- 1Department of Neuroscience, Schulich School of Medicine and Dentistry, Western University, London, ON, Canada
- 2Department of Psychiatry, Schulich School of Medicine and Dentistry, Western University, London, ON, Canada
Although the manifestation of trauma in the body is a phenomenon well-endorsed by clinicians and traumatized individuals, the neurobiological underpinnings of this manifestation remain unclear. The notion of somatic sensory processing, which encompasses vestibular and somatosensory processing and relates to the sensory systems concerned with how the physical body exists in and relates to physical space, is introduced as a major contributor to overall regulatory, social-emotional, and self-referential functioning. From a phylogenetically and ontogenetically informed perspective, trauma-related symptomology is conceptualized to be grounded in brainstem-level somatic sensory processing dysfunction and its cascading influences on physiological arousal modulation, affect regulation, and higher-order capacities. Lastly, we introduce a novel hierarchical model bridging somatic sensory processes with limbic and neocortical mechanisms regulating an individual’s emotional experience and sense of a relational, agentive self. This model provides a working framework for the neurobiologically informed assessment and treatment of trauma-related conditions from a somatic sensory processing perspective.
Introduction
Our physical body is the home within which our brain resides, and as such it provides an anchor to who we are and how we move about in the world. The body is connected to the brain via afferent and efferent tracts, or bundles of nerve fibers, which allow swift and efficient integration of sensation, emotion, cognition, and action. Elementally, an organism receives sensory input, contextualizes it with emotional and cognitive information, and effects an appropriate response. As such, sensory processing refers the capacity to register, organize, and modulate incoming sensory information from the internal or external milieu, where it is then integrated with sensory input from other modalities and utilized to guide a goal-oriented behavioral response (Gilbert and Sigman, 2007; Miller et al., 2007; Alais et al., 2010; Atick, 2011). Here, we con sider how alterations in the neural pathways crucial for the processing and integration of somatic, or body-based, sensations may be off-balance due to severe or chronic traumatization, with the diagnoses of post-traumatic stress disorder (PTSD) and its dissociative subtype (PTSD + DS) as well-researched examples. Alterations to multisensory processing have been shown to mediate higher-order cognition (Alais et al., 2010; Wallace et al., 2020), emotion (Klasen et al., 2012), social capacities (Baranek et al., 2018; Stevenson et al., 2018; Thye et al., 2018), and sense of self (Panksepp and Northoff, 2009; Tsakiris, 2017), which are globally impacted in trauma-related disorders.
An altered neural defense circuitry leading to persistent sensory and emotional overwhelm in response to stimuli is evident in trauma-related disorders. The symptomology of PTSD and PTSD + DS indicates maladaptive responsivity to incoming information, including physiological arousal dysregulation, impulsivity, diminished sense of agency, altered sense of time, and social difficulties or isolation (American Psychiatric Association [APA], 2013; Cox et al., 2014). PTSD is diagnosed after a traumatic event which elicited feelings of terror and/or a threat to one’s or another’s life. Symptoms include flashbacks or dissociative re-experiencing, hypervigilance, increased startle responses, behavioral avoidance of potential triggers, and pervasive negative cognitions (American Psychiatric Association [APA], 2013). Additionally, PTSD + DS occurs in up to 44% of individuals with PTSD and is characterized by additional symptoms of derealization and depersonalization (Lanius et al., 2010b; Steuwe et al., 2012; Wolf et al., 2012b; Stein et al., 2013; Armour et al., 2014; Blevins et al., 2014; for a review, see Hansen et al., 2017; White et al., 2022). In derealization, an individual feels as though their external environment is slowed down, foggy, dream-like, or otherwise unreal, while in depersonalization the individual feels as if they are detached from or even floating above their physical body. PTSD + DS is highly associated with early life traumatization, where dissociation acts as an adaptive response to inescapable threat (Nijenhuis et al., 1998a; Chu et al., 1999; Spiegel et al., 2011; Wolf et al., 2012a; Stein et al., 2013; Lowenstein, 2018). Here, pathological dissociation may be an “internal mechanism by which terrorized people are silenced” (Herman, 1992, p. 239), with adaptive neurobiological mechanisms in place for suppressing the sensory and emotional overwhelm of chronic trauma. During a traumatic experience (peritraumatically) negatively valenced sensory input overwhelms lower-level processing regions within the brainstem and midbrain. Peritraumatically, individuals feel unsafe, under threat, out of control and prevented from getting their (or another’s) survival needs met; trauma-related disorders arise when these perceptions persist post-traumatically. For those with a history of chronic emotional and/or physical neglect, lower-level brain regions are starved for the type of sensory input inherent in positively valenced and safe physical and social interactions. Mammalian survival needs include attuned caregiving during neurodevelopment and reciprocal relationships in adulthood for collective safety, survival, and continuation of the species. Therefore, emotional neglect is traumatizing as it engenders a perpetual fear that survival needs will not be met.
Post-traumatic stress disorder (PTSD) has been broadly associated with sensory modulation difficulties, manifested as either hyper- or hypo-responsivity to sensory input (Shalev et al., 2000; Engel-Yeger et al., 2013; Devine et al., 2020; Yochman and Pat-Horenczyk, 2020; Joseph et al., 2021). Sensory hyper-responsivity is most evident in PTSD and characterized by lower thresholds for registering and thus alerting to incoming sensory information even in the absence of threat, resulting in frequent states of overwhelm. A persistently heightened arousal state due to facilitated threat detection circuitry may explain the enhanced detection of innocuous stimuli as threatening (Harricharan et al., 2021). Alternatively, those with dissociative symptoms experience states of sensory hypo-sensitivity including analgesia/anesthesia (Nijenhuis et al., 1998b), suggesting heightened neurological thresholds for sensory registration. Depersonalization corresponds with somatic sensory hypo-responsivity, while derealization suggests exteroceptive sensory hypo-responsivity. These lowered and heightened thresholds may be linked with under- and over-modulation, respectively, of raw sensory and emotional input from subcortical regions. In PTSD, subcortical regions drive cortical overload, while in PTSD + DS cortical regions over-modulate lower regions resulting in bodily and emotional detachment (Lanius et al., 2010b). Together, traumatogenic conditions may be a manifestation of disrupted subcortico-cortical or vertical integration, where the bipartisan modulation between lower and higher regions is off-balance, particularly within the midline neural circuitry poised to engender a primordial sense of bodily and affective self as a coherent and stable entity in relation to its environment (Panksepp and Northoff, 2009). This alteration to vertical integration has a cascading impact on thalamo-cortical and cortico-cortical horizontal integration of cortical brain structures. Vertical and horizontal integration relates to neural synchrony, in that lower and higher, as well as medial and lateral, reaches of the brain may be structurally sound yet lacking in fluid communication (Llinás, 1970).
Here, we introduce a neurobiologically informed perspective from which to view trauma-based conditions considering how sensory processing contributes to an organism’s ability to regulate physiological arousal, emotions, and actions (Harricharan et al., 2021). Specifically, we focus on the importance of the somatic (vestibular and somatosensory) senses given their direct relevance to the physical body, their positions of phylogenetic and ontogenetic primacy, and the major roles they play in attenuating and orchestrating our multisensory experience in the present moment. Somatic sensory processing is hypothesized to give rise to adequate and efficient processing of interoceptive and exteroceptive (visual, auditory, olfactory, gustatory) sensory information. The overall aim of this review is to offer a transdisciplinary, neuroscientifically informed perspective of how somatic sensory processing contributes to trauma-related symptomology. We review (1) an overview of the vestibular and somatosensory systems; (2) phylogenetic and ontogenetic development of the somatic sensory systems; (3) the neurobiology of sensory processing as mediated by the periaqueductal gray; (4) trauma as an assault on the senses; (5) somatic sensory contributions to the sense of self after trauma; and (6) what neuroscience can teach us about connecting somatic sensory processing and trauma-related disorders.
Overview of the somatic sensory systems: Vestibular and somatosensory
The vestibular system
The vestibular system, comprised of angular motion-detecting semicircular canals and linear motion-detecting otolith organs in the inner ear, informs us of three-dimensional head acceleration as a function of the linear pull of gravity (Lopez and Blanke, 2011; Holstein, 2012; Jamon, 2014). It is a sensory system that never “sleeps” – gravity is an omnipresent and unchanging force which perpetually stimulates the vestibular system, impacting everyday movements in relation to the physical and social world around us. As a constant, gravity is predictable and something we come to subconsciously understand during our formative years. As such, developing a reference to gravity is critical in orienting our bodies in space, navigating through the environment, maintaining an upright and vertical orientation as bipeds, and sensing the passage of time as a function of terrestrial motion (Brandt et al., 1994; Lacquaniti et al., 2015; Ferrè and Haggard, 2016). The vestibular system’s workings remain subconscious until we unexpectedly trip or accelerate, increasing autonomic arousal via the vestibular nuclei’s descending projections modulating vestibulo-sympathetic reflexive influences on breathing, heart rate, and blood flow (Hernandez and Das, 2020) and their connections with the reticular activating system (RAS; Peterson et al., 1975). Anyone who has unexpectedly lost balance can attest to the quickened heart rate, dropped sensation in the gut, and gasping that ensue, procuring our immediate attention. These autonomic and motoric responses modulate arousal and maintain our physical safety prior to reaching awareness. Vestibular processing, therefore, is inextricable with a sense of security, grounding, and safety.
Vestibular stimulation occurs when crystals and fluid are displaced in the otoliths and semicircular canals, respectively, sending signals to the vestibular nuclei via the pontomedullary junction (Carmona et al., 2009; Jamon, 2014) or the flocculonodular lobes of the cerebellum via the inferior cerebellar peduncle (Highstein and Holstein, 2006). Proprioceptive input from the head, neck and trunk integrates with vestibular input at the vestibular nuclei, thalamus, and cerebellum, contextualizing the motion as self- or other-initiated (Deecke et al., 1977; Gdowski and McCrea, 2000; Luan et al., 2013). Neurons from the vestibular nuclei project down the lateral vestibulospinal tract to influence muscle tone and postural control (Yoo and Mihaila, 2020), down to innervate vestibulo-sympathetic reflexes of the heart and lungs (Hernandez and Das, 2020), across to the flocculonodular lobe in the cerebellum for balance, coordination, and emotion processing (Britton and Arshad, 2019), and up to the inferior and superior colliculi of the midbrain and multisensory integrative areas of the cortex to contribute toward body awareness, spatial processing/memory, arousal modulation, first-person perspective, and social cognition (Ferrè and Haggard, 2020; Rabellino et al., 2022) (Figure 1).
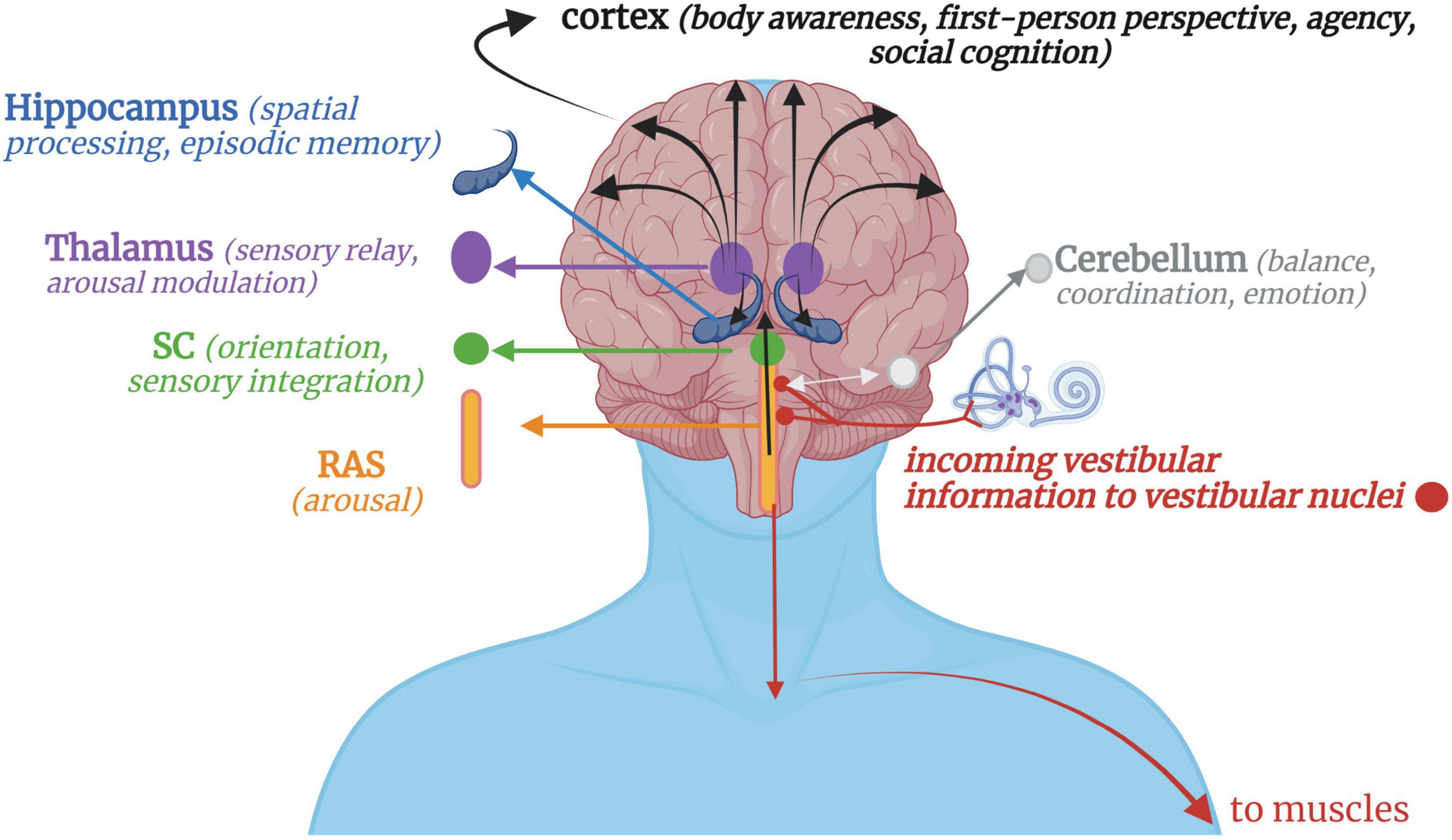
Figure 1. A simplified schematic of vestibular projections throughout the nervous system. Afferent input from gravitational forces arrives at the vestibular nuclei, which integrate with somatosensory input via the cerebellum, reticular formation, and spinal afferent tracts. Downward vestibular efferent projections send signals for muscular extension and postural control. Reciprocal connections with the cerebellum, mainly its flocculonodular lobe, allow for feedback-feedforward mechanisms of motor coordination and fluency, with the cerebellum projecting information back to the vestibular nuclei or directly up to the cortex. Ascending vestibular projections integrate with the RAS and SC for arousal modulation and orienting responses before continuing on to higher cortical structures via the thalamus. Vestibular projections to the cortex then contribute to higher-order cognitive processes such as a sense of agency, first-person perspective, social cognition, and bodily self-consciousness. RAS, Reticular activating system; SC, Superior colliculi.
Originally thought as solely involved in balance and oculomotor processes, vestibular projections are now known to be multifaceted in their influences given their widespread innervation of the brain and body (Carmona et al., 2009; Lopez and Blanke, 2011; Ferrè and Haggard, 2016) and their multisensory nature (Raiser et al., 2020; Huber et al., 2021). Based on anecdotal observations, meditative employment of rocking by Buddhist monks, whirling by Sufi semazen-artists, and mindful movements by yogic practitioners speaks to the universal and profound impact vestibular stimulation has on quieting the mind’s chatter and supporting a state of presence and tranquility. Functionally, vestibular input has been shown to be involved in motor control (Nandi and Luxon, 2008; Takakusaki, 2017), autonomic arousal regulation of respiration and blood pressure (Balaban and Porter, 1998; Biaggioni et al., 1998; Furman et al., 1998; Yates and Miller, 1998; Murakami et al., 2002), emotion regulation (Balaban and Thayer, 2001), social cognition (Mast et al., 2014; Deroualle et al., 2015), and maintenance of a coherent representation of the body (Mast et al., 2014; Ferrè and Haggard, 2016). Unlike other sensory systems which have a primary thalamic relay and cortical processing area, no focal processing region dedicated solely to the vestibular system has been defined (Hitier et al., 2014; Wijesinghe et al., 2015). A “vestibular cortex” has been delineated in the primate and other mammals (Akbarian et al., 1992; Guldin et al., 1992; for a review, see Guldin and Grüsser, 1998) but remains to be definitively mapped in the human brain largely due to the confounds presented by its integration with other sensory stimuli (zu Eulenburg et al., 2012; Frank and Greenlee, 2018). The most consistent and robust findings of vestibular-cortical projections have involved the insula and the surrounding parietal operculum, temporo-parietal junction (TPJ), superior temporal gyrus, somatosensory cortex, and mid-cingulate cortex (Guldin and Grüsser, 1998; Lopez and Blanke, 2011; Frank and Greenlee, 2018), the largest hubs being the parietal operculum (OP2) (zu Eulenburg et al., 2012; Huber et al., 2021; Ibitoye et al., 2022) and TPJ (Bottini et al., 1994, 2001; Lopez and Blanke, 2011). These multimodal processing regions are inclusive of auditory, visual, and somatosensory input, suggesting a multisensory binding role played by the vestibular system (Pfeiffer et al., 2013; Shayman et al., 2018). Multisensory binding then gives rise to a unified multisensory experience underlying self-representation and bodily self-awareness (Ferrè et al., 2014). This has been evidenced by lengthened multisensory temporal binding windows in individuals with vestibular hypofunction, such that multisensory inputs take longer for transmission and processing resulting in disjointed arrivals at multisensory integrative brain regions (Shayman et al., 2018).
Vestibular input purportedly modulates and manages sensory integrative processes by balancing sensory signals in response to internal and external environmental demands (Bense et al., 2001; Ferrè and Haggard, 2016). This modulatory role is crucial in multisensory integration, where the balancing of interoceptive and exteroceptive sensory input is critical in creating and maintaining our perception of reality while guiding adaptive and purposeful behavior (Harricharan et al., 2021). Vestibular stimulation has a modulating effect on proprioceptive (Gallagher et al., 2021), tactile, nociceptive (McGeoch et al., 2009; Ferrè et al., 2013), and visual-proprioceptive cues (Ponzo et al., 2018). Further, optogenetic stimulation of the medial vestibular nuclei results in enhanced cortical activations in response to visual and auditory stimuli (Leong et al., 2019), while caloric vestibular stimulation has an analgesic effect (Ferrè and Haggard, 2015) in healthy individuals. Here, vestibular input adjusts perceptual experience in relation to an ever-changing body-environment dynamic, thereby contributing to fluid and adaptive anticipation of and reaction to future events (Mast et al., 2014; Takakusaki, 2017).
Although the vestibular system’s unusually widespread influences on the brain support the notion that it contributes toward cognitive and emotional processes in health and disease (Balaban et al., 2011; Hoffe and Balaban, 2011; Ferrè and Haggard, 2020), its consideration in trauma-related conditions is relatively novel. Where vestibular signals are in a unique position to directly modulate both low-level multisensory processing as well as higher-order bodily representations, it may be uniquely positioned to contribute to the widespread alterations in sensory modulation and one’s orientation toward reality seen in dissociative episodes and flashbacks. In PTSD, it is well-known that hypervigilance, exaggerated startle response, and pervasive negative emotionality plagues everyday existence. Traumatized individuals feel unsafe or under threat when confronted with a barrage of sensory signals within the context of a currently or previously threatening situation, resulting in a hyperfocus on potentially dangerous exteroceptive stimuli. Although the mechanisms are less than clear, the role of vestibular information in modulating multisensory inputs may be faulty in PTSD and contribute toward state-dependent sensory hyper and hypo-sensitivities to exteroceptive stimuli. Disturbed temporal binding of sensory information engenders perceptual chaos and lack of coherence, which may lead to bodily disconnect (“I feel dead inside”) as well as states of hypervigilance (“I have to be on guard all of the time”) (Foa et al., 1999). Importantly, significant variation in vestibular nuclei connectivity between PTSD and PTSD + DS has been shown (Harricharan et al., 2017), with PTSD + DS exhibiting significantly decreased connectivity with the TPJ and cortical regions. Given the dissociative symptomology within this population, a lack of vestibular innervation of regions involved in bodily consciousness may result in frequent and profound detachment from the body and/or environment manifesting as depersonalization and derealization symptoms, respectively.
The somatosensory system
The somatosensory system is comprised of the skin, muscles, and joints which detect light touch, deep pressure, pain, temperature, and proprioceptive input. The somatosensory system contributes toward both interoceptive and exteroceptive processing due to its perception of stimuli originating inside and outside of the body, impacting higher order awareness of the physiological state of the body and the immediate external environment, respectively (Abraira and Ginty, 2013). Tactile and proprioceptive information provide crucial information about the body in physical space and as it relates to the environment and others. Given that tactile input has a broad impact on bodily function and percept, ranging from simple reflexes to arousal regulation to complex social processes, it is also highly influential in numerous central nervous system processes (Ayres, 1972; McGlone et al., 2014; Lane et al., 2019).
Touch is detected through specialized cutaneous mechanoreceptors (Gillespie and Walker, 2001; del Valle et al., 2012) and can be further delineated into non-affective and affective touch (McGlone et al., 2014). Newborns respond differentially to affective and non-affective touch (della Longa et al., 2021), indicating that these two channels operate separately from birth. Discriminative or non-affective touch travels via myelinated A-beta afferents projecting to the primary somatosensory cortex (S1) (Olausson et al., 2010; Manzotti et al., 2019). It perceives and localizes the shape and surface structure of objects, guides motor actions, and provides feedback from the body’s active engagement with its environment (McGlone et al., 2007). This pathway is also important for postural schema, which relates to the perceptual representation of the size and shape of the body (Longo et al., 2009; Serino and Haggard, 2010). Deep touch pressure and vibration stimulate Pacinian corpuscles, another type of mechanoreceptor deeper in the skin (Bell et al., 1994), which have effects on autonomic arousal; deep pressure for even brief time periods have resulted in decreased sympathetic arousal (Reynolds et al., 2015) while moderate deep pressure massage has been associated with increased parasympathetic activity (Diego and Field, 2009). Pain and temperature are detected through differentiated high-threshold mechanoreceptors which travel through the unmyelinated spinothalamic tract. The spinothalamic tract travels from the body to the thalamus via the reticular formation, influencing arousal and behavior in response to potential harm or danger (Fregoso et al., 2019).
Affective touch is experienced through the activation of low-threshold tactile mechanoreceptors (C-LTMR) which respond preferentially to gentle, stroking, skin-temperature touch on the hairy regions of the skin (Löken et al., 2009; Morrison et al., 2011; McGlone et al., 2014). Unmyelinated “CT-afferent” pathways (Ackerley et al., 2014; Björnsdotter et al., 2014) transmit affective touch and selectively activate the posterior insula (PI) (Olausson et al., 2002, 2008; Davidovic et al., 2019; Tuulari et al., 2019), a cortical region involved in awareness of the body’s physiological status (Craig, 2002; Olausson et al., 2010). Stimulation of CT-afferent pathways relates to positive affect (Pawling et al., 2017), relief of physical and emotional pain (von Mohr et al., 2017, 2018), reduction of heart rate (Ditzen et al., 2007; Fairhurst et al., 2014; Manzotti et al., 2019), improvement of interoceptive awareness (Crucianelli et al., 2018; Crucianelli and Ehrsson, 2022), attenuation of anxiety/defensive responses (Liu et al., 2018; Walker et al., 2020; Wang et al., 2020), and seeking of social contact as mediated by oxytocin release (Olausson et al., 2002; Morrison et al., 2010; Brzozowska et al., 2022). In rat studies, maternal care is indexed by maternal grooming (licking) behavior toward pups, which has repeatedly been shown to attenuate stress and regulate the development of hormonal, emotional, and cognitive responses to stressors (Caldji et al., 1998; Liu et al., 2000; Meaney, 2001). Increased receptor levels for oxytocin are reported with repeated provision of tactile-rich grooming stimuli in young rats (Francis et al., 2000; Champagne et al., 2001). Importantly, CT-afferent pathway stimulation simulating maternal grooming is sufficient for enhancing oxytocin neuronal firing and promoting prosocial behaviors when rats reached adulthood, while touch deprivation leads to social isolation and diminished preference for social contexts (Bourinet et al., 2021; Yu et al., 2022). Therefore, affective touch is a medium through which we display social concern and attenuate stress, non-verbally communicating our presence in real time and space with another (Gallace and Spence, 2010; Cascio et al., 2019; Ciaunica et al., 2021a; Bohic and Abraira, 2022).
While tactile receptors provide information about the body’s contact with its environment and others, proprioceptors inform where the physical body is in space. Proprioception is detected through Golgi tendon bodies in the joints and muscle spindle fibers in muscle tissue, and informs of joint position and muscle fiber recruitment (Tuthill and Azim, 2018). Skin mechanoreceptors also contribute to proprioception when skin stretches in response to changes in joint angle (Taylor, 2009). The integration of proprioceptive input from the neck, trunk, and limbs with vestibular input at the brainstem level is crucial to inform us whether just our head, our trunk and head, or our whole body has moved in space. Vibration applied to tendons elicits illusory limb motion and dimensionality, impacting body schema and balance (Eklund, 1972; Goodwin et al., 1972; Lackner, 1988; McGlone et al., 2002; Romaiguère et al., 2003; McIntyre and Seizova-Cajic, 2007; Seizova-Cajic and Sachtler, 2007; Fasold et al., 2008; Palluel et al., 2011) and illuminating the importance of proprioception for our sense of stability and constancy with regard to the body in space (Taylor, 2009).
Several somatosensory tracts exist depending on the location and type of input (Figure 2). Non-affective tactile and proprioceptive input project along the dorsal column-medial lemniscal pathway to the superior colliculus, thalamus, and S1, where a representation of the physical body is constructed (Edwards et al., 1979; Kosinski et al., 1988; Taylor, 2009). Proprioceptive input travels to the cerebellum via the spinocerebellar and cuneocerebellar tracts for motor coordination, motoric adaptations to environmental demands, and arousal regulation via connections with the RAS (Ayres, 1972), a structure within the reticular formation which spans the brainstem from the medulla to the mesencephalon (French and Magoun, 1952; Wijdicks, 2019). Proprioception also projects to the cortex via the thalamus to allow for awareness and voluntary control over the body’s position and movements (Taylor, 2009; Tuthill and Azim, 2018). It also innervates the brainstem, including the vestibular nuclei, suggesting widespread influences and elusiveness with regard a primary processing area.
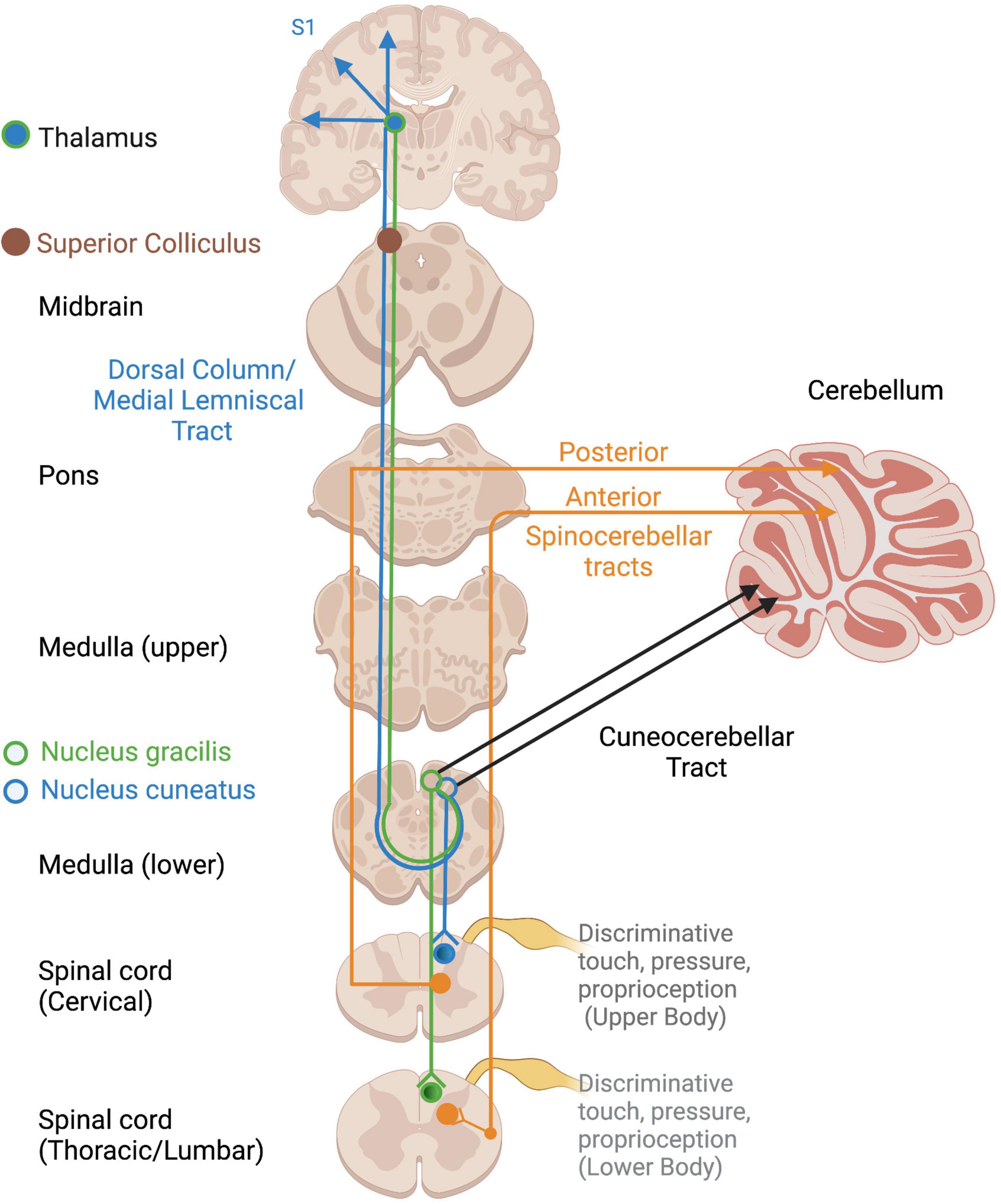
Figure 2. Ascending cortical and cerebellar somatosensory tracts. Open circles reflect terminations of sensory tracts, after which projections take various pathways. DC/ML, Dorsal column/Medial lemniscus; VPL, ventral posterior lateral nucleus of thalamus.
