- 1Departamento de Farmacologia, Faculdade de Medicina de Ribeirão da Universidade de São Paulo (FMRP-USP), Ribeirão Preto, SP, Brazil
- 2Department of Psychology and Institute for Neuroscience, University of Texas at Austin, Austin, TX, United States
- 3Centro Universitário UNIFAMINAS (UNIFAMINAS), Muriaé, MG, Brazil
- 4Hospital do Câncer de Muriaé, Fundação Cristiano Varella (FCV), Muriaé, MG, Brazil
Background: Neuroinflammation is a response that involves different cell lineages of the central nervous system, such as neurons and glial cells. Among the non-pharmacological interventions for neuroinflammation, photobiomodulation (PBM) is gaining prominence because of its beneficial effects found in experimental brain research. We systematically reviewed the effects of PBM on laboratory animal models, specially to investigate potential benefits of PBM as an efficient anti-inflammatory therapy.
Methods: We conducted a systematic search on the bibliographic databases (PubMed and ScienceDirect) with the keywords based on MeSH terms: photobiomodulation, low-level laser therapy, brain, neuroinflammation, inflammation, cytokine, and microglia. Data search was limited from 2009 to June 2022. We followed the Preferred Reporting Items for Systematic Reviews and Meta-Analyses (PRISMA) guideline. The initial systematic search identified 140 articles. Among them, 54 articles were removed for duplication and 59 articles by screening. Therefore, 27 studies met the inclusion criteria.
Results: The studies showed that PBM has anti-inflammatory properties in several conditions, such as traumatic brain injury, edema formation and hyperalgesia, ischemia, neurodegenerative conditions, aging, epilepsy, depression, and spinal cord injury.
Conclusion: Taken together, these results indicate that transcranial PBM therapy is a promising strategy to treat brain pathological conditions induced by neuroinflammation.
Introduction
Neuroinflammation is a response that involves cells of the central nervous system (CNS) such as neurons, macroglia and microglia (DiSabato et al., 2016; Schain and Kreisl, 2017; Shabab et al., 2017). This response is mainly mediated by cytokines, chemokines, secondary messengers, and reactive oxygen species (ROS) (Glass et al., 2010; Park et al., 2011; DiSabato et al., 2016; Norden et al., 2016). Neuroinflammation also can be a pathological condition in a variety of neurodegenerative diseases ((Schain and Kreisl, 2017)). For example, the activation of microglia, pro-inflammatory cytokines and signaling pathways linked to inflammation such as nuclear factor kappa-light-chain-enhancer of activated B cells (NF-κB) pathway can trigger neurodegeneration (Glass et al., 2010; Harry and Kraft, 2012; Lyman et al., 2014). The prolonged release of pro-inflammatory mediators such as tumor necrosis factor-alpha (TNF-α), interleukin (IL)-1α, IL-1β, and IL-6 allow leukocytes to migrate into the brain and induce pathogenesis in the CNS (De Vries et al., 1996; Laflamme et al., 1999). In addition, this inflammatory reaction leads to synaptic gene dysregulation, tissue damage, and potentially cell death (Cunningham et al., 1996; Carson et al., 2006; DiSabato et al., 2016). Given this scenario, new therapeutic approaches are needed to modulate neuroinflammatory responses in pathological conditions.
Photobiomodulation (PBM), or low-level laser/light therapy (LLLT) (Anders et al., 2015), is a non-invasive light-driven intervention that involves the use of red and near-infrared (NIR) light to stimulate healing processes, reduce pain, protect the aging brain and decrease inflammation in several tissues, including the nervous tissue (Rojas and Gonzalez-Lima, 2011, 2013; Almeida et al., 2013; Arany, 2016; Hamblin, 2017; Cardoso et al., 2021a,b). These effects may be mediated by multiple mechanisms. However, cytochrome c oxidase (CCO), the fourth enzyme complex in the electron transport chain within mitochondria, is the main photoacceptor when cells are irradiated with the red to NIR light used for PBM (Karu, 1999). Cellular studies have also shown that PBM promotes ATP synthesis in mitochondria (Karu et al., 1995), and release of mitochondrial ROS and nitric oxide (Karu et al., 2005; Huang et al., 2009). These upstream processes contribute to increased cellular metabolism, altered mitochondrial dynamics, increased vasodilation, and mainly decreased inflammation (Pastore et al., 1994; Karu et al., 2005; Muili et al., 2012; Plass et al., 2012). In the brain in vivo, a primary PBM mechanism has been confirmed to be photonic oxidation of mitochondrial CCO (Wang et al., 2017a; Saucedo et al., 2021), being this mechanism independent of heat/thermal effects induced by light (Wang et al., 2017b). Brain PBM leads secondarily to increased cerebrovascular oxygenation (Tian et al., 2016; Holmes et al., 2019), the activation of metabolic pathways (Cardoso et al., 2021c), and of intracellular signaling molecules (Cardoso et al., 2021d), some of them relevant for inflammation (Cardoso et al., 2021a). The mitochondrial mechanism of PBM may provide a link between PBM and inflammation considering that recent studies have uncovered mitochondrial molecules, called mitochondrial alarmins, with inflammatory signaling properties (Grazioli and Pugin, 2018).
In favor of idea, it has been noted that PBM can alter the levels of inflammatory mediators in various animal models (Gupta et al., 2015; Martins et al., 2016; Yoshimura et al., 2016). For example, Gupta et al. (2015) demonstrated that 904 nm laser PBM enhances the healing of burn wounds in rats and attenuates inflammation by decreasing the expression of TNF-α and NF-kB, and by up-regulated expression of VEGF, FGFR-1, HSP-60, and HIF-1α at 4- and 7-days post-wounding. Martins et al. (2016) administered 950 nm laser PBM therapy in an animal model of inflammatory pain and found that the animals exhibited a reduced pain and an improvement of antioxidant enzymes and high levels of the anti-inflammatory cytokine IL-10. In a mouse model of obesity and type 2 diabetes mellitus, six sessions of 830 nm laser PBM were also able to reduce abdominal adipose tissue inflammation (Yoshimura et al., 2016).
In recent years, promising evidence has emerged to support the anti-inflammatory effects of the PBM therapy in various animal models in different neurological conditions (Khuman et al., 2012; Hamblin, 2017; Salehpour et al., 2019a,b; Cho et al., 2020; Cardoso et al., 2021a; Yang et al., 2021a). In this systematic review, we analyzed the neuroinflammatory effects of PBM on animal models of brain pathological conditions, in special to investigate potential translational benefits of PBM as an anti-inflammatory transcranial therapy.
Materials and methods
Data sources and search strategy
The search was conducted from 2009 to June 2022. PubMed and ScienceDirect were searched electronically with the keywords “photobiomodulation” or “low-level laser therapy” or “LLLT”; and “brain”; and “inflammation” or “cytokine” or “microglia” or “neuroinflammation” (Table 1). To ensure the clarity and transparency of the articles, we used the Preferred Reporting Items for Systematic Reviews and Meta-Analyses (PRISMA) guidelines (Moher et al., 2010). Two independent researchers screened the title, abstract, and the full text of the articles and judged the searched materials against the inclusion and exclusion criteria. Disagreements were resolved by consensus.
