amagata, A., Maeder, C. I., Mei, J. J., Sideris, S., Kosaka, Y., et al. (2019). Targeting ferroptosis: A novel therapeutic strategy for the treatment of mitochondrial disease-related epilepsy. PLoS One 14:e0214250. doi: 10.1371/journal.pone.0214250
Kanter, J. E., Tang, C., Oram, J. F., and Bornfeldt, K. E. (2012). Acyl-CoA synthetase 1 is required for oleate and linoleate mediated inhibition of cholesterol efflux through ATP-binding cassette transporter A1 in macrophages. Biochim. Biophys. Acta 1821, 358–364. doi: 10.1016/j.bbalip.2011.10.008
Kenny, E. M., Fidan, E., Yang, Q., Anthonymuthu, T. S., New, L. A., Meyer, E. A., et al. (2019). Ferroptosis contributes to neuronal death and functional outcome after traumatic brain injury. Crit. Care Med. 47, 410–418. doi: 10.1097/CCM.0000000000003555
Kim, H. C., Lee, S. W., Cho, Y. Y., Lim, J. M., Ryoo, Z. Y., and Lee, E. J. (2009). RNA interference of long-chain acyl-CoA synthetase 6 suppresses the neurite outgrowth of mouse neuroblastoma NB41A3 cells. Mol. Med. Rep. 2, 669–674. doi: 10.3892/mmr_00000155
Klett, E. L., Chen, S., Yechoor, A., Lih, F. B., and Coleman, R. A. (2017). Long-chain acyl-CoA synthetase isoforms differ in preferences for eicosanoid species and long-chain fatty acids. J. Lipid Res. 58, 884–894. doi: 10.1194/jlr.M072512
Kram, H., Prokop, G., Haller, B., Gempt, J., Wu, Y., Schmidt-Graf, F., et al. (2022). Glioblastoma relapses show increased markers of vulnerability to ferroptosis. Front. Oncol. 12:841418. doi: 10.3389/fonc.2022.841418
Kuwata, H., and Hara, S. (2019). Role of acyl-CoA synthetase ACSL4 in arachidonic acid metabolism. Prostaglandins Other Lipid Mediat. 144:106363.
Li, C., Sun, G., Chen, B., Xu, L., Ye, Y., He, J., et al. (2021). Nuclear receptor coactivator 4-mediated ferritinophagy contributes to cerebral ischemia-induced ferroptosis in ischemic stroke. Pharmacol. Res. 174:105933. doi: 10.1016/j.phrs.2021.105933
Li, M., Meng, Z., Yu, S., Li, J., Wang, Y., Yang, W., et al. (2022). Baicalein ameliorates cerebral ischemia-reperfusion injury by inhibiting ferroptosis via regulating GPX4/ACSL4/ACSL3 axis. Chem. Biol. Interact. 366:110137. doi: 10.1016/j.cbi.2022.110137
Li, S., Wang, M., Wang, Y., Guo, Y., Tao, X., Wang, X., et al. (2021). p53-mediated ferroptosis is required for 1-methyl-4-phenylpyridinium-induced senescence of PC12 cells. Toxicol. Vitro 73:105146. doi: 10.1016/j.tiv.2021.105146
Li, Y., Feng, D., Wang, Z., Zhao, Y., Sun, R., Tian, D., et al. (2019). Ischemia-induced ACSL4 activation contributes to ferroptosis-mediated tissue injury in intestinal ischemia/reperfusion. Cell Death Differ. 26, 2284–2299. doi: 10.1038/s41418-019-0299-4
Liang, J., Shen, Y., Wang, Y., Huang, Y., Wang, J., Zhu, Q., et al. (2022). Ferroptosis participates in neuron damage in experimental cerebral malaria and is partially induced by activated CD8(+) T cells. Mol. Brain 15:57. doi: 10.1186/s13041-022-00942-7
Liao, S., Apaijai, N., Luo, Y., Wu, J., Chunchai, T., Singhanat, K., et al. (2021). Cell death inhibitors protect against brain damage caused by cardiac ischemia/reperfusion injury. Cell Death Discov. 7:312.
