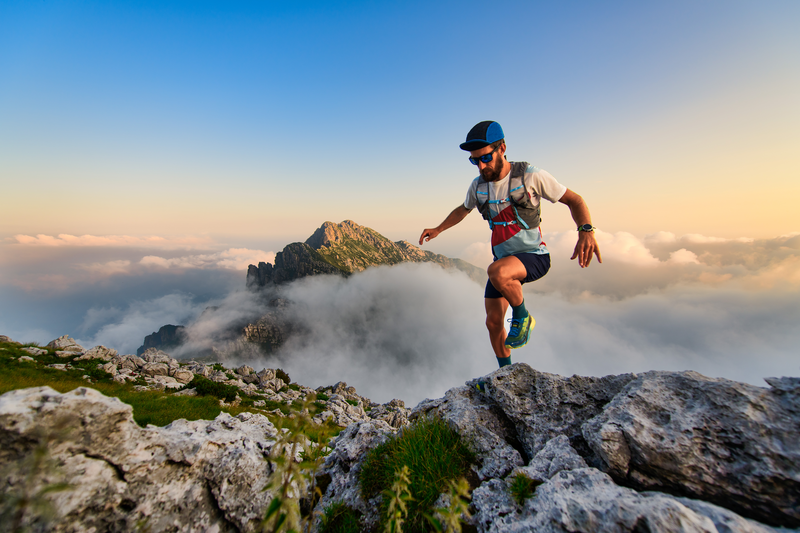
94% of researchers rate our articles as excellent or good
Learn more about the work of our research integrity team to safeguard the quality of each article we publish.
Find out more
METHODS article
Front. Neurosci. , 06 August 2021
Sec. Neurodegeneration
Volume 15 - 2021 | https://doi.org/10.3389/fnins.2021.676579
This article is part of the Research Topic From Mechanisms All the Way up to New Innovations – New Insights into Glaucomatous Neurodegeneration View all 5 articles
Pseudoexfoliation syndrome (PEXS) and glaucoma (PEXG) are assumed to be caused by a generalized elastosis leading to the accumulation of PEX material in ocular as well as in extraocular tissues. The exact pathophysiology of PEXS is still elusive. PEXG, the most common type of secondary open-angle glaucoma (OAG), is characterized by large peaks of intraocular pressure (IOP) with a progressive loss of the visual field. Agonistic autoantibodies (agAAbs) against the β2-adrenergic receptor (AR) have been shown to be present in sera of patients with primary and secondary OAG and ocular hypertension and are seemingly linked to IOP. In the present study, we investigated the autoantibodies directed against the β2-AR in sera of patients with PEXS and PEXG. We recruited 15, 10, and 15 patients with PEXG, PEXS, and primary OAG, respectively. Ten healthy individuals served as controls. All patients underwent standard ophthalmological examination with Octopus G1 perimetry. agAAbs prepared from serum samples were analyzed in a rat cardiomyocyte–based bioassay for the presence of agAAbs. We identified the interacting loop of the β2-AR and the immunoglobulin G (IgG) subclasses using synthetic peptides corresponding to the extracellular loops of the receptors and enzyme-linked immunosorbent assay, respectively. None of the controls were β2-agAAb–positive (0.2 ± 0.5 U). No β2-agAAbs (0.2 ± 0.4 U), but inhibitory β2-AAbs were observed in 80% of the patients that partially blocked the drug-induced β2-adrenergic stimulation; 5.8 ± 1.7 U vs. 11.1 ± 0.9 U for clenbuterol in the absence and the presence of sera from patients with PEXS, respectively. Epitope analyses identified the third extracellular loop of the β2-AR as the target of the inhibitory β2-AAbs, being of IgG3 subtype in PEXS patients. In contrast, patients with PEXG showed β2-agAAbs (5.6 ± 0.9 U), but no inhibitory ones. The β2-agAAbs levels of patients with PEXG and primary OAG patients (3.9 ± 2.8 U; p > 0.05) were at a similar level. In two cases of PEXG, the β2-agAAbs exert synergistic effects with clenbuterol. The activity increased from 11.5 ± 0.3 (clenbuterol only) to 16.3 ± 0.9 U. As autoimmune mechanisms were reportedly involved in the pathogenesis of glaucoma, agonistic and inhibitory β2-AAbs seem to be a part of this multifactorial interplay.