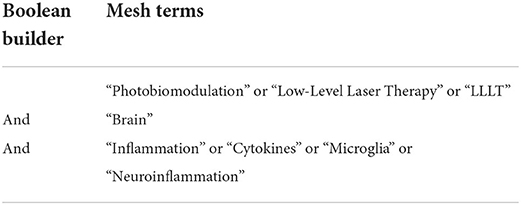
Table 1. Summary of laboratory animal studies on neuroinflammatory effects of the photobiomodulation.
Selection criteria
We selected all in vivo studies to obtain findings related to neuroinflammatory effect of PBM in brain disorders (Hamblin, 2017). The search strategy included experimental in vivo animal studies conducting PBM. We accepted only publications written in English. Neither in vitro approaches, clinical original articles, conference papers, nor review articles were included.
Data extraction and data synthesis
The included articles were divided according to pathological conditions. For data extraction, groups were subdivided according to the references (author and year), characteristics of the population (animals/species, sex and age), PBM parameters: light source/wavelength, continuous wave (CW) or pulsing mode, output power, irradiance per session, irradiation time, fluence per session, energy per session, irradiation approach/site, number of treatment sessions, and studies outcomes.
Results
The initial systematic search in PubMed and ScienceDirect databases identified 140 articles. Among them, 54 articles were removed for duplication and 59 articles by screening selection criteria. Then, 27 studies met the inclusion criteria (Figure 1).
Characteristics of the studies
Twenty seven articles reported experiments in rodents, of which 3 were performed on albino BALB/c mice (Salehpour et al., 2019a,b; Hosseini et al., 2022), 6 used C57BL/6 mice (Khuman et al., 2012; Zhang et al., 2014; Gonçalves et al., 2016; Lee et al., 2016, 2017; Duarte et al., 2018), 1 was conducted on the 5XFAD transgenic mice (pigmented C57BL/6 background) (Cho et al., 2020), 1 was performed on the APP/PS1 transgenic mice (Wu et al., 2021), 2 used the TgF344 transgenic mice (Yang et al., 2021b, 2022), 6 were performed using Wistar rats (Moreira et al., 2009; Prianti et al., 2014; Cardoso et al., 2021a, 2022; Gerace et al., 2021; Vogel et al., 2021), and 8 used Sprague-Dawley rats (Lu et al., 2017; Esenaliev et al., 2018; Yang et al., 2018, 2021a; O'Brien and Austin, 2019; Di Paolo, 2021; Wang et al., 2021; Tsai et al., 2022). In these laboratory rodent studies, the age of animals varied from 7 weeks old to 20 months old. In order to analyze the anti-inflammatory effects of PBM on brain, we reviewed various animal models such as focal brain damage (Moreira et al., 2009), controlled cortical impact (Khuman et al., 2012), peripheral inflammation (Prianti et al., 2014), mild traumatic brain injury (Zhang et al., 2014), spinal cord injury (Wang et al., 2021), Aβ-treatment (Lu et al., 2017), Aβ and PS1 transgenic rodents (Cho et al., 2020), PS1 transgenic rodents (Wu et al., 2021), TgF344 transgenic rodents (Yang et al., 2021b, 2022), brain ischemia (Lee et al., 2016, 2017; Gerace et al., 2021; Vogel et al., 2021), multiple sclerosis (Gonçalves et al., 2016), natural aging (Cardoso et al., 2021a, 2022), blast injury (Esenaliev et al., 2018), retinal degeneration (Di Paolo, 2021), induced aging (Hosseini et al., 2022), cuprizone-induced demyelination (Duarte et al., 2018), photothrombotic stroke (Yang et al., 2018), lipopolysaccharide-induced Parkinson's disease (O'Brien and Austin, 2019), experimental model of epilepsy (Tsai et al., 2022), restraint stress-induced depression experimental model (Salehpour et al., 2019a), transient global brain ischemia and artificially aging (Salehpour et al., 2019b), and neonatal hypoxic ischemia (Yang et al., 2021a).
The laser parameters used in the studies showed wide divergence:
Light source/ wavelength (nm): LED and LASER, 610 to 905;
Operation mode: CW and pulsed;
Output power (W): 0.03 to 1.91;
Irradiance (mW/cm2): 0.457 to 100;
Irradiation time per session (s): 3 to 3,600;
Total fluence (J/cm2): 1.0 to 535.7;
Energy (J): 0.6 to 294;
Irradiation approach/sites: contact to the rat skin immediately over the lesion site, transcranial, in the open craniotomy, in the spinal cord, on the abdomen of pregnant rats, in the cells and percutaneous.
Number of treatment sessions: 1 to 207.
The selected studies were summarized in a chronological order shown in Table 2.

Table 2. Summary of laboratory animal studies on neuroinflammatory effects of the photobiomodulation.
Findings and discussion
The purpose of this systematic review was to investigate the neuroinflammatory effects of PBM therapy. Studies have shown interesting findings on the anti-inflammatory effects of PBM in various animal models of neurological diseases in different neurological conditions, such as traumatic brain injury, edema formation and hyperalgesia, ischemia, neurodegenerative conditions, aging, and depression.
Traumatic brain injury
Studies using laboratory animals have shown that PBM reduces the level of pro-inflammatory cytokines and the microglia activation of TBI animal models (Moreira et al., 2009; Khuman et al., 2012; Esenaliev et al., 2018; Yang et al., 2018). For example, Khuman et al. (2012) demonstrated that 800 nm laser PBM inhibited the microglia activation, accompanied by improvement in cognitive deficits after controlled cortical impact. Esenaliev et al. (2018) observed that one session of 808 nm nano-pulsed laser PBM therapy applied 1 h after blast injury significantly inhibited microglia activation and reduced the number of cortical neurons expressing activated caspase-3 in a rat model of blast-induced neurotrauma. Also, in the study conducted by Yang et al. (2018), 9 sessions of 808 nm laser PBM was able to change the phenotype of microglial polarization from the M1 pro-inflammatory phenotype to the M2 anti-inflammatory phenotype. Since TBI is accompanied by an increase in cytokine and chemokine levels (Woodcock and Morganti-Kossmann, 2013; Bergold, 2016), the severity of brain damage is linked to a higher and more prolonged inflammatory response (Kumar and Loane, 2012; White et al., 2013; Woodcock and Morganti-Kossmann, 2013; Lozano et al., 2015). In animals, an increase in cerebral cortical levels of the inflammatory cytokines IL-1β, TNFα, and IL-6 has been shown from 3 to 9 h after injury (Bachstetter et al., 2013). In clinical studies, levels of pro-inflammatory markers IL-6, TNFα, IL-10, IL-8, and monocyte chemoattractant protein-1 (MCP-1) have also been increased after 2 days of TBI (Morganti-Kossman et al., 1997; Csuka et al., 1999; Semple et al., 2010). In addition, the release of these cytokines was correlated with microglial activation and axonal dysfunction, suggesting an association between the activated immune response and brain injury (Frugier et al., 2010). Taken together, it seems that PBM can exert anti-inflammatory action against TBI through the modulation of both anti- and pro-inflammatory chemokines and cytokines.
