- Facultad de Medicina, Instituto de Investigación en Discapacidades Neurológicas (IDINE), Universidad de Castilla-La Mancha, Albacete, Spain
As it is well known, a worldwide improvement in life expectancy has taken place. This has brought an increase in chronic pathologies associated with aging. Cardiovascular, musculoskeletal, psychiatric, and neurodegenerative conditions are common in elderly subjects. As far as neurodegenerative diseases are concerned dementias and particularly, Alzheimer’s disease (AD) occupy a central epidemiological position given their high prevalence and their profound negative impact on the quality of life and life expectancy. The amyloid cascade hypothesis partly explains the immediate cause of AD. However, limited therapeutical success based on this hypothesis suggests more complex remote mechanisms underlying its genesis and development. For instance, the strong association of AD with another irreversible neurodegenerative pathology, without curative treatment and complex etiology such as presbycusis, reaffirms the intricate nature of the etiopathogenesis of AD. Recently, oxidative stress and frailty syndrome have been proposed, independently, as key factors underlying the onset and/or development of AD and presbycusis. Therefore, the present review summarizes recent findings about the etiology of the above-mentioned neurodegenerative diseases, providing a critical view of the possible interplay among oxidative stress, frailty syndrome, AD and presbycusis, that may help to unravel the common mechanisms shared by both pathologies. This knowledge would help to design new possible therapeutic strategies that in turn, will improve the quality of life of these patients.
Introduction: The Neurobiological Missing Links Between Age-Related Hearing Loss and Alzheimer’s Disease
Age-related hearing loss (ARHL) or presbycusis is a major public health burden worldwide that profoundly affects the quality of life of those who suffer from it (Mathers et al., 2000, 2008; World Health Organization [WHO], 2002, 2021). It is a progressive, chronic, and irreversible condition, which should be construed as a neurodegenerative disease, for which no curative treatment is available yet (Yamasoba et al., 2013; Melgar-Rojas et al., 2015; Parham et al., 2015; Wang and Puel, 2020). Also, it is the most frequent sensory impairment in the elderly. Estimates are that 4 out of 10 adults aged 60 years or older have some degree of limiting hearing loss (World Health Organization [WHO], 2021). Moreover, the prevalence of disabling hearing loss has been estimated at about 15% at 60 years of age, increasing up to 58% at 90 years of age, with all the consequences that this entails (World Health Organization [WHO], 2021). AD is also a progressive, chronic, and irreversible pathology without curative treatment. It represents around 70% of dementias, which affect about 55 million people worldwide (World Health Organization [WHO], 2017a; Zheng et al., 2017; Shen et al., 2018; Ralli et al., 2019; Guo et al., 2020). Life quality is severely affected since AD interferes with the ability to perform daily life activities. Therefore, autonomy and life expectancy are severely compromised (World Health Organization [WHO], 2017a; Guo et al., 2020).
Epidemiological studies show a strong independent association between ARHL and AD (Lin et al., 2011; Lin and Albert, 2014). Actually, recent data link 9% of sporadic AD to hearing loss starting at mid-life. Thus, ARHL emerges as the main preventable risk factor of AD, at least in this life period, even with causal implications (Livingston et al., 2017; Loughrey et al., 2018). Comorbidity between ARHL and AD will further aggravate the condition of the patients, multiplying health, social, economic, and sanitary impact. In sum, epidemiological data link ARHL with cognitive impairment and dementias, in particular AD, pointing to dynamic association between these two neurodegenerative conditions (Fortunato et al., 2016; Zheng et al., 2017; Shen et al., 2018; Panza et al., 2019; Ralli et al., 2019; Uchida et al., 2019; Guo et al., 2020; Llano et al., 2020; Slade et al., 2020; Johnson et al., 2021). Besides ARHL contributing to the pathogenesis of AD, the converse may also be the case. However, at present, the biological or mechanistic foundations of such interplay are unknown (Griffiths et al., 2020; Nadhimi and Llano, 2021). Several hypotheses/mechanisms have been put forth. These include existence of shared underlying pathologies, such as those of vascular origin; diminished auditory input that directly triggers brain atrophy as an expression of the complex chain of cellular events leading to dementia; overload of cognitive resources, diverted to process diminished auditory input (Uchida et al., 2019; Griffiths et al., 2020); existence of amyloid plaques (AP), intraneuronal neurofibrillary tangles (NFT) and cytoskeletal pathology in the cochlea, dorsal cochlear nucleus, superior olive, central nucleus of the inferior colliculus, medial geniculate body, primary auditory cortex and association area of the auditory cortex (Omata et al., 2016; Shen et al., 2018). These or another related hypothesis/mechanism do not exclude each other mutually. Whether such interplay is unidirectional from ARHL to AD, or bidirectional is also unknown. The challenge of testing such intricate and open-end hypotheses scenery, is the complexity and multiplicity of factors involved in the genesis and development of both neurodegenerative conditions. Frailty and related oxidative stress have recently drawn considerable attention (Kawada et al., 2015; Panza et al., 2015a,b; Huang et al., 2016; Shen et al., 2018; Wang and Puel, 2020; Misrani et al., 2021). In this review, we discuss the possibility that the oxidative stress linked to frailty, could be, at least in part, primarily involved in the interplay between ARHL and AD. We stress the need for the development and use of rodent models to target and integrate in a translational approach molecular, cellular, and behavioral mechanisms at the interphase between ARHL and AD.
Oxidative Stress in Age-Related Hearing Loss and Alzheimer’s Disease
When the endogenous antioxidant system is overcome either by production of excess free radicals, essentially reactive oxygen and nitrogen species (ROS/RNS), limited free radical handling or both, accumulation of toxic free radicals occurs, leading to oxidative stress-induced damage to lipids and proteins in cell membranes and the cytosol, as well as to the nuclear and mitochondrial genome (Ames et al., 1993; Halliwell, 2006; Fetoni et al., 2011; Böttger and Schacht, 2013; Nimse and Pal, 2015). The deleterious effects that excess of free radicals has on cells, seemingly is critically associated with the aging process, the genesis and/or development of different neurodegenerative pathologies such as amyotrophic lateral sclerosis, Huntington, Parkinson’s and Alzheimer’s diseases and presbycusis (Ames et al., 1993; Seidman, 2000; Chauhan and Chauhan, 2006; Uttara et al., 2009; Dai et al., 2014; Huang et al., 2016).