Pseudoexfoliation syndrome (PEXS) is an age-related disorder affecting the extracellular matrix of the whole body (Elhawy et al., 2012; Scharfenberg and Schlotzer-Schrehardt, 2012). It is characterized by the pathological accumulation of extracellular material (i.e., PEX material) in intraocular and extraocular tissues (Ritch and Schlotzer-Schrehardt, 2001; Elhawy et al., 2012). PEX material consists of fibrillar aggregates containing elastic fiber components such as elastin, fibrillin-1, fibulin, microfibril-associated glycoprotein-1 (MAGP-1), latent transforming growth factor β (LTBP)1/2, and cross-linking enzymes such as lysyl oxidase-like 1 (LOXL1) (Schlotzer-Schrehardt et al., 2000; Krumbiegel et al., 2009; Lee et al., 2009; Zenkel and Schlotzer-Schrehardt, 2014; Founti et al., 2015), which can accumulate in the trabecular meshwork, the main outflow pathway of the eye, and may reduce the outflow of aqueous humor and increased intraocular pressure (IOP) (Johnson and Brubaker, 1982). Consequently, PEXS is strongly associated with the development of PEX glaucoma (PEXG) (Schlotzer-Schrehardt et al., 2002; Anastasopoulos et al., 2015). The latter is the most common type of secondary open-angle glaucoma (SOAG) (Ritch, 2001). The pathophysiology of PEXS and PEXG is still elusive (Anastasopoulos et al., 2015; Schlotzer-Schrehardt and Khor, 2021). It is widely assumed that a generalized elastosis, induced by stress, is the principal process in the pathophysiology of PEXS (Schlotzer-Schrehardt et al., 2002; Anastasopoulos et al., 2015; Schlotzer-Schrehardt and Khor, 2021). Transforming growth factor β1 (TGF-β1), being increased in aqueous humor of PEXG and PEXS, and oxidative stress were considered to be the main contributors to the disease (Schlotzer-Schrehardt et al., 2001; Schlotzer-Schrehardt, 2010). PEX is reportedly associated with an increased risk of vascular diseases like myocardial infarction, coronary artery disease, aortic aneurysms, stroke, and cerebrovascular disease (Mitchell et al., 1997; Praveen et al., 2011; Yokusoglu, 2012; Gonen et al., 2013; Andrikopoulos et al., 2014; Siordia et al., 2016). Again the underlying mechanisms are not fully understood (Mitchell et al., 1997; Siordia et al., 2016). As with other glaucomas, the main target in treating PEXG is the increased IOP (Schlotzer-Schrehardt et al., 2002; Anastasopoulos et al., 2015). All conservative, laser, or surgical strategies aim to lower IOP up to an individual target level in order to win sighted lifetime (Lusthaus and Goldberg, 2019; Cvenkel and Kolko, 2020). Reduction of IOP by 1 mm Hg is known to prevent the visual field loss of patients by 10% (Leske et al., 2003) and should be made strictly forward (Musch et al., 2011). A main antiglaucomatous medication is timolol, a blocker of the β2-adrenergic receptor (AR) (Katz et al., 1976; Barnes and Moshirfar, 2021), that reduces the formation of aqueous humor by up to 50% (Coakes and Brubaker, 1978; Yablonski et al., 1978; Dailey et al., 1982; Topper and Brubaker, 1985; Mccannel et al., 1992; Wayman et al., 1997; Larsson, 2001). As β1-AR blockers (e.g., betaxolol) reportedly lower IOP less effectively than β2-AR blockers, the role of the latter in regulating IOP appears to be predominant (Gaul et al., 1989). β2-ARs are expressed on cells of the ciliary body and the trabecular meshwork, the tissues involved in aqueous humor production and outflow (Wax and Molinoff, 1987; Crider and Sharif, 2002). Cells of the optic nerve and retinal pericytes are further tissues that express β2-AR (Mantyh et al., 1995; Feher et al., 2005). agAAbs against β2-AR (β2-agAAbs) have been detected in the sera of patients with primary open-angle glaucoma (POAG) and SOAG, as well as ocular hypertension (OHT) (Junemann et al., 2018; Hohberger et al., 2019a). These agAAbs were mainly of the immunoglobulin G3 (IgG3) subtype and were directed against the second extracellular loop of the β2-AR (peptide 181–192) (Junemann et al., 2018). The immunological targeting of the β2-AR is the basis for the hypothesis of an involvement of β2-agAAbs in dysregulation of IOP (Zimmerman, 1977). The IOP is the result of production (ciliary body) and outflow (trabecular meshwork, uveosclera) of the aqueous humor (Johnstone et al., 2020; Kaufman, 2020; Sunderland and Sapra, 2021). Clinical data of patients with POAG, showing a reduced IOP after removal of all circulating IgG antibodies by immunoadsorption, support this hypothesis (Junemann et al., 2018). These findings indicated a potential role for autoimmune processes in the dysregulation of IOP. As patients with PEXG typically show an increased IOP with large fluctuations (Heijl et al., 2009), we hypothesize a role of the β2-agAAbs in the development of PEXG. Various types of agAAbs, directed against the β2-AR, were described in literature (Wallukat and Wollenberger, 1991; Wallukat, 2002; Junemann et al., 2018): agonistic and inhibitory AAbs activate and block the β2-AR, respectively. It was the aim of this study to analyze serum samples from patients with PEXS and PEXG for agAAbs against β2-AR.