Edema formation and hyperalgesia
In the study by Prianti et al. (2014), they showed that 660 nm laser PBM reduces COX-2 mRNA expression in animals receiving carrageenan. This is a worthwhile result as in inflammatory conditions COX-2 is highly expressed, increasing the release of pro-inflammatory markers (Schuligoi et al., 2003; Grill et al., 2006, 2008), and plays a key role in chronic pain (Narita et al., 2008).
Brain ischemia
Ischemia can trigger an imbalance between pro- and anti-inflammatory mediators (Yilmaz and Granger, 2008), which play a key role in the progression and pathogenesis of ischemia (Barone and Feuerstein, 1999; Samson et al., 2005; Chamorro and Hallenbeck, 2006; Wang et al., 2007). For example, the inhibition of inflammatory response in ischemic patients can decrease the brain injury (Yilmaz and Granger, 2008). The therapeutic effects of PBM on ischemia have been addressed in the works by Zhang et al. (2014), Lee et al. (2016, 2017), Salehpour et al. (2019b), Vogel et al. (2021), Yang et al. (2021a) and Gerace et al. (2021). Lee et al. (2016, 2017) treated mice submitted to a focal brain ischemia experimental model with a 610 nm laser and observed an inhibition of Iba-1 and GFAP-labeled cells, accompanied by a regulation of pro-inflammatory cytokines and suppression of mitogen activated protein kinase (MAPK) (a signaling pathway linked to inflammation and cell death) and NF-kB activation. Salehpour et al. (2019b) showed that 14 sessions of 810 nm laser PBM significantly decreased iNOS, TNF-α, and IL-1β levels in the brain of transient global cerebral cortex ischemia model using artificially aged mice. This evidence is promising since activation of MAPKs p38, ERK and JNK regulate pro-inflammatory genes that activate NF-kB in microglia.
Neurodegenerative conditions
Abnormal microglial activation and inflammatory response may contribute to the pathology of several neurodegenerative conditions (Chen et al., 2016; Swaroop et al., 2016; Shabab et al., 2017; Voet et al., 2019). It is known that patients with multiple sclerosis (Huang et al., 2020), Alzheimer's disease (Licastro et al., 2000), and Parkinson's disease (Liu et al., 2003) exhibit elevated levels of pro-inflammatory markers in plasma. Transcranial PBM therapy has anti-inflammatory effects in several models of neurodegenerative conditions (Gonçalves et al., 2016; Lu et al., 2017; Duarte et al., 2018; O'Brien and Austin, 2019; Cho et al., 2020; Di Paolo, 2021; Wu et al., 2021; Yang et al., 2021b, 2022). For instance, Gonçalves et al. (2016) submitted mice to a model of multiple sclerosis to 30 sessions of either 660 nm or 904 nm laser PBM therapy. PBM-treated mice exhibited decreased levels of IL-1β, IL-17 and interferon-γ (IFN-γ) in the spinal cord, when compared to the control group mice. In addition, Duarte et al. (2018) demonstrated that 6 sessions of 808 nm laser PBM modulate microglial and astrocytes activation induced by cuprizone. In Aβ-treated mice, PBM treatment also reduced microglia (Iba-1 immunoreactivity) in the cerebral cortex (Cho et al., 2020), and attenuated the elevation of glial activation and IL-1β, IL-6, and TNF-α levels in the hippocampal CA1 region (Lu et al., 2017). Furthermore, O'Brien and Austin (2019) observed that PBM protected against lipopolysaccharide-induced dopaminergic cell death in a rat Parkinson's disease experimental model.
Aging
Brain aging is characterized by microglia reactivity and an imbalance between pro- and anti-inflammatory cytokines (Godbout and Johnson, 2009; Jurgens and Johnson, 2012). However, a couple of recent studies involving our group have shown that PBM can improve the inflammatory response in the aging brain (Salehpour et al., 2019b; Cardoso et al., 2021a, 2022; Hosseini et al., 2022). For example, a protocol with 58 consecutive days of 810 nm laser PBM therapy was able to change the inflammatory profile of the aging brain in rats. We showed that PBM increased cerebral cortex levels of IL-10, IL-6, and TNF-α. In addition, PBM therapy significantly decreased cerebral cortex levels of IL-5 and hippocampal levels of IP-10 and fractalkine (Cardoso et al., 2021a). In addition, we reported that 10 sessions of 660 nm laser PBM Increased levels of IL-1α and decreased levels of IL-5 in the cerebral cortex. In the hippocampus, the laser treatment increased the levels of IL-1α and decreased levels of IL-5, IL-18, and fractalkine (Cardoso et al., 2022). These findings are promising, since the expression of pro-inflammatory cytokines, oxidative stress, and glial activation are increased during the aging (Lee et al., 2000; Blalock et al., 2003; Godbout et al., 2005; Bishop et al., 2010).
Epilepsy
In the study conducted by Tsai et al. (2022), they showed that 608 nm laser PBM reduced neuron-specific enolase (NSE) and glial fibrillary acid protein (GFAP) immunoreactivity in hippocampus in an animal model of epilepsy. These results are promising since studies suggest inflammation as a biomarker in epilepsy (Ravizza et al., 2008; Auvin et al., 2010; Vezzani and Friedman, 2011; Vezzani et al., 2016). For example, blocking IL-1β (a pro-inflammatory interleukin) prevents generalized seizure and increases the threshold for induction of afterdischarge (Ravizza et al., 2008; Auvin et al., 2010).
Depression
Salehpour et al. (2019a) observed that 5 sessions of 810 nm laser PBM suppressed neuroinflammatory responses in the neocortex and hippocampus of mice submitted to a restraint stress-induced depression model by decreasing NF-kB, p38, and JNK levels. In addition, PBM decreased the serum levels of cortisol, corticosterone, TNF-α, and IL-6 induced by restraint stress. These results are promising since evidence suggests that these pro-inflammatory proteins are involved in the pathology of major depressive disorder (Kubera et al., 2011; Liu et al., 2012), as well as neurotransmission and mood regulation (Du et al., 2008; Villanueva, 2013).
Spinal cord injury
In the study conducted by Wang et al. (2021) it was observed that microglia and astrocytes begin to be activated after spinal cord injury, participating in secondary damage and tissue repair. However, 810 nm laser PBM during two consecutive weeks was able to inhibit microglia/macrophage and astrocyte activation after spinal cord injury. In this regard, PBM may be a useful tool for the treatment of spinal cord injury, in association to antibody-based approaches to interrupt endothelial-monocyte interactions, reducing macrophage activation at the injured spinal cord and also to pharmacological therapies focusing on immunomodulation and promotion of reparative glia activity (Orr and Gensel, 2018).