In the auditory system, besides ARHL, oxidative stress is at the core of noise-induced hearing loss (NIHL) and drug-induced hearing loss (DIHL; Henderson et al., 2006; Le Prell et al., 2007a,b; Fetoni et al., 2013). This has led to the notion that oxidative stress is a common pathogenic pathway, shared by the above-mentioned auditory pathologies (Alvarado et al., 2015, 2018). In the peripheral auditory system, the cochlea is particularly exposed to damage from oxidative stress. This is likely due to the metabolic demands of mechanoelectrical transduction (Robles and Ruggero, 2001; Fettiplace and Kim, 2014), for which large electrochemical gradients have to be maintained, particularly for the generation of the endocochlear potential, the driving force behind mechanoelectrical transduction. Also, fast contractile properties of outer hair cells (OHCs) allowing them to act as active signal amplifiers (Robles and Ruggero, 2001), are energetically demanding. High physiological oxidative metabolism rates in the organ of Corti may result in increased production of free radicals, more demanding to keep within a normal range. Possible convergence with different scenarios of mitochondrial dysfunction (Böttger and Schacht, 2013) makes the auditory receptor prone to imbalances in the endogenous antioxidant system, which will lead to unchecked accumulation of ROS/RNS and damage sensory cells, stria vascularis and spiral ganglion cells, leading to altered auditory function (Henderson et al., 2006; Fetoni et al., 2013; Fujimoto and Yamasoba, 2014; Alvarado et al., 2015, 2018). Meanwhile, there is also mounting evidence that the central auditory pathway is affected directly by oxidative stress leading to increases in the expression of NADPH oxidase 2 and 8-hydroxy-2-deoxyguanosine, levels of lipid peroxidation and mitochondrial DNA deletion, and also to neurodegenerative changes in the cochlear nucleus, inferior colliculus and auditory cortex (Du et al., 2014; Li et al., 2018; Tavanai et al., 2019). Supporting this, antioxidant-based therapies should mitigate the hearing damage induced by oxidative stress. Accordingly, several antioxidant and combinations has proven to be effective, mainly at the level of proof principle, for the treatment of NIHL (Yamasoba et al., 1999; Kopke et al., 2000, 2007; Tanaka et al., 2005; Le Prell et al., 2007a,2011), DIHL (Campbell et al., 2007; Tokgoz et al., 2011; Le Prell et al., 2014) and ARHL (Alvarado et al., 2018; Cai et al., 2020). This confirms the central role that oxidative stress plays in the development and/or progression of many auditory pathologies.
In relation to AD, the view of the disease as a proteinopathy, summarized in the amyloid cascade and tauopathy hypotheses has been the source of essential advances in the understanding of the disease, in spite of yet limited therapeutical success (Karran et al., 2011). However, this should not obscure the fact that oxidative stress has also been implicated in the pathogenesis of AD. Actually, it has even been postulated that oxidative stress-induced damage precedes AP and NFT deposits, characteristic of this neurodegenerative disease. Several biomarkers of oxidative stress have been detected in blood, neural tissue or cerebrospinal fluid in AD patients, indicating lipid peroxidation (2-propenal, 4-hydroxynonenal, F2-isoprostanes, malondialdehyde), protein oxidation (3-nitrotyrosine) and DNA oxidation (8-hydroxydeoxyguanosine, 8-hydroxyguanosine), even at early stages of the disease (Perry et al., 2002; Castellani et al., 2006; Chauhan and Chauhan, 2006; Uttara et al., 2009; Gella and Bole, 2011; Chen and Zhong, 2014; Huang et al., 2016).
Although it is not clear which factors trigger the oxidative stress in AD, both the amyloid-β protein (Aβ), either in its soluble or fibrillar forms, and the hyperphosphorylated microtubule-stabilizing protein tau, that lead to NFT formation, contribute to such oxidative stress-induced damage. Aβ has binding sites with high affinity for metal ions such as iron, copper and zinc, which would produce chelation of the Aβ and consequently accumulation of AP, with highly toxic oxidative capacity for the neurons (Chauhan and Chauhan, 2006; Uttara et al., 2009; Gella and Bole, 2011; Chen and Zhong, 2014; Huang et al., 2016). The hyperphosphorylated tau, due to the oxidative imbalance, might be modified by a non-enzymatic glycation process, with formation of advanced glycation end products (AGEs; Perry et al., 2002; Castellani et al., 2006; Chauhan and Chauhan, 2006). The AGEs are highly reactive and thus, they would generate a large amount of ROS/RNS that are not only harmful for neurons “per se”, but could increase, even more, Aβ levels, thus generating a vicious circle that could worsen the manifestations of AD. Nevertheless, it is also worth noting that antioxidant properties have been attributed to Aβ and hyperphosphorylated tau, suggesting that they are also part of the endogenous neuronal protection system against oxidative stress (Zou et al., 2002; Castellani et al., 2006; Baruch-Suchodolsky and Fischer, 2009; Uttara et al., 2009; Gella and Bole, 2011). Therefore, increases in ROS/RNS levels would lead directly or indirectly to an increase in the levels of those two AD biomarkers. Without ruling out other possibilities, this “dual” role seems to be related in part to the aging process, as these molecules would exert their protective effect before aging when the disease is not present yet, while their deleterious effect occurs during aging after the onset of the disease (Zou et al., 2002; Castellani et al., 2006; Baruch-Suchodolsky and Fischer, 2009; Uttara et al., 2009; Gella and Bole, 2011). As opposed to ARHL, antioxidant-based therapies have not yielded the expected results in AD. However, similar to other therapies, failures can be attributed to experimental design, including late start of the therapies when the disease is already established, the individual and not combined use of micronutrients or the difficulty of crossing the blood-brain barrier, which would decrease its bioavailability at the brain level (Persson et al., 2014). Therefore, new studies avoiding these, and other limitations are needed in order to draw valid conclusions about the use of antioxidants as possible therapies for the treatment of AD.
Frailty Syndrome in Age-Related Hearing Loss and Alzheimer’s Disease
The World Health Organization (World Health Organization [WHO], 2017b), defines frailty as “a clinically recognizable state in which the ability of older people to cope with every day or acute stressors is compromised by an increased vulnerability brought by age-associated declines in physiological reserve and function across multiple organ systems”. Recent conceptualization currently limits consensus on how to diagnose frailty. Different models have been proposed, “the phenotype model” being one of the most used. In this model, physical/functional capacity is evaluated based on five criteria: weight loss, weakness, slowness, exhaustion, and low levels of physical activity. A patient is considered frail when presenting at least 3 and pre-frail if 1 or 2 of them are present (Xue, 2011; Wou and Conroy, 2013; Leng et al., 2014; Kane et al., 2016). It is important to note that although frailty mainly occurs during aging, frailty and aging are not synonymous and not all aged people will develop it, in fact it is estimated that, depending on diagnostic criteria, its prevalence varies between 4 to 59% among people 65 years or older (Kojima et al., 2019). Although frailty is a multifactorial condition that affects multiple organs and systems, underlying mechanisms are not clear. It has been proposed that pro-inflammatory states, sarcopenia, hormonal and metabolic imbalances, DNA damage, and more recently, oxidative stress are critical in the development of this pathology (Mulero et al., 2011; Xue, 2011; Inglés et al., 2014; Leng et al., 2014; Namioka et al., 2017; Soysal et al., 2017; World Health Organization [WHO], 2017b; Bisset and Howlett, 2019; El Assar et al., 2020). Regarding the latter, it has been suggested that it could be a primary mechanism triggering frailty, since oxidative stress-induced damage could serve as background or starting point of the multiple alterations described in subjects with frailty (Mulero et al., 2011; El Assar et al., 2020). For instance, while the oxidative stress-induced mitochondrial dysfunction could contribute to the distinctive sarcopenia that leads to weight loss, weakness and slowness, the DNA oxidation would induce the DNA damage observed in frailty (El Assar et al., 2020).