Somatosensory information of homeostatic relevance (pain, temperature, affective touch) is conveyed by unmyelinated, phylogenetically older afferent tracts (Marshall and McGlone, 2020). Pain and temperature detected through nociceptors and thermoreceptors travel in an anatomically distinct pathway to the thalamus and S1 via smaller-diameter, unmyelinated axons of the spinothalamic tract (Taylor, 2009) (Figure 3). Given the vast literature in pain processing and our focus on somatosensory contributions to embodiment and self, it is beyond the scope of this paper and will not be covered in this review (for a review of trauma-related pain disorders, see Brennstuhl et al., 2015; Kind and Otis, 2019). Several pathways which innervate subcortical regions such as the inferior and superior colliculi and reticular formation also carry somatosensory and nociceptive information and may be evolutionary relics of phylogenetically primitive animals without neocortices (Figure 3). Direct somatosensory input to the midbrain tectum via the spinotectal tract, as well as the reticular formation via the spinoreticular tract, is an important consideration for how somatosensory input mediates multisensory integration.
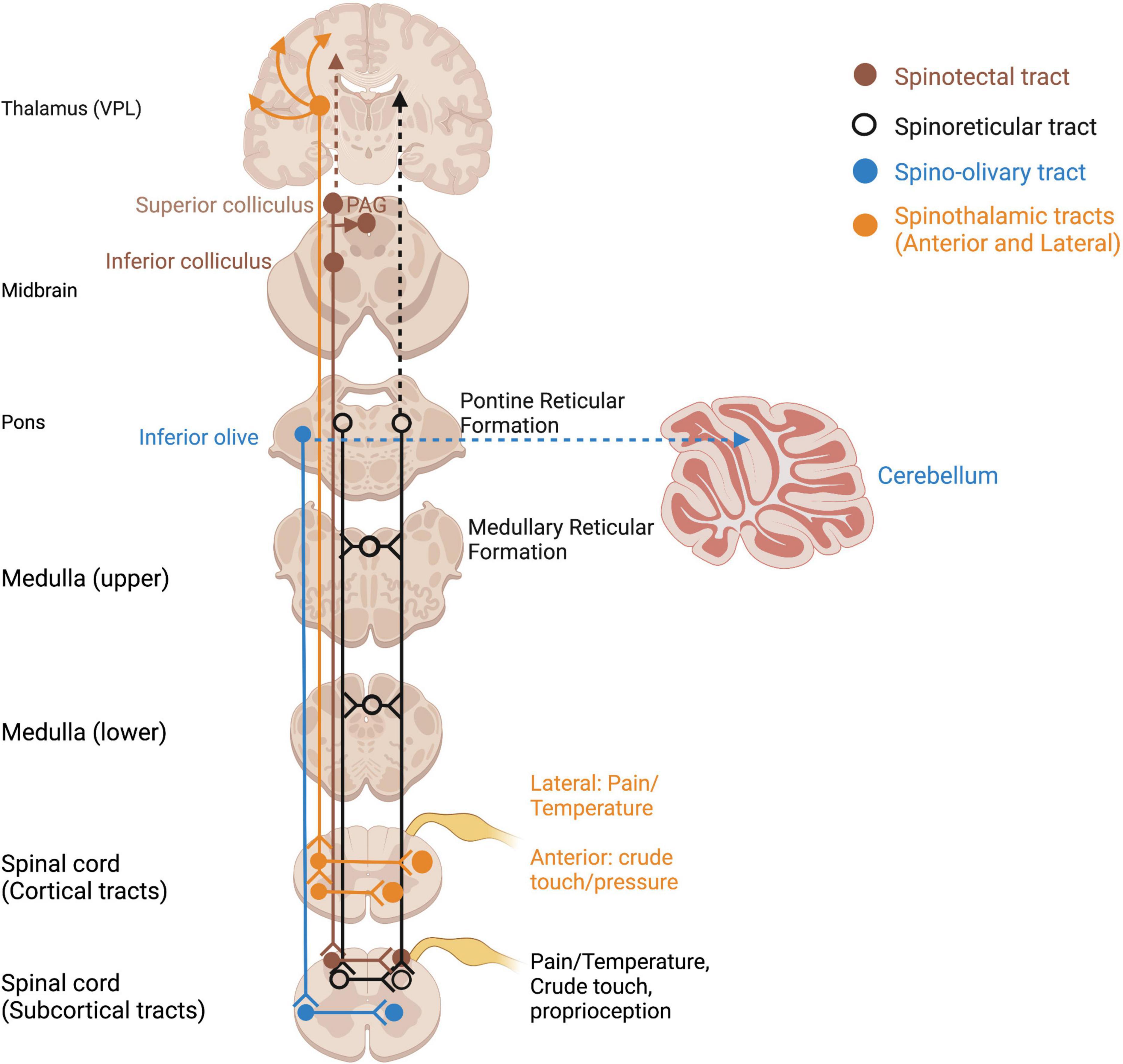
Figure 3. Ascending spinothalamic and subcortical somatosensory tracts. Open circles reflect terminations of sensory tracts, after which projections take various pathways. PAG, periaqueductal gray; VPL, ventral posterior lateral nucleus of thalamus.
Given its recent discovery as a separate somatosensory phenomenon, the neural pathway taken by affective touch remains speculative. Figure 4 presents a hypothetical CT-afferent pathway based on its innervation of the posterior insula in humans (Olausson et al., 2002, 2008; Björnsdotter et al., 2009; McGlone et al., 2014; Jönsson et al., 2018; Tuulari et al., 2019) and the periaqueductal gray (PAG) and hypothalamus in mice (Yu et al., 2022). Indeed, damage to the right insula reduces pleasantness ratings of CT-optimized touch (Kirsch et al., 2020). Some researchers have categorized affective touch as an interoceptive sensation as it conveys information about the body’s internal state and contributes to experiences of emotion and physiological state (Björnsdotter et al., 2014; Marshall and McGlone, 2020); however, it should be noted that others regard any externally applied stimulus as exteroceptive, reserving the term interoception for internally generated feedback informing upon the state of the body (Craig, 2002; Critchley and Garfinkel, 2017; Allen, 2020). Interestingly, while CT-optimized touch is perceived as highly pleasant in healthy individuals (Löken et al., 2009), individuals with PTSD and/or history of trauma can report it as feeling unpleasant and intense (Badura-Brack et al., 2015; Strauss et al., 2019; Maier et al., 2020). Individuals who experienced a lack of positively valenced, affectionate touch in childhood, such as in cases of neglect and abuse, present with blunted sensitivity to the social value of touch (Devine et al., 2020) and lower blood plasma oxytocin levels (Heim et al., 2009; Donadon et al., 2018), which may be tied to disruptions in CT-afferent driven neurodevelopment.
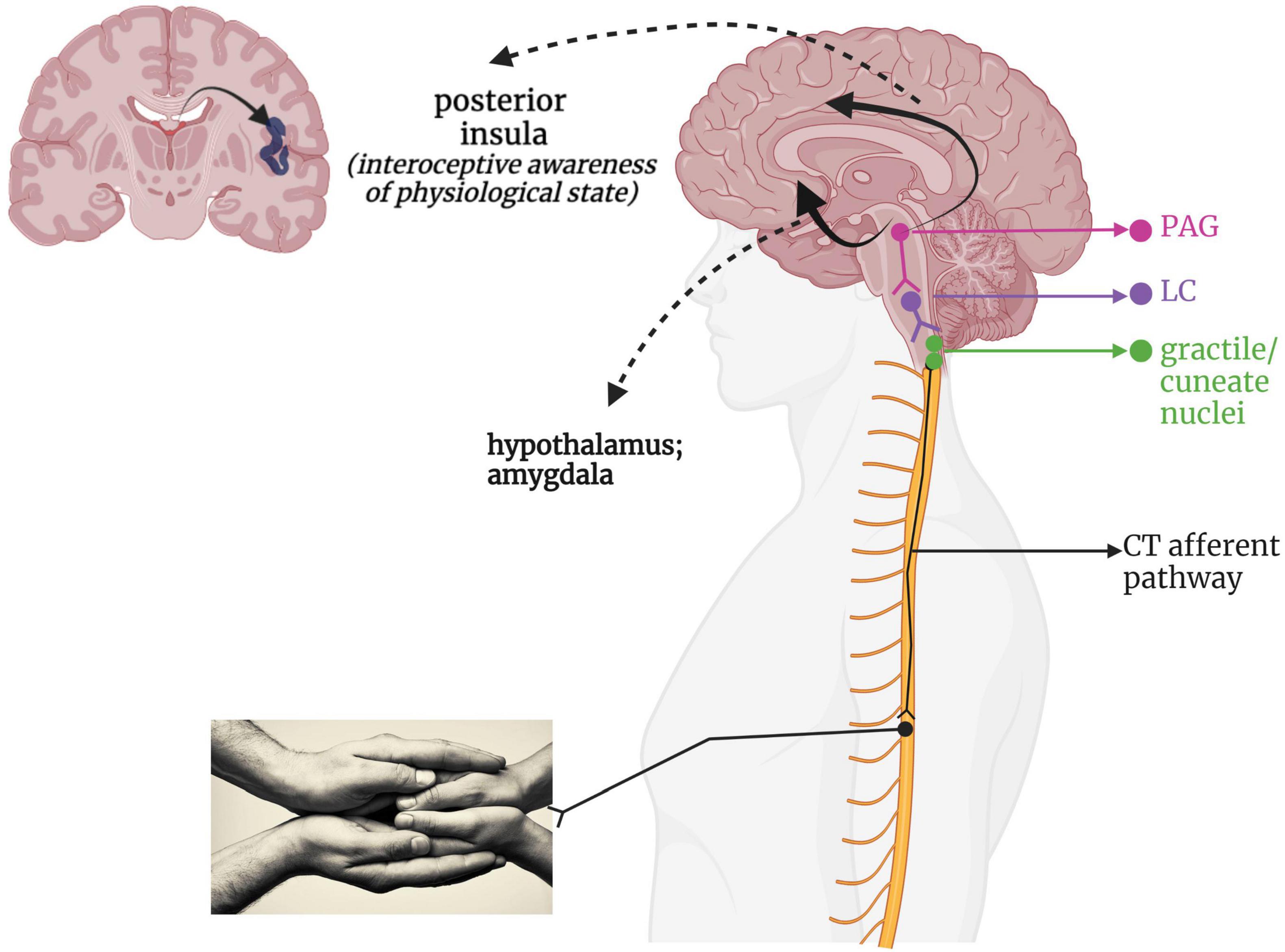
Figure 4. A putative CT-afferent pathway. Light stroking touch to the skin first arrives at the gracile and cuneate nuclei within the brainstem, which interacts with the LC within the RAS to influence arousal. This information flows onward to the midbrain PAG for determination of affective valence and survival relevance. This integrated information then is relayed via a ventral stream to the amygdala and hypothalamus for further emotional processing and endocrine responses, as well as a dorsal stream providing indirect connections to the cingulate cortex and posterior insula. LC, locus coeruleus; PAG, periaqueductal gray; PI, posterior insula; RAS, reticular activating system.
Multisensory integration: Phylogenetic and ontogenetic development
“Most full-grown trees have a remarkable canopy of branches and leaves that interact dynamically with the environment. However, the spreading branches cannot function or survive without the nourishment and support they receive from the roots and trunk. We may appreciate the tree for its spreading leaves, but our understanding must begin with the seed, the roots, and the emerging trunk.” (Panksepp, 1998, p. 302).
An optimal understanding of how trauma impacts somatic sensory processing requires a deeper appreciation of the evolutionary neurobiology of the mammalian brain. The spinal cord and brainstem are the first central nervous system structures to evolve in vertebrates and develop in the mammalian womb, and are centrally concerned with survival. Likewise, the vestibular and somatosensory systems are known to be the phylogenetically and ontogenetically oldest systems initially concerned with survival-related processes. These somatic systems are more foundational than primarily exteroceptive visual and auditory systems, which develop as the next sensory “layer” (Ayres, 1972; Roley et al., 2007). With mammalian evolution came the development of additional social-emotional circuitry to ensure offspring nurturance and social relationship maintenance for survival purposes. The development of limbic and neocortical structures afforded the ability to act upon the environment as opposed to passively respond to sensory stimuli, and initiate and maintain prosocial contact (Panksepp and Northoff, 2009). Piaget (1952) and Herrick (1956) postulated that the brain retained its older structures and reorganized them with each cortically directed evolutionary step, similar to the ontogenetic pattern of neurodevelopment. Maclean’s (1985) triune brain theory is in agreeance, suggesting the brain to be a phylogenetically layered system that began with the “reptilian” or subcortical brain. Upon the foundation of subcortical structures developed the limbic system, now nestled within the cortical midline and heavily involved in emotion and self-related processing (Panksepp and Northoff, 2009). The final neocortical layer engenders higher cognitive capacities and behavioral flexibility (Maclean, 1985). Despite general focus on the neocortex as our evolved and “smart” master control center, sub-cortical regions maintain a critical role in primitive survival functions and sensorimotor control. This pre-reflective, multisensory integrative region may be most impacted by threats to survival, giving rise to deficits in regulatory capacities and post-traumatic symptoms (Perry and Hambrick, 2008; Perry, 2019). A neurodevelopmental and phylogenetic framework contextualizes the disparity between which aspects of trauma can be articulated and which remain trapped or pre-reflectively experienced by the brainstem and body.
Phylogenetic development
Vestibular system
Even the most primitive of mobile organisms must recoil from noxious stimuli, maintain motoric control and orientation while moving about in the environment, and respond with either motion or defensive posturing to external threats. Vestibular function was established in ancient vertebrates and has remained largely unchanged from fish to humans, as opposed to auditory structures and networks which adapted to terrestrial life and mammalian dynamics (Fritzsch et al., 2014; Lipovsek and Wingate, 2018). The ipsilateral vestibulospinal and reticulospinal tracts are the most ancient motor tracts in the human body, dating back to a time before forebrain lateralization (Vulliemoz et al., 2005; Mora et al., 2019). The vestibulospinal pathway originates at the vestibular nuclei, controlling posture and balance while regulating muscle tone (Akaike, 1983; Sengul and Watson, 2015). For instance, the linear acceleration sensed while stumbling sends excitatory input down the vestibulospinal tract to engage the extensor musculature responsible for equilibrium responses and postural control, reflexively protecting the head and body from impact (Shinoda et al., 2006; McCall et al., 2017). The reticulospinal tract originates within the pontine and medullary reticular formation and supports anticipatory postural responses, regulation of muscle tone, and autonomic regulation (Sengul and Watson, 2015). Efficient reflexive responses of the body have evolved to maintain efficient movement through space, giving eventual rise to a sense of agency over purposeful bodily action.
Somatosensory system
The function of skin tissue is in a sense analogous to that of brain tissue in that both are designed to be an interface between the body and the outside world (Panksepp, 1998). Phylogenetically, the receipt of touch from another is a ubiquitous experience for both aquatic and terrestrial species. Fish that seek cleaning of ectoparasites and tactile stimulation from “cleaner fish” have lower cortisol levels as well as preserved motivation to visit a cleaner fish “replica” for tactile stimulation in and of itself, showing that tactile stimulation alone imparts physiological changes (Soares et al., 2011). A phylogenetically older, aquatic touch system may have been co-opted by social mammals in keeping with evolutionary continuity to preserve a tactile-mediated sense of safety and security (Schirmer et al., 2013). This corresponds with the unmyelinated, small-diameter axon morphology of CT-afferent tracts in humans, suggesting phylogenetic precociousness. Ubiquitously, tactile stimuli functions to relieve stress and enhance health in mammals and non-mammals alike (Caldji et al., 1998; Liu et al., 2000; Meaney, 2001; Raussi et al., 2003; Schmied et al., 2010; Soares et al., 2011) to the extent that evolution has provided specialized receptors and pathways. This suggests that tactile facilitation of physiological regulation and recovery from fear has roots in our evolutionary beginnings, and thus has implications for how primal fear and survival-based circuitry may be altered by trauma.
Ontogenetic development
Vestibular system
The vestibular system is the first sensory system to begin development in utero (Sidebotham, 1988; Blayney, 1997), and the morphology of the vestibular apparatus is mature within the first month of gestation (Nandi and Luxon, 2008; Jamon, 2014). The vestibulocochlear nerve is the first cranial nerve to complete myelination and the system is fully developed by the eighth month of intrauterine life (Blayney, 1997; Nandi and Luxon, 2008). Although morphologically mature, developmental changes in vestibular connectivity with the cerebellum and cortex continue through early life as children move to learn and grow (Lane et al., 2019). Initially, the fetus detects the mother’s movement in the womb, with movement becoming less externally controlled and more self-initiated with development. The neurodevelopmentally healthy child has a strong instinctual drive to master bodily control against gravity (von Hofsten, 2007), from the repetitive battles for head control in infancy through the joyful tests of balance on the playground in early childhood. This increase in bodily control against gravity develops in tandem with the child’s sense of agency, or sense that they are in control of their body as an object capable of intentional action. We suggest that the detection of gravity provides an anchor for affective-somatic instinctual happenings, an idea informed by the ontogenetic primacy given to the vestibular system’s development in conjunction with its widespread connectivity within the central nervous system.
Somatosensory system
The source of both the skin and the nervous system is the embryonic ectoderm, which differentiates into the surface ectoderm and neuroectoderm, respectively (Montonen et al., 1998; Bond et al., 2012). Fetal somatosensory receptor density is exuberant compared to that of an adult (Ashwell and Waite, 2012), suggesting an essential role in driving neurodevelopment. The fetus responds to touch as early as 8 weeks (Lecanuet and Schaal, 2002; Hadders-Algra, 2007) and elicits self-initiated movements by 12 weeks (Kurjak et al., 2008). The fetal somatosensory system is directly and frequently stimulated by movement of the amniotic fluid over fetal lanugo hairs (Bystrova, 2009; Sasaki et al., 2013), as well as through self-touch (Piontelli, 2015) and self-motion within the confined space of the womb (Marx and Nagy, 2015). These sensory-motor interactions occur well before the infant is born to interact with the outside world. The experience of pleasantness from affective touch has been linked with improved autonomic regulation in newborn humans and animals (Morrison, 2016; van Puyvelde et al., 2019; Farroni et al., 2022), as young children need to be touched and swaddled to co-regulate their emotional worlds. While breastfeeding duration has been associated with improved cognitive development in children even when controlling for socioeconomic position (Pereyra-Elías et al., 2022), the degree that somatosensory input coupled with oxytocin release plays a role is indeterminate yet intriguing. Lastly, affective tactile interactions create a perceptual and physical boundary between self and other within the first months of extrauterine life and beyond (Farroni et al., 2022).
Somatic sensory contributions to secure and insecure attachment
Attachment theory (Bowlby, 1973) posits that humans have an inborn neurobiological system in place that seeks proximity and care from an attachment figure in times of physical and emotional need, resulting in an ingrained sense of security, safety, and acceptance (Schore, 2003; Mikulincer et al., 2015). Inherently, the development of secure attachment bonds with a primary caregiver requires soothing and comforting sensory input. Somatic “co-embodiment” with the mother forms the basis for an emerging sense of self-embodiment in the young child (Ciaunica et al., 2021a). During uterine development, the fetus experiences co-embodiment on a sensory level in that the fetus’ physical and regulatory developmental needs are dependent upon the mother and intrauterine sensory experiences during prenatal development (Ponzo et al., 2018; Ciaunica et al., 2021a). In a secure attachment relationship, an infant continues to be co-regulated post-natally by an attuned caregiver through somatic sensory experiences such as rocking, swaddling and bodily contact. The caregiver intuitively problem solves which rhythmical patterns of movement and touch will calm their child’s distress and instinctually regulates their child’s physiological state and emotional world until they can self-soothe. Here, somatic sensory input is embedded in the manipulation and regulation of arousal (Balaban and Porter, 1998; Yates and Miller, 1998; Manzotti et al., 2019) given its connections with and integration at the RAS. Indeed, increased frequency of linear and rhythmical rocking attenuates arousal in infants (Vrugt and Pederson, 1973). This caregiver-scaffolded regulation of the infant, termed co-regulation or co-homeostasis (Ciaunica et al., 2021a), not only assists the infant in the moment, but also creates a secure, reliable, and safe attachment bond. It is through this physical and physiological attunement that children sense that they are protected, cherished, and safe. Speculatively, this foundation solidifies the child’s connection to the earth and confidence to emerge from the co-regulated bond, eager to explore as a separate and capable organism worthy of the social connection crucial for survival and reproduction later in life. In secure attachment, the higher reaches of the child’s limbic system and neocortex develop upon the foundation of an integrated and modulated subcortical layer (Figure 5A).
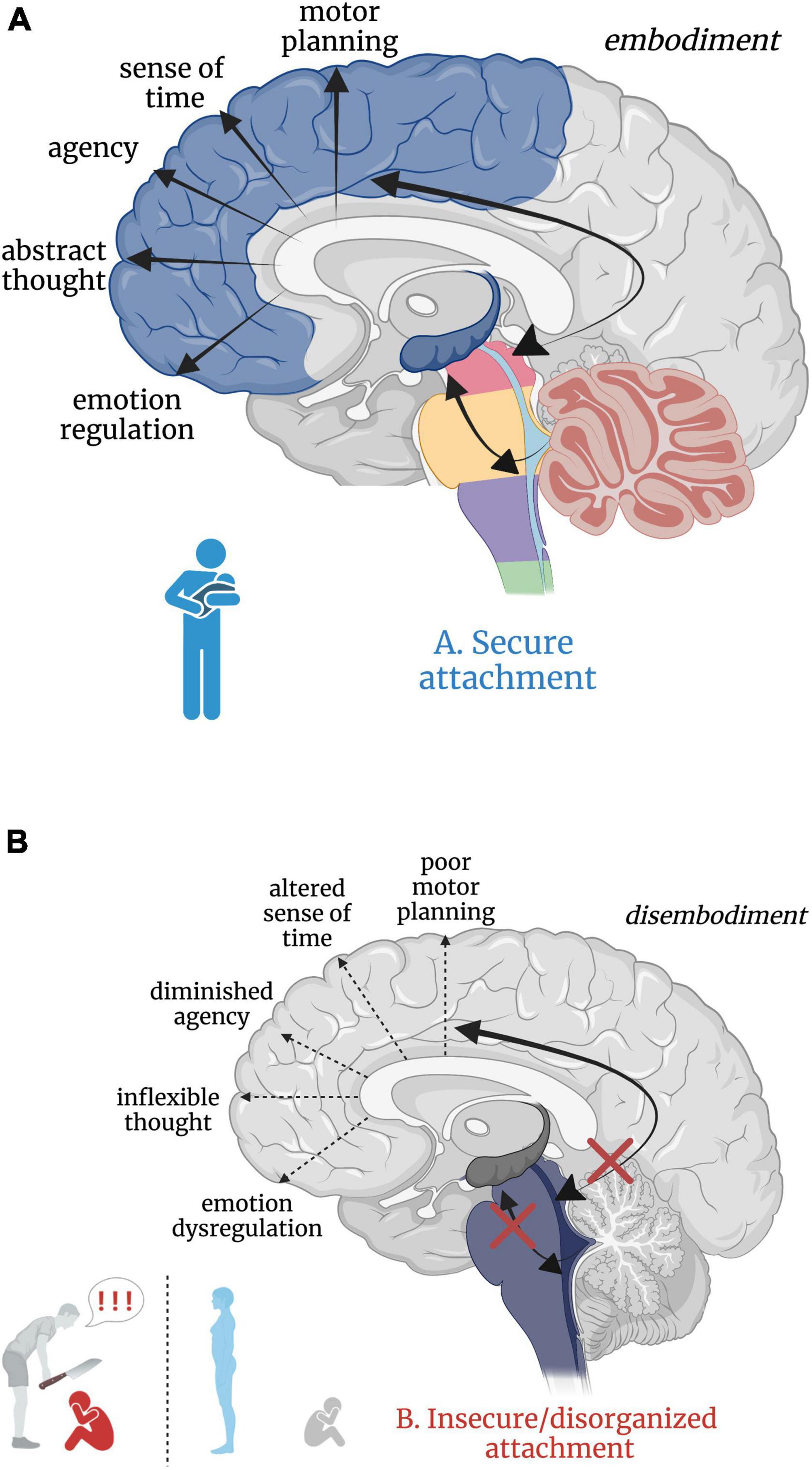
Figure 5. (A,B) Good versus poor somatic sensory integration during development with a secure versus insecure attachment figure, respectively. (A) Healthy brainstem level multisensory integration as a result of attuned, nurturing, somatic sensory-rich caregiving provides the foundation for higher-order limbic and cortical capacities. (B) An overwhelmed or malnourished brainstem due to abusive or neglectful caregiving. The child does not receive comforting touch or attuned rhythmical movement with a safe affective caregiver. Somatic sensory experiences are paired with unsafe or insecure attachment relationships, which has cascading aversive effects on higher-order limbic and cortical capacities.
Insecure/disorganized attachment results from early experiences with negatively valenced, absent, or inconsistent physical and/or emotional interpersonal interactions. Here, physical and emotional harm occurs through active abuse, withdrawal of physical comfort, or somatic sensory experience interacting with negative or withdrawn affect. Though there are distinct differences between anxious-insecure, avoidant-insecure, and disorganized attachment patterns, it is beyond the scope of this paper (instead see Bowlby, 1973; Ainsworth et al., 1978; Paetzold et al., 2015; Beeney et al., 2017) and thus we consider all non-secure attachment patterns here as ‘insecure’. Insecure attachment patterns with primary caregivers are posited to manifest as dissociative response patterns (i.e. freezing/tonic immobility) in adulthood (Ogawa et al., 1997; Schore, 2003; Lyons-Ruth et al., 2006), where emotional abuse or neglect is most predictive of dissociation (Sar et al., 2009). Those who experience early life abuse or neglect from a caregiver may develop dissociative response patterns as an adaptive means of escape from childhood stress and show reduced oxytocin levels in adulthood (Heim et al., 2009; Opacka-Juffry and Mohiyeddini, 2012; Bertsch et al., 2013), diminishing protection from stress and reducing propensity to form close relationships. However, the relationship between severity of early life trauma, genetics, and oxytocin has proved complicated, warranting further investigation (Chatzittofis et al., 2014; Myers et al., 2014; for a review, see Donadon et al., 2018). From a neurobiological perspective, subcortical sensory integrative regions crucial for higher limbic and cortical development are overwhelmed by alarming and distressing sensory input, starved for sensory-affective nourishment, or conflicted by the inconsistent provision and withdrawal of sensory-affective nourishment in abusive, neglectful, or disorganized caregiver relationships, respectively. We hypothesize that insecure attachment has both a somatic sensory component and a cascading effect on ontogenetic development of the higher reaches of the brain important for emotion regulation, motor planning, abstract thought, sense of time, agency, curiosity, and dynamic social relationships (Figure 5B). Though speculative, aberrant somatic sensory integration correlated with insecure attachment patterning may set the stage for later psychiatric dysfunction, providing insight into the high correspondence between early life traumatization with insecure attachment and the later development of psychopathology and/or PTSD (Roche et al., 1999; Besser and Neria, 2012; Mikulincer et al., 2015; Ogle et al., 2015; Woodhouse et al., 2015; for a review, see Marshall and Frazier, 2019).
Neurobiology of somatic sensory processing: Role of the brainstem and midbrain in arousal, defensive responding, and trauma-related symptomology
While subcortical brain regions integrate somatic and exteroceptive sensory information to create a coherent body representation, they are implicated in evolutionarily conserved threat detection and response circuitry (Darwin, 1872; Adamo, 2012; Tovote et al., 2016; for a review, see Terpou et al., 2019b) as well as in instinctual social interactions for mammalian survival. As the somatic sensory systems give rise to a general experience of the physical body while preserving its safety and viability, they are highly implicated in the body’s response to traumatic events. Alterations to muscle tonicity protect the body from harm, ranging from immobile rigidity to flaccidity to prevent detection from a predator or the experience of overwhelmingly noxious sensation, respectively. Somatosensory feedback from the peripheral somatosensory system contributes toward safety or threat detection, the latter resulting in the maintenance of a midbrain-mediated, survival-focused loop. We suggest that neurobiological pathways arising from the soma, as mediated by vestibular and somatosensory feedback, are principal in integrating incoming exteroceptive sensory stimulation (related to the external environment) and interoceptive stimulation (related to the viscera and internal physiological state) with contextual information regarding safety or potential danger at the level of the midbrain. The direct connections these somatic sensory systems have with brainstem and mesencephalic regions influence a subconscious maintenance of homeostasis and a felt sense of relational security with our surroundings and with others.