Liu, Z., Huang, Y., Hu, W., Huang, S., Wang, Q., Han, J., et al. (2014). dAcsl, the Drosophila ortholog of acyl-CoA synthetase long-chain family member 3 and 4, inhibits synapse growth by attenuating bone morphogenetic protein signaling via endocytic recycling. J. Neurosci. 34, 2785–2796. doi: 10.1523/JNEUROSCI.3547-13.2014
Liu, Z., Huang, Y., Zhang, Y., Chen, D., and Zhang, Y. Q. (2011). Drosophila Acyl-CoA synthetase long-chain family member 4 regulates axonal transport of synaptic vesicles and is required for synaptic development and transmission. J. Neurosci. 31, 2052–2063. doi: 10.1523/JNEUROSCI.3278-10.2011
Lopes-Marques, M., Cunha, I., Reis-Henriques, M. A., Santos, M. M., and Castro, L. F. (2013). Diversity and history of the long-chain acyl-CoA synthetase (Acsl) gene family in vertebrates. BMC Evol. Biol. 13:271. doi: 10.1186/1471-2148-13-271
Magtanong, L., Ko, P. J., To, M., Cao, J. Y., Forcina, G. C., Tarangelo, A., et al. (2019). Exogenous monounsaturated fatty acids promote a ferroptosis-resistant cell state. Cell Chem. Biol. 26, 420–432.e9. doi: 10.1016/j.chembiol.2018.11.016
Mao, X. Y., Zhou, H. H., and Jin, W. L. (2019). Redox-related neuronal death and crosstalk as drug targets: Focus on epilepsy. Front. Neurosci. 13:512. doi: 10.3389/fnins.2019.00512
Marszalek, J. R., Kitidis, C., Dirusso, C. C., and Lodish, H. F. (2005). Long-chain acyl-CoA synthetase 6 preferentially promotes DHA metabolism. J. Biol. Chem. 280, 10817–10826.
Mashima, T., Sato, S., Okabe, S., Miyata, S., Matsuura, M., Sugimoto, Y., et al. (2009a). Acyl-CoA synthetase as a cancer survival factor: Its inhibition enhances the efficacy of etoposide. Cancer Sci. 100, 1556–1562. doi: 10.1111/j.1349-7006.2009.01203.x
Mashima, T., Sato, S., Sugimoto, Y., Tsuruo, T., and Seimiya, H. (2009b). Promotion of glioma cell survival by acyl-CoA synthetase 5 under extracellular acidosis conditions. Oncogene 28, 9–19. doi: 10.1038/onc.2008.355
Meloni, I., Parri, V., De Filippis, R., Ariani, F., Artuso, R., Bruttini, M., et al. (2009). The XLMR gene ACSL4 plays a role in dendritic spine architecture. Neuroscience 159, 657–669. doi: 10.1016/j.neuroscience.2008.11.056
Miao, Z., Tian, W., Ye, Y., Gu, W., Bao, Z., Xu, L., et al. (2022). Hsp90 induces Acsl4-dependent glioma ferroptosis via dephosphorylating Ser637 at Drp1. Cell Death Dis. 13:548. doi: 10.1038/s41419-022-04997-1
Modi, H. R., Basselin, M., and Rapoport, S. I. (2014). Valnoctamide, a non-teratogenic amide derivative of valproic acid, inhibits arachidonic acid activation in vitro by recombinant acyl-CoA synthetase-4. Bipolar Disord. 16, 875–880. doi: 10.1111/bdi.12220
Modi, H. R., Ma, K., Chang, L., Chen, M., and Rapoport, S. I. (2017). Valnoctamide, which reduces rat brain arachidonic acid turnover, is a potential non-teratogenic valproate substitute to treat bipolar disorder. Psychiatry Res. 254, 279–283. doi: 10.1016/j.psychres.2017.04.048
Moujalled, D., Strasser, A., and Liddell, J. R. (2021). Molecular mechanisms of cell death in neurological diseases. Cell Death Differ. 28, 2029–2044.