Given the relevance that, from the clinical point of view, frailty has for different age-related pathologies, the concept has evolved, incorporating in addition to the physical/functional domain, the emotional and cognitive, known as frailty syndrome (FS; Xue, 2011; Wou and Conroy, 2013; Leng et al., 2014; Khezrian et al., 2017; Kojima et al., 2019). It has been suggested that the FS, mainly the emotional and cognitive domains, are essential for the development or progression of AD, which has a high prevalence (32%) of coexisting frailty (Kojima et al., 2019). This is crucial considering that the potentially modifiable nature of the risk factors that could lead to emotional or cognitive frailty, might be useful to identify possible therapeutic targets for AD. For instance, it has been proposed that cognitive frailty is pivotal in the pathogenesis of AD. One of the key risk factors for the development of this frailty is hearing impairment and particularly, ARHL (Figure 1) (Panza et al., 2006, 2015a,b, 2019; Khezrian et al., 2017). As previously mentioned, epidemiological studies highlight the association between hearing loss in older adults with dementia and cognitive decline. In these subjects, the rate of cognitive decline is accelerated, increasing the risk of developing dementia, including AD, and this risk is proportional to the level of hearing loss observed (Lin et al., 2011; Lin and Albert, 2014; Deal et al., 2016; Thomson et al., 2017). The emergence of the concept of FS, opens the possibility that at least part of the high prevalence of hearing loss observed in patients with AD and its consequences for the progression of the disease may be amplified through FS, and this will need to be explored.
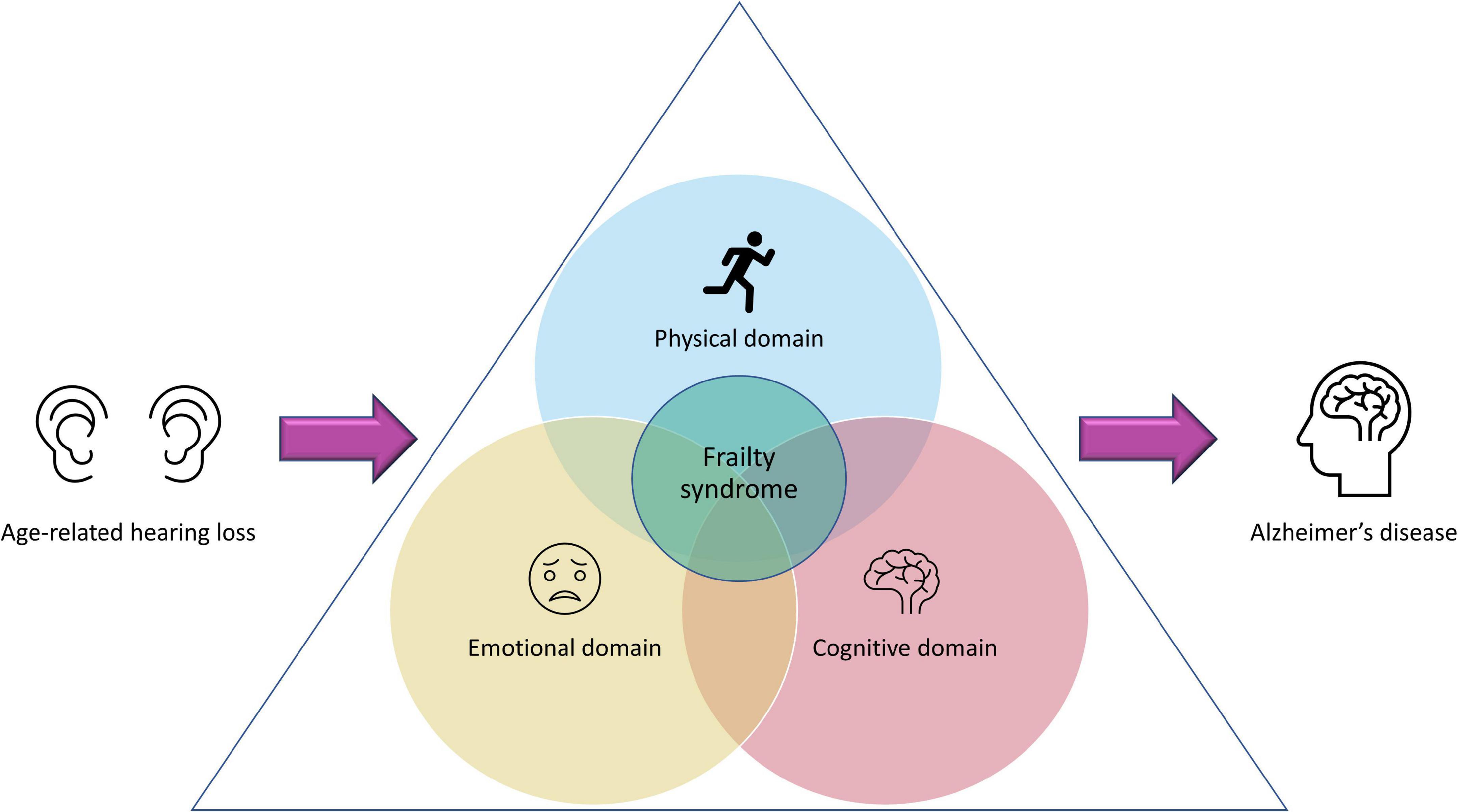
Figure 1. Age-related hearing loss and frailty syndrome. Presbycusis has been proposed as a preventable risk factor for the development of frailty. Depending on the magnitude of the hearing loss, its impact could go far beyond auditory dysfunction, affecting also the physical, emotional, and cognitive domains. If presbycusis-induced alterations of these three domains co-exist, this could lead to a “frailty syndrome” that, consequently, will have a profound negative impact in the aggravation of Alzheimer’s disease, if there is comorbidity between FS and AD.