Fifty subjects were recruited from the Department of Ophthalmology, University of Erlangen-Nuremberg: patients [n = 40; PEXG (n = 15), PEX (n = 10), POAG (n = 15)] and controls (n = 10). All patients underwent a complete ophthalmological examination including slit-lamp biomicroscopy, funduscopy, and measurement of IOP by Goldmann applanation tonometry (Haag Streit, Koeniz, Switzerland). In addition, we performed standard white-on-white full-field perimetry (Octopus 500, G1 protocol; Interzeag, Schlieren, Switzerland).
Diagnosis of PEXG was based on an open anterior chamber angle, the presence of PEX material on anterior segment structures, repeated elevated IOP > 21 mm Hg, glaucomatous alterations of the optic disc according to Jonas’ classification (Jonas et al., 1988), and perimetric field loss, confirmed at least once.
Patients with PEXS showed PEX material deposits in the anterior chamber, but without any signs of glaucoma (Scharfenberg and Schlotzer-Schrehardt, 2012; Hohberger et al., 2019b).
Diagnosis of POAG was based on the glaucoma criteria for PEXG, yet without any signs of PEX material on ocular structures (Jonas et al., 1988; Barnes and Moshirfar, 2021).
Tonometry, slit-lamp inspection, funduscopy, and papillometry were normal in control subjects. No signs of PEX or glaucoma were observed, yet the presence of cataract was allowed.
Blood samples were collected according to clinical all-day life procedures and centrifuged, and sera were stored at −80°C previous to analysis. All individuals declared informed consent. The study was performed according to the tenets of the Declaration of Helsinki and approved by the Ethic Committee of the University of Erlangen and the Max-Delbrück-Centrum for Molecular Medicine, Berlin-Buch (Y 9004/19, 02.11.2020).
The cardiomyocyte bioassay was used as described previously (Junemann et al., 2018). Cell culture of cardiac myocytes (heart ventricle of 1–3-day-old Sprague–Dawley rats) was digested with a 0.25% solution of crude porcine trypsin (Serva, Germany). The cells were dispersed and suspended in SM20-I medium (Biochrom, Germany), glutamine (Serva, Germany), which contained streptomycin (HEFA Pharma, Germany), penicillin (Heyl, Germany), hydrocortisone (Merck, Germany), 10% heat-inactivated neonatal calf serum (Gibco, Germany), and fluorodeoxyuridine (Serva, Germany). The cells were seeded with a field density of 160,000 cells/cm2. After 24 h, the culture medium was replaced, respectively. Before stimulation, the cardiomyocytes were cultured for 3–4 days (37°C). Two hours before the experiment, the medium was renewed. After incubation with the immunoglobulin fractions of the sera of each proband (1:40, for 60 min), the beating rates (BRs) of the cardiomyocytes were counted for 7–10 spontaneously beating cells for 15 s at a heated stage inverted microscope (37°C). One unit reflects 4 beats/min (i.e., adrenergic activity). Samples of less than 2 units of adrenergic activity were considered as normal. Differentiation between agonistic and inhibitory β2-AAbs was done after additional incubation with 3 μM clenbuterol; the latter one partially blocked the clenbuterol effect. Application of ICI-118,551 (2 μL ICI to 2 mL medium in order to avoid dilution) was done for identification of the receptor type (β2-receptor).
The IgGs were prepared from sera of patients by direct ammonium sulfate precipitation (final concentration of 40%) overnight at 4°C. Afterward, the precipitates were centrifuged (4,000 × g, 30 min). The pellets were dissolved in dialysis buffer (154 mmol/L NaCl, 10 mmol/L Na2HPO4/NaH2PO4, pH 7.2). Precipitating, washing, and dissolving were repeated twice. The samples were dialyzed against phosphate-buffered saline (4°C, 3 days) prior to application to the cardiomyocyte bioassay. The samples were stored at –20°C.
Characterization of the target loop was done as described previously (Junemann et al., 2018). Synthetic peptides corresponding to the extracellular loops I, II, and III of the human β2-AR were used to identify the molecular domain of the inhibitory β2-AAbs:
1. loop I (HILMKMWTFGNFWCEFWT).
2. loop II (HWYRATHQEAINCYANETCCDFFTNQ).
3. loop III (VIQDNLIRKEV).
After shaking 0.5 μg peptide in 50 μL IgG fraction, they were incubated for 1 h at room temperature before adding them to the bioassay (final IgG dilution of 1:40).
IgG subclass analysis of the inhibitory AAbs was done as described previously (Junemann et al., 2018). IgGs (50 μL) from selected patients were treated with murine monoclonal anti-human IgG1, IgG2, IgG3, and IgG4 antibodies (3 μL, SEROTEC, Germany). After incubation (1 h, room temperature), each sample was treated with 3 μL of a polyclonal anti-mouse Fc antibodies (1 h) in order to increase the immune complex. Centrifugation of the samples (at 10,000 g) was done, and the supernatants were added to the cardiomyocyte bioassay (see above; dilution of 1:40).