Nakahara, K., Ohkuni, A., Kitamura, T., Abe, K., Naganuma, T., Ohno, Y., et al. (2012). The Sjögren-Larsson syndrome gene encodes a hexadecenal dehydrogenase of the sphingosine 1-phosphate degradation pathway. Mol. Cell 46, 461–471. doi: 10.1016/j.molcel.2012.04.033
Nakamura, R., Misawa, K., Tohnai, G., Nakatochi, M., Furuhashi, S., Atsuta, N., et al. (2020). A multi-ethnic meta-analysis identifies novel genes, including ACSL5, associated with amyotrophic lateral sclerosis. Commun. Biol. 3:526. doi: 10.1038/s42003-020-01251-2
Pang, Y., Liu, X., Wang, X., Shi, X., Ma, L., Zhang, Y., et al. (2022). Edaravone modulates neuronal GPX4/ACSL4/5-LOX to promote recovery after spinal cord injury. Front. Cell Dev. Biol. 10:849854. doi: 10.3389/fcell.2022.849854
Peng, W., Zhu, Z., Yang, Y., Hou, J., Lu, J., Chen, C., et al. (2021). N2L, a novel lipoic acid-niacin dimer, attenuates ferroptosis and decreases lipid peroxidation in HT22 cells. Brain Res. Bull. 174, 250–259. doi: 10.1016/j.brainresbull.2021.06.014
Pontifex, M. G., Martinsen, A., Saleh, R. N. M., Harden, G., Tejera, N., Müller, M., et al. (2021). APOE4 genotype exacerbates the impact of menopause on cognition and synaptic plasticity in APOE-TR mice. FASEB J. 35:e21583. doi: 10.1096/fj.202002621RR
Qiu, P. C., Lu, Y. Y., Zhang, S., Li, H., Bao, H., Ji, Y. Q., et al. (2020). Reduction of SCUBE3 by a new marine-derived asterosaponin leads to arrest of glioma cells in G1/S. Oncogenesis 9:71. doi: 10.1038/s41389-020-00252-4
Qu, X. F., Liang, T. Y., Wu, D. G., Lai, N. S., Deng, R. M., Ma, C., et al. (2021). Acyl-CoA synthetase long chain family member 4 plays detrimental role in early brain injury after subarachnoid hemorrhage in rats by inducing ferroptosis. CNS Neurosci. Ther. 27, 449–463. doi: 10.1111/cns.13548
Quan, J., Bode, A. M., and Luo, X. (2021). ACSL family: The regulatory mechanisms and therapeutic implications in cancer. Eur. J. Pharmacol. 909:174397. doi: 10.1016/j.ejphar.2021.174397
Rodriguez, J. D., Bhat, S. S., Meloni, I., Ladd, S., Leslie, N. D., Doyne, E. O., et al. (2010). Intellectual disability, midface hypoplasia, facial hypotonia, and Alport syndrome are associated with a deletion in Xq22.3. Am. J. Med. Genet. A 152A, 713–717. doi: 10.1002/ajmg.a.33208
Rossi Sebastiano, M., and Konstantinidou, G. (2019). Targeting long chain Acyl-CoA synthetases for cancer therapy. Int. J. Mol. Sci. 20:3624.
Saez-Atienzar, S., Bandres-Ciga, S., Langston, R. G., Kim, J. J., Choi, S. W., Reynolds, R. H., et al. (2021). Genetic analysis of amyotrophic lateral sclerosis identifies contributing pathways and cell types. Sci. Adv. 7:eabd9036.