Limitations
Our review presents limitations. The researches highlighted in this review describe several brain conditions and methodologies, and the lack of some details about the PBM parameters used in each work make it difficult to replicate these approaches. Standardization of the protocols for each condition would facilitate comparison between the findings of the studies and could improve the translational application of PBM therapy.
Conclusion
Neuroinflammation is a pathological condition in a variety of brain insults and neurodegenerative conditions. Despite using very different protocols, the reviewed studies showed that the therapeutic effects of transcranial PBM therapy in animal models of neurological and psychiatric diseases are related to the capacity to reduce levels of pro-inflammatory mediators and increase levels of anti-inflammatory mediators. In addition, no adverse effects of PBM on the brain were found. Therefore, PBM could safely fit in to complement current treatments for the conditions listed above. These results mean that for the current use of PBM, controlled human studies are needed as a next-step of research to build on these animal studies. Despite not excluding human studies as a keyword, no controlled human studies were discovered in the present review, and all the included studies were animal studies. Human studies could bring new perspectives on the anti-inflammatory property of PBM in brain disorders. The reviewed animal studies together with consistent human studies of PBM in the treatment of neuroinflammation can suggest that transcranial PBM is a promising strategy for the treatment of neuroinflammation-induced brain diseases.
Data availability statement
The raw data supporting the conclusions of this article will be made available by the authors, without undue reservation.
Author contributions
All authors listed have made a substantial, direct, and intellectual contribution to the work and approved it for publication.
Funding
This study was supported by Coordenação de Aperfeiçoamento de Pessoal de Nível Superior (CAPES) and Fundação de Amparo à Pesquisa do Estado de São Paulo (FAPESP) (grants 2017/16443-0, 2021/06473-4, and 2020/15050-7). FG-L was supported by the Oskar Fischer Project Fund.
Conflict of interest
The authors declare that the research was conducted in the absence of any commercial or financial relationships that could be construed as a potential conflict of interest.
Publisher's note
All claims expressed in this article are solely those of the authors and do not necessarily represent those of their affiliated organizations, or those of the publisher, the editors and the reviewers. Any product that may be evaluated in this article, or claim that may be made by its manufacturer, is not guaranteed or endorsed by the publisher.
Supplementary material
The Supplementary Material for this article can be found online at: https://www.frontiersin.org/articles/10.3389/fnins.2022.1006031/full#supplementary-material
References
Almeida, P., Lopes-Martins, R. Á., Tomazoni, S. S., Albuquerque-Pontes, G. M., Santos, L. A., Vanin, A. A., et al. (2013). Low-level laser therapy and sodium diclofenac in acute inflammatory response induced by skeletal muscle trauma: effects in muscle morphology and mRNA gene expression of inflammatory markers. Photochem. Photobiol. 89, 501–507. doi: 10.1111/j.1751-1097.2012.01232.x
Anders, J. J., Lanzafame, R. J., and Arany, P. R. (2015). Low-level light/laser therapy versus photobiomodulation therapy. Photomed. Laser Surg. 33, 183–184. doi: 10.1089/pho.2015.9848
Arany, P. R. (2016). Craniofacial wound healing with photobiomodulation therapy: new insights and current challenges. J. Dent. Res. 95, 977–984. doi: 10.1177/0022034516648939
Auvin, S., Shin, D., Mazarati, A., and Sankar, R. (2010). Inflammation induced by LPS enhances epileptogenesis in immature rat and may be partially reversed by IL1RA. Epilepsia 51, 34–38. doi: 10.1111/j.1528-1167.2010.02606.x
Bachstetter, A. D., Rowe, R. K., Kaneko, M., Goulding, D., Lifshitz, J., and Van Eldik, L. J. (2013). The p38α MAPK regulates microglial responsiveness to diffuse traumatic brain injury. J. Neurosci. 33, 6143–6153. doi: 10.1523/JNEUROSCI.5399-12.2013
Barone, F. C., and Feuerstein, G. Z. (1999). Inflammatory mediators and stroke: new opportunities for novel therapeutics. J. Cereb. Blood Flow Metab. 19, 819–834. doi: 10.1097/00004647-199908000-00001
Bergold, P. J. (2016). Treatment of traumatic brain injury with anti-inflammatory drugs. Exp. Neurol. 275, 367–380. doi: 10.1016/j.expneurol.2015.05.024
Bishop, N. A., Lu, T., and Yankner, B. A. (2010). Neural mechanisms of ageing and cognitive decline. Nature 464, 529–535. doi: 10.1038/nature08983
Blalock, E. M., Chen, K. C., Sharrow, K., Herman, J. P., Porter, N. M., Foster, T. C., et al. (2003). Gene microarrays in hippocampal aging: statistical profiling identifies novel processes correlated with cognitive impairment. J. Neurosci. 23, 3807–3819. doi: 10.1523/JNEUROSCI.23-09-03807.2003
Cardoso, F., Tavares, C. D. S. O., Araujo, B. H. S., Mansur, F., Lopes-Martins, R. A. B., and Gomes da Silva, S. (2021a). Improved spatial memory and neuroinflammatory profile changes in aged rats submitted to photobiomodulation therapy. Cell. Mol. Neurobiol. 42, 1875–1886. doi: 10.1007/s10571-021-01069-4
Cardoso, F. D. S., dos Santos, J. C. C., Gonzalez-Lima, F., Araújo, B. H. S., Lopes-Martins, R. Á. B., and Gomes da Silva, S. (2021c). Effects of chronic photobiomodulation with transcranial near-infrared laser on brain metabolomics of young and aged rats. Mol. Neurobiol. 58, 2256–2268. doi: 10.1007/s12035-020-02247-z
Cardoso, F. D. S., Gonzalez-Lima, F., and Gomes da Silva, S. (2021b). Photobiomodulation for the aging brain. Ageing Res. Rev. 70, 101415. doi: 10.1016/j.arr.2021.101415