Arousal
Where traumatization is strongly characterized by incapacitated arousal modulation (Pitman et al., 1987; van der Kolk, 1987; Bryant et al., 2000; McMillen et al., 2000; Bryant, 2003; Risser et al., 2006; for a review, see Pole, 2007; Pitman et al., 2012), the sensory-motor contributions toward arousal are crucial to consider. Arousal level is directly influenced by sensory stimuli, and responses to sensory stimuli are influenced by arousal, suggesting an inextricable and bidirectional relationship. The RAS, comprised of numerous nuclei, generates raw arousal and “alarm” mainly within the locus coeruleus (LC) and pedunculopontine nuclei (PPN) (Berridge and Waterhouse, 2003; Aston-Jones and Cohen, 2005; Horn and Adamzyk, 2012; Pfaff et al., 2012) and has reciprocal interactions with the brainstem vestibular nuclei (Yules et al., 1966; Pompeiano et al., 1984; Manzoni et al., 1989; de Cicco et al., 2018) and somatosensory dorsal column (gracile and cuneate) nuclei (Guzmán-Flores et al., 1962; Odutola, 1977). The appropriate level of attention and alertness required for higher order emotional and cognitive processes is then mediated by the thalamus (LaBerge et al., 1992; Coull, 1997; Portas et al., 1998).
Both hyper- and hypoarousal are common phenomena in traumatized individuals. Hyperarousal results in heightened sensory and emotional reactivity (Pfaff et al., 2012), and falls within the DSM-V diagnostic criteria for PTSD (American Psychiatric Association [APA], 2013). Alternatively, decreased arousal may contribute toward the dampened emotionality and hypo-responsivity to stimuli evident in the dissociative subtype (Frewen and Lanius, 2006; Ludäscher et al., 2007; Sack et al., 2012; D’Andrea et al., 2013; Thome et al., 2019). Individuals with PTSD + DS exhibit altered resting-state functional connectivity (rsFC) between the PPN and the amygdala and ventromedial prefrontal cortex (vmPFC), and rsFC between the PPN and anterior thalamus correlates negatively with derealization/depersonalization symptoms (Thome et al., 2019). While heightened RAS-amygdala and RAS-vmPFC rsFC suggests enhanced threat processing (Liddell et al., 2005; Williams et al., 2006; Lanius et al., 2017), a negative relationship between RAS-thalamus rsFC and dissociative symptomology may indicate disrupted transmission of multimodal sensory information to the cortex. When the latter result is coalesced with findings of diminished vestibular nuclei rsFC with cortical regions in PTSD + DS (Harricharan et al., 2017), we converge on a hypothesis that dissociative symptomology is related to cortical-somatic sensory deafferentation, resulting in hypo-arousal and altered perceptual awareness of the body and its surroundings. Stress or trauma recall-induced dissociation has been linked to blunted psychophysiological arousal responses in traumatized individuals with high dissociative symptoms (Griffin et al., 1997; Koopman et al., 2004; Sack et al., 2012; D’Andrea et al., 2013; for a review, see Boulet et al., 2022), as well as in individuals with psychiatric conditions highly associated with severe early life traumatization including dissociative disorder (DD) (Schäflein et al., 2018), borderline personality disorder (BPD) (Ebner-Priemer et al., 2005; Bichescu-Burian et al., 2018) and dissociative identity disorder (DID) (Williams et al., 2003; see Putnam et al., 1986 for a review). Tendencies toward hyper-arousal and blunted arousal states in PTSD and PTSD + DS, respectively, point toward a psychophysiological manifestation of PTSD symptomology and different patterns of arousal-related neural circuitry in non-dissociative and dissociative trauma-related disorders. Importantly, a blend of intermittent hyper- and hypo-arousal may exist in traumatized individuals, indicative of broadly disrupted arousal modulation patterns that may be difficult to capture in statistical research methodology which centers or averages physiological or self-reported data on arousal.
Arousal can be attenuated through rhythmical linear vestibular stimulation (Vrugt and Pederson, 1973; Pederson, 1975; Lubeck et al., 2020; for a review, see Sandler and Coren, 1981), deep touch pressure (Diego and Field, 2009; Reynolds et al., 2015), and light touch (López-Solà et al., 2019; Reddan et al., 2020) within the context of safety. Individuals seek linear somatic sensory input in the form of walking, cycling, and running to calm their “nerves” or racing thoughts, and touch from a loved one to alleviate distress or improve mood. Conversely, sudden acceleration, angular motion, itching, light crawling touch from a bug, and pain will increase arousal to direct our attention to a potentially precarious or threatening bodily situation. Foundationally, somatic sensory input is primarily concerned with our survival and safety given the potential repercussions of falling on our skull, enduring a poisonous spider bite, or disconnecting from a primary caregiver or protective adult relationship, lending to strong connections with arousal centers. In a suboptimal arousal state, we cannot function or sleep properly, undermining higher-order capacities such as learning, memory, and social cognition (Siegel, 2001).
Muscle tone, posture, and orientation to threat/connection
An optimal baseline level of muscle tonicity, innervated by the vestibulospinal and reticulospinal tracts, allows for adaptive motor actions and responses. Increased muscle tension occurs during states of high stress or fear to prepare the physical body for fighting or fleeing (Figure 6). If an animal carries out a survival-oriented action to completion, the system receives vestibular and somatosensory feedback. A thwarted or incomplete motor response to a threatening situation may explain how traumatized individuals often experience their body reflexively enters exaggerated reactive motor patterns and/or defensive states such as tonic immobility or collapse in the face of everyday stressors or trauma triggers. Exaggeratedly heightened tonicity and decreased postural sway create a tonic immobility or “freeze” response, exhibited by animals (Webster et al., 1981; Fleischmann and Urca, 1988; Porro and Carli, 1988) and humans (Suarez and Gallup, 1979; Heidt et al., 2005; Marx et al., 2008) who experience imminent threat (for a review, see Terpou et al., 2019b). This response is mediated by somatosensory and vestibular feedback (Klemm, 1971; Gallup, 1977; Fleischmann and Urca, 1988), and is hypothesized to deter predators who are wired to detect motion in their prey and enable the animal to monitor its environment and flee if necessary (Kozlowska et al., 2015). Tonic immobility has been reported by study participants with PTSD under traumatic memory recall (Volchan et al., 2011; Fragkaki et al., 2016; de Kleine et al., 2018), suggesting that traumatic memories remain connected to subconscious postural responses. Tonic immobility can be elicited in animals using species-specific forms of repetitive tactile or vestibular stimulation, including inversion and somatosensory restraint (Klemm, 1971; Webster et al., 1981; Fleischmann and Urca, 1988; Porro and Carli, 1988; for a review, see Kozlowska et al., 2015). Likewise, tonic immobility in humans is more common under fear-inducing conditions of somatosensory restraint, such as rape (Suarez and Gallup, 1979; Heidt et al., 2005; Marx et al., 2008). Alternatively, hypo-arousal and decreased muscle tone occur during emotional shut-down or feign death as a passive defensive response to inescapable or prolonged threat (Hofer, 1970; Depaulis et al., 1994; Bracha, 2004). Here, drastically reduced arousal and tonicity diminish the conscious experience of further injury and psychic distress during inevitable or prolonged attack, sometimes to the point of an out-of-body experience or complete loss of consciousness (Figure 7). These passive responses are likely when traumatization is chronic, or during childhood where the victim is ill-equipped for self-defense and the perceived potential of escape is low (Nijenhuis et al., 1998b; van der Kolk, 2003; Schauer and Elbert, 2010; Krammer et al., 2016; Kratzer et al., 2018; for a review, see Foa and Hearst-Ikeda, 1996). Accordingly, those with trauma-related dissociative disorders are thought to exhibit top-down overmodulation of subcortical brain activity (Lanius et al., 2010b; Nicholson et al., 2017; Terpou et al., 2020), which putatively suppresses somatic sensory information from reaching higher-order regions involved in its integration into awareness.
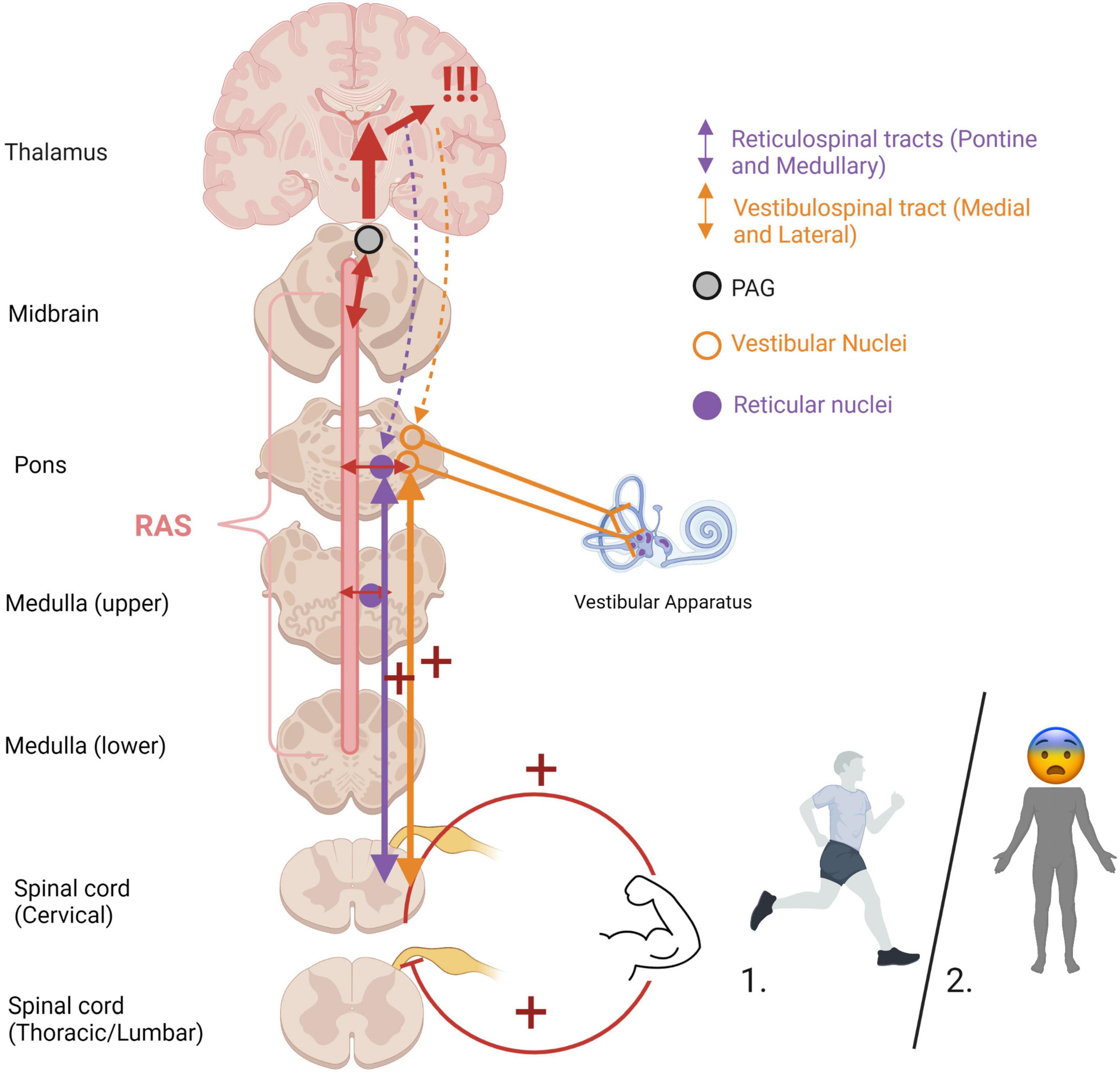
Figure 6. Heightened muscle tonicity during fight/flight (1) and freeze/tonic immobility (2) responses. Overwhelm of subcortical arousal-affective circuitry (RAS and PAG) during traumatic stress cues heightened muscle tonicity, mediated by the vestibular and somatosensory systems. If the body carries out an active survival response, the system receives vestibular and somatosensory feedback from muscle engagement and bodily action. In situations of imminent danger, tonic immobility renders the body rigid, an evolutionarily primitive response to avoid predatory detection. Cortical structures are overaroused, and top-down modulatory influences are weakened (indicated by dashed lines), allowing for primitive brainstem and midbrain regions to dictate defensive responding and heightened muscle tonicity. If the body is prevented from carrying out defensive motor actions (i.e., fighting off, pushing away, running away), it may become pre-disposed to or “stuck” in this defensive posture post-traumatically. RAS, reticular activating system; PAG, periaqueductal gray.
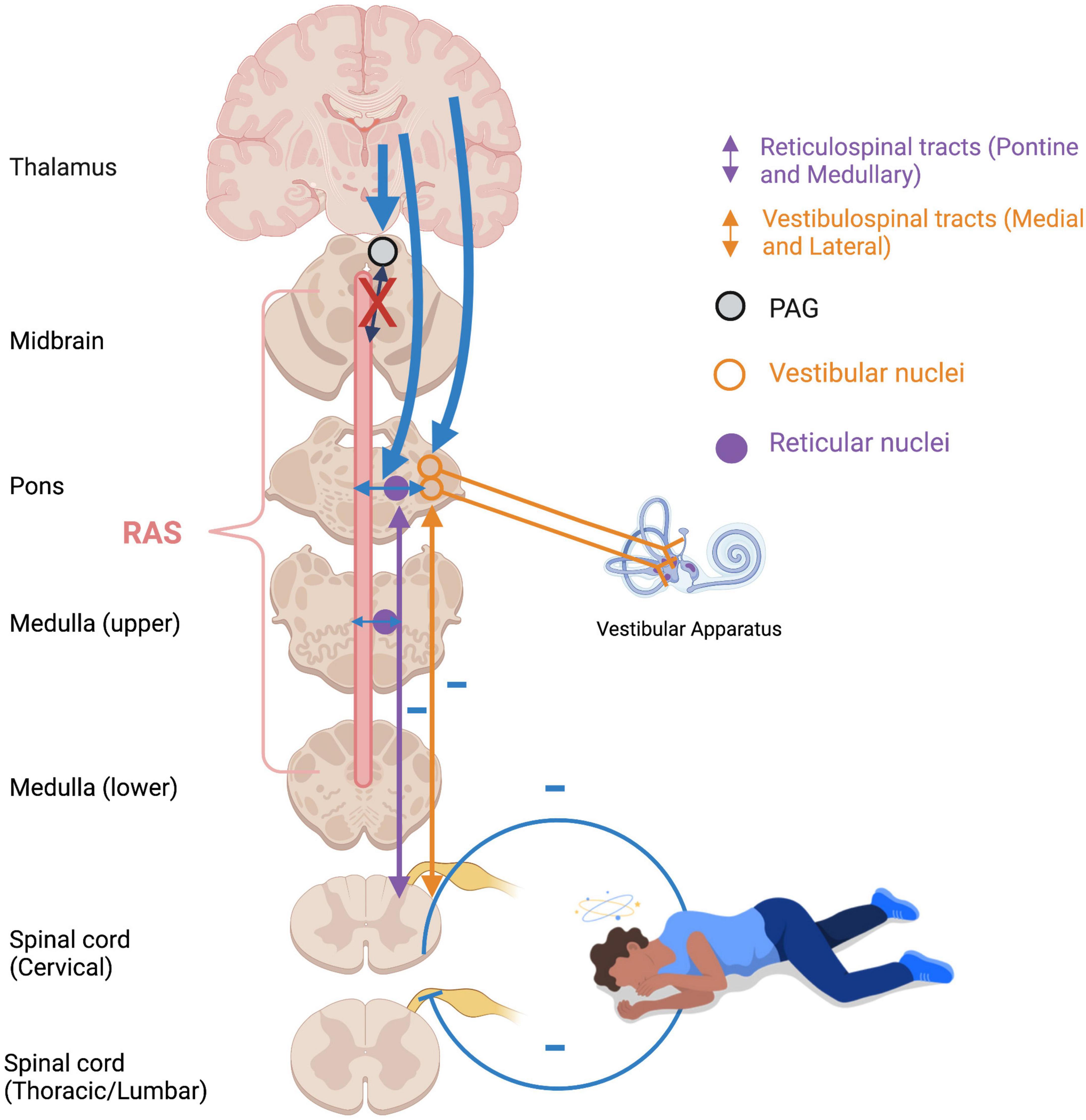
Figure 7. Flaccidity of muscle tone during emotional shutdown/feign death/dissociative responses. Over-modulation of subcortical activation by higher structures (thick blue arrows) as an adaptive defensive response to repeated traumatic stress results in diminished arousal and weakened vestibular efferents to extensor musculature. Feedback from deafferented, flaccid muscles creates a somatosensory-vestibular feedback loop which subconsciously maintains a collapsed, dissociated posture and hypo-aroused state. RAS: reticular activating system; PAG: periaqueductal gray.
The superior colliculi (SC) of the midbrain tectum (mesencephalon) are powerful multisensory integrative centers containing layered topographical maps of the visual, auditory, and somatosensory systems (Meredith et al., 1992; Cuppini et al., 2018; Basso et al., 2021). The SC are best known for their involvement in subconscious orienting responses to salient visual stimuli (Dean et al., 1989; Grantyn et al., 2005; May, 2006; Horn and Adamzyk, 2012). They send afferents to oculomotor neurons, the pontine and medullary reticular formation, and cervical and upper thoracic motor neurons to orient the eyes, head and neck toward or away from stimuli (May and Porter, 1992; Scudder et al., 1996; May, 2006). Importantly, the SC receive ascending somatosensory feedback from the spinotectal (spinomesencephalic) tract (refer back to Figure 3) and have reciprocal connections with the vestibular nuclei innervating head and neck musculature (Rubelowski et al., 2013; Leong et al., 2019). Vestibular and proprioceptive inputs to the SC are required to appropriately gauge the force and speed required for a rapid yet ergonomically sound orienting response. Pertinently, this orienting response is geared toward instinctually relevant sensory stimuli, including threatening and nurturing interpersonal contact (Dean et al., 1989), playing a major role in approach and defense/withdrawal responses in early attachment and traumatic interpersonal dynamics (Corrigan and Christie-Sands, 2020). Aberrant rsFC of the SC has been shown in PTSD and PTSD + DS, suggesting different patterns of defensive responding in dissociative individuals (Olivé et al., 2018).
Taken together, heavy involvement of somatic sensory processing is likely in defensive posturing and motoric responses to traumatic events. Post-traumatically, the somatic sensory systems may prioritize their role of self-protection and defense, superseding their roles in orienting toward social contacts and maintaining a coherent multisensory experience. Further, primal defensive responses may remain online as a person feels “stuck” between an activated (or hypo-activated) autonomic response and the completion of self-protective action (Ogden et al., 2006). When the body is unable to self-protect (or protect another), the individual’s sense of agency is attacked and self-trust falters. When the execution of movement to self- or other-protect is disrupted, states of psychic anger or rumination may be disconnected from the body, or bodily activation may be disconnected from awareness (Corrigan and Christie-Sands, 2020). This disrupted subcortico-cortical information flow engenders pervasive senses of defectiveness and defenselessness, and may contribute to unintegrated traumatic memory manifesting at somatic or affective levels (Janet, 1889; Nijenhuis et al., 1998b).
The periaqueductal gray, affect, and defensive responses
The periaqueductal gray (PAG), a region of gray matter surrounding the cerebral aqueduct of the midbrain, acts as both a sensory relay and a sensory-affective integrative center that coordinates subcortical defense or approach behaviors in light of contextual information (Bandler and Depaulis, 1991; De Oca et al., 1998; Panksepp, 1998; Carrive and Morgan, 2012; for a review, see Kozlowska et al., 2015). The dorsal PAG plays a key role in fight/flight defensive responses (Brandão and Lovick, 2019), while the lateral/ventrolateral PAG is indicated in passive defensive responding including tonic immobility and emotional shutdown/collapse (Keay and Bandler, 2001; Lovick and Bandler, 2005; Koutsikou et al., 2014) as well as affective touch-mediated social behaviors in mice (Yu et al., 2022). The PAG receives sensory information from the pontine and medullary reticular formation, superior colliculus, and inferior colliculus among other brainstem regions, and integrates this with positive or negative affective valence from descending limbic inputs from the amygdala, hypothalamus, insula, and posterior cingulate (Carrive and Morgan, 2012). Affect laden sensory information then projects to cortical structures via the thalamus and hypothalamus (Damasio, 1998; Buck, 1999; Northoff et al., 2006; Hurley et al., 2010; Carrive and Morgan, 2012; Koelsch et al., 2015). Importantly, the PAG relays information to the insula and anterior cingulate cortex, giving rise to the salience network (SN) (see section “PAG and the salience network”) and contributing toward interoceptive and emotional awareness (Craig, 2003, 2009; Critchley et al., 2004). Top-down projections to the PAG serve to modulate arousal after contextual appraisal and maintain homeostasis (Critchley et al., 2004; Wiens, 2005; Barrett and Simmons, 2015). In particular, the amygdala participates in conditioned fear responses via a descending amygdalo-hypothalamo-PAG circuit, which Panksepp (2000) defined as the FEAR system, one of the basic raw affective systems engendered at the PAG. Top-down influences from the hypothalamus may also be involved in the conditioning of positive emotional experiences such as nurturance (Panksepp and Trevarthen, 2009; Yu et al., 2022). Overall, the PAG elaborates incoming sensory stimuli with affect to ascertain whether an environment or another animal is safe or threatening. For instance, light touch from a romantic partner at home will elicit a very different physiological and emotional response than light touch while walking alone in a dark alley. Likewise, a previous experience of assault in a dark alley will render the context of an alleyway or a grab to the arm sufficient to activate amygdalo-hypothalamo-PAG FEAR circuitry. Taken together, sensitization may occur where a stimulus or constellation of stimuli experienced during a previous event re-invokes a somatic and emotional response, facilitating either defensive or palliative, pro-social behavior (Anagnostaras and Robinson, 1996; Yehuda et al., 1998; Milligan-Saville et al., 2017; for a review, see Perry et al., 1995).
Periaqueductal gray and the salience network
The PAG is a node of the salience network (SN), an intrinsic connectivity network that activates when presented with unexpected, intense, or instinctually relevant sensory or emotional input (Menon, 2015). The SN is activated when lower-level sensory input meets a threshold for orienting our attention. The SN also plays a role in adaptive switching between the central executive network (CEN), involved in focused attentional states, and the default mode network (DMN), active during introspective self-referential thought and social emotional processes (Amodio and Frith, 2006; Sridharan et al., 2008; Menon and Uddin, 2010; Spreng and Grady, 2010; Menon, 2015). Individuals with PTSD have shown hyperactivation and hyperconnectivity of the SN (Thome et al., 2014; Abdallah et al., 2019; for a review, see Akiki et al., 2017), as well as diminished coherence across the DMN (Bluhm et al., 2009; Lanius et al., 2010a; Sripada et al., 2012; for a review, see Akiki et al., 2017; Lanius et al., 2020). Hypothetically, any form of salient sensory stimulation including slight movements, loud noise, or unexpected touch may trigger a heightened arousal response within a quick PAG-mediated defense mechanism short-circuited from receiving higher-order contextualization. Alternatively, those with dissociative symptomology may experience top-down over-modulation of the PAG as a defense mechanism, resulting in hypo-responsivity to salient sensory input and emotional numbness. Individuals with PTSD have shown hyperactivation of the PAG in response to direct eye-contact (Steuwe et al., 2014) as well as to subliminal threat stimuli (Terpou et al., 2019a). Individuals with PTSD show increased rsFC of the PAG with the anterior insula and anterior cingulate, major nodes of the SN (Harricharan et al., 2016). Alternatively, individuals with PTSD + DS exhibited increased v/l PAG functional connectivity with OP2 and TPJ, primary vestibular-multisensory processing regions (Ibitoye et al., 2022) associated with depersonalization symptoms (Lanius et al., 2005; Harricharan et al., 2017).
Trauma: An assault on the senses
Where a traumatic event poses a perceptual threat to one’s own bodily safety, or that of another, while eliciting feelings of extreme fear, helplessness, or horror (American Psychiatric Association [APA], 2013), it can also be conceptualized as a negatively valenced multisensory experience- an assault on the senses. Any form of traumatic event is a strongly arousing and affective multisensory experience, thus impacting the lower reaches of the brain. For example, a combat soldier experiences searing heat on the skin, a grotesque scene displayed on the retina, and gut-wrenching interoceptive sensations when a comrade is unable to be saved; a rape victim experiences aversive touch, imposed bodily movement and somatosensory restraint; a child beaten by a caregiver feels bodily pain, sees an expression of rage on their caregiver’s face, and senses a racing heart prevented from fleeing. In the case of chronic emotional neglect or abandonment in childhood, the threat on survival exists in the void of predictable, developmentally crucial sensory experiences such as synchronous movement, touch, and warmth. Taken together, brainstem-level multisensory integrative processes, typically grounding our experience in the present environment and physiological state of the body, interact with raw affect at the PAG to create an overwhelming, aversive experience. Whether a highly stressful event can be adaptively managed or modulated may depend upon the individual’s history of multisensory-affectively bound attachment experiences. Given that extreme stress activates an individual’s innate attachment system (Bowlby, 1973), an inability to seek co-regulation from another or engage neural circuitry related to self-regulatory mechanisms due to a history of insecure attachment and somatic sensory disintegration results in persisting trauma-related symptoms. In more extreme cases where the individual experiences tonic immobility or emotional shutdown, a sensory feedback-motor action plan for survival is thwarted and intention to obtain survival needs is decoupled from expected or intended action. This sensory-motor mismatch is experienced by the body as an inadequate response to existential threat (Fischer and Riedesser, 1999; Sar and Öztürk, 2008), and corresponds with a disrupted sense of agency and connectedness to/trust in the body.
Secure/insecure attachment as a buffer from/predisposition toward trauma-related conditions
Solidity in subcortical somatic sensory integration from a secure attachment history may act as a buffer against long-term ramifications of trauma. An individual with a history of secure attachment during critical developmental periods is better equipped to tolerate sensory and affective stress through fluid bottom-up and top-down integrative information processing and return to a solid baseline functioning post-stressor (Dieperink et al., 2001; Mikulincer et al., 2015). However, destabilized somatic sensory processing coupled with an insecure attachment history may render the individual vulnerable to stress and aberrant neural re-wiring in the wake of trauma. Further, traumatic experiences may exacerbate attachment-related insecurities and tendencies toward defensive (as opposed to connection-seeking) responding (Alexander, 1993; Muller and Rosenkranz, 2009; Mikulincer et al., 2015). Individuals with insecure attachment styles exhibit increased severity of psychiatric and PTSD symptoms (Mikulincer et al., 1993, 1999; Solomon et al., 1998; Dieperink et al., 2001; Zakin et al., 2003; Declercq and Willemsen, 2006; Fraley et al., 2006; Ghafoori et al., 2008; Bogaerts et al., 2009; Midolo et al., 2020), and attachment style has shown to be a mediating (Roche et al., 1999; Shapiro and Levendosky, 1999; Twaite and Rodriguez-Srednicki, 2004; Sandberg et al., 2010) and moderating (Sandberg, 2010; Scott and Babcock, 2010) variable between childhood exposure to trauma and psychiatric distress. The repeated inability to fight, flee, or receive physical and emotional safety during critical neurodevelopmental periods leaves hyperactivated brainstem and midbrain regions with no regulatory outlet, leading to cascading effects on higher-order capacities and a vulnerability toward the development of trauma-related dysfunction (Figure 8).
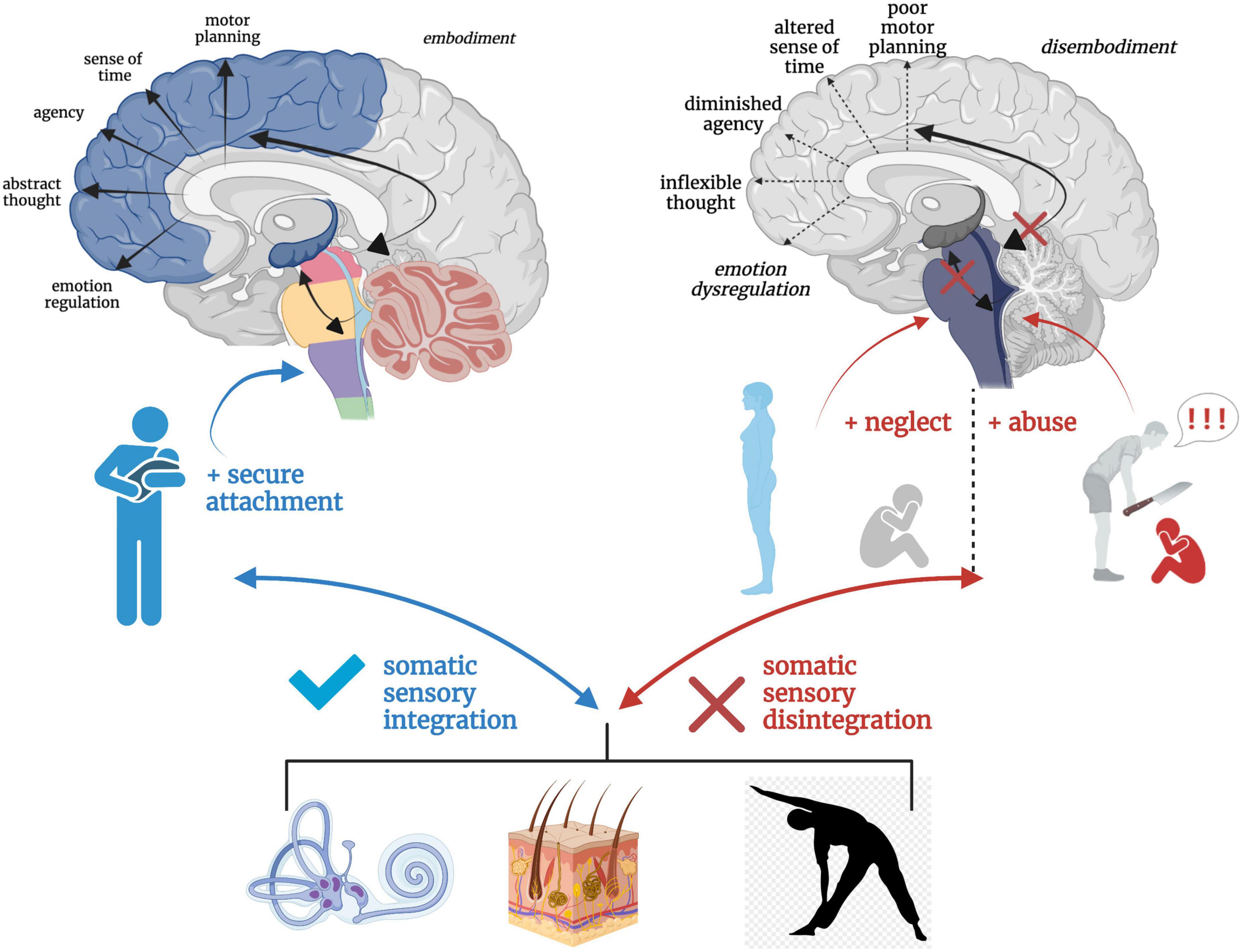
Figure 8. A hierarchical schematic of how somatic sensory systems, mediated by early attachment patterning, provide a foundation for vertical and horizontal integration in the brain. Vestibular, tactile and proprioceptive input drive healthy neurodevelopmental processes and the development of emotion regulation, motor planning, sense of time, agency, and a sense of embodiment when scaffolded by an attuned, secure attachment figure. However, a paucity or lack of safe and nourishing somatic sensory experiences interacting with contexts of abuse and/or neglect results in overwhelmed, fragmented and disintegrated brainstem-level sensory integration, which has cascading effects on higher order capacities of limbic and cortical brain regions.