Statistical calculations were done with Microsoft Excel 2016 and the GraphPad Prism Software version 8.3. Data are shown as means and standard errors of the mean for each group. The Kolmogorov–Smirnov test was run prior to analysis for testing the normal distribution. According to this, the one-way analysis of variance (normally distributed data) and the Kruskal–Wallis test (non–normally distributed data) with a post-test for multiple comparisons were applied, respectively. When only two groups were compared, the Mann–Whitney test or the Student’s t-test was applied, accordingly. p < 0.05 was considered significant. A list of all abbreviations can be seen in Table 1.
We analyzed the sera of patients with PEXS and PEXG and controls for the presence of agonistic and inhibitory AAbs directed against the β2-AR. None of the control samples showed a β2-agAAb seropositivity (neither agonistic nor inhibitory) of 0.2 ± 0.5 U in the cardiomyocyte bioassay. The cutoff was set at mean ± 3 standard deviation of the controls (1.66 U).
None of the 10 patients with PEXS showed β2-agAAbs (mean = 0.2 ± 0.1 U) (Figure 1). Clenbuterol alone increased the BR value of the cardiomyocytes by 11.1 ± 0.5 U. After coincubation of the IgG with 3 μM clenbuterol (mean), a decreased BR value was observed in 8 (80%) of 10 PEXS patients (5.8 ± 0.6 U, p < 0.001). This decrease was attributed to the inhibitory β2-AAbs that partially blocked the clenbuterol effect (p < 0.001; Figure 2).
Figure 1. Adrenergic activity of β2-AAbs in sera of patients with PEX syndrome (PEXS), PEX glaucoma (PEXG), and controls. Beating heart cell rates were shown for each group [absolute data, mean ± standard deviation (SD)]: controls, patients with PEXG and PEXS. One unit (U) reflects 4 beats/min. The values represent the adrenergic activity of β2-Abs. Samples less than 2 units of adrenergic activity were considered as normal. Increased beating heart cell rate was to be observed for patients with PEXG (red) when compared to patients with PEXS and controls as measured by rat cardiomyocyte beating heart cell rates.
Figure 2. β2-AAbs in patients with PEXS are of inhibitory nature. Beating heart cell rates after single application of sera of patients with PEXS without (green) and with additional clenbuterol (CB, pink); reference: single incubation with CB (blue); 1 unit (U) reflects 4 beats/min; mean ± standard deviation (SD). β2-AAbs inhibit the increased beating heart cell rate, due to the effect of clenbuterol, and are thus of inhibitory nature. *p < 0.001, with a post-test for multiple comparisons.
Clenbuterol yielded an increase in the BR (mean = 12.4 ± 0.2 U). We performed the epitope analyses employing specific competitive inhibitory peptides of the second and third extracellular loops of the β2-AR (Figure 3). Loop III but not loop II peptides neutralized the inhibitory β2-AAbs. These data indicate that inhibitory β2-AAbs in the sera of patients with PEXS are directed against the third extracellular loop of the β2-AR.
Figure 3. Epitope mapping of the inhibitory anti–β2-AAb in patients with PEX syndrome revealed that inhibitory anti–β2-AAb targets extracellular loop III. Peptides of the β2-adrenergic receptor extracellular loops II and III were used for epitope mapping of the inhibitory anti–β2-AAb in patients with PEX syndrome in the presence of clenbuterol (Cb): peptides against the third loop did block the binding site of the inhibitory anti–β2-AAb. Consequently, additional application of Cb did show an increase in the beating rate (orange); peptides against the second loop did not block the binding site of the inhibitory anti–β2-AAb. Consequently, the inhibitory nature of the inhibitory anti–β2-AAb reduced significantly the Cb effect (∗p = 0.001, green); application of clenbuterol alone (control, blue); 1 unit (U) reflects 4 beats/min; mean ± standard deviation (SD).
The analyses of the IgG subtype were performed by immune precipitation with antibodies against the immunoglobulins IgG1–4. The residual inhibitory β2-AAb activities after preincubation with IgG subclass–specific antibodies were 4.3 ± 0.3 U (IgG1), 4.1 ± 0.2 U (IgG2), 11.7 ± 0.4 U (IgG3), and 4.0 ± 0.3 U (IgG4), respectively. The activity of the inhibitory β2-AAbs was eliminated by preincubation with anti-IgG3 antibodies. Thus, the inhibitory β2-AAbs are of the IgG3 subclass (Figure 4).