Regarding the emotional frailty, ARHL has a profound impact on the quality of life of these patients causing low self-esteem, social withdrawal, isolation, frustration, and depression which would undoubtedly affect their emotional domain (World Health Organization [WHO], 2002, 2017a,b; Huang and Tang, 2010; Ciorba et al., 2012; Kidd and Bao, 2012), producing or aggravating the emotional frailty (Figure 1). Since emotional frailty can coexist with cognitive frailty (Khezrian et al., 2017), the latter can be affected, which would increase the risk of suffering from dementia and AD in older people. Finally, the relationship between ARHL and physical frailty has been less studied. However, there is no doubt that hearing loss in older people also has a negative impact on their physical activity. Accordingly, it has been suggested that ARHL: (1) induces to perform less physical activity because subjects are socially isolated; (2) affects cognitive resources and attention, which are essential for maintaining posture and balance and (3) restricts the ability to effectively monitor the environment (hearing footsteps and other auditory cues that provide guidance), which reduces the probability of engaging in physical activities (Gispen et al., 2014; Fortunato et al., 2016). Additionally, common neural degeneration, which affects both the cochlea and the vestibular organ, involved in controlling balance, may explain decreases in physical activity (Gispen et al., 2014; Fortunato et al., 2016). All these scenarios may influence the onset and/or progression of physical frailty (Figure 1). Finally, a high association between presbycusis and falls over time in older people has been described, which might have repercussions leading to decreased physical activity and therefore, worsening frailty (Kamil et al., 2016).
Conclusion
Oxidative imbalance and related FS due to it, may be part of multiple pathologies that affect geriatric patients. The oxidative imbalance, either due to overproduction or lack of elimination of ROS is critical not only for the onset and/or development of ARHL or AD but also for triggering FS (Figure 2). The latter is also affected by ARHL which in turn, enhances the frailty condition in older subjects. Considering that oxidative stress, ARHL and FS individually might be critical factors in the development of AD (Figure 2), their coexistence in the same patient would be decisive for the onset, progression, and severity of this neurodegenerative disease. More so if it is considered that dementias such as AD may negatively affect ARHL, generating a vicious circle between both conditions with devastating consequences. Hence, due to the complex relationship that exists between ARHL and AD in which oxidative stress and related FS may play unexplored roles, it would be logical to expect that therapies aimed at specific targets of a single pathogenic pathway would have little beneficial effect. Therefore, the optimal therapeutic approach should not only focus on a single pathway, instead it should be multifaceted, as the combination of therapeutic strategies aimed at different pathogenic pathways or different points of the same pathway would guarantee a synergistic interaction enhancing the beneficial effect of the therapy which in turn, would have a positive impact on the patient’s health quality of life.
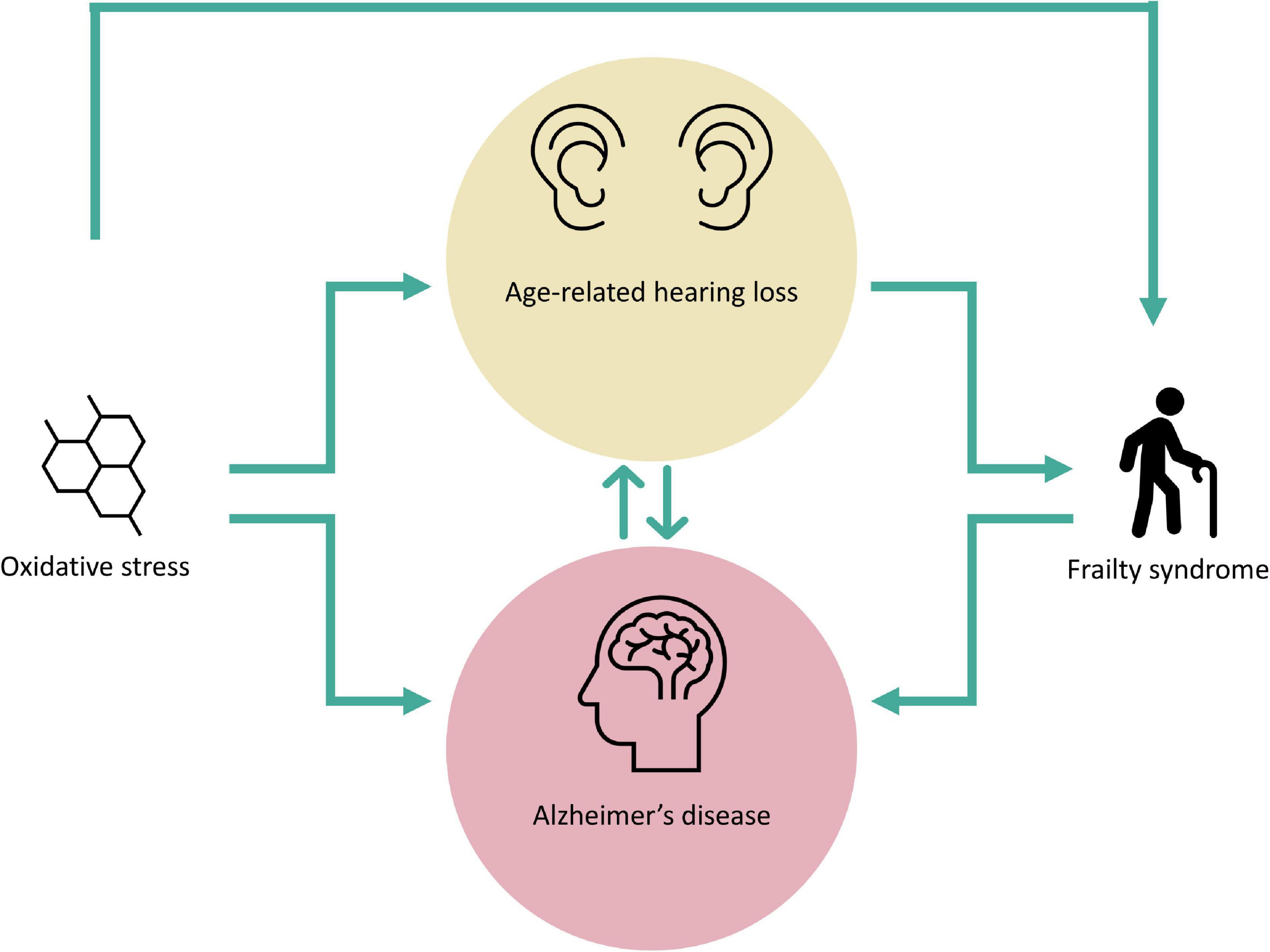
Figure 2. Oxidative stress and frailty syndrome in age-related hearing loss and Alzheimer’s disease. The figure shows the complex interplay among oxidative stress, frailty syndrome, age-related hearing loss and Alzheimer’s disease. Oxidative stress-damage represents a possible trigger for both age-related hearing loss and Alzheimer’s disease, and also for the genesis of frailty syndrome. Presbycusis is a fundamental risk factor for the development of Alzheimer’s disease and frailty syndrome while, frailty syndrome may contribute to the exacerbation of Alzheimer’s disease. Note a hypothetical biunivocal relationship between age-related hearing loss and Alzheimer’s disease, as these neurodegenerative pathological conditions may influence each other.