Shao, C., Liu, Y., Chen, Z., Qin, Y., Wang, X., Wang, X., et al. (2022). 3D two-photon brain imaging reveals dihydroartemisinin exerts antiepileptic effects by modulating iron homeostasis. Cell Chem. Biol. 29, 43–56.e12. doi: 10.1016/j.chembiol.2021.12.006
Shao, C., Yuan, J., Liu, Y., Qin, Y., Wang, X., Gu, J., et al. (2020). Epileptic brain fluorescent imaging reveals apigenin can relieve the myeloperoxidase-mediated oxidative stress and inhibit ferroptosis. Proc. Natl. Acad. Sci. U.S.A. 117, 10155–10164. doi: 10.1073/pnas.1917946117
Smetana, J., Vallova, V., Wayhelova, M., Hladilkova, E., Filkova, H., Horinova, V., et al. (2021). Case report: Contiguous Xq22.3 deletion associated with ATS-ID syndrome: From genotype to further delineation of the phenotype. Front. Genet. 12:750110. doi: 10.3389/fgene.2021.750110
Song, L. M., Xiao, Z. X., Zhang, N., Yu, X. Q., Cui, W., Xie, J. X., et al. (2021). Apoferritin improves motor deficits in MPTP-treated mice by regulating brain iron metabolism and ferroptosis. iScience 24:102431. doi: 10.1016/j.isci.2021.102431
Soupene, E., and Kuypers, F. A. (2008). Mammalian long-chain acyl-CoA synthetases. Exp. Biol. Med. (Maywood) 233, 507–521.
Stockwell, B. R. (2022). Ferroptosis turns 10: Emerging mechanisms, physiological functions, and therapeutic applications. Cell 185, 2401–2421. doi: 10.1016/j.cell.2022.06.003
Tan, S., Hou, X., and Mei, L. (2020). Dihydrotanshinone I inhibits human glioma cell proliferation via the activation of ferroptosis. Oncol. Lett. 20:122. doi: 10.3892/ol.2020.11980
Tang, Y., Zhou, J., Hooi, S. C., Jiang, Y. M., and Lu, G. D. (2018). Fatty acid activation in carcinogenesis and cancer development: Essential roles of long-chain acyl-CoA synthetases. Oncol. Lett. 16, 1390–1396. doi: 10.3892/ol.2018.8843
Thomas, M. H., Paris, C., Magnien, M., Colin, J., Pelleïeux, S., Coste, F., et al. (2017). Dietary arachidonic acid increases deleterious effects of amyloid-β oligomers on learning abilities and expression of AMPA receptors: Putative role of the ACSL4-cPLA(2) balance. Alzheimers Res. Ther. 9:69.
Tuo, Q. Z., Liu, Y., Xiang, Z., Yan, H. F., Zou, T., Shu, Y., et al. (2022). Thrombin induces ACSL4-dependent ferroptosis during cerebral ischemia/reperfusion. Signal Transduct. Target. Ther. 7:59. doi: 10.1038/s41392-022-00917-z
Van Horn, C. G., Caviglia, J. M., Li, L. O., Wang, S., Granger, D. A., and Coleman, R. A. (2005). Characterization of recombinant long-chain rat acyl-CoA synthetase isoforms 3 and 6: Identification of a novel variant of isoform 6. Biochemistry 44, 1635–1642. doi: 10.1021/bi047721l
Wang, H., Huo, X., Han, C., Ning, J., Chen, H., Li, B., et al. (2021). Ferroptosis is involved in the development of neuropathic pain and allodynia. Mol. Cell. Biochem. 476, 3149–3161. doi: 10.1007/s11010-021-04138-w
Wang, J., Scholtens, D., Holko, M., Ivancic, D., Lee, O., Hu, H., et al. (2013). Lipid metabolism genes in contralateral unaffected breast and estrogen receptor status of breast cancer. Cancer Prev. Res. (Phila) 6, 321–330.