Figure 4. Identification of the IgG3 subclass of the inhibitory anti–β2-AAbs in patients with PEX syndrome (PEXS). IgG subclass analysis was done by IgG, prepared from sera of patients with PEXS by direct ammonium sulfate precipitation. The samples were treated with murine monoclonal anti-human IgG1, IgG2, IgG3, and IgG4 antibodies prior to application to the cardiomyocyte bioassay. Beating heart cell rates after immune precipitation of IgG1 (red), IgG2 (dark orange), IgG3 (green), and IgG4 (blue) in the presence of additional application of clenbuterol (Cb); absolute date, mean ± standard deviation (SD); 1 unit (U) reflects 4 beats/min. Only immune precipitation of IgG3 removed the inhibitory effect of inhibitory anti–β2-AAbs on the Cb effect. Consequently, the inhibitory anti–β2-AAbs are of IgG3 subtype.
In contrast to patients with PEXS and controls, 12 (80%) of 15 of those with PEXG had β2-agAAbs. The mean was 4.55 ± 0.6 U in all the PEXG patients (Figure 5). The BR between patients with PEXG and those with POAG did not differ (3.9 ± 0.7 U; p > 0.05). In contrast, no inhibitory β2-AAbs were to be observed. Figure 6 shows that 2 (13.3%) of the 15 of the patients with PEXG showed synergistic β2-AAbs that markedly increased BR values after coincubation with 1 μM clenbuterol (16.3 ± 0.6 U vs. 11.5 ± 0.1 U for clenbuterol alone). Only 1 (6.7%) of 15 PEXG patients showed neither agonistic nor inhibitory β2-AAbs.
Figure 5. Agonistic β2-agAAbs in sera of open-angle glaucoma. (A) Beating heart cell rates after incubation with sera of patients with primary open-angle glaucoma (POAG, red) and PEX glaucoma (PEXG, orange); mean ± standard deviation (SD); 1 unit (U) reflects 4 beats/min. An increase of the beating heart cell rate was observed in POAG and PEXG, showing its agonistic effect. Beating heart cell rate of POAG and PEXG did not differ significantly (p > 0.05). (B) Beating heart cell rates after application of sera without (green) and with ICI-118,551 (black): the agonistic effect of the β2-AAbs is shown. The agonistic nature of β2-agAAbs activated the receptor with consequent increase of the beating heart cell rate. These agonistic β2-agAAbs were observed in sera of patients with open-angle glaucoma.
Figure 6. Synergistic β2-AAbs enhanced the effect of Cb alone and enhanced the agonistic effect of β2-agAAbs. Beating cell rates after incubation of live rat cardiomyocyte cells with patient sera from PEX glaucoma (PEXG): sera without (orange) and with the β2-blocker ICI-118,551 (gray–green); synergistic β2-AAbs (red) after coincubation of the beating heart cells with clenbuterol (Cb); reference: beating heart cell rate after incubation with clenbuterol alone (blue) and of control subjects (green). One unit (U) reflects 4 beats/min. Sera of PEX glaucoma showed synergistic and agonistic β2-AAbs: agonistic β2-AAbs were present as beating heart cell rates were increased compared to controls, reaching Cb effect alone. Synergistic β2-AAbs enhanced the effect of Cb alone and enhanced the agonistic effect of β2-agAAbs. ICI blocked the effect of the β2-adrenergic receptor AAbs demonstrating the specificity for the β2-adrenergic receptor on the beating heart cells.
Analysis of IgG subclasses showed that the β2-agAAbs were of the IgG3 subclass (Figure 7), as only precipitation with IgG3 antibodies eliminated the increase in the BR value (0.29 ± 0.1 U). Consistently, no alterations of the BR were seen after precipitation of IgG1 (5.0 ± 0.3 U), IgG2 (5.0 ± 0.5 U), and IgG4 (5.1 ± 0.5 U). Epitope analyses of β2-AR showed that the β2-agAAbs were directed against the second extracellular loop of this G-protein–coupled receptor (in full) as described previously (Junemann et al., 2018).
Figure 7. Identification of IgG3 subclass of β2-agAAbs from patients with PEX glaucoma. Beating heart cell rates after incubation pretreated with anti-human IgG1 (red), IgG2 (green), IgG3 (dark orange), and IgG4 (blue); absolute date, mean ± standard deviation (SD); 1 unit (U) reflects 4 beats/min. The increase in the beating heart cell rate was eliminated only after incubation with anti-human IgG3 (dark orange). Only peptides against IgG3 inhibited the agonistic nature of β2-agAAbs, visualized by the increase of the beating heart cell rate. Consequently, the agonistic β2-agAAbs are of the IgG3 subtype.