On defense: Neural circuitry in trauma
When presented with sensory stimulation construed as threatening, less integration or “talking” between neocortical and subcortical regions may result in cortical and subcortical “loops” that take on a life of their own. A subcortical loop from the body to the midbrain and back again short-circuits the frontal regions for efficiency, priming the traumatized individual for rapid defensive responding (Lanius et al., 2017). Cortico-thalamo-cortical circuits may result in self-perpetuating cycles of rumination, obsessive thoughts, and a hollow sense of bodily self (Corrigan and Christie-Sands, 2020). Relatedly, those with trauma-based dissociative disorders may have adapted to chronic traumatization by suppressing lower-level survival responses and maintaining cortico-cortical hyperconnectivity, resulting in disembodiment and a lack of connection to raw emotion and sensorimotor experience. Indeed, upstream alterations in functional connectivity have been found at the level of the vestibular nuclei (Harricharan et al., 2017), PAG (Harricharan et al., 2016), superior colliculi (Olivé et al., 2018), RAS (Thome et al., 2019), and thalamus (Terpou et al., 2018) reflecting trauma’s widespread, cascading impact on vertical and thus horizontal connectivity in the brain.
Feeling too much or too little: Over- and under-modulation
A massively hyperactivated brainstem and a tremendous sense of fear lead to prolonged disruptions in physiological homeostasis and exaggerated defensive responses. In PTSD, cortical structures have difficulty modulating incoming sensory and emotional stimuli, contributing to chronic hyper-arousal (Lanius et al., 2010b). Without a grounded, stable, and physiologically regulated soma generated through somatic sensory integration, exteroceptive input such as bright lights, sudden noises, and unexpected touch can over-activate the SN, disturb the DMN, and throw multisensory integrative balance off kilter (Figure 9A). Here, one “feels too much” and lacks the ability to self-regulate resulting in aggression, emotional/physical overwhelm, and startle hyperresponsivity. Alternatively, chronic detachment from bodily sensations and emotions in conjunction with fluctuating hypoarousal presents in PTSD + DS. A vicious cycle of overwhelm and shutdown occurs in a desperate attempt to attenuate chronically overbearing feelings. Over-modulation of arousal and affect by prefrontal structures dampens sensory experiences as a protective mechanism, resulting in emotional numbness or feeling “dead inside” (Lanius et al., 2010b) (Figure 9B). Further, chronic childhood traumatization may alter development of SN and DMN circuitry, where highly activating or threatening sensory stimuli feel familiar or normal (Lanius et al., 2020). These individuals may seek to engage the SN and thus gain access to the DMN through intense or primally threatening somatic sensory experiences, such as car racing, extreme sports, and promiscuous sex (van der Kolk, 1989, 2015; Lanius et al., 2020). For severe and lifelong overmodulation, any experience of bodily sensation feels foreign, threatening to flood the cortical defense mechanism created to protect the brain from overwhelm. Those who are overcome by any experience of bodily sensation may avoid certain environments, physical activity, and intimacy which elicit too triggering of sensations. Consideration of pre-reflective sensorimotor brainstem regions in therapeutic attempts to restore, or in the case of chronic childhood trauma, primarily establish, the capacity for safety and trust in the body is crucial.
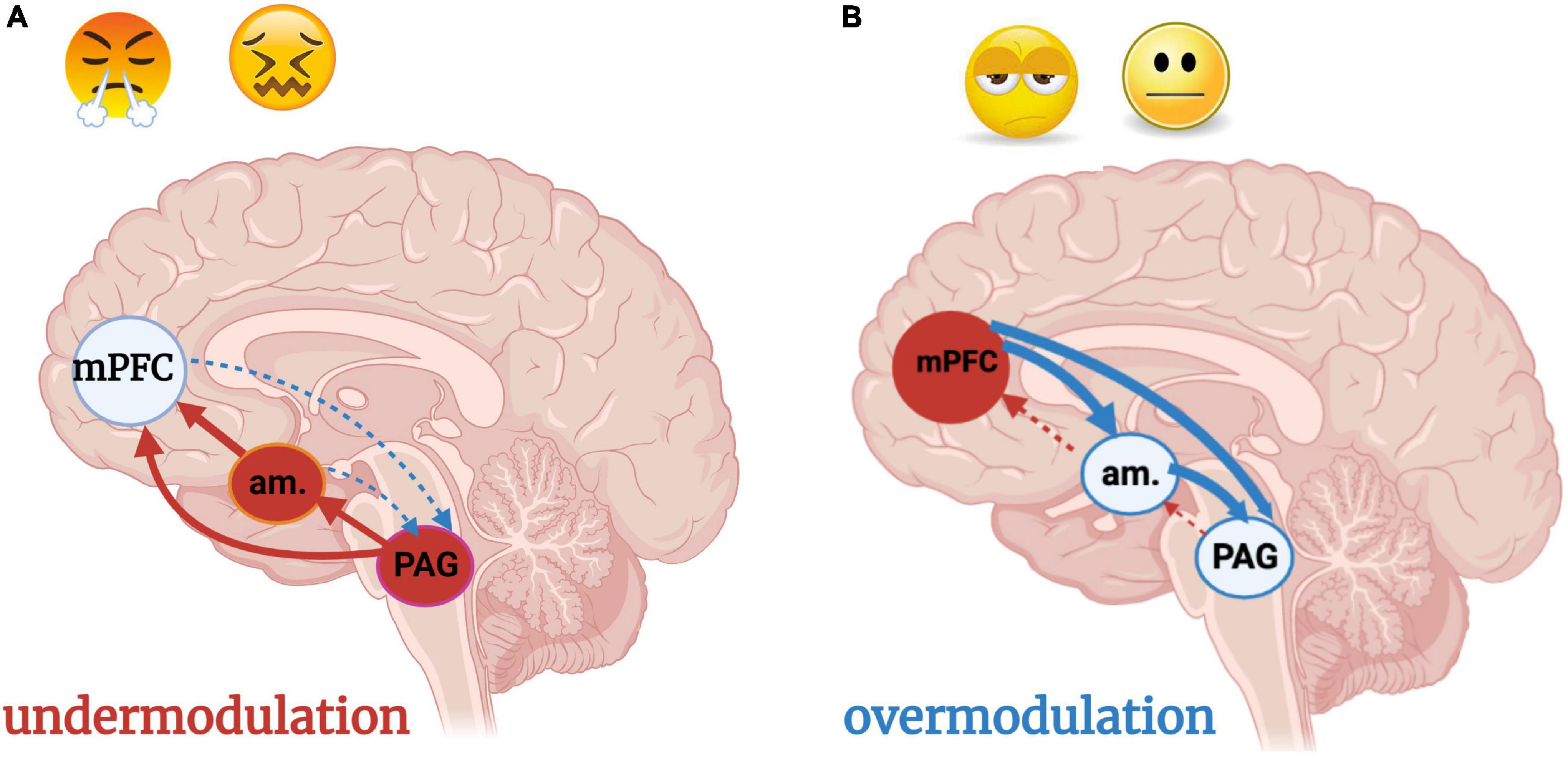
Figure 9. (A,B) Under- and over-modulation of emotion and arousal in trauma-related conditions. Image adapted with permission from Nicholson et al. (2017). An individual who encounters a traumatic situation is flooded with negatively valenced sensory stimuli, resulting in hyperactivation of the brainstem and midbrain PAG. An individual who develops a post-traumatic condition experiences persistent over-or under-modulated activity of the PAG and amygdala, resulting in hyper- and hypo- sensory-affective responsivity, respectively, at the level of the PAG. (A) Under-modulation or bottom-up predominance (solid arrows) results in hyperarousal and weak top-down neocortical modulation (dashed arrows) of sensory input and emotions. Situations incorporating sensory or emotional stimuli similar to the traumatic event, often over-generalized with regard to valence (i.e., every sensation of butterflies in the stomach is labeled as a sign of danger) triggers hyperarousal and a fight/flight response. (B) Over-modulation of lower brainstem and midbrain arousal and alarm centers (amygdala, PAG) is driven by frontal neocortical regions including the mPFC. An individual who experiences chronic, repeated traumatization, such as an adolescent who has grown up in a household with domestic violence and physical abuse, adapts to persistent threat through top-down blunting and avoidance. The adolescent is emotionally numb, withdrawn from relationships, and dissociative due to a scarcity of bottom-up sensory integrative influence and processing. Subcortical regions are prevented from nourishing the cortex with either negative or positive raw affective feelings or sensations unless they are extreme. am, amygdala; mPFC, medial prefrontal cortex; PAG: periaqueductal gray.
The sense of self in health and trauma through a sensory processing lens
The experience of inhabiting one’s own body, or embodiment, is an ineffable, subconscious perception rooted in sensorimotor processes (Ciaunica et al., 2021a). Embodiment is a state in which the mind is oriented in physical space, giving rise to a first-person perspective (Lenggenhager et al., 2006, 2007; Blanke and Metzinger, 2009). This spatial union of the mind with the physical body (Ferrè et al., 2014) provides a fundamental reference point for higher-order cortical function as it directs our attention and intentions in volitional thought and action (Panksepp, 1998). Ultimately, it provides us with an anchor to who we are, where we are, and what we want.
Somatic sensory contributions toward the sense of self
The somatic sensory systems are deemed foundational for the simplest or “minimal” self as it relates to physical world (Panksepp and Northoff, 2009; Metzinger, 2013). The infantile co-embodied self is relational to gravity and interpersonal touch, forming the basis for an agentive, separate self grounded in the physical body (what is happening to “me”- I am moving, I am being touched) (Fotopoulou and Tsakiris, 2017; Crucianelli and Filippetti, 2020; Ciaunica et al., 2021a). Bodily movement, which requires vestibular and somatosensory feedback, begets a sense of a bodily self (Ferrè and Haggard, 2015). This subcortical self-generating system is shared across species and forms the basis for higher forms of selfhood in humans (Panksepp and Northoff, 2009). In corroboration, removing the cortex of young mammals does not appear to compromise the sensorimotor coherence, basic autonomic processes, or raw affective responses required for a brainstem-level sense of self (Kolb and Tees, 1990; Panksepp et al., 1994; Shewmon et al., 1999; Panksepp, 2005; Merker, 2007). Further, manipulations of somatic sensory input in healthy adults have cascading effects on higher self-related constructs such as agency (Synofzik et al., 2008; Lopez et al., 2012; Lenggenhager and Lopez, 2015), embodiment (Schwabe and Blanke, 2008; Ferrè et al., 2014; Macauda et al., 2015; Lopez, 2016; Lopez et al., 2018), self-other distinction and first person perspective (Ferrè et al., 2014), and bodily self-awareness (Lenggenhager et al., 2006, 2012; Blanke, 2012).
Based on previous work by Panksepp and Northoff (2009) and Ferrè and Haggard (2016), we propose a hierarchical model depicting how somatic sensory information contributes toward primordial selfhood, and how this lays a foundation for higher forms of affective and embodied selves (Figure 10). Sensory integrative processes impact arousal and meet affect at the PAG. The PAG as a node of the SN allows for switching between salience detection and self-referential processing within the cortical midline structures (DMN) (Menon, 2015). These midline structures are involved in self-reflection, autobiographical memory processing and higher-order emotional processing and regulation (Northoff et al., 2006; Tsakiris, 2010). Henceforth, an abstract and subjective self arises from the intricacies of the lateral neocortex given its communication with both more medial and lower reaches of the brain. Without an integrated somatic sensory foundation, abstract knowledge about the body as a physical and emotional agent over which one has control is vulnerable to disruption (Longo et al., 2008, 2009; Ferrè and Haggard, 2015, 2016). Given the functional breakdown of arousal modulation, emotion regulation, sense of self, and SN/DMN connectivity evident in traumatized individuals, we find this model particularly relevant for how somatic sensory processing may contribute toward trauma-related symptomology.
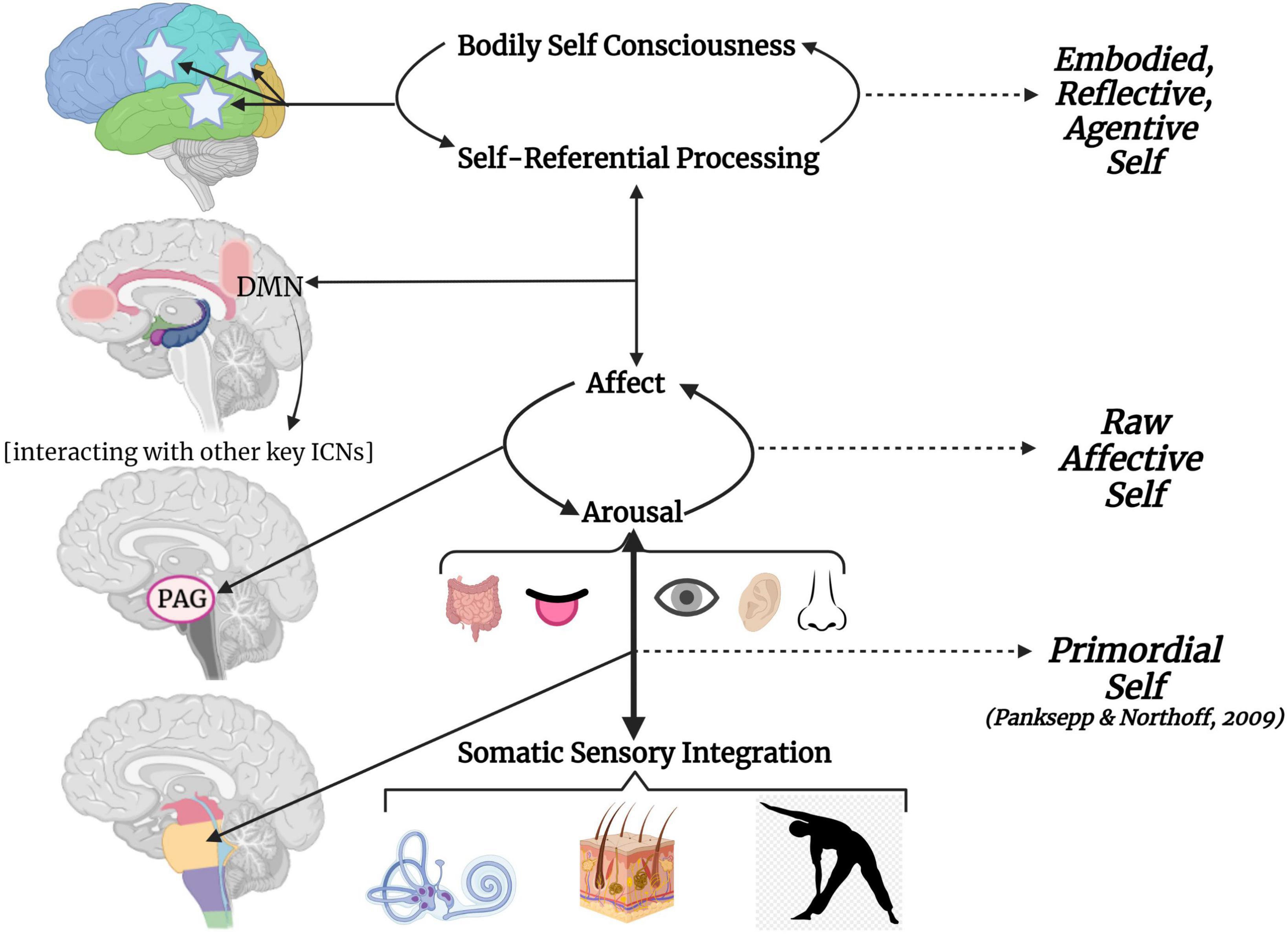
Figure 10. The embodied, reflective, agentive self grounded in somatic sensory integration. This hierarchical model visualizes how somatic sensory processing provides a foundation for exteroceptive and interoceptive sensory processing, influences arousal, mediates how arousal meets affect, and ultimately impacts higher-order self-referential processing and bodily self-consciousness. The left side of the graphic visualizes how neurodevelopmental vertical integration leads to horizontal integration of the cortex; the right side depicts hierarchical complexity of selfhood. Brainstem-level somatic sensory integration provides a subconscious sense of primordial self as it relates to the physical environment. Somatic sensory processing then mediates how arousal meets affect at the level of the midbrain PAG, giving rise to rudimentary awareness of a raw affective self. The PAG as a node of the SN allows for switching between salience detection and self-referential processing within the cortical midline structures (DMN). The DMN fluidly interacts with other key ICNs (SN, CEN) to influence behavioral states. Horizontal integration from cortical midline to lateral structures elaborate upon self-referential processing giving rise to an embodied, reflective, agentive sense of higher self that is anchored to the body. CEN, central executive network; DMN, default mode network; ICN, intrinsic connectivity network; PAG, periaqueductal gray; SN, salience network.
Trauma and the disembodied self
An ineffable disconnection from a sense of self is extensively reported in PTSD, such as “I am not myself,” and “I feel like an object, not a person” (Foa et al., 1999; Bluhm et al., 2009; Lanius et al., 2011, 2020). Experiences of deep emotion and perceptions of being terrestrially grounded, socially connected, and/or emotionally intuitive are often dulled or completely lost in trauma’s aftermath. Trauma engenders the sense that one’s body is not safe, is not under one’s own control, and/or is a cauldron of disgust and shame- particularly when it is chronic, interpersonal, and early in onset (Frewen and Lanius, 2015). Traumatogenic dissociative symptomology manifests as severe distortions in how the bodily self is perceived and experienced in relation to the outside world and often corresponds with trauma during critical periods of sensorimotor development (van der Hart et al., 2005; van der Kolk, 2005; Schmahl et al., 2010). Without adequate somatic sensory integration, we do not know where our body begins or where it ends; statements such as “I feel as if I am outside my body,” and “I feel like there is no boundary around my body,” highlight how alterations to the bodily self is a common experience in dissociative individuals (Frewen and Lanius, 2015; Lanius et al., 2020; Ciaunica et al., 2021b,c). Severe early life neurodevelopmental disruptions may also give rise to the experience of multiple fragmented, discontinuous, and disembodied selves, such as those experienced in dissociative identity disorder (DID), a condition highly co-existent with early and severe traumatization (Reinders et al., 2003; Dorahy et al., 2014; Schlumpf et al., 2014; Reinders and Veltman, 2021). These various forms of self-disconnection are often impenetrable by means of neocortically targeted therapeutic approaches. A return to the foundation of selfhood via somatic sensory-based therapeutic approaches is crucial to consider.
What neuroscience can teach us about connecting somatic sensory processing and trauma-related disorders: Treatment implications and future directions
“While we all want to move beyond trauma, the part of our brain that is devoted to ensuring our survival (deep below our rational brain) is not very good at denial” (van der Kolk, 2015, p. 2).
If somatic sensory input directly impacts arousal and gives rise to our primordial self, exploiting its power to modulate arousal and bind a coherent sense of self will be an effective therapeutic avenue. Encouraging re-connection with felt bodily experiences of movement and touch within a positively valenced therapeutic alliance will contradict previous negatively valenced multisensory experiences and attachment disruptions (van der Kolk, 2015), leading to upstream regulation of arousal and affect, modulation of exteroceptive sensory input, and embodied cognitive capacities. Although cognitive-behavioral therapies are considered a first line of treatment for traumatized individuals (Malejko et al., 2017; Yang et al., 2018), lower than 50% efficacy in PTSD patients has been reported (Marks et al., 1998; Bradley et al., 2005; Mendes et al., 2008; Kar, 2011), and they can be less beneficial during states of stress (van der Kolk and Fisler, 1995; Raio et al., 2013) or dissociation (Michelson et al., 1998; Rufer et al., 2006; Spitzer et al., 2007; Kleindienst et al., 2011; LeBois et al., 2022; but see also Halvorsen et al., 2014; Zoet et al., 2018; Vancappel et al., 2022). However, we do not suggest a purely “bottom-up” approach; alternatively, we highlight the importance of combining bottom-up with top-down strategies in consideration of brainstem level sensory integration, ontogenetic development, and the fostering of vertical and horizontal integration. The combination of somatic sensory stimulation with awareness, where the individual takes notice of the presence, intensity, and quality of somatic input, may bridge the brain-body disconnect that is often so difficult to address in cognitive therapies alone- thus, somatic sensory approaches may optimize cognitively-focused interventions. Sensory-based interventions are increasing in utility (Fraser et al., 2017; McGreevy and Boland, 2020) and several approaches are discussed in turn.
Somatic sensory-based psychotherapy
Sensorimotor approaches to psychotherapy, such as Sensorimotor Psychotherapy (SP; Ogden et al., 2006), Somatic Experiencing (Levine, 2010), and Sensory Motor Arousal Regulation Therapy (SMART; Warner et al., 2013), emphasize mindful attention to touch, postures, and movement. Grounded in concepts of experience-dependent neuroplasticity and Hebbian learning (Hebb, 1949; Rao and Sejnowski, 2001), these approaches are posited to repeatedly evoke an organizing response conducive to environmental and self-mastery, or the consistent and satisfying ability to produce meaningful goal-directed action (Ayres, 1972). Small-scale clinical trials of SP-informed group therapy (Langmuir et al., 2012; Gene-Cos et al., 2016; Classen et al., 2021) and case-studies of SMART (Warner et al., 2014; Finn et al., 2018) and SE (Payne et al., 2015) have shown promising results in arousal regulation and reductions in PTSD symptoms. Deep brain re-orienting (DBR) is an approach that similarly targets the multisensory integrative brainstem and midbrain regions by specifically focusing on orienting and postural responses. While trauma results in overwhelming alarm (at the level of the LC and SC) and/or affect (at the level of the PAG), therapeutic attention to the muscular tension in the neck, indicative of SC activation (Corneil and Camp, 2018) elicited in response to a traumatic memory provides information on its pre-affective components. DBR is highly relevant for those with chronic early life trauma given the importance of muscle activation, head turning, and reaching in both approach toward and withdrawal from early attachment figures (Corrigan and Christie-Sands, 2020). These approaches exploit subcortical processes by means of somatic sensory feedback and facilitate awareness and experience of somatic sensations in the present moment of safety as opposed to a past trauma (Fisher, 2011, 2019). This process may be the antithesis to dissociative flashbacks, derealization, and depersonalization and a catalyst for reinstating a sense of agency and trust in the body.
Eye movement desensitization and reprocessing
Eye Movement Desensitization and Reprocessing (EMDR) therapy utilizes saccadic eye movements to elicit an innate relaxation response and integrate maladaptively stored trauma memories (Shapiro, 1995; Oren and Solomon, 2012). Although the mechanism behind EMDR’s therapeutic benefits remains unclear, oculomotor muscles have extensive reciprocal connections with the brainstem reticular formation, vestibular nuclei, and superior colliculi (Horn and Adamzyk, 2012; Harricharan et al., 2019) influencing arousal and multisensory integration. Reduced physiological arousal is reported during EMDR sessions (Aubert-Khalfa et al., 2008; Sack et al., 2008; Söndergaard and Elofsson, 2008), which then attenuates the intensity of negative affect (Oren and Solomon, 2012). Significant reductions in PTSD symptoms have been found in as few as three to eight sessions (Rothbaum, 1997; Wilson et al., 1997; Marcus et al., 2004) although longer durations are recommended for severe and chronic early life traumatization (van der Kolk et al., 2007).
Neurofeedback
Electroencephalographic (EEG) neurofeedback (NFB) training is a non-invasive technique based on the premise that cognitive and motor function depend upon proper communication of neuronal assemblies organized into constellatory networks (Neuper and Pfurtscheller, 2001; Nicholson et al., 2020b). The provision of sensory feedback reflecting neuronal activity in real time has shown to be an effective way to voluntarily promote low frequency, high amplitude alpha oscillations associated with a calm, introspective state. Alpha synchrony may act as a sensory gating mechanism to inhibit irrelevant stimuli (Herrmann and Knight, 2001; Jensen and Mazaheri, 2010; Jones and Sliva, 2020; Saj et al., 2021) and is typically found in deeper midline structures, including the thalamus (Lopes da Silva, 1991) supporting its role in vertical integration. Alpha-targeted neurofeedback has been shown to normalize SN and DMN connectivity and diminish symptom severity in PTSD (Kluetsch et al., 2014; Nicholson et al., 2016, 2020a; van der Kolk et al., 2016; Ros et al., 2017; Rogel et al., 2020) and relieve sensory hyper-sensitivities (Hamed et al., 2022).
Play therapy
For the traumatized child, play-based approaches inclusive of vestibular and somatosensory feedback and affective interpersonal dynamics invite the clinician into the child’s primordial relational world. The richness of vestibular and somatosensory input in classical childhood games spanning generational and cultural boundaries such as “peek-a-boo,” “hide-and-seek,” and “tag” informs the importance of somatic sensory systems in neurodevelopment and in navigating social relationships. For the traumatized child, engaging in these games within a therapeutic context has the promise of re-organizing brainstem level sensory and affective circuitry. By further exploiting the power of a basic affective motivational (PLAY) system at the level of the PAG (Panksepp, 1998), movement play-based therapy restores the intrinsic capacity for orienting to others, enriches the child’s capacity for positive affective responding, and restores the primal urge to seek relational connection through somatic sensory experience (Crenshaw and Kenney-Noziska, 2014; Corrigan and Christie-Sands, 2020).
Yoga
The importance of mindful movement and proprioceptive feedback to ground the body and attune to its needs has been implicit in yogic tradition for centuries. Trauma-informed yoga practitioners guide clients in tolerating titrated somatic sensation and/or the affective states somatic sensory input can arouse. Additionally, mindful movements and postures support top-down regulation of somatically induced arousal. Trauma-Sensitive Yoga (TSY; Emerson et al., 2009; Emerson and Hopper, 2011) is one such example of the utilization of trauma-informed yoga within a therapeutic group setting. Two randomized controlled trials of women with treatment-resistant PTSD showed reductions in symptoms and dissociation; the shorter-duration study (10-weeks) resulted in symptom decreases comparable to psychopharmaceutical and psychotherapeutic interventions (van der Kolk et al., 2014) while extended yoga practice (20-weeks) drove even greater improvements (Price et al., 2017).
Equine assisted therapy
For individuals with severe early life trauma and attachment disruptions, feeling safe within any human relationship may be difficult or impossible. Equine-facilitated psychotherapy (EFP) facilitates attunement to horses (Lentini and Knox, 2015), where individuals learn how to read non-verbal cues and be within a non-threatening, attuned, co-regulatory relationship. EFP involves grooming and caring for the horse to build trust through somatosensory means, as well as mounting (riding the horse with or without a saddle, maintaining postural control, riding with eyes closed) with the guidance of a trained therapist (Naste et al., 2018). A sense of mastery is built through client-directed touch, balance, and bodily control within the context of a safe, co-regulatory dynamic (Kendall and Maujean, 2015). Several non-randomized, small sample-sized studies reported reductions in depressive and PTSD-associated symptoms after EFP (Nevins et al., 2013; Signal et al., 2013; Yorke et al., 2013; Kemp et al., 2014; McCullough et al., 2015; for a review, see Lentini and Knox, 2015; O’Haire et al., 2015).
Expressive arts therapy
Expressive arts therapy, including the therapeutic application of music, dance, theatre, art, and creative writing, is another approach that consistency engages the body through purposeful action (Malchiodi, 2020). Here, the “felt” sense of trauma, experienced within and through the body, is expressed in non-verbal, sensory-based, action-oriented artforms that tap the implicit embodied experiences of trauma defying narrative or logical expression (Terr, 1990; Malchiodi, 2020). Each form of expressive arts therapy incorporates the physical body and meaningful, purposeful action which produces an intentional result, forming or re-forming sensory-motor feedback loops which engender a sense of agency, power, and positive self-environment relationship. Further, the non-verbal rhythmicity inherent in music therapy may provide vibratory stimulation and an external source of rhythm when internal rhythms and sensorimotor synchrony is disrupted due to somatic sensory disintegration. Moderately reduced trauma-related symptoms have been reported in individuals receiving art therapy (Chapman et al., 2001; Curry and Kasser, 2005; Henderson et al., 2007; for a review, see Schouten et al., 2015) and music therapy (Carr et al., 2012) interventions for PTSD.