To achieve the goal of unveiling pathogenic mechanisms of AD in relation to ARHL, it is necessary to design studies in appropriate animal models reproducing the pathological complexities of AD, in particular comorbidity with ARHL, oxidative stress and frailty syndrome. Such animal models, besides presenting clinical and biological markers of AD, must reproduce ARHL traits, comparable to those found in humans. The results obtained from such studies will allow a more “humanized” and reality-adjusted analysis of the data that will help to comprehend the interplay between these important neurodegenerative pathologies, which will facilitate a better understanding of the etiopathogenic mechanisms involved in their onset, progression, and severity.
Author Contributions
JA and VF-S: drafting of the manuscript and design of figures. JA, VF-S, and JJ: critical revision of the manuscript for important intellectual content. All authors contributed to the article and approved the submitted version.
Funding
This study was supported by grant SBPLY/17/180501/000544 from the regional government of Castilla-La Mancha (Gobierno de Castilla-La Mancha, Consejería de Educación y Ciencia).
Conflict of Interest
The authors declare that the research was conducted in the absence of any commercial or financial relationships that could be construed as a potential conflict of interest.
Publisher’s Note
All claims expressed in this article are solely those of the authors and do not necessarily represent those of their affiliated organizations, or those of the publisher, the editors and the reviewers. Any product that may be evaluated in this article, or claim that may be made by its manufacturer, is not guaranteed or endorsed by the publisher.
References
Alvarado, J. C., Fuentes-Santamaría, V., Gabaldón-Ull, M. C., and Juiz, J. M. (2018). An Oral Combination of Vitamins A, C, E, and Mg++ Improves Auditory Thresholds in Age-Related Hearing Loss. Front. Neurosci. 12:527. doi: 10.3389/fnins.2018.00527
Alvarado, J. C., Fuentes-Santamaría, V., Melgar-Rojas, P., Valero, M. L., Gabaldón-Ull, M. C., Miller, J. M., et al. (2015). Synergistic effects of free radical scavengers and cochlear vasodilators: a new otoprotective strategy for age-related hearing loss. Front. Aging Neurosci. 7:86. doi: 10.3389/fnagi.2015.00086
Ames, B. N., Shigenaga, M. K., and Hagen, T. M. (1993). Oxidants, antioxidants, and the degenerative diseases of aging. Proc. Natl. Acad. Sci. U. S. A. 90, 7915–7922.
Baruch-Suchodolsky, R., and Fischer, B. (2009). Aβ 40, either Soluble or Aggregated, Is a Remarkably Potent Antioxidant in Cell-Free Oxidative Systems. Biochemistry 48, 4354–4370. doi: 10.1021/bi802361k
Bisset, E. S., and Howlett, S. E. (2019). The biology of frailty in humans and animals: understanding frailty and promoting translation. Aging Med. 2, 27–34. doi: 10.1002/agm2.12058
Böttger, E. C., and Schacht, J. (2013). The mitochondrion: a perpetrator of acquired hearing loss. Hear. Res. 303, 12–19. doi: 10.1016/j.heares.2013.01.006
Cai, H., Han, B., Hu, Y., Zhao, X., He, Z., Chen, X., et al. (2020). Metformin attenuates the D-galactose-induced aging process via the UPR through the AMPK/ERK1/2 signaling pathways. Int. J. Mol. Med. 45, 715–730. doi: 10.3892/ijmm.2020.4453
Campbell, K. C. M., Meech, R. P., Klemens, J. J., Gerberi, M. T., Dyrstad, S. S. W., Larsen, D. L., et al. (2007). Prevention of noise- and drug-induced hearing loss with d-methionine. Hear. Res. 226, 92–103. doi: 10.1016/j.heares.2006.11.012
Castellani, R. J., Lee, H.-G., Zhu, X., Nunomura, A., Perry, G., and Smith, M. A. (2006). Neuropathology of Alzheimer disease: pathognomonic but not pathogenic. Acta Neuropathol. 111, 503–509. doi: 10.1007/s00401-006-0071-y
Chauhan, V., and Chauhan, A. (2006). Oxidative stress in Alzheimer’s disease. Pathophysiology 13, 195–208. doi: 10.1016/j.pathophys.2006.05.004
Chen, Z., and Zhong, C. (2014). Oxidative stress in Alzheimer’s disease. Neurosci. Bull. 30, 271–281. doi: 10.1007/s12264-013-1423-y
Ciorba, A., Bianchini, C., Pelucchi, S., and Pastore, A. (2012). The impact of hearing loss on the quality of life of elderly adults. Clin. Interv. Aging 7, 159–163. doi: 10.2147/CIA.S26059
Dai, D.-F., Chiao, Y., Marcinek, D. J., Szeto, H. H., and Rabinovitch, P. S. (2014). Mitochondrial oxidative stress in aging and healthspan. Longev. Healthspan 3:6. doi: 10.1186/2046-2395-3-6
Deal, J. A., Betz, J., Yaffe, K., Harris, T., Purchase-Helzner, E., Satterfield, S., et al. (2016). Hearing Impairment and Incident Dementia and Cognitive Decline in Older Adults: the Health ABC Study. J. Gerontol. A Biol. Sci. Med. Sci. 72, 703–709. doi: 10.1093/gerona/glw069
Du, Z., Yang, Q., Zhou, T., Liu, L., Li, S., Chen, S., et al. (2014). D-galactose-induced mitochondrial DNA oxidative damage in the auditory cortex of rats. Mol. Med. Rep. 10, 2861–2867. doi: 10.3892/mmr.2014.2653
El Assar, M., Angulo, J., and Rodríguez-Mañas, L. (2020). Frailty as a phenotypic manifestation of underlying oxidative stress. Free Radic. Biol. Med. 149, 72–77. doi: 10.1016/j.freeradbiomed.2019.08.011
Fetoni, A. R., De Bartolo, P., Eramo, S. L. M., Rolesi, R., Paciello, F., Bergamini, C., et al. (2013). Noise-Induced Hearing Loss (NIHL) as a Target of Oxidative Stress-Mediated Damage: cochlear and Cortical Responses after an Increase in Antioxidant Defense. J. Neurosci. 33, 4011–4023. doi: 10.1523/JNEUROSCI.2282-12.2013
Fetoni, A. R., Picciotti, P. M., Paludetti, G., and Troiani, D. (2011). Pathogenesis of presbycusis in animal models: a review. Exp. Gerontol. 46, 413–425. doi: 10.1016/j.exger.2010.12.003
Fettiplace, R., and Kim, K. X. (2014). The Physiology of Mechanoelectrical Transduction Channels in Hearing. Physiol. Rev. 94, 951–986.