PEXS and PEXG are age-related ocular and systemic diseases associated with the accumulation of abnormal fibrillar material in intraocular and extraocular tissues (Elhawy et al., 2012; Scharfenberg and Schlotzer-Schrehardt, 2012; Schlotzer-Schrehardt and Khor, 2020). With an annual incidence of 1.81%, PEXS is a common condition (Astrom et al., 2007), yet its prevalence varies worldwide (Cashwell and Shields, 1988; Krishnadas et al., 2003; Topouzis et al., 2019). It accounts for 25% and up to 70% of all open-angle glaucoma (OAG), depending on the country (Ritch, 1994; Mitchell et al., 1999; Andrikopoulos et al., 2009). Although PEXS is a major risk factor for glaucoma, it is important to note that only 30–40 of all individuals with PEXS will develop PEXG (Thorleifsson et al., 2007). In longitudinal population-based studies, the probability to develop glaucoma after 10–12 years was found to be 9–15% (Jeng et al., 2007; Topouzis et al., 2019). PEXG is a hypertensive type of glaucoma associated with high levels and fluctuations of the IOP, as well as an increased outflow resistance (Kozobolis et al., 2012; Huchzermeyer et al., 2015). Eyes of patients with PEXS tend to have higher IOP levels as healthy eyes but can be normotensive as well (Koz et al., 2009). Although PEXS is an established risk factor for PEXG (Mitchell et al., 1999; Topouzis et al., 2011), the exact relationship between the two conditions is not well understood (Topouzis et al., 2019). Similarly, it remains unclear why only a proportion of PEXS patients develop glaucoma, whereas the majority of them will not (Anastasopoulos et al., 2015; Schlotzer-Schrehardt and Khor, 2021). In a longitudinal population-based study, statistically significant risk factors for the development of PEXG among PEXS patients included higher levels of IOP, with 26% increased risk per mm Hg, and a history of heart attack (Topouzis et al., 2019). It is also conceivable that an underlying defect in the flow dynamics of the aqueous humor or additional involvement of glaucoma susceptibility genes may predispose to glaucoma development in PEXS eyes (Schlotzer-Schrehardt and Naumann, 1995; Kozobolis et al., 2012; Founti et al., 2015; Huchzermeyer et al., 2015; Schlotzer-Schrehardt and Khor, 2021). It has been suggested that in addition to IOP, further factors contribute to the development of glaucoma in patients with PEXS. These include genetic, epigenetic, environmental, and tissue factors such as increased susceptibility of the lamina cribrosa to optic nerve damage (Topouzis et al., 2011; Braunsmann et al., 2012; Hohberger et al., 2018; Schlotzer-Schrehardt and Khor, 2021). Autoimmune mechanisms have also been suggested to participate. Interestingly, there was no difference between the antibody patterns in the aqueous humor of patients with POAG and PEXG. This indicates common pathomechanisms in dysregulation of aqueous humor dynamics and IOP in these glaucoma subtypes (Joachim et al., 2007). Previously, we have identified specific agAAbs affecting the β2-AR in sera of patients with PEXG, as has been also shown for patients with POAG (Hohberger et al., 2019a). A subsequent study showed that β2-agAAbs were also present in aqueous humor samples of patients with POAG or PEXG (Hohberger et al., 2020). It has been hypothesized that via an impaired blood-aqueous barrier in PEXS/PEXG, serum proteins including β2-agAAbs enter the anterior chamber, as observed in a previous study (Schlotzer-Schrehardt and Naumann, 2006). Alternatively, the β2-agAAbs may be produced locally in ocular tissues. As the β2-agAAbs already occur in very early stages of glaucoma and OHT [e.g., patients without optic disc alterations, yet increased IOP (OHT)] (Junemann et al., 2018), we were interested to find out if patients with risk of PEXG (e.g., PEXS) harbor β2-agAAbs. The findings of the present study showed that patients with PEXS lacked any agAAbs. Instead, 80% contained inhibitory β2-AAbs of the IgG3 subtype, which is directed against the third extracellular loop of the β2-AR. In contrast, patients with PEXG had β2-agAAbs, yet no inhibitory ones. Interestingly, synergistic β2-agAAbs were to be observed in the sera of two patients with PEXG, overtopping the effect of the β2-adrenergic agonist clenbuterol. The presence of synergistic β2-AAbs had never been published before. The data of the present study suggest that inhibitory AAbs present in the majority of PEXS patients may contribute to maintenance of normal IOP by reducing aqueous humor production and counterbalance the age-related decline in outflow facility. In addition, we hypothesized that agAAbs may contribute to an increase in IOP by stimulating aqueous humor production in the presence of increased outflow resistance due to abnormal deposits in the trabecular meshwork. This scenario potentially contributes to the etiopathogenesis of glaucoma.