Conclusion
Janet (1889) classically characterized trauma as an inability to be fully alive in the present moment. If the essence of trauma is the severance of affiliative bonds with the self and others (van der Kolk, 1987), and healing from trauma is indicated by one’s ability to be in an optimal state of arousal while experiencing affective sensations/emotions in the body, then further research into the neurobiological correlates of vestibular and somatosensory processing in trauma-related disorders is imperative and pressing. For those flooded by sensation and emotion, building tolerance of somatic sensation using top-down strategies is essential. For those wired to “shut down” or avoid somatic sensation as a defense mechanism, identification of somatic cues related to shifts in arousal and/or emotion may be facilitated through titrated somatic sensory feedback. Therapeutic approaches centered around attunement to somatic sensory-affective experiences may approach the roots of the dynamic system. This may in turn facilitate a mind-body connection that was, or was not ever, there before, thereby laying the foundation for the restoration of an embodied self that is capable of feeling fully alive in the aftermath of trauma.
Author contributions
Both authors listed have made a substantial, direct, and intellectual contribution to the work, and approved it for publication.
Funding
This work was supported by the Trauma Research Foundation and the Canadian Institute of Health Research.
Acknowledgments
All figures were created with BioRender (www.BioRender. com).
Conflict of interest
The authors declare that the research was conducted in the absence of any commercial or financial relationships that could be construed as a potential conflict of interest.
Publisher’s note
All claims expressed in this article are solely those of the authors and do not necessarily represent those of their affiliated organizations, or those of the publisher, the editors and the reviewers. Any product that may be evaluated in this article, or claim that may be made by its manufacturer, is not guaranteed or endorsed by the publisher.
References
Abdallah, C. G., Averill, C. L., Ramage, A. E., Averill, L. A., Goktas, S., Nemati, S., et al. (2019). Salience network disruption in U.S. army soldiers with posttraumatic stress disorder. Chronic Stress 3, 1–10. doi: 10.1177/2470547019850467
Abraira, V. E., and Ginty, D. D. (2013). The sensory neurons of touch. Neuron 79, 618–639. doi: 10.1016/j.neuron.2013.07.051
Ackerley, R., Backlund Wasling, H., Liljencrantz, J., Olausson, H., Johnson, R. D., and Wessberg, J. (2014). Human C-tactile afferents are tuned to the temperature of a skin-stroking caress. J. Neurosci. 34, 2879–2883. doi: 10.1523/JNEUROSCI.2847-13.2014
Adamo, S. A. (2012). The effects of the stress response on immune function in invertebrates: An evolutionary perspective on an ancient connection. Horm. Behav. 62, 324–330. doi: 10.1016/j.yhbeh.2012.02.012
Ainsworth M. D. S., Blehar M. C., Waters E., & Wall S. (1978). Patterns of attachment: A psychological study of the strange situation. Hillsdale, NJ: Erlbaum.
Akaike, T. (1983). Neuronal organization of the vestibulospinal system in the cat. Brain Res. 259, 217–227. doi: 10.1016/0006-8993(83)91252-0
Akbarian, S., Grüsser, O. J., and Guldin, W. O. (1992). Thalamic connections of the vestibular cortical fields in the squirrel monkey (Saimiri sciureus). J. Comp. Neurol. 326, 423–441. doi: 10.1002/cne.903260308
Akiki, T. J., Averill, C. L., and Abdallah, C. G. (2017). A network-based neurobiological model of PTSD: Evidence from structural and functional neuroimaging studies. Curr. Psychiatry Rep. 19, 1–10. doi: 10.1007/s11920-017-0840-4
Alais, D., Newell, F. N., and Mamassian, P. (2010). Multisensory processing in review: From physiology to behaviour. Seeing Perceiving 23, 3–38. doi: 10.1163/187847510X488603
Alexander, P. C. (1993). The differential effects of abuse characteristics and attachment in the prediction of long-term effects of sexual abuse. J. Interpers. Violence 8, 346–362. doi: 10.1177/088626093008003004
Allen, M. (2020). Unravelling the neurobiology of interoceptive inference. Trends Cogn. Sci. 24, 265–266. doi: 10.1016/j.tics.2020.02.002
American Psychiatric Association (2013). Diagnostic and statistical manual of mental disorders, 5th Edn. Arlington, VA: American Psychiatric Publishing.
Amodio, D. M., and Frith, C. D. (2006). Meeting of minds: The medial frontal cortex and social cognition. Nat. Rev. Neurosci. 7, 268–277. doi: 10.1038/nrn1884
Anagnostaras, S. G., and Robinson, T. E. (1996). Sensitization to the psychomotor stimulant effects of amphetamine: Modulation by associative learning. Behav. Neurosci. 110, 1397–1414. doi: 10.1037/0735-7044.110.6.1397
Armour, C., Karstoft, K. I., and Richardson, J. D. (2014). The co-occurrence of PTSD and dissociation: Differentiating severe PTSD from dissociative-PTSD. Soc. Psychiatry Psychiatr. Epidemiol. 49, 1297–1306. doi: 10.1007/s00127-014-0819-y
Ashwell, K. W. S., and Waite, P. M. E. (2012). “Development of the peripheral nervous system,” in The human nervous system, 3rd Edn, eds J. K. Mai and G. Paxinos (Amsterdam: Elsevier), 14–30.
Aston-Jones, G., and Cohen, J. D. (2005). An integrative theory of locus coeruleus-norepinephrine function: Adaptive gain and optimal performance. Annu. Rev. Neurosci. 28, 403–450. doi: 10.1146/annurev.neuro.28.061604.135709
Atick, J. J. (2011). Could information theory provide an ecological theory of sensory processing? Network 22, 4–44. doi: 10.3109/0954898x.2011.638888
Aubert-Khalfa, S., Roques, J., and Blin, O. (2008). Evidence of a decreae in heart rate and skin conductance responses in PTSD patients after a single EMDR session. J. EMDR Pract. Res. 2, 51–56. doi: 10.1891/1933-3196.2.1.51
Ayres, A. J. (1972). Sensory integration and learning disorders. Torrance: Western Psychological Services.
Badura-Brack, A. S., Becker, K. M., McDermott, T. J., Ryan, T. J., Becker, M. M., Hearley, A. R., et al. (2015). Decreased somatosensory activity to non-threatening touch in combat veterans with posttraumatic stress disorder. Psychiatry Res. Neuroimaging 233, 194–200. doi: 10.1016/j.pscychresns.2015.06.012
Balaban, C. D., and Porter, J. D. (1998). Neuroanatomic substrates for vestibulo-autonomicinteractions. J. Vestib. Res. Equilibrium Orientat. 8, 7–16. doi: 10.3233/ves-1998-8102
Balaban, C. D., and Thayer, J. F. (2001). Neurological bases for balance-anxiety links. J. Anxiety Disorder. 15, 53–79. doi: 10.1016/S0887-6185(00)00042-6
Balaban, C. D., Jacob, R. G., and Furman, J. M. (2011). Neurologic bases for comorbidity of balance disorders, anxiety disorders and migraine: Neurotherapeutic implications. Exp. Rev. Neurother. 11, 379–394. doi: 10.1586/ern.11.19
Bandler, R., and Depaulis, A. (1991). “Midbrain periaqueductal grey control of defensive behaviour in the cat and the rat,” in The midbrain periaqueductal gray matter, eds A. Depaulis and R. Bandler (Boston, MA: Springer), 175–198.
Baranek, G. T., Woynaroski, T. G., Nowell, S., Turner-Brown, L., DuBay, M., Crais, E. R., et al. (2018). Cascading effects of attention disengagement and sensory seeking on social symptoms in a community sample of infants at-risk for a future diagnosis of autism spectrum disorder. Dev. Cogn. Neurosci. 29, 30–40. doi: 10.1016/j.dcn.2017.08.006
Barrett, L. F., and Simmons, W. K. (2015). Interoceptive predictions in the brain. Nat. Rev. Neurosci. 16, 419–429. doi: 10.1038/nrn3950
Basso, M. A., Bickford, M. E., and Cang, J. (2021). Unraveling circuits of visual perception and cognition through the superior colliculus. Neuron 109, 918–937. doi: 10.1016/j.neuron.2021.01.013
Beeney, J. E., Wright, A. G. C., Stepp, S. D., Hallquist, M. N., Lazarus, S. A., Beeney, J. R. S., et al. (2017). Disorganized attachment and personality functioning in adults: A latent class analysis. Pers. Disord. 8, 206–216. doi: 10.1037/per0000184
Bell, J., Bolanowski, S., and Holmes, M. H. (1994). The structure and function of pacinian corpuscles: A review. Prog. Neurobiol. 42, 918–937. doi: 10.1016/0301-0082(94)90022-1
Bense, S., Stephan, T., Yousry, T. A., Brandt, T., and Dieterich, M. (2001). Multisensory cortical signal increases and decreases during vestibular galvanic stimulation (fMRI). J. Neurophysiol. 85, 886–899. doi: 10.1152/jn.2001.85.2.886
Berridge, C. W., and Waterhouse, B. D. (2003). The locus coeruleus-noradrenergic system: Modulation of behavioral state and state-dependent cognitive processes. Brain Res. Rev. 42, 33–84. doi: 10.1016/S0165-0173(03)00143-7
Bertsch, K., Schmidinger, I., Neumann, I. D., and Herpertz, S. C. (2013). Reduced plasma oxytocin levels in female patients with borderline personality disorder. Horm. Behav. 63, 424–429. doi: 10.1016/j.yhbeh.2012.11.013
Besser, A., and Neria, Y. (2012). When home isn’t a safe haven: Insecure attachment orientations, perceived social support, and PTSD symptoms among Israeli evacuees under missile threat. Psychol. Trauma Theory, Res. Pract. Policy 4, 34–46. doi: 10.1037/a0017835
Biaggioni, I., Costa, F., and Kaufmann, H. (1998). Vestibular influences on autonomic cardiovascular control in humans. J. Vestib. Res. Equilibrium Orientat. 8, 35–41. doi: 10.3233/ves-1998-8105
Bichescu-Burian, D. M., Grieb, B., Steinert, T., Uhlmann, C., and Steyer, J. (2018). Use of a psychophysiological script-driven imagery experiment to study trauma-related dissociation in borderline personality disorder. J. Vis. Exp. 133:E56111. doi: 10.3791/56111
Björnsdotter, M., Gordon, I., Pelphrey, K. A., Olausson, H., and Kaiser, M. D. (2014). Development of brain mechanisms for processing affective touch. Front. Behav. Neurosci. 8:24. doi: 10.3389/fnbeh.2014.00024
Björnsdotter, M., Löken, L., Olausson, H., Vallbo, Å, and Wessberg, J. (2009). Somatotopic organization of gentle touch processing in the posterior insular cortex. J. Neurosci. 29, 9314–9320. doi: 10.1523/JNEUROSCI.0400-09.2009
Blanke, O. (2012). Multisensory brain mechanisms of bodily self-consciousness. Nat. Rev. Neurosci. 13, 556–571. doi: 10.1038/nrn3292
Blanke, O., and Metzinger, T. (2009). Full-body illusions and minimal phenomenal selfhood. Trends Cogn. Sci. 13, 7–13. doi: 10.1016/j.tics.2008.10.003
Blayney, A. W. (1997). “Vestibular disorders,” in Scott-Brown’s Otolaryngology: Paediatric otolaryngology, 6th Edn, Vol. 6, eds D. A. Adams and M. J. Cinnamond (Boca Raton, FL: CRC Press), 1–29.
Blevins, C. A., Weathers, F. W., and Witte, T. K. (2014). Dissociation and posttraumatic stress disorder: A latent profile analysis. J. Trauma. Stress 27, 388–396. doi: 10.1002/jts.21933
Bluhm, R. L., Williamson, P. C., Osuch, E. A., Frewen, P. A., Stevens, T. K., Boksman, K., et al. (2009). Alterations in default network connectivity in posttraumatic stress disorder related to early-life trauma. J. Psychiatry Neurosci. 34, 187–194.
Bogaerts, S., Kunst, M. J. J., and Winkel, F. W. (2009). Dismissive attachment and posttraumatic stress disorder among securely and insecurely attached Belgian security workers. Psychol. Rep. 105, 889–899. doi: 10.2466/PR0.105.3.889-899
Bohic, M., and Abraira, V. E. (2022). Wired for social touch: The sense that binds us to others. Curr. Opin. Behav. Sci. 43, 207–215. doi: 10.1016/j.cobeha.2021.10.009
Bond, A. M., Bhalala, O. G., and Kessler, J. A. (2012). The dynamic role of bone morphogenetic proteins in neural stem cell fate and maturation. Dev. Neurobiol. 72, 1068–1084. doi: 10.1002/dneu.22022
Bottini, G., Karnath, H. O., Vallar, G., Sterzi, R., Frith, C. D., Frackowiak, R. S. J., et al. (2001). Cerebral representations for egocentric space: Functional-anatomical evidence from caloric vestibular stimulation and neck vibration. Brain 124, 1182–1196. doi: 10.1093/brain/124.6.1182
Bottini, G., Sterzi, R., Paulesu, E., Vallar, G., Cappa, S. F., Erminio, F., et al. (1994). Identification of the central vestibular projections in man: A positron emission tomography activation study. Exp. Brain Res. 99, 164–169. doi: 10.1007/BF00241421
Boulet, C., Lopez-Castroman, J., Mouchabac, S., Olié, E., Courtet, P., Thouvenot, E., et al. (2022). Stress response in dissociation and conversion disorders: A systematic review. Neurosci. Biobehav. Rev. 132, 957–967. doi: 10.1016/j.neubiorev.2021.10.049
Bourinet, E., Martin, M., Huzard, D., Jeanneteau, F., Mery, P.-F., and François, A. (2021). The impact of C-Tactile Low threshold mechanoreceptors on affective touch and social interactions in mice. BioRxiv [Preprint]. doi: 10.1101/2021.01.13.426492
Bowlby, J. (1973). Attachment and loss: Volume II: Separation, anxiety and anger. New York, NY: Penguin Books, LTD.
Bracha, H. S. (2004). Freeze, flight, fight, fright, faint: Adaptationist perspectives on the acute stress response spectrum. CNS Spectrums 9, 679–685. doi: 10.1017/S1092852900001954
Bradley, R., Greene, J., Russ, E., Dutra, L., and Westen, D. (2005). A multidimensional meta-analysis of psychotherapy for PTSD. Am. J. Psychiatry 162, 214–227. doi: 10.1176/appi.ajp.162.2.214
Brandão, M. L., and Lovick, T. A. (2019). Role of the dorsal periaqueductal gray in posttraumatic stress disorder: Mediation by dopamine and neurokinin. Transl. Psychiatry 9, 1–9. doi: 10.1038/s41398-019-0565-8
Brandt, T., Dieterich, M., and Danek, A. (1994). Vestibular cortex lesions affect the perception of verticality. Ann. Neurol. 35, 403–412. doi: 10.1002/ana.410350406
Brennstuhl, M. J., Harvey, A. G., Gurthrie, R. M., and Moulds, M. L. (2015). Chronic pain and PTSD: Evolving views on their comorbidity. Perspect. Psychiatr. Care 51, 295–304. doi: 10.1111/ppc.12093
Britton, Z., and Arshad, Q. (2019). Vestibular and multi-sensory influences upon self-motion perception and the consequences for human behavior. Front. Neurol. 10:63. doi: 10.3389/fneur.2019.00063
Bryant, R. A. (2003). Early predictors of posttraumatic stress disorder. Biol. Psychiatry 53, 789–795. doi: 10.1016/S0006-3223(02)01895-4
Bryant, R. A., Harvey, A. G., Guthrie, R. M., and Moulds, M. L. (2000). A prospective study of psychophysiological arousal, acute stress disorder, and posttraumatic stress disorder. J. Abnorm. Psychol. 109, 341–344. doi: 10.1037/0021-843X.109.2.341
Brzozowska, A., Longo, M. R., Mareschal, D., Wiesemann, F., and Gliga, T. (2022). Oxytocin but not naturally occurring variation in caregiver touch associates with infant social orienting. Dev. Psychobiol. 64:e22290. doi: 10.1002/dev.22290
Buck, R. (1999). The biological affects: A typology. Psychol. Rev. 106, 301–336. doi: 10.1037/0033-295X.106.2.301
Bystrova, K. (2009). Novel mechanism of human fetal growth regulation: A potential role of lanugo, vernix caseosa and a second tactile system of unmyelinated low-threshold C-afferents. Med. Hypotheses 72, 143–146. doi: 10.1016/j.mehy.2008.09.033
Caldji, C., Tannenbaum, B., Sharma, S., Francis, D., Plotsky, P. M., and Meaney, M. J. (1998). Maternal care during infancy regulates the development of neural systems mediating the expression of fearfulness in the rat. Proc. Natl. Acad. Sci. U.S.A. 95, 5335–5340. doi: 10.1073/pnas.95.9.5335
Carmona, J. E., Holland, A. K., and Harrison, D. W. (2009). Extending the functional cerebral systems theory of emotion to the vestibular modality: A systematic and integrative approach. Psychol. Bull. 135, 286–302. doi: 10.1037/a0014825
Carr, C., D’Ardenne, P., Sloboda, A., Scott, C., Wang, D., and Priebe, S. (2012). Group music therapy for patients with persistent post-traumatic stress disorder – An exploratory randomized controlled trial with mixed methods evaluation. Psychol. Psychother. Theory Res. Pract. 85, 179–202. doi: 10.1111/j.2044-8341.2011.02026.x
Carrive, P., and Morgan, M. M. (2012). “Development of the peripheral nervous system,” in The human nervous system, 3rd Edn, eds J. K. Mai and G. Paxinos (Amsterdam: Elsevier), 367–400.
Cascio, C. J., Moore, D., and McGlone, F. (2019). Social touch and human development. Dev. Cogn. Neurosci. 35, 5–11. doi: 10.1016/j.dcn.2018.04.009
Champagne, F., Diorio, J., Sharma, S., and Meaney, M. J. (2001). Naturally occurring variations in maternal behavior in the rat are associated with differences in estrogen-inducible central oxytocin receptors. Proc. Natl. Acad. Sci. U.S.A. 98, 12736–12741. doi: 10.1073/pnas.221224598
Chapman, L., Morabito, D., Knudson, M. M., Ladakakos, C., and Schreier, H. (2001). The effectiveness of art therapy interventions in reducing Post Traumatic Stress Disorder (PTSD) symptoms in pediatric trauma patients. Art Ther. 18, 100–104. doi: 10.1080/07421656.2001.10129750
Chatzittofis, A., Nordström, P., Uvnäs-Moberg, K., Åsberg, M., and Jokinen, J. (2014). CSF and plasma oxytocin levels in suicide attempters, the role of childhood trauma and revictimization. Neuroendocrinol. Lett. 35, 213–217.
Chu, J. A., Frey, L. M., Ganzel, B. L., and Matthews, J. A. (1999). Memories of childhood abuse: Dissociation, amnesia, and corroboration. Am. J. Psychiatry 156, 749–755. doi: 10.1176/ajp.156.5.749
Ciaunica, A., Constant, A., Preissl, H., and Fotopoulou, K. (2021a). The first prior: From co-embodiment to co-homeostasis in early life. Conscious. Cogn. 91:103117. doi: 10.1016/J.CONCOG.2021.103117
Ciaunica, A., Hesp, C., Seth, A., Limanowski, J., and Friston, K. (2021b). I overthink- therefore I am not: Altered sense of self in depersonalisation disorder. Conscious. Cogn. 101:103320. doi: 10.1016/j.concog.2022.103320
Ciaunica, A., Roepstorff, A., Fotopoulou, A. K., and Petreca, B. (2021c). Whatever next and close to my self- The transparent senses and the “second skin”: Implications for the case of depersonalization. Front. Psychol. 1:613587. doi: 10.3389/fpsyg.2021.613587
Classen, C. C., Hughes, L., Clark, C., Hill Mohammed, B., Woods, P., and Beckett, B. (2021). A Pilot RCT of A Body-oriented group therapy for complex trauma survivors: An adaptation of sensorimotor psychotherapy. J. Trauma Dissoc. 22, 52–68. doi: 10.1080/15299732.2020.1760173
Corneil, B. D., and Camp, A. J. (2018). Animal models of vestibular evoked myogenic potentials: The past, present, and future. Front. Neurol. 9:489. doi: 10.3389/fneur.2018.00489
Corrigan, F. M., and Christie-Sands, J. (2020). An innate brainstem self-other system involving orienting, affective responding, and polyvalent relational seeking: Some clinical implications for a “Deep Brain Reorienting” trauma psychotherapy approach. Med. Hypotheses 136:109502. doi: 10.1016/j.mehy.2019.109502
Coull, J. T. (1997). The neural correlates of the noradrenergic modulation of human attention, arousal and learning. Eur. J. Neurosci. 9, 589–598. doi: 10.1111/j.1460-9568.1997.tb01635.x
Cox, K. S., Resnick, H. S., and Kilpatrick, D. G. (2014). Prevalence and correlates of posttraumautic distorted beliefs: Evaluating DSM-5 PTSD expanded cognitive symptoms in a national sample. J. Trauma. Stress 27, 299–306. doi: 10.1002/jts.21925
Craig, A. D. (2002). How do you feel? Interoception: The sense of the physiological condition of the body. Nat. Rev. Neurosci. 3, 655–656. doi: 10.1038/nrn894
Craig, A. D. (2003). Interoception: The sense of the physiological condition of the body. Curr. Opin. Neurobiol. 13, 500–505. doi: 10.1016/s0959-4388(03)00090-4
Craig, A. D. (2009). Emotional moments across time: A possible neural basis for time perception in the anterior insula. Philos. Trans. Royal Soc. B Biol. Sci. 364, 1933–1942. doi: 10.1098/rstb.2009.0008
Crenshaw, D. A., and Kenney-Noziska, S. (2014). Therapeutic presence in play therapy. Int. J. Play Ther. 23, 31–43. doi: 10.1037/a0035480
Critchley, H. D., and Garfinkel, S. N. (2017). Interoception and emotion. Curr. Opin. Psychol. 17, 7–14. doi: 10.1016/j.copsyc.2017.04.020
Critchley, H. D., Wiens, S., Rotshtein, P., Öhman, A., and Dolan, R. J. (2004). Neural systems supporting interoceptive awareness. Nat. Neurosci. 7, 189–195. doi: 10.1038/nn1176
Crucianelli, L., and Ehrsson, H. (2022). The role of the skin in interoception: A neglected organ? PsyArXiv [Preprint]. doi: 10.31234/osf.io/qfu87
Crucianelli, L., and Filippetti, M. L. (2020). Developmental Perspectives on Interpersonal Affective Touch. Topoi 39, 575–586. doi: 10.1007/s11245-018-9565-1
Crucianelli, L., Krahé, C., Jenkinson, P. M., and Fotopoulou, A. K. (2018). Interoceptive ingredients of body ownership: Affective touch and cardiac awareness in the rubber hand illusion. Cortex 104, 180–192. doi: 10.1016/j.cortex.2017.04.018
Cuppini, C., Stein, B. E., and Rowland, B. A. (2018). Development of the mechanisms governing midbrain multisensory integration. J. Neurosci. 38, 3453–3465. doi: 10.1523/JNEUROSCI.2631-17.2018
Curry, N. A., and Kasser, T. (2005). Can coloring mandalas reduce anxiety? Art Ther. 22, 81–85. doi: 10.1080/07421656.2005.10129441
D’Andrea, W., Pole, N., DePierro, J., Freed, S., and Wallace, D. B. (2013). Heterogeneity of defensive responses after exposure to trauma: Blunted autonomic reactivity in response to startling sounds. Int. J. Psychophysiol. 90, 80–89. doi: 10.1016/j.ijpsycho.2013.07.008
Damasio, A. R. (1998). Emotion in the perspective of an integrated nervous system. Brain Res. Rev. 26, 83–86. doi: 10.1016/S0165-0173(97)00064-7
Darwin, C. (1872). The expression of the emotions in man and animals. Oxford: Oxford University Press.
Davidovic, M., Starck, G., and Olausson, H. (2019). Processing of affective and emotionally neutral tactile stimuli in the insular cortex. Dev. Cogn. Neurosci. 35, 94–103. doi: 10.1016/j.dcn.2017.12.006
de Cicco, V., Tramonti Fantozzi, M. P., Cataldo, E., Barresi, M., Bruschini, L., Faraguna, U., et al. (2018). Trigeminal, visceral and vestibular inputs may improve cognitive functions by acting through the locus coeruleus and the ascending reticular activating system: A new hypothesis. Front. Neuroanat. 11:130. doi: 10.3389/fnana.2017.00130
de Kleine, R. A., Haganaars, M. A., and van Minnen, A. (2018). Tonic immobility during re-experiencing the traumatic event in posttraumatic stress disorder. Psychiatry Res. 270, 1105–1109. doi: 10.1016/j.psychres.2018.06.051
De Oca, B. M., DeCola, J. P., Maren, S., and Fanselow, M. S. (1998). Distinct regions of the periaqueductal gray are involved in the acquisition and expression of defensive responses. J. Neurosci. 18, 3426–3432. doi: 10.1523/jneurosci.18-09-03426.1998
Dean, P., Redgrave, P., and Westby, G. W. M. (1989). Event or emergency? Two response systems in the mammalian superior colliculus. Trends Neurosci. 12, 137–147. doi: 10.1016/0166-2236(89)90052-0
Declercq, F., and Willemsen, J. (2006). Distress and post-traumatic stress disorders in high risk professionals: Adult attachment style and the dimensions of anxiety and avoidance. Clin. Psychol. Psychother. 13, 256–263. doi: 10.1002/cpp.492
Deecke, L., Schwarz, D. W. F., and Fredrickson, J. M. (1977). Vestibular responses in the Rhesus monkey ventroposterior thalamus. II. Vestibulo-proprioceptive convergence at thalamic neurons. Exp. Brain Res. 30, 219–232. doi: 10.1007/BF00237252
del Valle, M. E., Cobo, T., Cobo, J. L., and Vega, J. A. (2012). Mechanosensory neurons, cutaneous mechanoreceptors, and putative mechanoproteins. Microscopy Res. Technique 75, 1033–1043. doi: 10.1002/jemt.22028
della Longa, L., Carnevali, L., Patron, E., Dragovic, D., and Farroni, T. (2021). Psychophysiological and visual behavioral responses to faces associated with affective and non-affective touch in four-month-old infants. Neuroscience 464, 67–78. doi: 10.1016/j.neuroscience.2020.07.053
Depaulis, A., Keay, K. A., and Bandler, R. (1994). Quiescence and hyporeactivity evoked by activation of cell bodies in the ventrolateral midbrain periaqueductal gray of the rat. Exp. Brain Res. 99, 75–83. doi: 10.1007/BF00241413
Deroualle, D., Borel, L., Devèze, A., and Lopez, C. (2015). Changing perspective: The role of vestibular signals. Neuropsychologia 79, 175–185. doi: 10.1016/j.neuropsychologia.2015.08.022
Devine, S. L., Walker, S. C., Makdani, A., Stockton, E. R., McFarquhar, M. J., McGlone, F. P., et al. (2020). Childhood adversity and affective touch perception: A comparison of United Kingdom care leavers and non-care leavers. Front. Psychol. 11:557171. doi: 10.3389/fpsyg.2020.557171
Diego, M. A., and Field, T. (2009). Moderate pressure massage elicits a parasympathetic nervous system response. Int. J. Neurosci. 119, 630–638. doi: 10.1080/00207450802329605
Dieperink, M., Leskela, J., Thuras, P., and Engdahl, B. (2001). Attachment style classification and posttraumatic stress disorder in former prisoners of war. Am. J. Orthopsychiatry 71, 374–378. doi: 10.1037/0002-9432.71.3.374
Ditzen, B., Neumann, I. D., Bodenmann, G., von Dawans, B., Turner, R. A., Ehlert, U., et al. (2007). Effects of different kinds of couple interaction on cortisol and heart rate responses to stress in women. Psychoneuroendocrinology 32, 565–574. doi: 10.1016/j.psyneuen.2007.03.011
Donadon, M. F., Martin-Santos, R., Osório, F., and de, L. (2018). The associations between oxytocin and trauma in humans: A systematic review. Front. Pharmacol. 9:154. doi: 10.3389/fphar.2018.00154
Dorahy, M. J., Brand, B. L., Şar, V., Krüger, C., Stavropoulos, P., Martínez-Taboas, A., et al. (2014). Dissociative identity disorder: An empirical overview. Austr. New Zealand J. Psychiatry 48, 402–417. doi: 10.1177/0004867414527523
Ebner-Priemer, U. W., Badeck, S., Beckmann, C., Wagner, A., Feige, B., Weiss, I., et al. (2005). Affective dysregulation and dissociative experience in female patients with borderline personality disorder: A startle response study. J. Psychiatr. Res. 39, 85–92. doi: 10.1016/j.jpsychires.2004.05.001
Edwards, S. B., Ginsburgh, C. L., Henkel, C. K., and Stein, B. E. (1979). Sources of subcortical projections to the superior colliculus in the cat. J. Comp. Neurol. 184, 309–329. doi: 10.1002/cne.901840207
Eklund, G. (1972). General features of vibration-induced effects on balance. Upsala J. Med. Sci. 77, 112–124. doi: 10.1517/03009734000000016
Emerson, D., and Hopper, E. (2011). Overcoming trauma through yoga: Reclaiming your body. Berkeley, CA: North Atlantic Books.