Fortunato, S., Forli, F., Guglielmi, V., De Corso, E., Paludetti, G., Berrettini, S., et al. (2016). A review of new insights on the association between hearing loss and cognitive decline in ageing. Acta Otorhinolaryngol. Ital. 36, 155–166. doi: 10.14639/0392-100X-993
Fujimoto, C., and Yamasoba, T. (2014). Oxidative Stresses and Mitochondrial Dysfunction in Age-Related Hearing Loss. Oxid. Med. Cell. Longev. 2014, 1–6. doi: 10.1155/2014/582849
Gella, A., and Bole, I. (2011). “Oxidative Stress in Alzheimer’s Disease: pathogenesis, Biomarkers and Therapy,” in Alzheimer’s Disease Pathogenesis-Core Concepts, Shifting Paradigms and Therapeutic Targets, ed. S. De La Monte (London, UK: InTech), doi: 10.5772/16683
Gispen, F. E., Chen, D. S., Genther, D. J., and Lin, F. R. (2014). Association Between Hearing Impairment and Lower Levels of Physical Activity in Older Adults. J. Am. Geriatr. Soc. 62, 1427–1433. doi: 10.1111/jgs.12938
Griffiths, T. D., Lad, M., Kumar, S., Holmes, E., McMurray, B., Maguire, E. A., et al. (2020). How can hearing loss cause dementia? Neuron 108, 401–412. doi: 10.1016/j.neuron.2020.08.003
Guo, T., Zhang, D., Zeng, Y., Huang, T. Y., Xu, H., and Zhao, Y. (2020). Molecular and cellular mechanisms underlying the pathogenesis of Alzheimer’s disease. Mol. Neurodegener. 15:40. doi: 10.1186/s13024-020-00391-7
Halliwell, B. (2006). Reactive Species and Antioxidants. Redox Biology Is a Fundamental Theme of Aerobic Life. Plant Physiol. 141, 312–322. doi: 10.1104/pp.106.077073
Henderson, D., Bielefeld, E. C., Harris, K. C., and Hu, B. H. (2006). The role of oxidative stress in noise-induced hearing loss. Ear Hear. 27, 1–19. doi: 10.1097/01.aud.0000191942.36672.f3
Huang, Q., and Tang, J. (2010). Age-related hearing loss or presbycusis. Eur. Arch. Otorhinolaryngol. 267, 1179–1191. doi: 10.1007/s00405-010-1270-7
Huang, W.-J., Zhang, X., and Chen, W.-W. (2016). Role of oxidative stress in Alzheimer’s disease. Biomed. Rep. 4, 519–522. doi: 10.3892/br.2016.630
Inglés, M., Gambini, J., Carnicero, J. A., García-García, F. J., Rodríguez-Mañas, L., Olaso-González, G., et al. (2014). Oxidative Stress Is Related to Frailty, Not to Age or Sex, in a Geriatric Population: lipid and Protein Oxidation as Biomarkers of Frailty. J. Am. Geriatr. Soc. 62, 1324–1328. doi: 10.1111/jgs.12876
Johnson, J. C. S., Marshall, C. R., Weil, R. S., Bamiou, D.-E., Hardy, C. J. D., and Warren, J. D. (2021). Hearing and dementia: from ears to brain. Brain 144, 391–401. doi: 10.1093/brain/awaa429
Kamil, R. J., Betz, J., Powers, B. B., Pratt, S., Kritchevsky, S., Ayonayon, H. N., et al. (2016). Association of Hearing Impairment With Incident Frailty and Falls in Older Adults. J. Aging Health 28, 644–660. doi: 10.1177/0898264315608730
Kane, A., Hilmer, S., Mach, J., Mitchell, S., de Cabo, R., and Howlett, S. (2016). Animal models of frailty: current applications in clinical research. Clin. Interv. Aging 11, 1519–1529. doi: 10.2147/CIA.S105714
Karran, E., Mercken, M., and Strooper, B. D. (2011). The amyloid cascade hypothesis for Alzheimer’s disease: an appraisal for the development of therapeutics. Nat. Rev. Drug Discov. 10, 698–712. doi: 10.1038/nrd3505
Kawada, H., Blessing, K., Kiyota, T., Woolman, T., Winchester, L., and Kador, P. F. (2015). Effects of Multifunctional Antioxidants on Mitochondrial Dysfunction and Amyloid-β Metal Dyshomeostasis. J. Alzheimers Dis. 44, 297–307. doi: 10.3233/JAD-132471
Khezrian, M., Myint, P. K., McNeil, C., and Murray, A. D. (2017). A Review of Frailty Syndrome and Its Physical, Cognitive and Emotional Domains in the Elderly. Geriatrics 2:36. doi: 10.3390/geriatrics2040036
Kidd, A. R. III, and Bao, J. (2012). Recent Advances in the Study of Age-Related Hearing Loss: a Mini-Review. Gerontology 58, 490–496. doi: 10.1159/000338588
Kojima, G., Liljas, A., and Iliffe, S. (2019). Frailty syndrome: implications and challenges for health care policy. Risk Manag. Healthc. Policy 12, 23–30. doi: 10.2147/RMHP.S168750
Kopke, R. D., Jackson, R. L., Coleman, J. K. M., Liu, J., Bielefeld, E. C., and Balough, B. J. (2007). NAC for noise: from the bench top to the clinic. Hear. Res. 226, 114–125. doi: 10.1016/j.heares.2006.10.008
Kopke, R. D., Weisskopf, P. A., Boone, J. L., Jackson, R. L., Wester, D. C., Hoffer, M. E., et al. (2000). Reduction of noise-induced hearing loss using L-NAC and salicylate in the chinchilla. Hear. Res. 149, 138–146. doi: 10.1016/S0378-5955(00)00176-3
Le Prell, C. G., Dolan, D. F., Bennett, D. C., and Boxer, P. A. (2011). Nutrient plasma levels achieved during treatment that reduces noise-induced hearing loss. Transl. Res. 158, 54–70. doi: 10.1016/j.trsl.2011.02.003
Le Prell, C. G., Hughes, L., and Miller, J. M. (2007a). Free radical scavengers vitamins A, C, and E plus magnesium reduce noise trauma. Free Radic. Biol. Med. 42, 1454–1463. doi: 10.1016/j.freeradbiomed.2007.02.008
Le Prell, C. G., Yamashita, D., Minami, S. B., Yamasoba, T., and Miller, J. M. (2007b). Mechanisms of noise-induced hearing loss indicate multiple methods of prevention. Hear. Res. 226, 22–43. doi: 10.1016/j.heares.2006.10.006
Le Prell, C. G., Ojano-Dirain, C., Rudnick, E. W., Nelson, M. A., DeRemer, S. J., Prieskorn, D. M., et al. (2014). Assessment of Nutrient Supplement to Reduce Gentamicin-Induced Ototoxicity. J. Assoc. Res. Otolaryngol. 15, 375–393.