Cardoso, F. D. S., Mansur, F. C. B., Araújo, B. H. S., Gonzalez-Lima, F., and Gomes da Silva, S. (2022). Photobiomodulation improves the inflammatory response and intracellular signaling proteins linked to vascular function and cell survival in the brain of aged rats. Mol. Neurobiol. 59, 420–428. doi: 10.1007/s12035-021-02606-4
Cardoso, F. D. S., Mansur, F. C. B., Lopes-Martins, R. À. B., Gonzalez-Lima, F., and Gomes da Silva, S. (2021d). Transcranial laser photobiomodulation improves intracellular signaling linked to cell survival, memory and glucose metabolism in the aged brain: a preliminary study. Front. Cell. Neurosci. 15, 683127. doi: 10.3389/fncel.2021.683127
Carson, M. J., Thrash, J. C., and Walter, B. (2006). The cellular response in neuroinflammation: the role of leukocytes, microglia and astrocytes in neuronal death and survival. Clin. Neurosci. Res. 6, 237–245. doi: 10.1016/j.cnr.2006.09.004
Chamorro, Á., and Hallenbeck, J. (2006). The harms and benefits of inflammatory and immune responses in vascular disease. Stroke. 37, 291–293. doi: 10.1161/01.STR.0000200561.69611.f8
Chen, W. W., Zhang, X. I. A., and Huang, W. J. (2016). Role of neuroinflammation in neurodegenerative diseases. Mol. Med. Rep. 13, 3391–3396. doi: 10.3892/mmr.2016.4948
Cho, G. M., Lee, S. Y., Park, J. H., Kim, M. J., Park, K. J., Choi, B. T., et al. (2020). Photobiomodulation using a low-level light-emitting diode improves cognitive dysfunction in the 5XFAD mouse model of Alzheimer's disease. J. Gerontol. A Biol. Sci. Med. Sci. 75, 631–639. doi: 10.1093/gerona/gly240
Csuka, E., Morganti-Kossmann, M. C., Lenzlinger, P. M., Joller, H., Trentz, O., and Kossmann, T. (1999). IL-10 levels in cerebrospinal fluid and serum of patients with severe traumatic brain injury: relationship to IL-6, TNF-α, TGF-β1 and blood–brain barrier function. J. Neuroimmunol. 101, 211–221. doi: 10.1016/S0165-5728(99)00148-4
Cunningham, A. J., Murray, C. A., O'neill, L. A. J., Lynch, M. A., and O'connor, J. J. (1996). Interleukin-1β (IL-1β) and tumour necrosis factor (TNF) inhibit long-term potentiation in the rat dentate gyrus in vitro. Neurosci. Lett. 203, 17–20. doi: 10.1016/0304-3940(95)12252-4
De Vries, H. E., Blom-Roosemalen, M. C., van Oosten, M., De Boer, A. G., Van Berkel, T. J., Breimer, D. D., et al. (1996). The influence of cytokines on the integrity of the blood-brain barrier in vitro. J. Neuroimmunol. 64, 37–43. doi: 10.1016/0165-5728(95)00148-4
Di Paolo, M. (2021). Sequential PBM–saffron treatment in an animal model of retinal degeneration. Medicina 57, 1059. doi: 10.3390/medicina57101059
DiSabato, D. J., Quan, N., and Godbout, J. P. (2016). Neuroinflammation: the devil is in the details. J. Neurochem. 139, 136–153. doi: 10.1111/jnc.13607
Du, J., Creson, T. K., Wu, L. J., Ren, M., Gray, N. A., Falke, C., et al. (2008). The role of hippocampal GluR1 and GluR2 receptors in manic-like behavior. J. Neurosci. 28, 68–79. doi: 10.1523/JNEUROSCI.3080-07.2008
Duarte, K. C. N., Soares, T. T., Magri, A. M. P., Garcia, L. A., Le Sueur-Maluf, L., Renno, A. C. M., et al. (2018). Low-level laser therapy modulates demyelination in mice. J. Photochem. Photobiol. B. 189, 55–65. doi: 10.1016/j.jphotobiol.2018.09.024
Esenaliev, R. O., Petrov, I. Y., Petrov, Y., Guptarak, J., Boone, D. R., Mocciaro, E., et al. (2018). Nano-pulsed laser therapy is neuroprotective in a rat model of blast-induced neurotrauma. J. Neurotrauma 35, 1510–1522. doi: 10.1089/neu.2017.5249
Frugier, T., Morganti-Kossmann, M. C., O'Reilly, D., and McLean, C. A. (2010). In situ detection of inflammatory mediators in post mortem human brain tissue after traumatic injury. J. Neurotrauma 27, 497–507. doi: 10.1089/neu.2009.1120
Gerace, E., Cialdai, F., Sereni, E., Lana, D., Nosi, D., Giovannini, M. G., et al. (2021). NIR Laser photobiomodulation induces neuroprotection in an in vitro model of cerebral hypoxia/ischemia. Mol. Neurobiol. 58, 5383–5395. doi: 10.1007/s12035-021-02496-6
Glass, C. K., Saijo, K., Winner, B., Marchetto, M. C., and Gage, F. H. (2010). Mechanisms underlying inflammation in neurodegeneration. Cell, 140, 918–934. doi: 10.1016/j.cell.2010.02.016
Godbout, J. P., Chen, J., Abraham, J., Richwine, A. F., Berg, B. M., Kelley, K. W., et al. (2005). Exaggerated neuroinflammation and sickness behavior in aged mice after activation of the peripheral innate immune system. FASEB J. 19, 1329–1331. doi: 10.1096/fj.05-3776fje
Godbout, J. P., and Johnson, R. W. (2009). Age and neuroinflammation: a lifetime of psychoneuroimmune consequences. Immunol. Allergy Clin. North Am. 29, 321–337. doi: 10.1016/j.iac.2009.02.007
Gonçalves, E. D., Souza, P. S., Lieberknecht, V., Fidelis, G. S., Barbosa, R. I., Silveira, P. C., et al. (2016). Low-level laser therapy ameliorates disease progression in a mouse model of multiple sclerosis. Autoimmunity. 49, 132–142. doi: 10.3109/08916934.2015.1124425
Grazioli, S., and Pugin, J. (2018). Mitochondrial damage-associated molecular patterns: from inflammatory signaling to human diseases. Front. Immunol. 9, 832. doi: 10.3389/fimmu.2018.00832
Grill, M., Heinemann, A., Hoefler, G., Peskar, B. A., and Schuligoi, R. (2008). Effect of endotoxin treatment on the expression and localization of spinal cyclooxygenase, prostaglandin synthases, and PGD2 receptors. J. Neurochem. 104, 1345–1357. doi: 10.1111/j.1471-4159.2007.05078.x
Grill, M., Peskar, B. A., Schuligoi, R., and Amann, R. (2006). Systemic inflammation induces COX-2 mediated prostaglandin D2 biosynthesis in mice spinal cord. Neuropharmacology. 50, 165–173. doi: 10.1016/j.neuropharm.2005.08.005
Gupta, A., Keshri, G. K., Yadav, A., Gola, S., Chauhan, S., Salhan, A. K., et al. (2015). Superpulsed (Ga-As, 904 nm) low-level laser therapy (LLLT) attenuates inflammatory response and enhances healing of burn wounds. J. Biophotonics. 8, 489–501. doi: 10.1002/jbio.201400058