Emerson, D., Sharma, R., Chaudhry, S., and Turner, J. (2009). Trauma-Sensitive Yoga: Principles, practice, and research. Int. J. Yoga Ther. 19, 123–128. doi: 10.17761/ijyt.19.1.h6476p8084l22160
Engel-Yeger, B., Palgy-Levin, D., and Lev-Wiesel, R. (2013). The sensory profile of people with post-traumatic stress symptoms. Occup. Ther. Ment. Health 29, 266–278. doi: 10.1080/0164212X.2013.819466
Fairhurst, M. T., Löken, L., and Grossmann, T. (2014). Physiological and behavioral responses reveal 9-month-old infants’ sensitivity to pleasant touch. Psychol. Sci. 25, 1124–1131. doi: 10.1177/0956797614527114
Farroni, T., della Longa, L., and Valori, I. (2022). The self-regulatory affective touch: A speculative framework for the development of executive functioning. Curr. Opin. Behav. Sci. 43, 167–173. doi: 10.1016/j.cobeha.2021.10.007
Fasold, O., Heinau, J., Trenner, M. U., Villringer, A., and Wenzel, R. (2008). Proprioceptive head posture-related processing in human polysensory cortical areas. Neuroimage 40, 1232–1242. doi: 10.1016/j.neuroimage.2007.12.060
Ferrè, E. R., and Haggard, P. (2015). Vestibular-somatosensory interactions: A mechanism in search of a function? Multisens. Res. 28, 559–579. doi: 10.1163/22134808-00002487
Ferrè, E. R., and Haggard, P. (2016). The vestibular body: Vestibular contributions to bodily representations. Cogn. Neuropsychol. 33, 67–81. doi: 10.1080/02643294.2016.1168390
Ferrè, E. R., and Haggard, P. (2020). Vestibular cognition: State-of-the-art and future directions. Cogn. Neuropsychol. 37, 413–420. doi: 10.1080/02643294.2020.1736018
Ferrè, E. R., Bottini, G., Iannetti, G. D., and Haggard, P. (2013). The balance of feelings: Vestibular modulation of bodily sensations. Cortex 49, 748–758. doi: 10.1016/j.cortex.2012.01.012
Ferrè, E. R., Lopez, C., and Haggard, P. (2014). Anchoring the self to the body: Vestibular contribution to the sense of self. Psychol. Sci. 25, 2106–2108. doi: 10.1177/0956797614547917
Finn, H., Warner, E., Price, M., and Spinazzola, J. (2018). The boy who was hit in the face: Somatic regulation and processing of preverbal complex trauma. J. Child Adolesc. Trauma 11, 277–288. doi: 10.1007/s40653-017-0165-9
Fischer, G., and Riedesser, P. (1999). Lehrbuch der psychotraumatologie (Textbook of psychotraumatology). Stuttgart: UTB.
Fisher, J. (2011). Sensorimotor approaches to trauma treatment. Adv. Psychiatr. Treatment 17, 171–177. doi: 10.1192/apt.bp.109.007054
Fisher, J. (2019). Sensorimotor psychotherapy in the treatment of trauma. Pract. Innov. 4, 156–165. doi: 10.1037/pri0000096
Fleischmann, A., and Urca, G. (1988). Clip-induced analgesia and immobility in the mouse: Pharmacological characterization. Neuropharmacology 27, 641–648. doi: 10.1016/0028-3908(88)90187-6
Foa, E. B., and Hearst-Ikeda, D. (1996). “Emotional dissociation in response to trauma: An information-processing approach,” in Handbook of dissociation, eds L. K. Michelson and W. J. Ray (New York, NY: Springer), 207–225. doi: 10.1007/978-1-4899-0310-5_10
Foa, E. B., Ehlers, A., Clark, D. M., Tolin, D. F., and Orsillo, S. M. (1999). The posttraumatic cognitions inventory (PTCI): Development and validation. Psychol. Assess. 11, 303–314. doi: 10.1037/1040-3590.11.3.303
Fotopoulou, A., and Tsakiris, M. (2017). Mentalizing homeostasis: The social origins of interoceptive inference. Neuropsychoanalysis 19, 3–28. doi: 10.1080/15294145.2017.1294031
Fragkaki, I., Stins, J., Roelofs, K., Jongedijk, R. A., and Haganaars, M. A. (2016). Tonic immobility differentiates stress responses in PTSD. Brain Behav. 6:e00546. doi: 10.1002/brb3.546
Fraley, R. C., Fazzari, D. A., Bonanno, G. A., and Dekel, S. (2006). Attachment and psychological adaptation in high exposure survivors of the September 11th attack on the World Trade Center. Pers. Soc. Psychol. Bull. 32, 538–551. doi: 10.1177/0146167205282741
Francis, D. D., Champagne, F. C., and Meaney, M. J. (2000). Variations in maternal behaviour are associated with differences in oxytocin receptor levels in the rat. J. Neuroendocrinol. 12, 1145–1148. doi: 10.1046/j.1365-2826.2000.00599.x
Frank, S. M., and Greenlee, M. W. (2018). The parieto-insular vestibular cortex in humans: More than a single area? J. Neurophysiol. 120, 1438–1450. doi: 10.1152/jn.00907.2017
Fraser, K., MacKenzie, D., and Versnel, J. (2017). Complex trauma in children and youth: A scoping review of sensory-based interventions. Occup. Ther. Ment. Health 33, 199–216. doi: 10.1080/0164212X.2016.1265475
Fregoso, G., Wang, A., Tseng, K., and Wang, J. (2019). Transition from acute to chronic pain: Evaluating risk for chronic postsurgical pain. Pain Phys. 22, 479–488. doi: 10.36076/ppj/2019.22.479
French, J. D., and Magoun, H. W. (1952). Effects of chronic lesions in central cephalic brain stem of monkeys. Arch. Neurol. Psychiatry 68, 591–604. doi: 10.1001/archneurpsyc.1952.02320230017002
Frewen, P. A., and Lanius, R. A. (2006). Toward a psychobiology of posttraumatic self-dysregulation: Reexperiencing, hyperarousal, dissociation, and emotional numbing. Ann. N Y Acad. Sci. 1071, 110–124. doi: 10.1196/annals.1364.010
Frewen, P. A., and Lanius, R. A. (2015). Healing the traumatized self: Consciousness, neuroscience, treatment. New York, NY: North and Company.
Fritzsch, B., Kopecky, B. J., and Duncan, J. S. (2014). “Development of the mammalian ‘vestibular’ system: Evolution of form to detect angular and gravity acceleration,” in Development of auditory and vestibular systems, eds R. Romand I, and Varela-Nieto (San Diego, CA: Academic Press), 339–367.
Furman, J. M., Jacob, R. G., and Redfern, M. S. (1998). Clinical evidence that the vestibular system participates in autonomic control. J. Vestib. Res. Equilibrium Orientat. 8, 27–34. doi: 10.3233/ves-1998-8104
Gallace, A., and Spence, C. (2010). The science of interpersonal touch: An overview. Neurosci. Biobehav. Rev. 34, 246–259. doi: 10.1016/j.neubiorev.2008.10.004
Gallagher, M., Kearney, B., and Ferrè, E. (2021). Where is my hand in space? The internal model of gravity influences proprioception. Biol. Lett. 17:20210115. doi: 10.1098/rsbl.2021.0115
Gallup, G. G. (1977). Tonic immobility: The role of fear and predation. Psychol. Record 27, 41–61. doi: 10.1007/bf03394432
Gdowski, G. T., and McCrea, R. A. (2000). Neck proprioceptive inputs to primate vestibular nucleus neurons. Exp. Brain Res. 135, 511–526. doi: 10.1007/s002210000542
Gene-Cos, N., Fisher, J., Ogden, P., and Cantrel, A. (2016). Sensorimotor psychotherapy group therapy in the treatment of complex PTSD. Ann. Psychiatry Ment. Health 4, 156–165.
Ghafoori, B., Hierholzer, R., Howsepian, B., and Boardman, A. (2008). The role of adult attachment, parental bonding, and spiritual love in the adjustment to military trauma. J. Trauma Dissoc. 9, 85–106. doi: 10.1080/15299730802073726
Gilbert, C. D., and Sigman, M. (2007). Brain states: Top-down influences in sensory processing. Neuron 54, 677–696. doi: 10.1016/j.neuron.2007.05.019
Gillespie, P. G., and Walker, R. G. (2001). Molecular basis of mechanosensory transduction. Nature 413, 194–202. doi: 10.1038/35093011
Goodwin, G. M., Mccloskey, D. I., and Matthews, P. B. C. (1972). Proprioceptive illusions induced by muscle vibration: Contribution by muscle spindles to perception? Science 175, 1382–1384. doi: 10.1126/science.175.4028.1382
Grantyn, A., Moschovakis, A. K., and Kitama, T. (2005). Control of orienting movements: Role of multiple tectal projections of the lower brainstem. Prog. Brain Res. 143, 423–438. doi: 10.1016/S0079-6123(03)43040-9
Griffin, M. G., Resick, P. A., and Mechanic, M. B. (1997). Objective assessment of peritraumatic dissociation: Psychophysiological indicators. Am. J. Psychiatry 154, 1081–1088. doi: 10.1176/ajp.154.8.1081
Guldin, W. O., Akbarian, S., and Grüsser, O. J. (1992). Cortico-cortical connections and cytoarchitectonics of the primate vestibular cortex: A study in squirrel monkeys (Saimiri sciureus). J. Comp. Neurol. 326, 375–401. doi: 10.1002/cne.903260306
Guldin, W. O., and Grüsser, O. J. (1998). Is there a vestibular cortex? Trends Neurosci. 21, 254–259. doi: 10.1016/S0166-2236(97)01211-3
Guzmán-Flores, C., Buendía, N., Anderson, C., and Lindsley, D. B. (1962). Cortical and reticular influences upon evoked responses in dorsal column nuclei. Exp. Neurol. 5, 37–46. doi: 10.1016/0014-4886(62)90068-7
Hadders-Algra, M. (2007). Putative neural substrate of normal and abnormal general movements. Neurosci. Biobehav. Rev. 31, 1181–1190. doi: 10.1016/j.neubiorev.2007.04.009
Halvorsen, J. Ø., Stenmark, H., Neuner, F., and Nordahl, H. M. (2014). Does dissociation moderate treatment outcomes of narrative exposure therapy for PTSD? A secondary analysis from a randomized controlled clinical trial. Behav. Res. Ther. 57: 21–28. doi: 10.1016/j.brat.2014.03.010
Hamed, R., Mizrachi, L., Granovsky, Y., Issachar, G., Yuval-Greenberg, S., and Bar-Shalita, T. (2022). Neurofeedback therapy for sensory over-responsiveness—A feasibility study. Sensors 22:1845. doi: 10.3390/s22051845
Hansen, M., Ross, J., and Armour, C. (2017). Evidence of the dissociative PTSD subtype: A systematic literature review of latent class and profile analytic studies of PTSD. J. Affect. Disorder. 213, 59–69. doi: 10.1016/j.jad.2017.02.004
Harricharan, S., McKinnon, M. C., and Lanius, R. A. (2021). How processing of sensory information from the internal and external worlds shape the perception and engagement with the world in the aftermath of trauma: Implications for PTSD. Front. Neurosci. 15:625490. doi: 10.3389/fnins.2021.625490
Harricharan, S., McKinnon, M. C., Tursich, M., Densmore, M., Frewen, P., Théberge, J., et al. (2019). Overlapping frontoparietal networks in response to oculomotion and traumatic autobiographical memory retrieval: Implications for eye movement desensitization and reprocessing. Eur. J. Psychotraumatol. 10:1586265. doi: 10.1080/20008198.2019.1586265
Harricharan, S., Nicholson, A. A., Densmore, M., Théberge, J., McKinnon, M. C., Neufeld, R. W. J., et al. (2017). Sensory overload and imbalance: Resting-state vestibular connectivity in PTSD and its dissociative subtype. Neuropsychologia 106, 169–178. doi: 10.1016/j.neuropsychologia.2017.09.010
Harricharan, S., Rabellino, D., Frewen, P. A., Densmore, M., Théberge, J., McKinnon, M. C., et al. (2016). fMRI functional connectivity of the periaqueductal gray in PTSD and its dissociative subtype. Brain Behav. 6:e00579. doi: 10.1002/brb3.579
Heidt, J. M., Marx, B. P., and Forsyth, J. P. (2005). Tonic immobility and childhood sexual abuse: A preliminary report evaluating the sequela of rape-induced paralysis. Behav. Res. Ther. 43, 1157–1171. doi: 10.1016/j.brat.2004.08.005
Heim, C., Young, L. J., Newport, D. J., Mletzko, T., Miller, A. H., and Nemeroff, C. B. (2009). Lower CSF oxytocin concentrations in women with a history of childhood abuse. Mol. Psychiatry 14, 954–958. doi: 10.1038/mp.2008.112
Henderson, P., Rosen, D., and Mascaro, N. (2007). Empirical study on the healing nature of mandalas. Psychol. Aesthet. Creat. Arts 1, 148–154. doi: 10.1037/1931-3896.1.3.148
Hernandez, E., and Das, J. M. (2020). Neuroanatomy, nucleus vestibular. Florida, FL: StatPearls Publishing.
Herrmann, C. S., and Knight, R. T. (2001). Mechanisms of human attention: Event-related potentials and oscillations. Neurosci. Biobehav. Rev. 25, 465–476. doi: 10.1016/S0149-7634(01)00027-6
Highstein, S. M., and Holstein, G. R. (2006). “The anatomy of the vestibular nuclei,” in Neuroanatomy of the oculomotor system, Vol. 151, ed. J. A. Büttner-Ennever (Amsterdam: Elsevier), 108–123.
Hitier, M., Besnard, S., Smith, P. F., and Pereira, A. (2014). Vestibular pathways involved in cognition. Front. Integr. Neurosci. 8:59. doi: 10.3389/fnint.2014.00059
Hofer, M. A. (1970). Cardiac and respiratory function during sudden prolonged immobility in wild rodents. Psychos. Med. 32, 633–648. doi: 10.1097/00006842-197011000-00008
Hoffe, M. E., and Balaban, C. D. (2011). Vestibular rehabilitation: Ready for the Mainstream. NeuroRehabilitation 29, 125–125. doi: 10.3233/NRE-2011-0686
Holstein, G. R. (2012). “The vestibular system,” in The human nervous system, 3rd Edn, eds J. K. Mai and G. Paxinos (Amsterdam: Elsevier), 1239–1269.
Horn, A. K., and Adamzyk, C. (2012). “Reticular formation: Eye movements, gaze and blinks,” in The human nervous system, 3rd Edn, eds J. K. Mai and G. Paxinos (Amsterdam: Elsevier), 328–366. doi: 10.1016/S0079-6123(08)00616-X
Huber, J., Ruehl, M., Flanagin, V., and zu Eulenburg, P. (2021). Delineating neural responses and functional connectivity changes during vestibular and nociceptive stimulation reveal the uniqueness of cortical vestibular processing. Brain Structure Funct. 227, 779–791. doi: 10.1007/s00429-021-02394-6
Hurley, R. A., Flashman, L. A., Chow, T. W., and Taber, K. H. (2010). The brainstem: Aanatomy, assessment, and clinical syndromes. J. Neuropsychiatry Clin. Neurosci. 22, iv–7. doi: 10.1176/jnp.2010.22.1.iv
Ibitoye, R. T., Mallas, E.-J., Bourke, N. J., Kaski, D., Bronstein, A. M., and Sharp, D. J. (2022). The human vestibular cortex: Functional anatomy of OP2, its connectivity and the effect of vestibular disease. Cereb. Cortex, bhac085, 1–16. doi: 10.1093/cercor/bhac085
Jamon, M. (2014). The development of vestibular system and related functions in mammals: Impact of gravity. Front. Integr. Neurosci. 8:11. doi: 10.3389/fnint.2014.00011
Janet, P. (1889). L’automatisme psychologique: Essay de psychologie experimentale sur les formes inferieures de l’activite humaine, Vol. 2. London: Forgotten Books.
Jensen, O., and Mazaheri, A. (2010). Shaping functional architecture by oscillatory alpha activity: Gating by inhibition. Front. Hum. Neurosci. 4:186. doi: 10.3389/fnhum.2010.00186
Jones, S. R., and Sliva, D. D. (2020). Is alpha asymmetry a byproduct or cause of spatial attention? New evidence alpha neurofeedback controls measures of spatial attention. Neuron 105, 404–406. doi: 10.1016/j.neuron.2019.12.033
Jönsson, E. H., Kotilahti, K., Heiskala, J., Wasling, H. B., Olausson, H., Croy, I., et al. (2018). Affective and non-affective touch evoke differential brain responses in 2-month-old infants. NeuroImage 169, 162–171. doi: 10.1016/j.neuroimage.2017.12.024
Joseph, R. Y., Casteleijn, D., van der Linde, J., and Franzsen, D. (2021). Sensory modulation dysfunction in child victims of trauma: A scoping review. J. Child Adolesc. Trauma 14, 455–470. doi: 10.1007/s40653-020-00333-x
Kar, N. (2011). Cognitive behavioral therapy for the treatment of post-traumatic stress disorder: A review. Neuropsychiatr. Dis. Treatment 7, 167–181. doi: 10.2147/NDT.S10389
Keay, K. A., and Bandler, R. (2001). Parallel circuits mediating distinct emotional coping reactions to different types of stress. Neurosci. Biobehav. Rev. 25, 669–678. doi: 10.1016/S0149-7634(01)00049-5
Kemp, K., Signal, T., Botros, H., Taylor, N., and Prentice, K. (2014). Equine facilitated therapy with children and adolescents who have been sexually abused: A program evaluation study. J. Child Fam. Stud. 23, 558–566. doi: 10.1007/s10826-013-9718-1
Kendall, E., and Maujean, A. (2015). Horse play: A brief psychological intervention for disengaged youths. J. Creat. Ment. Health 10, 46–61. doi: 10.1080/15401383.2014.962720
Kind, S., and Otis, J. D. (2019). The interaction between chronic pain and PTSD. Curr. Headache Pain Rep. 23, 1–7. doi: 10.1007/s11916-019-0828-3
Kirsch, L. P., Besharati, S., Papadaki, C., Crucianelli, L., Bertagnoli, S., Ward, N., et al. (2020). Damage to the right insula disrupts the perception of affective touch. eLife 9:e47895. doi: 10.7554/eLife.47895
Klasen, M., Chen, Y. H., and Mathiak, K. (2012). Multisensory emotions: Perception, combination and underlying neural processes. Rev. Neurosci. 23, 381–302. doi: 10.1515/revneuro-2012-0040
Kleindienst, N., Limberger, M. F., Ebner-Priemer, U. W., Keibel-Mauchnik, J., Dyer, A., Berger, M., et al. (2011). Dissociation predicts poor response to dialectical behavioral therapy in female patients with borderline personality disorder. J. Pers. Disorder. 25, 432–447. doi: 10.1521/pedi.2011.25.4.432
Klemm, W. R. (1971). Neurophysiologic studies of the immobility reflex (“animal hypnosis”). Neurosci. Res. 4, 165–212. doi: 10.1016/b978-0-12-512504-8.50011-1
Kluetsch, R. C., Ros, T., Théberge, J., Frewen, P. A., Calhoun, V. D., Schmahl, C., et al. (2014). Plastic modulation of PTSD resting-state networks and subjective wellbeing by EEG neurofeedback. Acta Psychiatr. Scand. 130, 123–136. doi: 10.1111/acps.12229
Koelsch, S., Jacobs, A. M., Menninghaus, W., Liebal, K., Klann-Delius, G., von Scheve, C., et al. (2015). The quartet theory of human emotions: An integrative and neurofunctional model. Phys. Life Rev. 13, 1–27. doi: 10.1016/j.plrev.2015.03.001
Koopman, C., Carrion, V., Butler, L. D., Sudhakar, S., Palmer, L., and Steiner, H. (2004). Relationships of dissociation and childhood abuse and neglect with heart rate in delinquent adolescents. J. Trauma. Stress 17, 47–54. doi: 10.1023/B:JOTS.0000014676.83722.35
Kosinski, R. J., Lee, H. S., and Mihailoff, G. A. (1988). A double retrograde fluorescent tracing analysis of dorsal column nuclear projections to the basilar pontine nuclei, thalamus, and superior colliculus. Neurosci. Lett. 85, 40–46. doi: 10.1016/0304-3940(88)90425-9
Koutsikou, S., Crook, J. J., Earl, E. V., Leith, J. L., Watson, T. C., Lumb, B. M., et al. (2014). Neural substrates underlying fear-evoked freezing: The periaqueductal grey-cerebellar link. J. Physiol. 592, 2197–2213. doi: 10.1113/jphysiol.2013.268714
Kozlowska, K., Walker, P., McLean, L., and Carrive, P. (2015). Fear and the defense cascade: Clinical implications and management. Harvard Rev. Psychiatry 23, 263–287. doi: 10.1097/HRP.0000000000000065
Krammer, S., Kleim, B., Simmen-Janevska, K., and Maercker, A. (2016). Childhood trauma and complex posttraumatic stress disorder symptoms in older adults: A study of direct effects and social-interpersonal factors as potential mediators. J. Trauma Dissoc. 17, 593–607. doi: 10.1080/15299732.2014.991861
Kratzer, L., Heinz, P., Pfitzer, F., Padberg, F., Jobst, A., and Schennach, R. (2018). Mindfulness and pathological dissociation fully mediate the association of childhood abuse and PTSD symptomatology. Eur. J. Trauma Dissoc. 2, 593–607. doi: 10.1016/j.ejtd.2017.06.004
Kurjak, A., Tikvica, A., Stanojevic, M., Miskovic, B., Ahmed, B., Azumendi, G., et al. (2008). The assessment of fetal neurobehavior by three-dimensional and four-dimensional ultrasound. J. Maternal Fetal Neonatal. Med. 21, 675–684. doi: 10.1080/14767050802212166
LaBerge, D., Carter, M., and Brown, V. (1992). A network simulation of thalamic circuit operations in selective attention. Neural. Comput. 4, 318–331. doi: 10.1162/neco.1992.4.3.318
Lackner, J. R. (1988). Some proprioceptive influences on the perceptual representation of body shape and orientation. Brain 111, 281–287. doi: 10.1093/brain/111.2.281
Lacquaniti, F., Bosco, G., Gravano, S., Indovina, I., la Scaleia, B., Maffei, V., et al. (2015). Gravity in the brain as a reference for space and time perception. Multisens. Res. 28, 397–426. doi: 10.1163/22134808-00002471
Lane, S. J., Mailloux, Z., Schoen, S., Bundy, A., May-Benson, T. A., Parham, L. D., et al. (2019). Neural foundations of Ayres Sensory Integration. Brain Sci. 9:153. doi: 10.3390/brainsci9070153
Langmuir, J. I., Kirsh, S. G., and Classen, C. C. (2012). A pilot study of body-oriented group psychotherapy: Adapting sensorimotor psychotherapy for the group treatment of trauma. Psychol. Trauma Theory Res. Pract. Policy 4, 214–220. doi: 10.1037/a0025588
Lanius, R. A., Bluhm, R. L., and Frewen, P. A. (2011). How understanding the neurobiology of complex post-traumatic stress disorder can inform clinical practice: A social cognitive and affective neuroscience approach. Acta Psychiatr. Scand. 124, 331–348. doi: 10.1111/j.1600-0447.2011.01755.x
Lanius, R. A., Bluhm, R. L., Coupland, N. J., Hegadoren, K. M., Rowe, B., Théberge, J., et al. (2010a). Default mode network connectivity as a predictor of post-traumatic stress disorder symptom severity in acutely traumatized subjects. Acta Psychiatr. Scand. 121, 33–40. doi: 10.1111/j.1600-0447.2009.01391.x
Lanius, R. A., Rabellino, D., Boyd, J. E., Harricharan, S., Frewen, P. A., and McKinnon, M. C. (2017). The innate alarm system in PTSD: Conscious and subconscious processing of threat. Curr. Opin. Psychol. 14, 109–115. doi: 10.1016/j.copsyc.2016.11.006
Lanius, R. A., Terpou, B. A., and McKinnon, M. C. (2020). The sense of self in the aftermath of trauma: Lessons from the default mode network in posttraumatic stress disorder. Eur. J. Psychotraumatol. 11:1807703. doi: 10.1080/20008198.2020.1807703
Lanius, R. A., Vermetten, E., Loewenstein, R. J., Brand, B., Christian, S., Bremner, J. D., et al. (2010b). Emotion modulation in PTSD: Clinical and neurobiological evidence for a dissociative subtype. Am. J. Psychiatry 167, 640–647. doi: 10.1176/appi.ajp.2009.09081168
Lanius, R. A., Williamson, P. C., Bluhm, R. L., Densmore, M., Boksman, K., Neufeld, R. W. J., et al. (2005). Functional connectivity of dissociative responses in posttraumatic stress disorder: A functional magnetic resonance imaging investigation. Biol. Psychiatry 57, 873–884. doi: 10.1016/j.biopsych.2005.01.011
LeBois, L. A. M., Harnett, N. G., van Rooij, S. J. H., Ely, T. D., Jovanovic, T., Bruce, S. E., et al. (2022). Persistent dissociation and its neural correlates in predicting outcomes after trauma exposure. Am. J. Psychiatry 179, 661–672. doi: 10.1176/appi.ajp.21090911
Lecanuet, J., and Schaal, B. (2002). Sensory performances in the human foetus: A brief summary of research. Intellectica 34, 29–56. doi: 10.3406/intel.2002.1072
Lenggenhager, B., and Lopez, C. (2015). “Vestibular contributions to the sense of body, self, and others,” in Open MIND, eds T. Metzinger and J. M. Windt (Frankfurt: MIND Group), 1–38. doi: 10.5167/uzh-139675
Lenggenhager, B., Pazzaglia, M., Scivoletto, G., Molinari, M., and Aglioti, S. M. (2012). The sense of the body in individuals with spinal cord injury. PLoS One 7:e50757. doi: 10.1371/journal.pone.0050757
Lenggenhager, B., Smith, S. T., and Blanke, O. (2006). Functional and neural mechanisms of embodiment: Importance of the vestibular system and the temporal parietal junction. Rev. Neurosci. 17, 643–657. doi: 10.1515/REVNEURO.2006.17.6.643
Lenggenhager, B., Tadi, T., Metzinger, T., and Blanke, O. (2007). Video ergo sum: Manipulating bodily self-consciousness. Science 317, 1096–1099. doi: 10.1126/science.1143439
Lentini, J. A., and Knox, M. S. (2015). Equine-facilitated psychotherapy with children and adolescents: An update and literature review. J. Creat. Ment. Health 10, 278–305. doi: 10.1080/15401383.2015.1023916
Leong, A. T. L., Gu, Y., Chan, Y. S., Zheng, H., Dong, C. M., Chan, R. W., et al. (2019). Optogenetic fMRI interrogation of brain-wide central vestibular pathways. Proc. Natl. Acad. Sci. U.S.A. 116, 10122–10129. doi: 10.1073/pnas.1812453116
Levine, P. A. (2010). In an unspoken voice: How the body releases trauma and restores goodness. Berkeley, CA: North Atlantic Books.
Liddell, B., Brown, K., Kemp, A., Barton, M., Das, P., Peduto, A., et al. (2005). A direct brainstem-amygdala-cortical ‘alarm’ system for subliminal signals of fear. NeuroImage 24, 235–243. doi: 10.1016/j.neuroimage.2004.08.016
Lipovsek, M., and Wingate, R. J. T. (2018). Conserved and divergent development of brainstem vestibular and auditory nuclei. eLife 7:e40232. doi: 10.7554/eLife.40232
Liu, D., Diorio, J., Day, J. C., Francis, D. D., and Meaney, M. J. (2000). Maternal care, hippocampal synaptogenesis and cognitive development in rats. Nat. Neurosci. 3, 799–806. doi: 10.1038/77702
Liu, X., Chen, C., Liu, Y., Wang, Z., Huang, K., Wang, F., et al. (2018). Gentle handling attenuates innate defensive responses to visual threats. Front. Behav. Neurosci. 12:239. doi: 10.3389/fnbeh.2018.00239
Llinás, R. (1970). “Neuronal operations in cerebellar transactions,” in The neurosciences: Second study program, ed. F. O. Schmitt (New York, NY: Rockefeller Univ Press), 409–426.
Löken, L. S., Wessberg, J., Morrison, I., McGlone, F., and Olausson, H. (2009). Coding of pleasant touch by unmyelinated afferents in humans. Nat. Neurosci. 12, 547–548. doi: 10.1038/nn.2312
Longo, M. R., Schüür, F., Kammers, M. P. M., Tsakiris, M., and Haggard, P. (2008). What is embodiment? A psychometric approach. Cognition 107, 978–998. doi: 10.1016/j.cognition.2007.12.004
Longo, M. R., Schüür, F., Kammers, M. P. M., Tsakiris, M., and Haggard, P. (2009). Self awareness and the body image. Acta Psychol. 132, 978–998. doi: 10.1016/j.actpsy.2009.02.003
Lopes da Silva, F. (1991). Neural mechanisms underlying brain waves: From neural membranes to networks. Electroencephalogr. Clin. Neurophysiol. 72, 81–93. doi: 10.1016/0013-4694(91)90044-5hu
Lopez, C. (2016). The vestibular system: Balancing more than just the body. Curr. Opin. Neurol. 29, 74–83. doi: 10.1097/WCO.0000000000000286
Lopez, C., and Blanke, O. (2011). The thalamocortical vestibular system in animals and humans. Brain Res. Rev. 67, 119–146. doi: 10.1016/j.brainresrev.2010.12.002
Lopez, C., Nakul, E., Preuss, N., Elzière, M., and Mast, F. W. (2018). Distorted own-body representations in patients with dizziness and during caloric vestibular stimulation. J. Neurol. 265, 86–94. doi: 10.1007/s00415-018-8906-8
Lopez, C., Schreyer, H. M., Preuss, N., and Mast, F. W. (2012). Vestibular stimulation modifies the body schema. Neuropsychologia 50, 1830–1837. doi: 10.1016/j.neuropsychologia.2012.04.008
López-Solà, M., Geuter, S., Koban, L., Coan, J. A., and Wager, T. D. (2019). Brain mechanisms of social touch-induced analgesia in females. Pain 160, 2072–2085. doi: 10.1097/j.pain.0000000000001599
Lovick, T. A., and Bandler, R. (2005). “The organisation of the midbrain periaqueductal grey and the integration of pain behaviours,” in The neurobiology of pain, eds S. P. Hunt and M. Koltzenburg (Oxford: Oxford University Press), 267–287.