Immune and autoimmune factors were recently reported to be involved in the pathogenesis of OAG (Tezel et al., 2007; Tezel and Fourth ARVO/Pfizer Ophthalmics Research Institute Conference Working Group, 2009; Joachim et al., 2013; Rieck, 2013; Kamat et al., 2016; Von Thun Und Hohenstein-Blaul et al., 2016; Chen et al., 2018; Hohberger et al., 2019a; Tsai et al., 2019). A spectrum of various autoantibodies, mostly directed against retinal antigens, has previously been detected in the sera of patients with PEXS and PEXG (Dervan et al., 2010; Altintas et al., 2012; Huflejt et al., 2014; Mazeikaite et al., 2016) and in the aqueous humor of patients with PEXG (Joachim et al., 2007). In the latter study, there were no significant differences in these antibody patterns between PEXG and POAG. β2-AgAAbs levels of PEXG and POAG were similar as well. The present study showed for the first time inhibitory β2-AAbs in the sera of patients with PEXS. Inhibitory β2-AAbs block the function of the β2-AR. This finding is supported by the clinical feature of a less pronounced mydriasis after application of 10% phenylephrine in PEXS eyes compared to eyes without PEXS (Puska, 2002). As reported in a previous study, the local level of β2-agAAbs in the aqueous humor was increased compared to the systemic levels (Hohberger et al., 2020). Thus, we propose that adrenergic β2-AAbs can be locally produced in ocular tissues. The adrenergic agAAbs in aqueous humor are the basis of our hypothesis of inhibitory β2-AAbs in PEXS: due to blocking of β2-AR by inhibitory β2-AAbs, endogenous catecholamine will shift to alternative AR. Consecutively, AR receptors will be uncoupled (acute response), phosphorylated, and internalized (desensitization of AR) (see Wallukat, 2002). Application of phenylephrine induces mydriasis by contraction of the iris dilatator in unaffected eyes. This mydriatic effect might be reduced in cells of the iris dilatator with desensitized AR.
The exact etiopathogeneses of PEXS and PEXG are still under debate. Two main questions remain unanswered: what is the pathomechanism behind the unilateral clinical manifestation of PEXS/PEXG? And why does only a subgroup of the eyes with PEXS convert to PEXG? It is assumed that the basis of PEXS is a genetic predisposition (e.g., LOXL1). In order to present the clinical features with PEX material on ocular structures, several endogenous and/or exogenous factors are engaged in this complex interplay. Inhibitory, agonistic, and even synergistic β2-AAbs turned out to be a common feature in PEXS/PEXG patients in samples of the present cohort (80%); thus, we propose that this form of autoimmunity contributes to the clinical characteristics of PEXS/PEXG. Patients with PEXS show inflammatory markers in their sera (Gonzalez-Iglesias et al., 2014) and aqueous humors [e.g., interleukin (IL-6), IL-8] (Koliakos et al., 2001; Zenkel et al., 2010; Borras, 2018), arguing for an underlying subclinical inflammation. According to the findings of the present study, we propose that because of this inflammatory status, autoimmune mechanisms occur with the generation of autoantibodies, being inhibitory (PEXS), agonistic, or synergistic (PEXG). The specific adrenergic agAAbs might further increase the variation of each clinical entity. Inhibition or overstimulation of the AR might result in a dysregulation of the homeostatic adrenergic balance. This non-canonical receptor–immune complex interaction inhibits or overactivates the adenylate cyclase, causes receptor internalization (due to overstimulation by β2-agAAbs), or even enhances AR expression in the sarcolemma membrane (e.g., due to inhibition by inhibitory β2-AAbs) (Wallukat et al., 2002). If this molecular dysbalance has sufficient “power,” clinical features might occur in patients with PEXS/PEXG (imbalanced autonomic theory; see Szentiványi, 1968).
Inhibitory β2-AAbs were observed in sera of patients with allergic bronchial asthma for the first time (Wallukat and Wollenberger, 1991). The present study showed inhibitory β2-AAbs in patients with PEXS as second clinical entity for the first time. Inhibitory β2-AAbs bind to β2-AR in a competitive way (Wallukat and Wollenberger, 1991). Thus, their binding site to the third loop seems to be different from the β2-agAAbs that bound to the first or second extracellular loop of the receptors. After binding, the AR function will be blocked, reducing the activity of adenylate cyclase with consecutive enzymatic dysbalance (Wallukat, 2002; Johnson, 2006). On the contrary, agonistic and even synergistic β2-AAbs were present in patients with PEXG. As described in previous studies, we propose that β2-agAAbs contribute to the increased IOP in patients with OAG (Junemann et al., 2018; Hohberger et al., 2019a). The presence of synergistic β2-AAbs in sera of patients with PEXG argues for the distinct IOP pattern in these eyes. IOP in PEXG is elevated as in all OAG eyes, yet with strongly enhanced diurnal fluctuations and worsened disease progression compared to POAG. This theory might be parallel to the “β-adrenergic theory of the atopic abnormality in bronchial asthma” (Szentiványi, 1968). Szentiványi (1968) hypothesized that decreased β-adrenergic sensitivity results in an autonomic dysbalance, featuring the typical clinical characteristics of asthma. Szentivanyi postulated that “its ubiquitous character suggests, therefore, that the ultimate clinical manifestation of the fundamentally same atopic abnormality will be determined by the type of cell primarily involved, that is, by the specialized cell system of the multicellular organism which harbors primarily the postulated enzymatic abnormality.” It would be of interest, if these autoimmune phenomena will change during disease progression: if either one agAAb subtype will disappear and another one appears, or the presence of a specific adrenergic agAAb subtype would potentially influence the clinical characteristics: PEXS (inhibitory β2-AAbs) or entities with increased IOP [OAG including PEXG and POAG (Hohberger et al., 2019a, 2020) and OHT (Junemann et al., 2018); β2-agAAbs, Table 2]. A longitudinal follow-up study would be of interest to focus on the pattern of adrenergic AAbs in progressive glaucoma patients. This offers the potential function of β2-AAbs as novel biomarkers, supporting diagnosis and monitoring of PEXS, PEXG, and POAG. It potentially provides novel therapeutic targets for one of the most serious types of glaucoma (PEXG), as well as the most common glaucoma form (POAG). The data of the present study already enable the use of adrenergic autoantibodies as novel biomarker, differentiating between PEXS and PEXG. Analysis of a potential presence of adrenergic AAbs locally in aqueous humor of PEXS and PEXG would give additional novel hints insight toward locus of production and pathogenesis.