Hamblin, M. R. (2017). Mechanisms and applications of the anti-inflammatory effects of photobiomodulation. AIMS Biophys. 4, 337–361. doi: 10.3934/biophy.2017.3.337
Harry, G. J., and Kraft, A. D. (2012). Microglia in the developing brain: a potential target with lifetime effects. Neurotoxicology 33, 191–206. doi: 10.1016/j.neuro.2012.01.012
Holmes, E., Barrett, D. W., Saucedo, C. L., O'Connor, P., Liu, H., and Gonzalez-Lima, F. (2019). Cognitive enhancement by transcranial photobiomodulation is associated with cerebrovascular oxygenation of the prefrontal cortex. Front. Neurosci. 13, 1129. doi: 10.3389/fnins.2019.01129
Hosseini, L., Farazi, N., Erfani, M., Mahmoudi, J., Akbari, M., Hosseini, S. H., et al. (2022). Effect of transcranial near-infrared photobiomodulation on cognitive outcomes in D-galactose/AlCl3 induced brain aging in BALB/c mice. Lasers Med. Sci. 37, 1787–1798. doi: 10.1007/s10103-021-03433-8
Huang, J., Khademi, M., Fugger, L., Lindhe, Ö., Novakova, L., Axelsson, M., et al. (2020). Inflammation-related plasma and CSF biomarkers for multiple sclerosis. Proc. Natl. Acad. Sci. 117, 12952–12960. doi: 10.1073/pnas.1912839117
Huang, Y. Y., Chen, A. C. H., Carroll, J. D., and Hamblin, M. R. (2009). Biphasic dose response in low level light therapy. Dose-response 7, 09–027. doi: 10.2203/dose-response.09-027.Hamblin
Jurgens, H. A., and Johnson, R. W. (2012). Dysregulated neuronal–microglial cross-talk during aging, stress and inflammation. Exp. Neurol. 233, 40–48. doi: 10.1016/j.expneurol.2010.11.014
Karu, T. (1999). Primary and secondary mechanisms of action of visible to near-IR radiation on cells. J. Photochem. Photobiol. B. Biol. 49, 1–17. doi: 10.1016/S1011-1344(98)00219-X
Karu, T., Pyatibrat, L., and Kalendo, G. (1995). Irradiation with He-Ne laser increases ATP level in cells cultivated in vitro. J. Photochem. Photobiol. B. Biol. 27, 219–223. doi: 10.1016/1011-1344(94)07078-3
Karu, T. I., Pyatibrat, L. V., and Afanasyeva, N. I. (2005). Cellular effects of low power laser therapy can be mediated by nitric oxide. Lasers Surg. Med. 36, 307–314. doi: 10.1002/lsm.20148
Khuman, J., Zhang, J., Park, J., Carroll, J. D., Donahue, C., and Whalen, M. J. (2012). Low-level laser light therapy improves cognitive deficits and inhibits microglial activation after controlled cortical impact in mice. J. Neurotrauma. 29, 408–417. doi: 10.1089/neu.2010.1745
Kubera, M., Obuchowicz, E., Goehler, L., Brzeszcz, J., and Maes, M. (2011). In animal models, psychosocial stress-induced (neuro) inflammation, apoptosis and reduced neurogenesis are associated to the onset of depression. Progr. Neuropsychopharmacol. Biol. Psychiatry. 35, 744–759. doi: 10.1016/j.pnpbp.2010.08.026
Kumar, A., and Loane, D. J. (2012). Neuroinflammation after traumatic brain injury: opportunities for therapeutic intervention. Brain Behav. Immun. 26, 1191–1201. doi: 10.1016/j.bbi.2012.06.008
Laflamme, N., Lacroix, S., and Rivest, S. (1999). An essential role of interleukin-1β in mediating NF-κB activity and COX-2 transcription in cells of the blood–brain barrier in response to a systemic and localized inflammation but not during endotoxemia. J. Neurosci. 19, 10923–10930. doi: 10.1523/JNEUROSCI.19-24-10923.1999
Lee, C. K., Weindruch, R., and Prolla, T. A. (2000). Gene-expression profile of the ageing brain in mice. Nat. Genet. 25, 294–297. doi: 10.1038/77046
Lee, H. I., Lee, S. W., Kim, N. G., Park, K. J., Choi, B. T., Shin, Y. I., et al. (2017). Low-level light emitting diode (LED) therapy suppresses inflammasome-mediated brain damage in experimental ischemic stroke. J. Biophotonics. 10, 1502–1513. doi: 10.1002/jbio.201600244
Lee, H. I., Park, J. H., Park, M. Y., Kim, N. G., Park, K. J., Choi, B. T., et al. (2016). Pre-conditioning with transcranial low-level light therapy reduces neuroinflammation and protects blood-brain barrier after focal cerebral ischemia in mice. Restor. Neurol. Neurosci. 34, 201–214. doi: 10.3233/RNN-150559
Licastro, F., Pedrini, S., Caputo, L., Annoni, G., Davis, L. J., Ferri, C., et al. (2000). Increased plasma levels of interleukin-1, interleukin-6 and α-1-antichymotrypsin in patients with Alzheimer's disease: peripheral inflammation or signals from the brain? J. Neuroimmunol. 103, 97–102. doi: 10.1016/S0165-5728(99)00226-X
Liu, B., Gao, H. M., and Hong, J. S. (2003). Parkinson's disease and exposure to infectious agents and pesticides and the occurrence of brain injuries: role of neuroinflammation. Environ. Health Perspect. 111, 1065–1073. doi: 10.1289/ehp.6361
Liu, Y., Ho, R. C. M., and Mak, A. (2012). Interleukin (IL)-6, tumour necrosis factor alpha (TNF-α) and soluble interleukin-2 receptors (sIL-2R) are elevated in patients with major depressive disorder: a meta-analysis and meta-regression. J. Affect. Disord. 139, 230–239. doi: 10.1016/j.jad.2011.08.003
Lozano, D., Gonzales-Portillo, G. S., Acosta, S., de la Pena, I., Tajiri, N., Kaneko, Y., et al. (2015). Neuroinflammatory responses to traumatic brain injury: etiology, clinical consequences, and therapeutic opportunities. Neuropsychiatr. Dis. Treat. 11, 97–106. doi: 10.2147/NDT.S65815
Lu, Y., Wang, R., Dong, Y., Tucker, D., Zhao, N., Ahmed, M. E., et al. (2017). Low-level laser therapy for beta amyloid toxicity in rat hippocampus. Neurobiol. Aging 49, 165–182. doi: 10.1016/j.neurobiolaging.2016.10.003
Lyman, M., Lloyd, D. G., Ji, X., Vizcaychipi, M. P., and Ma, D. (2014). Neuroinflammation: the role and consequences. Neurosci. Res. 79, 1–12. doi: 10.1016/j.neures.2013.10.004
Martins, D. F., Turnes, B. L., Cidral-Filho, F. J., Bobinski, F., Rosas, R. F., Danielski, L. G., et al. (2016). Light-emitting diode therapy reduces persistent inflammatory pain: Role of interleukin 10 and antioxidant enzymes. Neuroscience 324, 485–495. doi: 10.1016/j.neuroscience.2016.03.035