Leng, S., Chen, X., and Mao, G. (2014). Frailty syndrome: an overview. Clin. Interv. Aging 9, 433–441. doi: 10.2147/CIA.S45300
Li, Y., Zhao, X., Hu, Y., Sun, H., He, Z., Yuan, J., et al. (2018). Age-associated decline in Nrf2 signaling and associated mtDNA damage may be involved in the degeneration of the auditory cortex: implications for central presbycusis. Int. J. Mol. Med. 42, 3371–3385. doi: 10.3892/ijmm.2018.3907
Lin, F. R., and Albert, M. (2014). Hearing loss and dementia – who is listening? Aging Ment. Health 18, 671–673. doi: 10.1080/13607863.2014.915924
Lin, F. R., Metter, E. J., O’Brien, R. J., Resnick, S. M., Zonderman, A. B., and Ferrucci, L. (2011). Hearing Loss and Incident Dementia. Arch. Neurol. 68, 214–220. doi: 10.1001/archneurol.2010.362
Livingston, G., Sommerlad, A., Orgeta, V., Costafreda, S. G., Huntley, J., Ames, D., et al. (2017). Dementia prevention, intervention, and care. Lancet 390, 2673–2734. doi: 10.1016/S0140-6736(17)31363-6
Llano, D., Issa, L., Devanarayan, P., and Devanarayan, V., and Alzheimer’s Disease Neuroimaging Initiative [ADNI] (2020). Hearing Loss in Alzheimer’s Disease Is Associated with Altered Serum Lipidomic Biomarker Profiles. Cells 9:2556. doi: 10.3390/cells9122556
Loughrey, D. G., Kelly, M. E., Kelley, G. A., Brennan, S., and Lawlor, B. A. (2018). Association of Age-Related Hearing Loss With Cognitive Function, Cognitive Impairment, and Dementia: a Systematic Review and Meta-analysis. JAMA Otolaryngol. Neck Surg. 144:115. doi: 10.1001/jamaoto.2017.2513
Mathers, C., Fat, D. M., and Boerma, J. T., and World Health Organization [WHO] (eds) (2008). The global burden of disease: 2004 update. Geneva, Switzerland: World Health Organization.
Mathers, C., Smith, A., and Concha, M. (2000). Global burden of hearing loss in the year 2000. Glob. Burd. Dis. 18, 1–30.
Melgar-Rojas, P., Alvarado, J. C., Fuentes-Santamaría, V., and Juiz, J. M. (2015). “Cellular Mechanisms of Age-Related Hearing Loss,” in Free Radicals in ENT Pathology, eds J. M. Miller, C. G. Le Prell, and L. Rybak (Cham: Springer International Publishing), 305–333. doi: 10.1007/978-3-319-13473-4_15
Misrani, A., Tabassum, S., and Yang, L. (2021). Mitochondrial Dysfunction and Oxidative Stress in Alzheimer’s Disease. Front. Aging Neurosci. 13:617588. doi: 10.3389/fnagi.2021.617588
Mulero, J., Zafrilla, P., and Martinez-Cacha, A. (2011). Oxidative stress, frailty and cognitive decline. J. Nutr. Health Aging 15, 756–760. doi: 10.1007/s12603-011-0130-5
Nadhimi, Y., and Llano, D. A. (2021). Does hearing loss lead to dementia? A review of the literature. Hear. Res. 402:108038. doi: 10.1016/j.heares.2020.108038
Namioka, N., Hanyu, H., Hirose, D., Hatanaka, H., Sato, T., and Shimizu, S. (2017). Oxidative stress and inflammation are associated with physical frailty in patients with Alzheimer’s disease: oxidative stress in frail AD. Geriatr. Gerontol. Int. 17, 913–918. doi: 10.1111/ggi.12804
Nimse, S. B., and Pal, D. (2015). Free radicals, natural antioxidants, and their reaction mechanisms. RSC Adv. 5, 27986–28006. doi: 10.1039/C4RA13315C
Omata, Y., Tharasegaran, S., Lim, Y.-M., Yamasaki, Y., Ishigaki, Y., Tatsuno, T., et al. (2016). Expression of amyloid-β in mouse cochlear hair cells causes an early-onset auditory defect in high-frequency sound perception. Aging 8, 427–439. doi: 10.18632/aging.100899
Panza, F., D’Introno, A., Colacicco, A. M., Capurso, C., Parigi, A. D., Capurso, S. A., et al. (2006). Cognitive frailty: predementia syndrome and vascular risk factors. Neurobiol. Aging 27, 933–940. doi: 10.1016/j.neurobiolaging.2005.05.008
Panza, F., Lozupone, M., Sardone, R., Battista, P., Piccininni, M., Dibello, V., et al. (2019). Sensorial frailty: age-related hearing loss and the risk of cognitive impairment and dementia in later life. Ther. Adv. Chronic Dis. 10:204062231881100. doi: 10.1177/2040622318811000
Panza, F., Solfrizzi, V., and Logroscino, G. (2015a). Age-related hearing impairment—a risk factor and frailty marker for dementia and AD. Nat. Rev. Neurol. 11, 166–175. doi: 10.1038/nrneurol.2015.12
Panza, F., Solfrizzi, V., Seripa, D., Imbimbo, B. P., Capozzo, R., Quaranta, N., et al. (2015b). Age-related hearing impairment and frailty in Alzheimer’s disease: interconnected associations and mechanisms. Front. Aging Neurosci. 7:113. doi: 10.3389/fnagi.2015.00113
Parham, K., Lin, F. R., and Blakley, B. W. (2015). “Age-Related Hearing Loss,” in Geriatric otolaryngology, eds R. T. Sataloff, K. M. Kost, and M. M. Johns (New York: Thieme), 260.