Wang, J., Zhu, Q., Wang, Y., Peng, J., Shao, L., and Li, X. (2022). Irisin protects against sepsis-associated encephalopathy by suppressing ferroptosis via activation of the Nrf2/GPX4 signal axis. Free Radic. Biol. Med. 187, 171–184. doi: 10.1016/j.freeradbiomed.2022.05.023
Wang, Q., Cai, J., Fang, C., Yang, C., Zhou, J., Tan, Y., et al. (2018). Mesenchymal glioblastoma constitutes a major ceRNA signature in the TGF-β pathway. Theranostics 8, 4733–4749. doi: 10.7150/thno.26550
Wang, T., Tomas, D., Perera, N. D., Cuic, B., Luikinga, S., Viden, A., et al. (2022). Ferroptosis mediates selective motor neuron death in amyotrophic lateral sclerosis. Cell Death Differ. 29, 1187–1198. doi: 10.1038/s41418-021-00910-z
Wei, N., Lu, T., Yang, L., Dong, Y., and Liu, X. (2021). Lipoxin A4 protects primary spinal cord neurons from Erastin-induced ferroptosis by activating the Akt/Nrf2/HO-1 signaling pathway. FEBS Open Bio 11, 2118–2126. doi: 10.1002/2211-5463.13203
Wright, H. J., Hou, J., Xu, B., Cortez, M., Potma, E. O., Tromberg, B. J., et al. (2017). CDCP1 drives triple-negative breast cancer metastasis through reduction of lipid-droplet abundance and stimulation of fatty acid oxidation. Proc. Natl. Acad. Sci. U.S.A. 114, E6556–E6565. doi: 10.1073/pnas.1703791114
Xiao, X., Jiang, Y., Liang, W., Wang, Y., Cao, S., Yan, H., et al. (2019). miR-212-5p attenuates ferroptotic neuronal death after traumatic brain injury by targeting Ptgs2. Mol. Brain 12:78. doi: 10.1186/s13041-019-0501-0
Xu, C., Zhao, J., Song, J., Xiao, M., Cui, X., Xin, L., et al. (2022). lncRNA PRADX is a mesenchymal glioblastoma biomarker for cellular metabolism targeted therapy. Front. Oncol. 12:888922. doi: 10.3389/fonc.2022.888922
Yamashita, Y., Kumabe, T., Cho, Y. Y., Watanabe, M., Kawagishi, J., Yoshimoto, T., et al. (2000). Fatty acid induced glioma cell growth is mediated by the acyl-CoA synthetase 5 gene located on chromosome 10q25.1-q25.2, a region frequently deleted in malignant gliomas. Oncogene 19, 5919–5925. doi: 10.1038/sj.onc.1203981
Yan, H., Yan, Y., Gao, Y., Zhang, N., Kumar, G., Fang, Q., et al. (2022). Transcriptome analysis of fasudil treatment in the APPswe/PSEN1dE9 transgenic (APP/PS1) mice model of Alzheimer’s disease. Sci. Rep. 12:6625. doi: 10.1038/s41598-022-10554-9
Yan, S., Yang, X. F., Liu, H. L., Fu, N., Ouyang, Y., and Qing, K. (2015). Long-chain acyl-CoA synthetase in fatty acid metabolism involved in liver and other diseases: An update. World J. Gastroenterol. 21, 3492–3498. doi: 10.3748/wjg.v21.i12.3492
Yang, N., Guan, Q. W., Chen, F. H., Xia, Q. X., Yin, X. X., Zhou, H. H., et al. (2020). Antioxidants targeting mitochondrial oxidative stress: Promising neuroprotectants for epilepsy. Oxid. Med. Cell. Longev. 2020:6687185. doi: 10.1155/2020/6687185
Yao, M. Y., Liu, T., Zhang, L., Wang, M. J., Yang, Y., and Gao, J. (2021). Role of ferroptosis in neurological diseases. Neurosci. Lett. 747:135614.