Lowenstein, K. (2018). Shutting down the trauma to prison pipeline: Early, appropriate care for child-welfare involved youth. Boston, MA: Citizens for Juvenile Justice.
Luan, H., Gdowski, M. J., Newlands, S. D., and Gdowski, G. T. (2013). Convergence of vestibular and neck proprioceptive sensory signals in the cerebellar interpositus. J. Neurosci. 33, 1198–1210. doi: 10.1523/JNEUROSCI.3460-12.2013
Lubeck, J., Schaaf, R. C., and Dumont, R. L. (2020). Effects of rocking on attention and arousal. Philadelphia, PA: Thomas Jefferson University.
Lud?scher, C. A., Bohus, M., Lieb, K., Philipsen, A., Jochims, A., and Schmahl, C. (2007). Elevated pain thresholds correlate with dissociation and aversive arousal in patients with dissociation and aversive arousal in patients with borderline personality disorder. Psychiatry Res. 149, 291–296. doi: 10.1016/j.psychres.2005.04.009
Lyons-Ruth, K., Dutra, L., Schuder, M. R., and Bianchi, I. (2006). From infant attachment disorganization to adult dissociation: Relational adaptations or traumatic experiences? Psychiatr. Clin. North Am. 29, 63–86. doi: 10.1016/j.psc.2005.10.011
Macauda, G., Bertolini, G., Palla, A., Straumann, D., Brugger, P., and Lenggenhager, B. (2015). Binding body and self in visuo-vestibular conflicts. Eur. J. Neurosci. 41, 810–817. doi: 10.1111/ejn.12809
Maclean, P. D. (1985). Evolutionary psychiatry and the triune brain. Psychol. Med. 15, 219–221. doi: 10.1017/S0033291700023485
Maier, A., Gieling, C., Heinen-Ludwig, L., Stefan, V., Schultz, J., Gunturkun, O., et al. (2020). Association of childhood maltreatment with interpersonal distance and social touch preferences in adulthood. Am. J. Psychiatry 177, 37–46. doi: 10.1176/appi.ajp.2019.19020212
Malchiodi, C. A. (2020). Trauma and expressive arts therapy: Brain, body, and imagination in the healing process. New York, NY: Guilford Publications.
Malejko, K., Abler, B., Plener, P. L., and Straub, J. (2017). Neural correlates of psychotherapeutic treatment of post-traumatic stress disorder: A systematic literature review. Front. Psychiatry 8:85. doi: 10.3389/fpsyt.2017.00085
Manzoni, D., Pompeiano, O., Barnes, C. D., Stampacchia, G., and d’Ascanio, P. (1989). Convergence and interaction of neck and macular vestibular inputs on locus coeruleus and subcoeruleus neurons. Pfugers Arch. 413, 580–598. doi: 10.1007/bf00581807
Manzotti, A., Cerritelli, F., Esteves, J. E., Lista, G., Lombardi, E., la Rocca, S., et al. (2019). Dynamic touch reduces physiological arousal in preterm infants: A role for c-tactile afferents? Dev. Cogn. Neurosci. 39:100703. doi: 10.1016/j.dcn.2019.100703
Marcus, S., Marquis, P., and Sakai, C. (2004). Three- and 6-month follow-up of EMDR treatment of PTSD in an HMO setting. Int. J. Stress Manag. 11, 195–208. doi: 10.1037/1072-5245.11.3.195
Marks, I., Lovell, K., Noshirvani, H., Livanou, M., and Thrasher, S. (1998). Treatment of posttraumatic stress disorder by exposure and/or cognitive restructuring: A controlled study. Arch. Gen. Psychiatry 55, 317–325. doi: 10.1001/archpsyc.55.4.317
Marshall, A. G., and McGlone, F. P. (2020). Affective Touch: The enigmatic spinal pathway of the C-Tactile afferent. Neurosci. Insights 15, 1–5. doi: 10.1177/2633105520925072
Marshall, E. M., and Frazier, P. A. (2019). Understanding posttrauma reactions within an attachment theory framework. Curr. Opin. Psychol. 25, 167–171. doi: 10.1016/j.copsyc.2018.08.001
Marx, B. P., Forsyth, J. P., Gallup, G. G., Fusé, T., and Lexington, J. M. (2008). Tonic immobility as an evolved predator defense: Implications for sexual assault survivors. Clin. Psychol. Sci. Pract. 15, 74–90. doi: 10.1111/j.1468-2850.2008.00112.x
Marx, V., and Nagy, E. (2015). Fetal behavioural responses to maternal voice and touch. PLoS One 10:e0129118. doi: 10.1371/journal.pone.0129118
Mast, F. W., Preuss, N., Hartmann, M., and Grabherr, L. (2014). Spatial cognition, body representation and affective processes: The role of vestibular information beyond ocular reflexes and control of posture. Front. Integr. Neurosci. 8:44. doi: 10.3389/fnint.2014.00044
May, P. J. (2006). The mammalian superior colliculus: Laminar structure and connections. Prog. Brain Res. 151, 321–378. doi: 10.1016/S0079-6123(05)51011-2
May, P. J., and Porter, J. D. (1992). The laminar distribution of macaque tectobulbar and tectospinal neurons. Vis. Neurosci. 8, 257–276. doi: 10.1017/S0952523800002911
McCall, A. A., Miller, D. M., and Yates, B. J. (2017). Descending influences on vestibulospinal and vestibulosympathetic reflexes. Front. Neurol. 8:112. doi: 10.3389/fneur.2017.00112
McCullough, L., Risley-Curtiss, C., and Rorke, J. (2015). Equine facilitated psychotherapy: A pilot study of effect on posttraumatic stress symptoms in maltreated youth. J. Infant Child Adolesc. Psychother. 14, 158–173. doi: 10.1080/15289168.2015.1021658
McGeoch, P. D., Williams, L. E., Song, T., Lee, R. R., Huang, M., and Ramachandran, V. S. (2009). Post-stroke tactile allodynia and its modulation by vestibular stimulation: A MEG case study. Acta Neurol. Scand. 119, 404–409. doi: 10.1111/j.1600-0404.2008.01106.x
McGlone, F., Kelly, E. F., Trulsson, M., Francis, S. T., Westling, G., and Bowtell, R. (2002). Functional neuroimaging studies of human somatosensory cortex. Behav. Brain Res. 135, 147–158. doi: 10.1016/S0166-4328(02)00144-4
McGlone, F., Vallbo, A. B., Olausson, H., Loken, L., and Wessberg, J. (2007). Discriminative touch and emotional touch. Can. J. Exp. Psychol. 61, 173–183. doi: 10.1037/cjep2007019
McGlone, F., Wessberg, J., and Olausson, H. (2014). Discriminative and affective touch: Sensing and feeling. Neuron 82, 737–755. doi: 10.1016/j.neuron.2014.05.001
McGreevy, S., and Boland, P. (2020). Sensory-based interventions with adult and adolescent trauma survivors: An integrative review of the occupational therapy literature. Irish J. Occup. Ther. 48, 31–54. doi: 10.1108/IJOT-10-2019-0014
McIntyre, S., and Seizova-Cajic, T. (2007). Neck muscle vibration in full cues affects pointing. J. Vis. 7, 1–8. doi: 10.1167/7.5.9
McMillen, J. C., North, C. S., and Smith, E. M. (2000). What parts of PTSD are normal: Intrusion, avoidance, or arousal? Data from the Northridge, California, earthquake. J. Trauma. Stress 13, 57–75. doi: 10.1023/A:1007768830246
Meaney, M. J. (2001). Maternal care, gene expression, and the transmission of individual differences in stress reactivity across generations. Annu. Rev. Neurosci. 24, 1161–1192. doi: 10.1146/annurev.neuro.24.1.1161
Mendes, D. D., Mello, M. F., Ventura, P., Passarela, C. D. M., and Mari, J. D. J. (2008). A systematic review on the effectiveness of cognitive behavioral therapy for posttraumatic stress disorder. Int. J. Psychiatry Med. 38, 241–259. doi: 10.2190/PM.38.3.b
Menon, V. (2015). “Salience Network,” in Brain mapping: An encyclopedic reference, Vol. 2, ed. A. W. Toga (Elsevier: Academic Press), 597–611.
Menon, V., and Uddin, L. Q. (2010). Saliency, switching, attention and control: A network model of insula function. Brain Struct.Funct. 214, 655–667. doi: 10.1007/s00429-010-0262-0
Meredith, M. A., Wallace, M. T., and Stein, B. E. (1992). Visual, auditory and somatosensory convergence in output neurons of the cat superior colliculus: Multisensory properties of the tecto-reticulo-spinal projection. Exp. Brain Res. 88, 181–186. doi: 10.1007/BF02259139
Merker, B. (2007). Consciousness without a cerebral cortex: A challenge for neuroscience and medicine. Behav. Brain Sci. 30, 63–81. doi: 10.1017/S0140525X07000891
Metzinger, T. (2013). Why are dreams interesting for philosophers? The example of minimal phenomenal selfhood, plus an agenda for future research. Front. Psychol. 4:746. doi: 10.3389/fpsyg.2013.00746
Michelson, L., June, K., Vives, A., Testa, S., and Marchione, N. (1998). The role of trauma and dissociation in cognitive-behavioral psychotherapy outcome and maintenance for panic disorder with agoraphobia. Behav. Res. Ther. 36, 1011–1050. doi: 10.1016/S0005-7967(98)00073-4
Midolo, L. R., Santoro, G., Ferrante, E., Pellegriti, P., Russo, S., Costanzo, A., et al. (2020). Childhood trauma, attachment and psychopathology: A correlation network approach. Mediterr. J. Clin. Psychol. 8, 1–25. doi: 10.6092/2282-1619/mjcp-2418
Mikulincer, M., Florian, V., and Weller, A. (1993). Attachment styles, coping strategies, and posttraumatic psychological distress: The impact of the Gulf War in Israel. J. Personal. Soc. Psychol. 64, 817–826. doi: 10.1037/0022-3514.64.5.817
Mikulincer, M., Horesh, N., Eilati, I., and Kotler, M. (1999). The association between adult attachment style and mental health in extreme life-endangering conditions. Personal. Individual Differ. 27, 831–842. doi: 10.1016/S0191-8869(99)00032-X
Mikulincer, M., Shaver, P. R., and Solomon, Z. (2015). “An attachment perspective on traumatic and posttraumatic reactions,” in Future directions in post-traumatic stress disorder: Prevention, diagnosis, and treatment, eds M. Safir, H. Wallach, and A. Rizzo (New York: Springer), 79–96. doi: 10.1007/978-1-4899-7522-5_4
Miller, L. J., Anzalone, M. E., Lane, S. J., Cermak, S. A., and Osten, E. T. (2007). Concept evolution in sensory integration: A proposed nosology for diagnosis. Am. J. Occup. Ther. 61, 135–140. doi: 10.5014/ajot.61.2.135
Milligan-Saville, J. S., Paterson, H. M., Harkness, E. L., Marsh, A. M., Dobson, M., Kemp, R. I., et al. (2017). The amplification of common somatic symptoms by posttraumatic stress disorder in firefighters. J. Trauma. Stress 30, 142–148. doi: 10.1002/jts.22166
Montonen, O., Ezer, S., Saarialho-Kere, U. K., Herva, R., Karjalainen-Lindsberg, M. L., Kaitila, I., et al. (1998). The gene defective in anhidrotic ectodermal dysplasia is expressed in the developing epithelium, neuroectoderm, thymus, and bone. J. Histochem. Cytochem. 46, 281–289. doi: 10.1177/002215549804600301
Mora, C., Velásquez, C., and Martino, J. (2019). The neural pathway midline crossing theory: A historical analysis of Santiago Rámon y Cajal’s contribution on cerebral localization and on contralateral forebrain organization. Neurosurg. Focus 47, 1–6. doi: 10.3171/2019.6.FOCUS19341
Morrison, I. (2016). Keep calm and cuddle on: Social touch as a stress buffer. Adapt. Hum. Behav. Physiol. 2, 344–362. doi: 10.1007/s40750-016-0052-x
Morrison, I., Björnsdotter, M., and Olausson, H. (2011). Vicarious responses to social touch in posterior insular cortex are tuned to pleasant caressing speeds. J. Neurosci. 31, 9554–9562. doi: 10.1523/JNEUROSCI.0397-11.2011
Morrison, I., Löken, L. S., and Olausson, H. (2010). The skin as a social organ. Exp. Brain Res. 204, 305–314. doi: 10.1007/s00221-009-2007-y
Muller, R. T., and Rosenkranz, S. E. (2009). Attachment and treatment response among adults in inpatient treatment for posttraumatic stress disorder. Psychotherapy 46, 82–96. doi: 10.1037/a0015137
Murakami, D. M., Erkman, L., Hermanson, O., Rosenfeld, M. G., and Fuller, C. A. (2002). Evidence for vestibular regulation of autonomic functions in a mouse genetic model. Proc. Natl. Acad. Sci. U.S.A. 99, 17078–17082. doi: 10.1073/pnas.252652299
Myers, A. J., Williams, L., Gatt, J. M., McAuley-Clark, E. Z., Dobson-Stone, C., Schofield, P. R., et al. (2014). Variation in the oxytocin receptor gene is associated with increased risk for anxiety, stress and depression in individuals with a history of exposure to early life stress. J. Psychiatric Res. 59, 93–100. doi: 10.1016/j.jpsychires.2014.08.021
Nandi, R., and Luxon, L. M. (2008). Development and assessment of the vestibular system. Int. J. Audiol. 47, 566–577. doi: 10.1080/14992020802324540
Naste, T. M., Price, M., Karol, J., Martin, L., Murphy, K., Miguel, J., et al. (2018). Equine facilitated therapy for complex trauma (EFT-CT). J. Child Adolesc. Trauma 11, 289–303. doi: 10.1007/s40653-017-0187-3
Neuper, C., and Pfurtscheller, G. (2001). Event-related dynamics of cortical rhythms: Frequency-specific features and functional correlates. Int. J. Psychophysiol. 43, 41–58. doi: 10.1016/S0167-8760(01)00178-7
Nevins, R., Finch, S., Hickling, E. J., and Barnett, S. D. (2013). The Saratoga WarHorse project: A case study of the treatment of psychological distress in a veteran of Operation Iraqi Freedom. Adv. Mind Body Med. 27, 22–25.
Nicholson, A. A., Friston, K. J., Zeidman, P., Harricharan, S., McKinnon, M. C., Densmore, M., et al. (2017). Dynamic causal modeling in PTSD and its dissociative subtype: Bottom–up versus top–down processing within fear and emotion regulation circuitry. Hum. Brain Mapp. 38, 5551–5561. doi: 10.1002/hbm.23748
Nicholson, A. A., Ros, T., Densmore, M., Frewen, P. A., Neufeld, R. W. J., Théberge, J., et al. (2020a). A randomized, controlled trial of alpha-rhythm EEG neurofeedback in posttraumatic stress disorder: A preliminary investigation showing evidence of decreased PTSD symptoms and restored default mode and salience network connectivity using fMRI. NeuroImage 28, 1–15. doi: 10.1016/j.nicl.2020.102490
Nicholson, A. A., Ros, T., Frewen, P. A., Densmore, M., Théberge, J., Kluetsch, R. C., et al. (2016). Alpha oscillation neurofeedback modulates amygdala complex connectivity and arousal in posttraumatic stress disorder. NeuroImage 12, 506–516. doi: 10.1016/j.nicl.2016.07.006
Nicholson, A. A., Ros, T., Jetly, R., and Lanius, R. A. (2020b). Regulating posttraumatic stress disorder symptoms with neurofeedback: Regaining control of the mind. J. Mil. Veteran Fam. Health 6, 3–15. doi: 10.3138/jmvfh.2019-0032
Nijenhuis, E. R. S., Spinhoven, P., van Dyck, R., van der Hart, O., and Vanderlinden, J. (1998a). Degree of somatoform and psychological dissociation in dissociative disorder is correlated with reported trauma. J. Trauma. Stress 11, 711–730. doi: 10.1023/A:1024493332751
Nijenhuis, E. R. S., Vanderlinden, J., van der Hart, O., Spinhoven, P., and van Dyck, R. (1998b). Somatoform dissociative symptoms as related to animal defensive reactions to predatory imminence and injury. J. Abnorm. Psychol. 107, 63–73. doi: 10.1037/0021-843X.107.1.63
Northoff, G., Heinzel, A., de Greck, M., Bermpohl, F., Dobrowolny, H., and Panksepp, J. (2006). Self-referential processing in our brain: A meta-analysis of imaging studies on the self. Neuroimage 31, 440–457. doi: 10.1016/j.neuroimage.2005.12.002
O’Haire, M. E., Guérin, N. A., and Kirkham, A. C. (2015). Animal-assisted intervention for trauma: A systematic literature review. Front. Psychol. 6:1121. doi: 10.3389/fpsyg.2015.01121
Odutola, A. B. (1977). On the location of reticular neurons projecting to the cuneo-gracile nuclei in the rat. Exp. Neurol. 54, 54–59. doi: 10.1016/0014-4886(77)90234-5
Ogawa, J. R., Sroufe, L. A., Weinfield, N. S., Carlson, E. A., and Egeland, B. (1997). Development and the fragmented self: Longitudinal study of dissociative symptomatology in a nonclinical sample. Dev. Psychopathol. 9, 855–879. doi: 10.1017/s0954579497001478
Ogden, P., Pain, C., and Fisher, J. (2006). A sensorimotor approach to the treatment of trauma and dissociation. Psychiatric Clin. North Am. 29, 263–279. doi: 10.1016/j.psc.2005.10.012
Ogle, C. M., Rubin, D. C., and Siegler, I. C. (2015). The relation between insecure attachment and posttraumatic stress: Early life versus adulthood traumas. Psychol. Trauma 7, 324–332. doi: 10.1037/tra0000015
Olausson, H. W., Cole, J., Vallbo, Å, McGlone, F., Elam, M., Krämer, H. H., et al. (2008). Unmyelinated tactile afferents have opposite effects on insular and somatosensory cortical processing. Neurosci. Lett. 436, 128–132. doi: 10.1016/j.neulet.2008.03.015
Olausson, H., Lamarre, Y., Backlund, H., Morin, C., Wallin, B. G., Starck, G., et al. (2002). Unmyelinated tactile afferents signal touch and project to insular cortex. Nat. Neurosci. 5, 900–904. doi: 10.1038/nn896
Olausson, H., Wessberg, J., Morrison, I., McGlone, F., and Vallbo, Å (2010). The neurophysiology of unmyelinated tactile afferents. Neurosci. Biobehav. Rev. 34, 185–191. doi: 10.1016/j.neubiorev.2008.09.011
Olivé, I., Densmore, M., Harricharan, S., Théberge, J., McKinnon, M. C., and Lanius, R. (2018). Superior colliculus resting state networks in post-traumatic stress disorder and its dissociative subtype. Hum. Brain Mapp. 39, 563–574. doi: 10.1002/hbm.23865
Opacka-Juffry, J., and Mohiyeddini, C. (2012). Experience of stress in childhood negatively correlates with plasma oxytocin concentration in adult men. Stress 15, 1–10. doi: 10.3109/10253890.2011.560309
Oren, E., and Solomon, R. (2012). EMDR therapy: An overview of its development and mechanisms of action. Rev. Eur. Psychol. Appl. 62, 197–203. doi: 10.1016/j.erap.2012.08.005
Paetzold, R. L., Rholes, W. S., and Kohn, J. L. (2015). Disorganized attachment in adulthood: Theory, measurement, and implications for romantic relationships. Rev. Gen. Psychol. 19, 146–156. doi: 10.1037/gpr0000042
Palluel, E., Aspell, J. E., and Blanke, O. (2011). Leg muscle vibration modulates bodily self-consciousness: Integration of proprioceptive, visual, and tactile signals. J. Neurophysiol. 105, 2239–2247. doi: 10.1152/jn.00744.2010
Panksepp, J. (1998). Affective neuroscience: The foundations of human and animal emotions. Oxford: Oxford University Press.
Panksepp, J. (2000). “Fear and anxiety mechanisms of the brain: Clinical implications,” in Principles of Medical Biology (Vol. 14, Issue C), eds E. E. Bittar and N. Bittar (Stamford: JAI Press), 155–177. doi: 10.1016/S1569-2582(00)80010-0
Panksepp, J. (2005). Affective consciousness: Core emotional feelings in animals and humans. Conscious. Cogn. 14, 30–80. doi: 10.1016/j.concog.2004.10.004
Panksepp, J., and Northoff, G. (2009). The trans-species core SELF: The emergence of active cultural and neuro-ecological agents through self-related processing within subcortical-cortical midline networks. Conscious. Cogn. 18, 193–215. doi: 10.1016/j.concog.2008.03.002
Panksepp, J., and Trevarthen, C. (2009). “The neuroscience of emotion in music,” in Communicative musicality: Exploring the basis of human companionship, eds S. Malloch and C. Trevarthen (Oxford: Oxford University Press), 105–146.
Panksepp, J., Normansell, L., Cox, J. F., and Siviy, S. M. (1994). Effects of neonatal decortication on the social play of juvenile rats. Physiol. Behav. 56, 429–443. doi: 10.1016/0031-9384(94)90285-2
Pawling, R., Cannon, P. R., McGlone, F. P., and Walker, S. C. (2017). C-tactile afferent stimulating touch carries a positive affective value. PLoS One 12:e0173457. doi: 10.1371/journal.pone.0173457
Payne, P., Levine, P. A., and Crane-Godreau, M. A. (2015). Somatic experiencing: Using interoception and proprioception as core elements of trauma therapy. Front. Psychol. 6:1–18. doi: 10.3389/fpsyg.2015.00093
Pederson, D. R. (1975). The soothing effect of rocking as determined by the direction and frequency of movement. Can. J. Behav. Sci. 7, 237–243. doi: 10.1037/h0081911
Pereyra-Elías, R., Quigley, M. A., and Carson, C. (2022). To what extent does confounding explain the association between breastfeeding duration and cognitive development up to age 14? Findings from the UK Millennium Cohort Study. PLoS One 17, 237–243. doi: 10.1371/journal.pone.0267326
Perry, B. D., Pollard, R. A., Blakley, T. L., Baker, W. L., and Vigilante, D. (1995). Childhood trauma, the neurobiology of adaptation, and “use-dependent” development of the brain: How “states” become “traits. Infant Ment. Health J. 16, 271–291. doi: 10.1002/1097-0355(199524)16:4<271::AID-IMHJ2280160404<3.0.CO;2-B
Perry, B., and Hambrick, E. (2008). The neurosequential model of therapeutics. Reclaim. Child. Youth 17, 38–43.
Perry, N. E. (2019). Recognizing early childhood as a critical time for developing and supporting self-regulation. Metacogn. Learn. 14, 327–334. doi: 10.1007/s11409-019-09213-8
Peterson, B. W., Filion, M., Felpel, L. P., and Abzug, C. (1975). Responses of medial reticular neurons to stimulation of the vestibular nerve. Exp. Brain Res. 22, 335–350. doi: 10.1007/BF00234670
Pfaff, D. W., Martin, E. M., and Faber, D. (2012). Origins of arousal: Roles for medullary reticular neurons. Trends Neurosci. 35, 468–476. doi: 10.1016/j.tins.2012.04.008
Pfeiffer, C., Lopez, C., Schmutz, V., Duenas, J. A., Martuzzi, R., and Blanke, O. (2013). Multisensory origin of the subjective first-person perspective: Visual, tactile, and vestibular mechanisms. PLoS One 8:e61751. doi: 10.1371/journal.pone.0061751
Piaget, J. (1952). The origins of intelligence in children, Trans. M. Cook (New York: W.W. Norton and Co). doi: 10.1037/11494-000
Piontelli, A. (2015). Development of normal fetal movements: The last 15 weeks of gestation. Berlin: Springer e-Book. doi: 10.1007/978-88-470-5373-1
Pitman, R. K., Orr, S. P., Forgue, D. F., Jong, J. B., and Claiborn, J. M. (1987). Psychophysiologic assessment of posttraumatic stress disorder imagery in Vietnam combat veterans. Arch. Gen. Psychiatr. 44, 970–975. doi: 10.1001/archpsyc.1987.01800230050009
Pitman, R. K., Rasmusson, A. M., Koenen, K. C., Shin, L. M., Orr, S. P., Gilbertson, M. W., et al. (2012). Biological studies of post-traumatic stress disorder. Nat. Rev. Neurosci. 13, 769–787. doi: 10.1038/nrn3339
Pole, N. (2007). The psychophysiology of posttraumatic stress disorder: A meta-analysis. Psychol. Bull. 133, 725–746. doi: 10.1037/0033-2909.133.5.725
Pompeiano, O., Manzoni, D., Srivastava, U. C., and Stampacchia, G. (1984). Convergence and interaction of neck and macular vestibular inputs on reticulospinal neurons. Neuroscience 12, 111–128. doi: 10.1016/0306-4522(84)90142-8
Ponzo, S., Kirsch, L. P., Fotopoulou, A., and Jenkinson, P. M. (2018). Balancing body ownership: Visual capture of proprioception and affectivity during vestibular stimulation. Neuropsychologia 117, 311–321. doi: 10.1016/j.neuropsychologia.2018.06.020
Porro, C. A., and Carli, G. (1988). Immobilization and restraint effects on pain reactions in animals. Pain 32, 289–307. doi: 10.1016/0304-3959(88)90041-3
Portas, C. M., Rees, G., Howseman, A. M., Josephs, O., Turner, R., and Frith, C. D. (1998). A specific role for the thalamus in mediating the interaction of attention and arousal in humans. J. Neurosci. 18, 8979–8989. doi: 10.1523/jneurosci.18-21-08979.1998
Price, M., Spinazzola, J., Musicaro, R., Turner, J., Suvak, M., Emerson, D., et al. (2017). Effectiveness of an extended yoga treatment for women with chronic posttraumatic stress disorder. J. Alternat. Complement. Med. 23, 300–309. doi: 10.1089/acm.2015.0266
Putnam, F. W., Guroff, J. J., Silberman, E. K., Barban, L., and Post, R. M. (1986). The clinical phenomenology of multiple personality disorder: Review of 100 recent cases. J. Clin. Psychiatry 47, 285–293.
Rabellino, D., Thome, J., Densmore, M., Théberge, J., McKinnon, M. C., and Lanius, R. A. (2022). The vestibulocerebellum and the shattered self: A resting-state functional connectivity study in posttraumatic stress disorder and its dissociative subtype. Cerebellum. 1–15. doi: 10.1007/s12311-022-01467-4
Raio, C. M., Orederu, T. A., Palazzolo, L., Shurick, A. A., and Phelps, E. A. (2013). Cognitive emotion regulation fails the stress test. Proc. Natl. Acad. Sci. U.S.A. 110, 15139–15144. doi: 10.1073/pnas.1305706110
Raiser, T., Flanagin, V., Duering, M., van Ombergen, A., Ruehl, R., and zu Eulenburg, P. (2020). The human corticocortical vestibular network. NeuroImage 223:117362. doi: 10.1016/j.neuroimage.2020.117362
Rao, R. P. N., and Sejnowski, T. J. (2001). Spike-Timing-Dependent Hebbian Plasticity as Temporal Difference Learning. Neural Comput. 13, 2221–2237. doi: 10.1162/089976601750541787
Raussi, S., Lensink, B. J., Boissy, A., Pyykkönen, M., and Veissier, I. (2003). The effect of contact with conspecifics and humans on calves’ behaviour and stress responses. Anim. Welf. 12, 191–202.
Reddan, M. C., Young, H., Falkner, J., López-Solà, M., and Wager, T. D. (2020). Touch and social support influence interpersonal synchrony and pain. Soc. Cogn. Affect. Neurosci. 15, 1064–1075. doi: 10.1093/scan/nsaa048
Reinders, A. A. T. S., Nijenhuis, E. R. S., Paans, A. M. J., Korf, J., Willemsen, A. T. M., and den Boer, J. A. (2003). One brain, two selves. NeuroImage 20, 2119–2125. doi: 10.1016/j.neuroimage.2003.08.021
Reinders, A. A. T. S., and Veltman, D. J. (2021). Dissociative identity disorder: Out of theshadows at last? Br. J. Psychiatry 219, 413–414. doi: 10.1192/bjp.2020.168
Reynolds, S., Lane, S. J., and Mullen, B. (2015). Effects of deep pressure stimulation on physiological arousal. Am. J. Occup. Ther. 69, 6903350010p1–6903350010p5. doi: 10.5014/ajot.2015.015560.
Risser, H. J., Hetzel-Riggin, M. D., Thomsen, C. J., and McCanne, T. R. (2006). PTSD as a mediator of sexual revictimization: The role of reexperiencing, avoidance, and arousal symptoms. J. Trauma. Stress 19, 687–698. doi: 10.1002/jts.20156
Roche, D. N., Runtz, M. G., and Hunter, M. A. (1999). Adult attachment: A mediator between child sexual abuse and later psychological adjustment. J. Interpers. Violence 14, 187–207. doi: 10.1177/088626099014002006
Rogel, A., Loomis, A. M., Hamlin, E., Hodgdon, H., Spinazzola, J., and van der Kolk, B. A. (2020). The impact of neurofeedback training on children with developmental trauma: A randomized controlled study. Psychol. Trauma 12, 918–929. doi: 10.1037/tra0000648
Roley, S. S., Mailloux, Z., Miller-Kuhaneck, H., and Glennon, T. (2007). Understanding Ayres Sensory Integration§. OT Pract. CE1–CE8.