Moher, D., Liberati, A., Tetzlaff, J., and Altman, D. G. (2010). Preferred reporting items for systematic reviews and meta-analyses: the PRISMA statement. Int. J. Surg. 8, 336–341. doi: 10.1016/j.ijsu.2010.02.007
Moreira, M. S., Velasco, I. T., Ferreira, L. S., Ariga, S. K. K., Barbeiro, D. F., Meneguzzo, D. T., et al. (2009). Effect of phototherapy with low intensity laser on local and systemic immunomodulation following focal brain damage in rat. J. Photochem. Photobiol. 97, 145–151. doi: 10.1016/j.jphotobiol.2009.09.002
Morganti-Kossman, M. C., Lenzlinger, P. M., Hans, V., Stahel, P., Csuka, E., Ammann, E., et al. (1997). Production of cytokines following brain injury: beneficial and deleterious for the damaged tissue. Mol. Psychiatry 2, 133–136. doi: 10.1038/sj.mp.4000227
Muili, K. A., Gopalakrishnan, S., Meyer, S. L., Eells, J. T., and Lyons, J. A. (2012). Amelioration of experimental autoimmune encephalomyelitis in C57BL/6 mice by photobiomodulation induced by 670 nm light. PLoS ONE 7, e30655. doi: 10.1371/journal.pone.0030655
Narita, M., Shimamura, M., Imai, S., Kubota, C., Yajima, Y., Takagi, T., et al. (2008). Role of interleukin-1β and tumor necrosis factor-α-dependent expression of cyclooxygenase-2 mRNA in thermal hyperalgesia induced by chronic inflammation in mice. Neuroscience 152, 477–486. doi: 10.1016/j.neuroscience.2007.10.039
Norden, D. M., Trojanowski, P. J., Villanueva, E., Navarro, E., and Godbout, J. P. (2016). Sequential activation of microglia and astrocyte cytokine expression precedes increased iba-1 or GFAP immunoreactivity following systemic immune challenge. Glia 64, 300–316. doi: 10.1002/glia.22930
O'Brien, J. A., and Austin, P. J. (2019). Effect of photobiomodulation in rescuing lipopolysaccharide-induced dopaminergic cell loss in the male Sprague–Dawley rat. Biomolecules 9, 381. doi: 10.3390/biom9080381
Orr, M. B., and Gensel, J. C. (2018). Spinal cord injury scarring and inflammation: therapies targeting glial and inflammatory responses. Neurotherapeutics 15, 541–553. doi: 10.1007/s13311-018-0631-6
Park, S. E., Sapkota, K., Kim, S., Kim, H., and Kim, S. J. (2011). Kaempferol acts through mitogen-activated protein kinases and protein kinase B/AKT to elicit protection in a model of neuroinflammation in BV2 microglial cells. Br. J. Pharmacol. 164, 1008–1025. doi: 10.1111/j.1476-5381.2011.01389.x
Pastore, D., Greco, M., Petragallo, V. A., and Passarella, S. (1994). Increase in H+/e-ratio of the cytochrome c oxidase reaction in mitochondria irradiated with helium-neon laser. Biochem. Mol. Biol. Int. 34, 817–826.
Plass, C. A., Loew, H. G., Podesser, B. K., and Prusa, A. M. (2012). Light-induced vasodilation of coronary arteries and its possible clinical implication. Ann. Thorac. Surg. 93, 1181–1186. doi: 10.1016/j.athoracsur.2011.12.062
Prianti, A. C. G., Silva, J. A., Dos Santos, R. F., Rosseti, I. B., and Costa, M. S. (2014). Low-level laser therapy (LLLT) reduces the COX-2 mRNA expression in both subplantar and total brain tissues in the model of peripheral inflammation induced by administration of carrageenan. Lasers Med. Sci. 29, 1397–1403. doi: 10.1007/s10103-014-1543-2
Ravizza, T., Noé, F., Zardoni, D., Vaghi, V., Sifringer, M., and Vezzani, A. (2008). Interleukin converting enzyme inhibition impairs kindling epileptogenesis in rats by blocking astrocytic IL-1β production. Neurobiol. Dis. 31, 327–333. doi: 10.1016/j.nbd.2008.05.007
Rojas, J. C., and Gonzalez-Lima, F. (2011). Low-level light therapy of the eye and brain. Eye Brain 3, 49–67. doi: 10.2147/EB.S21391
Rojas, J. C., and Gonzalez-Lima, F. (2013). Neurological and psychological applications of transcranial lasers and LEDs. Biochem. Pharmacol. 86, 447–457. doi: 10.1016/j.bcp.2013.06.012
Salehpour, F., Farajdokht, F., Cassano, P., Sadigh-Eteghad, S., Erfani, M., Hamblin, M. R., et al. (2019a). Near-infrared photobiomodulation combined with coenzyme Q10 for depression in a mouse model of restraint stress: reduction in oxidative stress, neuroinflammation, and apoptosis. Brain Res. Bull. 144, 213–222. doi: 10.1016/j.brainresbull.2018.10.010
Salehpour, F., Farajdokht, F., Mahmoudi, J., Erfani, M., Farhoudi, M., Karimi, P., et al. (2019b). Photobiomodulation and coenzyme Q10 treatments attenuate cognitive impairment associated with model of transient global brain ischemia in artificially aged mice. Front. Cell. Neurosci. 13, 74. doi: 10.3389/fncel.2019.00074
Samson, Y., Lapergue, B., and Hosseini, H. (2005). Inflammation and ischaemic stroke: current status and future perspectives. Rev Neurol. 161(12 Pt 1), 1177–1182. doi: 10.1016/S0035-3787(05)85190-2
Saucedo, C. L., Courtois, E. C., Wade, Z. S., Kelley, M. N., Kheradbin, N., Barrett, D. W., et al. (2021). Transcranial laser stimulation: mitochondrial and cerebrovascular effects in younger and older healthy adults. Brain Stimul. 14, 440–449. doi: 10.1016/j.brs.2021.02.011
Schain, M., and Kreisl, W. C. (2017). Neuroinflammation in neurodegenerative disorders—a review. Curr. Neurol. Neurosci. Rep. 17, 25. doi: 10.1007/s11910-017-0733-2
Schuligoi, R., Ulcar, R., Peskar, B. A., and Amann, R. (2003). Effect of endotoxin treatment on the expression on cyclooxygenase-2 and prostaglandin synthases in spinal cord, dorsal root ganglia, and skin of rats. Neuroscience 116, 1043–1052. doi: 10.1016/S0306-4522(02)00783-2
Semple, B. D., Bye, N., Rancan, M., Ziebell, J. M., and Morganti-Kossmann, M. C. (2010). Role of CCL2 (MCP-1) in traumatic brain injury (TBI): evidence from severe TBI patients and CCL2–/– mice. J. Cereb. Blood Flow Metab. 30, 769–782. doi: 10.1038/jcbfm.2009.262
Shabab, T., Khanabdali, R., Moghadamtousi, S. Z., Kadir, H. A., and Mohan, G. (2017). Neuroinflammation pathways: a general review. Int. J. Neurosci. 127, 624–633. doi: 10.1080/00207454.2016.1212854
Swaroop, S., Sengupta, N., Suryawanshi, A. R., Adlakha, Y. K., and Basu, A. (2016). HSP60 plays a regulatory role in IL-1β-induced microglial inflammation via TLR4-p38 MAPK axis. J. Neuroinflammation 13, 1–19. doi: 10.1186/s12974-016-0486-x