Perry, G., Cash, A. D., and Smith, M. A. (2002). Alzheimer Disease and Oxidative Stress. J. Biomed. Biotechnol. 2, 120–123. doi: 10.1155/S1110724302203010
Persson, T., Popescu, B. O., and Cedazo-Minguez, A. (2014). Oxidative Stress in Alzheimer’s Disease: why Did Antioxidant Therapy Fail? Oxid. Med. Cell. Longev. 2014:427318. doi: 10.1155/2014/427318
Ralli, M., Gilardi, A., Stadio, A. D., Severini, C., Greco, A., de Vincentiis, M., et al. (2019). Hearing loss and Alzheimer’s disease: a Review. Int. Tinnitus J. 23, 79–85. doi: 10.5935/0946-5448.20190014
Robles, L., and Ruggero, M. A. (2001). Mechanics of the mammalian cochlea. Physiol. Rev. 81, 1305–1352.
Seidman, M. D. (2000). Effects of dietary restriction and antioxidants on presbyacusis. Laryngoscope 110, 727–738. doi: 10.1097/00005537-200005000-00003
Shen, Y., Ye, B., Chen, P., Wang, Q., Fan, C., Shu, Y., et al. (2018). Cognitive Decline, Dementia, Alzheimer’s Disease and Presbycusis: examination of the Possible Molecular Mechanism. Front. Neurosci. 12:394. doi: 10.3389/fnins.2018.00394
Slade, K., Plack, C. J., and Nuttall, H. E. (2020). The Effects of Age-Related Hearing Loss on the Brain and Cognitive Function. Trends Neurosci. 43, 810–821. doi: 10.1016/j.tins.2020.07.005
Soysal, P., Isik, A. T., Carvalho, A. F., Fernandes, B. S., Solmi, M., Schofield, P., et al. (2017). Oxidative stress and frailty: a systematic review and synthesis of the best evidence. Maturitas 99, 66–72. doi: 10.1016/j.maturitas.2017.01.006
Tanaka, K., Takemoto, T., Sugahara, K., Okuda, T., Mikuriya, T., Takeno, K., et al. (2005). Post-exposure administration of edaravone attenuates noise-induced hearing loss. Eur. J. Pharmacol. 522, 116–121. doi: 10.1016/j.ejphar.2005.08.026
Tavanai, E., Mohammadkhani, G., Farahani, S., and Jalaie, S. (2019). Protective Effects of Silymarin Against Age-Related Hearing Loss in an Aging Rat Model. Indian J. Otolaryngol. Head Neck Surg. 71, 1248–1257. doi: 10.1007/s12070-018-1294-7
Thomson, R. S., Auduong, P., Miller, A. T., and Gurgel, R. K. (2017). Hearing loss as a risk factor for dementia: a systematic review: hearing Loss and Dementia Systematic Review. Laryngoscope Investig. Otolaryngol. 2, 69–79. doi: 10.1002/lio2.65
Tokgoz, B., Ucar, C., Kocyigit, I., Somdas, M., Unal, A., Vural, A., et al. (2011). Protective effect of N-acetylcysteine from drug-induced ototoxicity in uraemic patients with CAPD peritonitis. Nephrol. Dial. Transplant. 26, 4073–4078. doi: 10.1093/ndt/gfr211
Uchida, Y., Sugiura, S., Nishita, Y., Saji, N., Sone, M., and Ueda, H. (2019). Age-related hearing loss and cognitive decline — The potential mechanisms linking the two. Auris Nasus Larynx 46, 1–9. doi: 10.1016/j.anl.2018.08.010
Uttara, B., Singh, A. V., Zamboni, P., and Mahajan, R. T. (2009). Oxidative stress and neurodegenerative diseases: a review of upstream and downstream antioxidant therapeutic options. Curr. Neuropharmacol. 7, 65–74. doi: 10.2174/157015909787602823
Wang, J., and Puel, J.-L. (2020). Presbycusis: an Update on Cochlear Mechanisms and Therapies. J. Clin. Med. 9:218. doi: 10.3390/jcm9010218
World Health Organization [WHO] (2017a). 10 facts on ageing and health. Geneva: World Health Organization
World Health Organization [WHO] (2017b). WHO clinical consortium on healthy ageing: topic focus: frailty and intrinsic capacity: report of consortium meeting, 1–2 December 2016 in Geneva. Switzerland: World Health Organization.
World Health Organization [WHO] (2021). World report on hearing. Geneva, Switzerland: World Health Organization
Wou, F., and Conroy, S. (2013). The frailty syndrome. Medicine 41, 13–15. doi: 10.1016/j.mpmed.2012.10.004
Xue, Q.-L. (2011). The Frailty Syndrome: definition and Natural History. Clin. Geriatr. Med. 27, 1–15. doi: 10.1016/j.cger.2010.08.009
Yamasoba, T., Lin, F. R., Someya, S., Kashio, A., Sakamoto, T., and Kondo, K. (2013). Current concepts in age-related hearing loss: epidemiology and mechanistic pathways. Hear. Res. 303, 30–38.
Yamasoba, T., Schacht, J., Shoji, F., and Miller, J. M. (1999). Attenuation of cochlear damage from noise trauma by an iron chelator, a free radical scavenger and glial cell line-derived neurotrophic factor in vivo. Brain Res. 815, 317–325.
Zheng, Y., Fan, S., Liao, W., Fang, W., Xiao, S., and Liu, J. (2017). Hearing impairment and risk of Alzheimer’s disease: a meta-analysis of prospective cohort studies. Neurol. Sci. 38, 233–239. doi: 10.1007/s10072-016-2779-3
Keywords: aging, dementia, neurodegenerative, presbycusis, reactive oxygen species
Citation: Alvarado JC, Fuentes-Santamaría V and Juiz JM (2022) Frailty Syndrome and Oxidative Stress as Possible Links Between Age-Related Hearing Loss and Alzheimer’s Disease. Front. Neurosci. 15:816300. doi: 10.3389/fnins.2021.816300
Received: 16 November 2021; Accepted: 29 December 2021;
Published: 18 January 2022.
Edited by:
Irving E. Vega, Michigan State University, United StatesReviewed by:
Dona M. P. Jayakody, Ear Science Institute Australia, AustraliaAhmet Turan Isik, Dokuz Eylül University, Turkey
Copyright © 2022 Alvarado, Fuentes-Santamaría and Juiz. This is an open-access article distributed under the terms of the Creative Commons Attribution License (CC BY). The use, distribution or reproduction in other forums is permitted, provided the original author(s) and the copyright owner(s) are credited and that the original publication in this journal is cited, in accordance with accepted academic practice. No use, distribution or reproduction is permitted which does not comply with these terms.
*Correspondence: Juan Carlos Alvarado, juancarlos.alvarado@uclm.es