Yee, P. P., Wei, Y., Kim, S. Y., Lu, T., Chih, S. Y., Lawson, C., et al. (2020). Neutrophil-induced ferroptosis promotes tumor necrosis in glioblastoma progression. Nat. Commun. 11:5424. doi: 10.1038/s41467-020-19193-y
Yi, R., Wang, H., Deng, C., Wang, X., Yao, L., Niu, W., et al. (2020). Dihydroartemisinin initiates ferroptosis in glioblastoma through GPX4 inhibition. Biosci. Rep. 40:BSR20193314. doi: 10.1042/BSR20193314
Yuan, B., Zhao, X. D., Shen, J. D., Chen, S. J., Huang, H. Y., Zhou, X. M., et al. (2022). Activation of SIRT1 alleviates ferroptosis in the early brain injury after subarachnoid hemorrhage. Oxid. Med. Cell. Longev. 2022:9069825. doi: 10.1155/2022/9069825
Zhang, H. L., Hu, B. X., Li, Z. L., Du, T., Shan, J. L., Ye, Z. P., et al. (2022). PKCβII phosphorylates ACSL4 to amplify lipid peroxidation to induce ferroptosis. Nat. Cell Biol. 24, 88–98. doi: 10.1038/s41556-021-00818-3
Zhang, X., Song, T., Zhao, M., Tao, X., Zhang, B., Sun, C., et al. (2022). Sirtuin 2 alleviates chronic neuropathic pain by suppressing ferroptosis in rats. Front. Pharmacol. 13:827016. doi: 10.3389/fphar.2022.827016
Zhang, Y., Chen, D., and Wang, Z. (2009). Analyses of mental dysfunction-related ACSl4 in Drosophila reveal its requirement for Dpp/BMP production and visual wiring in the brain. Hum. Mol. Genet. 18, 3894–3905. doi: 10.1093/hmg/ddp332
Zhou, H., Yin, C., Zhang, Z., Tang, H., Shen, W., Zha, X., et al. (2020). Proanthocyanidin promotes functional recovery of spinal cord injury via inhibiting ferroptosis. J. Chem. Neuroanat. 107:101807. doi: 10.1016/j.jchemneu.2020.101807
Zhou, L., Wang, Z., Hu, C., Zhang, C., Kovatcheva-Datchary, P., Yu, D., et al. (2019). Integrated metabolomics and lipidomics analyses reveal metabolic reprogramming in human glioma with IDH1 mutation. J. Proteome Res. 18, 960–969. doi: 10.1021/acs.jproteome.8b00663
Zhu, Z. Y., Liu, Y. D., Gong, Y., Jin, W., Topchiy, E., Turdi, S., et al. (2022). Mitochondrial aldehyde dehydrogenase (ALDH2) rescues cardiac contractile dysfunction in an APP/PS1 murine model of Alzheimer’s disease via inhibition of ACSL4-dependent ferroptosis. Acta Pharmacol. Sin. 43, 39–49. doi: 10.1038/s41401-021-00635-2
Keywords: long-chain acyl-coenzyme A synthetases (ACSLs), fatty acid (FA) metabolism, ferroptosis, nervous system diseases, targeted therapy
Citation: Wu Z, Sun J, Liao Z, Qiao J, Chen C, Ling C and Wang H (2022) An update on the therapeutic implications of long-chain acyl-coenzyme A synthetases in nervous system diseases. Front. Neurosci. 16:1030512. doi: 10.3389/fnins.2022.1030512
Received: 29 August 2022; Accepted: 07 November 2022;
Published: 24 November 2022.
Edited by:
Zhengwu Peng, Fourth Military Medical University, ChinaReviewed by:
Jeroen Bogie, University of Hasselt, BelgiumCaterina Bartolacci, University of Cincinnati, United States
Copyright © 2022 Wu, Sun, Liao, Qiao, Chen, Ling and Wang. This is an open-access article distributed under the terms of the Creative Commons Attribution License (CC BY). The use, distribution or reproduction in other forums is permitted, provided the original author(s) and the copyright owner(s) are credited and that the original publication in this journal is cited, in accordance with accepted academic practice. No use, distribution or reproduction is permitted which does not comply with these terms.
*Correspondence: Hui Wang, ZG9jdG9yd2FuZ2h1aUAxMjYuY29t
†These authors have contributed equally to this work