Tian, F., Hase, S. N., Gonzalez-Lima, F., and Liu, H. (2016). Transcranial laser stimulation improves human cerebral oxygenation. Lasers Surg. Med. 48, 343–349. doi: 10.1002/lsm.22471
Tsai, C. M., Chang, S. F., Li, C. C., and Chang, H. (2022). Transcranial photobiomodulation (808 nm) attenuates pentylenetetrazole-induced seizures by suppressing hippocampal neuroinflammation, astrogliosis, and microgliosis in peripubertal rats. Neurophotonics 9, 015006. doi: 10.1117/1.NPh.9.1.015006
Vezzani, A., and Friedman, A. (2011). Brain inflammation as a biomarker in epilepsy. Biomark. Med. 5, 607–614. doi: 10.2217/bmm.11.61
Vezzani, A., Lang, B., and Aronica, E. (2016). Immunity and inflammation in epilepsy. Cold Spring Harb. Perspect. Med. 6, a022699. doi: 10.1101/cshperspect.a022699
Villanueva, R. (2013). Neurobiology of major depressive disorder. Neural Plast. 2013, 873278. doi: 10.1155/2013/873278
Voet, S., Prinz, M., and van Loo, G. (2019). Microglia in central nervous system inflammation and multiple sclerosis pathology. Trends Mol. Med. 25, 112–123. doi: 10.1016/j.molmed.2018.11.005
Vogel, D. D., Ortiz-Villatoro, N. N., Araújo, N. S., Marques, M. J. G., Aimbire, F., Scorza, F. A., et al. (2021). Transcranial low-level laser therapy in an in vivo model of stroke: Relevance to the brain infarct, microglia activation and neuroinflammation. J. Biophotonics 14, e202000500. doi: 10.1002/jbio.202000500
Wang, Q., Tang, X. N., and Yenari, M. A. (2007). The inflammatory response in stroke. J. Neuroimmunol. 184, 53–68. doi: 10.1016/j.jneuroim.2006.11.014
Wang, X., Li, X., Zuo, X., Liang, Z., Ding, T., Li, K., et al. (2021). Photobiomodulation inhibits the activation of neurotoxic microglia and astrocytes by inhibiting Lcn2/JAK2-STAT3 crosstalk after spinal cord injury in male rats. J. Neuroinflammation 18, 1–20. doi: 10.1186/s12974-021-02312-x
Wang, X., Reddy, D. D., Nalawade, S. S., Pal, S., Gonzalez-Lima, F., and Liu, H. (2017b). Impact of heat on metabolic and hemodynamic changes in transcranial infrared laser stimulation measured by broadband near-infrared spectroscopy. Neurophotonics 5, 011004. doi: 10.1117/1.NPh.5.1.011004
Wang, X., Tian, F., Reddy, D. D., Nalawade, S. S., Barrett, D. W., Gonzalez-Lima, F., et al. (2017a). Up-regulation of cerebral cytochrome-c-oxidase and hemodynamics by transcranial infrared laser stimulation: a broadband near-infrared spectroscopy study. J. Cereb. Blood Flow Metab. 37, 3789–3802. doi: 10.1177/0271678X17691783
White, T. E., Ford, G. D., Surles-Zeigler, M. C., Gates, A. S., LaPlaca, M. C., and Ford, B. D. (2013). Gene expression patterns following unilateral traumatic brain injury reveals a local pro-inflammatory and remote anti-inflammatory response. BMC Genomics 14, 1–21. doi: 10.1186/1471-2164-14-282
Woodcock, T., and Morganti-Kossmann, C. (2013). The role of markers of inflammation in traumatic brain injury. Front. Neurol. 4, 18. doi: 10.3389/fneur.2013.00018
Wu, X., Shen, Q., Zhang, Z., Zhang, D., Gu, Y., and Xing, D. (2021). Photoactivation of TGFβ/SMAD signaling pathway ameliorates adult hippocampal neurogenesis in Alzheimer's disease model. Stem Cell Res. Ther. 12, 1–19. doi: 10.1186/s13287-021-02399-2
Yang, L., Dong, Y., Wu, C., Youngblood, H., Li, Y., Zong, X., et al. (2021a). Effects of prenatal photobiomodulation treatment on neonatal hypoxic ischemia in rat offspring. Theranostics 11, 1269. doi: 10.7150/thno.49672
Yang, L., Tucker, D., Dong, Y., Wu, C., Lu, Y., Li, Y., et al. (2018). Photobiomodulation therapy promotes neurogenesis by improving post-stroke local microenvironment and stimulating neuroprogenitor cells. Exp. Neurol. 299, 86–96. doi: 10.1016/j.expneurol.2017.10.013
Yang, L., Wu, C., Parker, E., Li, Y., Dong, Y., Tucker, L., et al. (2022). Non-invasive photobiomodulation treatment in an Alzheimer Disease-like transgenic rat model. Theranostics 12, 2205. doi: 10.7150/thno.70756
Yang, L., Wu, C., Tucker, L., Dong, Y., Li, Y., Xu, P., et al. (2021b). Photobiomodulation therapy attenuates anxious-depressive-like behavior in the TgF344 rat model. J Alzheimer's Dis. 83, 1415–1429. doi: 10.3233/JAD-201616
Yilmaz, G., and Granger, D. N. (2008). Cell adhesion molecules and ischemic stroke. Neurol. Res. 30, 783–793. doi: 10.1179/174313208X341085
Yoshimura, T. M., Sabino, C. P., and Ribeiro, M. S. (2016). Photobiomodulation reduces abdominal adipose tissue inflammatory infiltrate of diet-induced obese and hyperglycemic mice. J. Biophotonics 9, 1255–1262. doi: 10.1002/jbio.201600088
Keywords: photobiomodulation, low-level laser (light) therapy, brain, neuroinflammation, inflammation, cytokine, microglia
Citation: Cardoso FdS, Salehpour F, Coimbra NC, Gonzalez-Lima F and Gomes da Silva S (2022) Photobiomodulation for the treatment of neuroinflammation: A systematic review of controlled laboratory animal studies. Front. Neurosci. 16:1006031. doi: 10.3389/fnins.2022.1006031
Received: 28 July 2022; Accepted: 31 August 2022;
Published: 20 September 2022.
Edited by:
John Mitrofanis, Université Grenoble Alpes, FranceReviewed by:
Lew Lim, Vielight Inc., CanadaDan Johnstone, The University of Newcastle, Australia
Ann Liebert, Sydney Adventist Hospital, Australia
Copyright © 2022 Cardoso, Salehpour, Coimbra, Gonzalez-Lima and Gomes da Silva. This is an open-access article distributed under the terms of the Creative Commons Attribution License (CC BY). The use, distribution or reproduction in other forums is permitted, provided the original author(s) and the copyright owner(s) are credited and that the original publication in this journal is cited, in accordance with accepted academic practice. No use, distribution or reproduction is permitted which does not comply with these terms.
*Correspondence: Fabrízio dos Santos Cardoso, fabriziooscardoso@yahoo.com.br