Romaiguère, P., Anton, J. L., Roth, M., Casini, L., and Roll, J. P. (2003). Motor and parietal cortical areas both underlie kinaesthesia. Cogn. Brain Res. 16, 74–82. doi: 10.1016/S0926-6410(02)00221-5
Ros, T., Frewen, P., Théberge, J., Michela, A., Kluetsch, R., Mueller, A., et al. (2017). Neurofeedback Tunes Scale-Free Dynamics in Spontaneous Brain Activity. Cereb. Cortex 27, 4911–4922. doi: 10.1093/cercor/bhw285
Rothbaum, B. O. (1997). A controlled study of eye movement desensitization and reprocessing in the treatment of posttraumatic stress disordered sexual assault victims. Bull. Menninger Clin. 61, 317–334.
Rubelowski, J. M., Menge, M., Distler, C., Rothermel, M., and Hoffmann, K. P. (2013). Connections of the superior colliculus to shoulder muscles of the rat: A dual tracing study. Front. Neuroanat. 7:17. doi: 10.3389/fnana.2013.00017
Rufer, M., Held, D., Cremer, J., Fricke, S., Moritz, S., Peter, H., et al. (2006). Dissociation as a predictor of cognitive behavior therapy outcome in patients with obsessive-compulsive disorder. Psychother. Psychosom. 75, 40–46. doi: 10.1159/000089225
Sack, M., Cillien, M., and Hopper, J. W. (2012). Acute dissociation and cardiac reactivity to script-driven imagery in trauma-related disorders. Eur. J. Psychotraumatol. 3, 1–10. doi: 10.3402/ejpt.v3i0.17419
Sack, M., Hofmann, A., Wizelman, L., and Lempa, W. (2008). Psychophysiological Changes During EMDR and Treatment Outcome. J. EMDR Pract. Res. 2, 239–246. doi: 10.1891/1933-3196.2.4.239
Saj, A., Pierce, J. E., Ronchi, R., Ros, T., Thomasson, M., Bernati, T., et al. (2021). Real-time fMRI and EEG neurofeedback: A perspective on applications for the rehabilitation of spatial neglect. Ann. Phys. Rehabil. Med. 64:101561. doi: 10.1016/j.rehab.2021.101561
Sandberg, D. A. (2010). Adult attachment as a predictor of posttraumatic stress and dissociation. J. Trauma Dissociation 11, 293–307. doi: 10.1080/15299731003780937
Sandberg, D. A., Suess, E. A., and Heaton, J. L. (2010). Attachment Anxiety as a Mediator of the Relationship Between Interpersonal Trauma and Posttraumatic Symptomatology Among College Women. J. Interpers. Violence 25, 33–49. doi: 10.1177/0886260508329126
Sandler, A., and Coren, A. (1981). Vestibular stimulation in early childhood: A review. J. Early Interv. 3, 48–55. doi: 10.1177/105381518100300108
Sar, V., and Öztürk, E. (2008). What is trauma and dissociation? J. Trauma Pract. 4, 7–20. doi: 10.1300/J189v04n01_02
Sar, V., Islam, S., and Öztürk, E. (2009). Childhood emotional abuse and dissociation in patients with conversion symptoms. Psychiatry Clin. Neurosci. 63, 670–677. doi: 10.1111/j.1440-1819.2009.02012.x
Sasaki, R., Yamada, Y., Tsukahara, Y., and Kuniyoshi, Y. (2013). “Tactile stimuli from amniotic fluid guides the development of somatosensory cortex with hierarchical structure using human fetus simulation,” in 2013 IEEE 3rd Joint International Conference on Development and Learning and Epigenetic Robotics (ICDL), (Osaka). doi: 10.1109/DevLrn.2013.6652530
Schäflein, E., Sattel, H., Schmidt, U., and Sack, M. (2018). The enemy in the mirror: Self-perception-induced stress results in dissociation of psychological and physiological responses in patients with dissociative disorder. Eur. J. Psychotraumatol. 9:1472991. doi: 10.1080/20008198.2018.1472991
Schauer, M., and Elbert, T. (2010). Dissociation following traumatic stress etiology and treatment. J. Psychol. 218, 109–127. doi: 10.1027/0044-3409/a000018
Schirmer, A., Jesuthasan, S., and Mathuru, A. S. (2013). Tactile stimulation reduces fear in fish. Front. Behav. Neurosci 7:167. doi: 10.3389/fnbeh.2013.00167
Schlumpf, Y. R., Reinders, A. A. T. S., Nijenhuis, E. R. S., Luechinger, R., van Osch, M. J. P., and Jäncke, L. (2014). Dissociative part-dependent resting-state activity in dissociative identity disorder: A controlled fMRI perfusion study. PLoS One 9:e98795. doi: 10.1371/journal.pone.0098795
Schmahl, C., Lanius, R. A., Pain, C., and Vermetten, E. (2010). “Biological framework for traumatic dissociation related to early life trauma,” in The impact of early life trauma on health and disease: The hidden epidemic, eds R. A. Lanius, E. Vermetten, and C. Pain (Cambridge: Cambridge University Press), 178–188. doi: 10.1017/CBO9780511777042.021
Schmied, C., Boivin, X., Scala, S., and Waiblinger, S. (2010). Effect of previous stroking on reactions to a veterinary procedure behaviour and heart rate of dairy cows. Interact. Stud. 11, 467–481. doi: 10.1075/is.11.3.08sch
Schore, A. N. (2003). Effects of a secure attachment relationship on right brain development, affect regulation, and infant mental health. Infant Ment. Health J. 22, 7–66. doi: 10.1002/1097-0355(200101/04)22:1<7::AID-IMHJ2<3.0.CO;2-N
Schouten, K. A., de Niet, G. J., Knipscheer, J. W., Kleber, R. J., and Hutschemaekers, G. J. M. (2015). The effectiveness of art therapy in the treatment of traumatized adults: A systematic review on art therapy and trauma. Trauma Violence Abuse 16, 220–228. doi: 10.1177/1524838014555032
Schwabe, L., and Blanke, O. (2008). The vestibular component in out-of-body experiences: A computational approach. Front. Hum. Neurosci. 2:17. doi: 10.3389/neuro.09.017.2008
Scott, S., and Babcock, J. C. (2010). Attachment as a moderator between intimate partner violence and PTSD symptoms. J. Fam. Violence 25, 1–9. doi: 10.1007/s10896-009-9264-1
Scudder, C. A., Moschovakis, A. K., Karabelas, A. B., and Highstein, S. M. (1996). Anatomy and physiology of saccadic long-lead burst neurons recorded in the alert squirrel monkey. I. Descending projections from the mesencephalon. J. Neurophysiol. 76, 332–352. doi: 10.1152/jn.1996.76.1.332
Seizova-Cajic, T., and Sachtler, W. L. B. (2007). Adaptation of a bimodal integration stage: Visualinput needed during neck muscle vibration to elicit a motion aftereffect. Exp. Brain Res. 181, 117–129. doi: 10.1007/s00221-007-0910-7
Sengul, G., and Watson, C. (2015). “Ascending and Descending Pathways in the Spinal Cord,” in The Rat Nervous System, Fourth Edn, ed. G. Paxinos (Amsterdam: Elsevier), 115–130. doi: 10.1016/B978-0-12-374245-2.00008-5
Serino, A., and Haggard, P. (2010). Touch and the body. Neurosci. Biobehav. Rev. 34, 224–236. doi: 10.1016/j.neubiorev.2009.04.004
Shalev, A. Y., Peri, T., Brandes, D., Freedman, S., Orr, S. P., and Pitman, R. K. (2000). Auditory startle response in trauma survivors with posttraumatic stress disorder: A prospective study. Am. J. Psychiatry 157, 255–261. doi: 10.1176/appi.ajp.157.2.255
Shapiro, D. L., and Levendosky, A. A. (1999). Adolescent survivors of childhood sexual abuse: The mediating role of attachment style and coping in psychological and interpersonal functioning. Child Abuse Neglect 23, 1175–1191. doi: 10.1016/S0145-2134(99)00085-X
Shapiro, F. (1995). Eye movement desensitization and reprocessing: Basic principles, protocols, and procedures. New York, NY: Guilford Press.
Shayman, C. S., Seo, J. H., Oh, Y., Lewis, R. F., Peterka, R. J., and Hullar, T. E. (2018). Relationship between vestibular sensitivity and multisensory temporal integration. J. Neurophysiol. 120, 1572–1577. doi: 10.1152/jn.00379.2018
Shewmon, D. A., Holmes, G. L., and Byrne, P. A. (1999). Consciousness in congenitally decorticate children: Developmental vegetative state as self-fulfilling prophecy. Dev. Med. Child Neurol. 41, 364–374. doi: 10.1017/S0012162299000821
Shinoda, Y., Sugiuchi, Y., Izawa, Y., and Hata, Y. (2006). Long descending motor tract axons and their control of neck and axial muscles. Prog. Brain Res. 151, 527–563. doi: 10.1016/S0079-6123(05)51017-3
Sidebotham, P. (1988). Balance through the ages of Man. J. Laryngol. Otol. 102, 203–208. doi: 10.1017/S0022215100104530
Siegel, D. J. (2001). Toward an interpersonal neurobiology of the developing mind: Attachment relationships, “mindsight,” and neural integration. Infant Ment. Health J. 22, 67–94. doi: 10.1002/1097-0355(200101/04)22:1<67::AID-IMHJ3<3.0.CO;2-G
Signal, T., Taylor, N., Botros, H., Prentice, K., and Lazarus, K. (2013). Whispering to horses: Childhood sexual abuse, depression and the efficacy of equine facilitated therapy. Sex. Abuse Aust. N. Z. 5, 24–32.
Soares, M. C., Oliveira, R. F., Ros, A. F. H., Grutter, A. S., and Bshary, R. (2011). Tactile stimulation lowers stress in fish. Nat. Commun. 2, 1–5. doi: 10.1038/ncomms1547
Solomon, Z., Ginzburg, K., Mikulincer, M., Neria, Y., and Ohry, A. (1998). Coping with war captivity: The role of attachment style. Eur. J. Pers. 12, 271–285. doi: 10.1002/(SICI)1099-0984(199807/08)12:4<271::AID-PER309<3.0.CO;2-U
Söndergaard, H. P., and Elofsson, U. (2008). Psychophysiological Studies of EMDR. J. EMDR Pract. Res. 2, 282–288. doi: 10.1891/1933-3196.2.4.282
Spiegel, D., Loewenstein, R. J., Lewis-Fernández, R., Sar, V., Simeon, D., Vermetten, E., et al. (2011). Dissociative disorders in DSM-5. Depress. Anxiety 28, 824–852. doi: 10.1002/da.20874
Spitzer, C., Barnow, S., Freyberger, H. J., and Grabe, H. J. (2007). Dissociation predicts symptom-related treatment outcome in short-term inpatient psychotherapy. Aust. N. Z. J. Psychiatry 41, 682–687. doi: 10.1080/00048670701449146
Spreng, R. N., and Grady, C. L. (2010). Patterns of brain activity supporting autobiographical memory, prospection, and theory of mind, and their relationship to the default mode network. J. Cogn. Neurosci. 22, 1112–1123. doi: 10.1162/jocn.2009.21282
Sridharan, D., Levitin, D. J., and Menon, V. (2008). A critical role for the right fronto-insular cortex in switching between central-executive and default-mode networks. Proc. Natl. Acad. Sci. U.S.A. 105, 12569–12574. doi: 10.1073/pnas.0800005105
Sripada, R. K., King, A. P., Welsh, R. C., Garfinkel, S. N., Wang, X., Sripada, C. S., et al. (2012). Neural dysregulation in posttraumatic stress disorder: Evidence for disrupted equilibrium between salience and default mode brain networks. Psychosom. Med. 74, 904–911. doi: 10.1097/PSY.0b013e318273bf33
Stein, D. J., Koenen, K. C., Friedman, M. J., Hill, E., McLaughlin, K. A., Petukhova, M., et al. (2013). Dissociation in posttraumatic stress disorder: Evidence from the world mental health surveys. Biol. Psychiatry 73, 302–312. doi: 10.1016/j.biopsych.2012.08.022
Steuwe, C., Daniels, J. K., Frewen, P. A., Densmore, M., Pannasch, S., Beblo, T., et al. (2014). Effect of direct eye contact in PTSD related to interpersonal trauma: An fMRI study of activation of an innate alarm system. Soc. Cogn. Affect. Neurosci. 9, 88–97. doi: 10.1093/scan/nss105
Steuwe, C., Lanius, R. A., and Frewen, P. A. (2012). Evidence for a dissociative subtype of PTSD by latent profile and confirmatory factor analyses in a civilian sample. Depress. Anxiety 29, 689–700. doi: 10.1002/da.21944
Stevenson, R. A., Segers, M., Ncube, B. L., Black, K. R., Bebko, J. M., Ferber, S., et al. (2018). The cascading influence of multisensory processing on speech perception in autism. Autism 22, 609–624. doi: 10.1177/1362361317704413
Strauss, T., Rottstädt, F., Sailer, U., Schellong, J., Hamilton, J. P., Raue, C., et al. (2019). Touch aversion in patients with interpersonal traumatization. Depress. Anxiety 36, 635–646. doi: 10.1002/da.22914
Suarez, S. D., and Gallup, G. G. (1979). Tonic immobility as a response to rape in humans: A theoretical note. Psychol. Rec. 29, 315–320. doi: 10.1007/bf03394619
Synofzik, M., Vosgerau, G., and Newen, A. (2008). I move, therefore I am: A new theoretical framework to investigate agency and ownership. Conscious. Cogn. 17, 411–424. doi: 10.1016/j.concog.2008.03.008
Takakusaki, K. (2017). Functional neuroanatomy for posture and gait control. J. Mov. Disord. 10, 1–17. doi: 10.14802/jmd.16062
Taylor, J. L. (2009). “Proprioception,” in Encyclopedia of neuroscience, Vol. 7, ed. L. R. Squire (Oxford: Academic Press), 1143–1149.
Terpou, B. A., Densmore, M., Théberge, J., Frewen, P., McKinnon, M. C., and Lanius, R. A. (2018). Resting-state pulvinar-posterior parietal decoupling in PTSD and its dissociative subtype. Hum. Brain Mapp. 39, 4228–4240. doi: 10.1002/hbm.24242
Terpou, B. A., Densmore, M., Théberge, J., Frewen, P., McKinnon, M. C., Nicholson, A. A., et al. (2020). The hijacked self: Disrupted functional connectivity between the periaqueductal gray and the default mode network in posttraumatic stress disorder using dynamic causal modeling. Neuroimage 27:102345. doi: 10.1016/j.nicl.2020.102345
Terpou, B. A., Densmore, M., Théberge, J., Thome, J., Frewen, P., McKinnon, M. C., et al. (2019a). The threatful self: Midbrain functional connectivity to cortical midline and parietal regions during subliminal trauma-related processing in PTSD. Chronic Stress 3, 1–12. doi: 10.1177/2470547019871369
Terpou, B. A., Harricharan, S., McKinnon, M. C., Frewen, P., Jetly, R., and Lanius, R. A. (2019b). The effects of trauma on brain and body: A unifying role for the midbrain periaqueductal gray. J. Neurosci. Res. 97, 1110–1140. doi: 10.1002/jnr.24447
Terr, L. (1990). Too scared to cry: Psychic trauma in childhood. New York, NY: Harper & Row Publishers.
Thome, J., Densmore, M., Koppe, G., Terpou, B., Théberge, J., McKinnon, M. C., et al. (2019). Back to the basics: Resting state functional connectivity of the reticular activation system in PTSD and its dissociative subtype. Chronic Stress 3, 1–14. doi: 10.1177/2470547019873663
Thome, J., Frewen, P., Daniels, J. K., Densmore, M., and Lanius, R. A. (2014). Altered connectivity within the salience network during direct eye gaze in PTSD. Borderline Personal. Disord. Emot. Dysregul. 1, 1–10. doi: 10.1186/2051-6673-1-17
Thye, M. D., Bednarz, H. M., Herringshaw, A. J., Sartin, E. B., and Kana, R. K. (2018). The impact of atypical sensory processing on social impairments in autism spectrum disorder. Dev. Cogn. Neurosci. 29, 151–167. doi: 10.1016/j.dcn.2017.04.010
Tovote, P., Esposito, M. S., Botta, P., Chaudun, F., Fadok, J. P., Markovic, M., et al. (2016). Midbrain circuits for defensive behaviour. Nature 534, 206–212. doi: 10.1038/nature17996
Tsakiris, M. (2010). My body in the brain: A neurocognitive model of body-ownership. Neuropsychologia 48, 703–712. doi: 10.1016/j.neuropsychologia.2009.09.034
Tsakiris, M. (2017). The multisensory basis of the self: From body to identity to others. Q. J. Exp. Psychol. 70, 597–609. doi: 10.1080/17470218.2016.1181768
Tuthill, J. C., and Azim, E. (2018). Proprioception. Curr. Biol. 28, R194–R203. doi: 10.1016/j.cub.2018.01.064
Tuulari, J. J., Scheinin, N. M., Lehtola, S., Merisaari, H., Saunavaara, J., Parkkola, R., et al. (2019). Neural correlates of gentle skin stroking in early infancy. Dev. Cogn. Neurosci. 35, 36–41. doi: 10.1016/j.dcn.2017.10.004
Twaite, J. A., and Rodriguez-Srednicki, O. (2004). Childhood sexual and physical abuse and adult vulnerability to PTSD: The mediating effects of attachment and dissociation. J. Child Sex. Abuse 13, 17–38. doi: 10.1300/J070v13n01_02
van der Hart, O., Nijenhuis, R. S. E., and Steele, K. (2005). Dissociation: An insufficiently recognised major feature of complex PTSD. J. Trauma. Stress 18, 413–423. doi: 10.1002/jts.20049
van der Kolk, B. A. (1989). The compulsion to repeat the trauma. Re-enactment, revictimization, and masochism. Psychiatr. Clin. North Am. 12, 389–411. doi: 10.1016/s0193-953x(18)30439-8
van der Kolk, B. A. (2003). The neurobiology of childhood trauma and abuse. Child Adolesc. Psychiatr. Clin. North Am. 12, 293–317. doi: 10.1016/S1056-4993(03)00003-8
van der Kolk, B. A. (2015). The body keeps the score: Brain, mind, and body in the healing of trauma. New York, NY: Viking.
van der Kolk, B. A., and Fisler, R. (1995). Dissociation and the fragmentary nature of traumatic memories: Overview and exploratory study. J. Trauma. Stress 8, 505–525. doi: 10.1007/BF02102887
van der Kolk, B. A., Hodgdon, H., Gapen, M., Musicaro, R., Suvak, M. K., Hamlin, E., et al. (2016). A randomized controlled study of neurofeedback for chronic PTSD. PLoS One 11:e0166752. doi: 10.1371/journal.pone.0166752
van der Kolk, B. A., Spinazzola, J., Blaustein, M. E., Hopper, J. W., Hopper, E. K., Korn, D. L., et al. (2007). A Randomized clinical trial of eye movement desensitization and reprocessing (EMDR), fluoxetine, and pill placebo in the treatment of posttraumatic stress disorder: Treatment effects and long-term maintenance. J. Clin. Psychiatry 68, 37–46. doi: 10.4088/JCP.v68n0105
van der Kolk, B. A., Stone, L., West, J., Rhodes, A., Emerson, D., Suvak, M., et al. (2014). Yoga as an adjunctive treatment for posttraumatic stress disorder: A randomized controlled trial. J. Clin. Psychiatry 75, e559–e565. doi: 10.4088/JCP.13m08561
van Puyvelde, M., Gorissen, A. S., Pattyn, N., and McGlone, F. (2019). Does touch matter? The impact of stroking versus non-stroking maternal touch on cardio-respiratory processes in mothers and infants. Physiol. Behav. 207, 55–63. doi: 10.1016/j.physbeh.2019.04.024
Vancappel, A., Suzan, L., Bailly, S., Fraigneau, M., Réveillère, C., and El-Hage, W. (2022). Exploring strategies to cope with dissociation and its determinants through functional analysis in patients suffering from PTSD: A qualitative study. Eur. J. Trauma Dissociation 6:100235. doi: 10.1016/j.ejtd.2021.100235
Volchan, E., Souza, G. G., Franklin, C. M., Norte, C. E., Rocha-Rego, V., Oliveira, J. M., et al. (2011). Is there tonic immobility in humans? Biological evidence from victims of traumatic stress. Biol. Psychol. 88, 13–19. doi: 10.1016/j.biopsycho.2011.06.002
von Hofsten, C. (2007). Action in development. Dev. Sci. 10, 54–60. doi: 10.1111/j.1467-7687.2007.00564.x
von Mohr, M., Kirsch, L. P., and Fotopoulou, A. (2017). The soothing function of touch:Affective touch reduces feelings of social exclusion. Sci. Rep. 7, 1–9. doi: 10.1038/s41598-017-13355-7
von Mohr, M., Krahe, C., Beck, B., and Fotopoulou, A. (2018). The social buffering of pain by affective touch: A laser-evoked potential study in romantic couples. Soc. Cogn. Affect. Neurosci. 13, 1121–1130. doi: 10.1093/scan/nsy085
Vrugt, D. T., and Pederson, D. R. (1973). The effects of vertical rocking frequencies on the arousal level in two-month-old infants. Child Dev. 44, 205–209. doi: 10.1111/j.1467-8624.1973.tb02139.x
Vulliemoz, S., Raineteau, O., and Jabaudon, D. (2005). Reaching beyond the midline: Why are human brains cross wired? Lancet Neurol. 4, 87–99. doi: 10.1016/S1474-4422(05)00990-7
Walker, S. C., Cavieres, A., Peñaloza-Sancho, V., El-Deredy, W., McGlone, F. P., and Dagnino-Subiabre, A. (2020). C-low threshold mechanoafferent targeted dynamic touch modulates stress resilience in rats exposed to chronic mild stress. Eur. J. Neurosci. 5, 2925–2938. doi: 10.1111/ejn.14951
Wallace, M. T., Woynaroski, T. G., and Stevenson, R. A. (2020). Multisensory integration as a window into orderly and disrupted cognition and communication. Annu. Rev. Psychol. 71, 193–219. doi: 10.1146/annurev-psych-010419-051112
Wang, C., Liu, H., Li, K., Wu, Z. Z., Wu, C., Yu, J. Y., et al. (2020). Tactile modulation of memory and anxiety requires dentate granule cells along the dorsoventral axis. Nat. Commun. 11, 1–18. doi: 10.1038/s41467-020-19874-8
Warner, E., Koomar, J., Lary, B., and Cook, A. (2013). Can the body change the score? Application of sensory modulation principles in the treatment of traumatized adolescents in residential settings. J. Fam. Violence 28, 729–738. doi: 10.1007/s10896-013-9535-8
Warner, E., Spinazzola, J., Westcott, A., Gunn, C., and Hodgdon, H. (2014). The body can change the score: Empirical support for somatic regulation in the treatment of traumatized adolescents. J. Child Adolesc. Trauma 7, 237–246. doi: 10.1007/s40653-014-0030-z
Webster, D. G., Lanthorn, T. H., Dewsbury, D. A., and Meyer, M. E. (1981). Tonic immobility and the dorsal immobility response in twelve species of muroid rodents. Behav. Neural Biol. 31, 32–41. doi: 10.1016/S0163-1047(81)91034-7
White, W. F., Burgess, A., Dalgleish, T., Halligan, S., Hiller, R., Oxley, A., et al. (2022). Prevalence of the dissociative subtype of post-traumatic stress disorder: A systematic review and meta-analysis. Psychological Medicine 52, 1629–1644. doi: 10.1017/S0033291722001647
Wiens, S. (2005). Interoception in emotional experience. Curr. Opin. Neurol. 18, 442–447. doi: 10.1097/01.wco.0000168079.92106.99
Wijdicks, E. F. (2019). The ascending reticular activating system. Neurocrit. Care 31, 419–422. doi: 10.1007/s12028-019-00687-7
Wijesinghe, R., Protti, D. A., and Camp, A. J. (2015). Vestibular interactions in the thalamus. Front. Neural Circuits 9:79. doi: 10.3389/fncir.2015.00079
Williams, C. L., Haines, J., and Sale, I. M. (2003). Psychophysiological and psychological correlates of dissociation in a case of dissociative identity disorder. J. Trauma Dissociation 4, 101–118. doi: 10.1300/J229v04n01_07
Williams, L. M., Palmer, D., Liddell, B. J., Song, L., and Gordon, E. (2006). The “when” and “where” of perceiving signals of threat versus non-threat. NeuroImage 31, 458–467. doi: 10.1016/j.neuroimage.2005.12.009
Wilson, S. A., Becker, L. A., and Tinker, R. H. (1997). Fifteen-month follow-up of eye movement desensitization and reprocessing (EMDR) treatment for posttraumatic stress disorder and psychological trauma. J. Consult. Clin. Psychol. 65, 1047–1056. doi: 10.1037/0022-006X.65.6.1047
Wolf, E. J., Lunney, C. A., Miller, M. W., Resick, P. A., Friedman, M. J., and Schnurr, P. P. (2012a). The dissociative subtype of PTSD: A replication and extension. Depress. Anxiety 29, 679–688. doi: 10.1002/da.21946
Wolf, E. J., Miller, M. W., Reardon, A. F., Ryabchenko, K. A., Castillo, D., and Freund, R. (2012b). A latent class analysis of dissociation and posttraumatic stress disorder: Evidence for a dissociative subtype. Arch. Gen. Psychiatry 69, 698–705. doi: 10.1001/archgenpsychiatry.2011.1574
Woodhouse, S., Ayers, S., and Field, A. P. (2015). The relationship between adult attachment style and post-traumatic stress symptoms: A meta-analysis. J. Anxiety Disord. 35, 103–117. doi: 10.1016/j.janxdis.2015.07.002
Yang, Z., Oathes, D., Linn, K. A., Bruce, S. E., Satterthwaite, T. D., Cook, P. A., et al. (2018). Cognitive behavioral therapy is associated with enhanced cognitive control network activity in major depression and posttraumatic stress disorder. Biol. Psychiatry 3, 311–319. doi: 10.1016/j.bpsc.2017.12.006
Yates, B. J., and Miller, A. D. (1998). Physiological evidence that the vestibular system participates in autonomic and respiratory control. J. Vestib. Res. 8, 17–25. doi: 10.3233/ves-1998-8103
Yehuda, R., McFarlane, A. C., and Shalev, A. Y. (1998). Predicting the development of posttraumatic stress disorder from the acute response to a traumatic event. Biol. Psychiatry 44, 1305–1313. doi: 10.1016/S0006-3223(98)00276-5
Yochman, A., and Pat-Horenczyk, R. (2020). Sensory Modulation in Children Exposed to Continuous Traumatic Stress. J. Child Adolesc. Trauma 13, 93–102. doi: 10.1007/s40653-019-00254-4
Yoo, H., and Mihaila, D. M. (2020). Neuroanatomy, vestibular pathways. Florida: StatPearls Publishing.
Yorke, J., Nugent, W., Strand, E., Bolen, R., New, J., and Davis, C. (2013). Equine-assisted therapy and its impact on cortisol levels of children and horses: A pilot study and meta-analysis. Early Child Dev. Care 183, 874–894. doi: 10.1080/03004430.2012.693486
Yu, H., Miao, W., Ji, E., Huang, S., Jin, S., Zhu, X., et al. (2022). Social touch-like tactile stimulation activates a tachykinin 1-oxytocin pathway to promote social interactions. Neuron 110, 1051–1067. doi: 10.1016/j.neuron.2021.12.022
Yules, R. B., Krebs, C. Q., and Gault, F. P. (1966). Reticular formation control of vestibular system. Exp. Neurol. 16, 349–358. doi: 10.1016/0014-4886(66)90103-8
Zakin, G., Solomon, Z., and Neria, Y. (2003). Hardiness, attachment style, and long term psychological distress among Israeli POWs and combat veterans. Personal. Individ. Differ. 34, 819–829. doi: 10.1016/S0191-8869(02)00073-9
Zoet, H. A., Wagenmans, A., van Minnen, A., and de Jongh, A. (2018). Presence of the dissociative subtype of PTSD does not moderate the outcome of intensive trauma focused treatment for PTSD. Eur. J. Psychotraumatol. 9:1468707. doi: 10.1080/20008198.2018.1468707
Keywords: vestibular, somatosensory, attachment, sensory processing, trauma, post-traumatic stress disorder (PTSD), sense of self, embodiment
Citation: Kearney BE and Lanius RA (2022) The brain-body disconnect: A somatic sensory basis for trauma-related disorders. Front. Neurosci. 16:1015749. doi: 10.3389/fnins.2022.1015749
Received: 10 August 2022; Accepted: 14 October 2022;
Published: 21 November 2022.
Edited by:
Mariella Pazzaglia, Sapienza University of Rome, ItalyReviewed by:
Gabriella Bottini, University of Pavia, ItalyOrestis Giotakos, Independent Researcher, Athens, Greece
Copyright © 2022 Kearney and Lanius. This is an open-access article distributed under the terms of the Creative Commons Attribution License (CC BY). The use, distribution or reproduction in other forums is permitted, provided the original author(s) and the copyright owner(s) are credited and that the original publication in this journal is cited, in accordance with accepted academic practice. No use, distribution or reproduction is permitted which does not comply with these terms.
*Correspondence: Breanne E. Kearney, YmtlYXJuZTNAdXdvLmNh; Ruth A. Lanius, cnV0aC5sYW5pdXNAbGhzYy5vbi5jYQ==