Table 2. Autoantibodies targeting the adrenergic β2-AR in glaucoma suspects and patients with glaucoma: pseudoexfoliation syndrome (PEXS), pseudoexfoliation glaucoma (PEXG), ocular hypertension (OHT), primary open-angle glaucoma (POAG), secondary open-angle glaucoma (SOAG), and pigment dispersion (PD).
The presence of inhibitory, agonistic, and even synergistic β2-AAbs in sera of PEXS and PEXG, respectively, argues for an imbalanced adrenergic control of the IOP (imbalanced autonomic theory). Each adrenergic agAAb subtype might contribute to the typical clinical characteristics (PEXS, PEXG). Whereas patients with PEXS showed inhibitory β2-AAbs, none of these AAbs were to be observed in the sera of PEXG, yielding agonistic and synergistic β2-AAbs. These observations argue for an involvement of the β2-AAbs early in the pathogenesis of glaucoma. In addition, β2-AAbs might offer a novel option as biomarker or therapeutic target in glaucoma.
The original contributions presented in the study are included in the article/supplementary material, further inquiries can be directed to the corresponding author/s.
The studies involving human participants were reviewed and approved by the Ethics Committee of the University of Erlangen-Nuremberg. The patients/participants provided their written informed consent to participate in this study.
US-S, RK, MH, and GW conceived the idea. GW performed the experimental work. BH wrote the draft of the manuscript. CM interpreted the results and revised the manuscript. RL revised the manuscript. BH, MH, and LM performed statistical analysis. All authors contributed to the article and approved the submitted version.
RK as the patent EP1832600A1. MH has the patent EP1832600A1. GW was employed by Berlin Cures GmbH, Berlin, Germany and holds the patent P1832600A1.
The remaining authors declare that the research was conducted in the absence of any commercial or financial relationships that could be construed as a potential conflict of interest.
All claims expressed in this article are solely those of the authors and do not necessarily represent those of their affiliated organizations, or those of the publisher, the editors and the reviewers. Any product that may be evaluated in this article, or claim that may be made by its manufacturer, is not guaranteed or endorsed by the publisher.
Altintas, O., Yuksel, N., Sonmez, G. T., Ozkan, B., Altintas, L., Caliskan, S., et al. (2012). Serum antiphospholipid antibody levels in pseudoexfoliation. J. Glaucoma 21, 326–330. doi: 10.1097/ijg.0b013e31821206cd
Anastasopoulos, E., Founti, P., and Topouzis, F. (2015). Update on pseudoexfoliation syndrome pathogenesis and associations with intraocular pressure, glaucoma and systemic diseases. Curr. Opin. Ophthalmol. 26, 82–89. doi: 10.1097/icu.0000000000000132
Andrikopoulos, G. K., Alexopoulos, D. K., and Gartaganis, S. P. (2014). Pseudoexfoliation syndrome and cardiovascular diseases. World J. Cardiol. 6, 847–854. doi: 10.4330/wjc.v6.i8.847
Andrikopoulos, G. K., Mela, E. K., Georgakopoulos, C. D., Papadopoulos, G. E., Damelou, A. N., Alexopoulos, D. K., et al. (2009). Pseudoexfoliation syndrome prevalence in Greek patients with cataract and its association to glaucoma and coronary artery disease. Eye 23, 442–447. doi: 10.1038/sj.eye.6702992
Astrom, S., Stenlund, H., and Linden, C. (2007). Incidence and prevalence of pseudoexfoliations and open-angle glaucoma in northern Sweden: II. Results after 21 years of follow-up. Acta Ophthalmol. Scand. 85, 832–837. doi: 10.1111/j.1600-0420.2007.00980.x
Borras, T. (2018). Growth factors, oxidative damage, and inflammation in exfoliation syndrome. J. Glaucoma 27(Suppl.1), S54–S60.
Braunsmann, C., Hammer, C. M., Rheinlaender, J., Kruse, F. E., Schaffer, T. E., and Schlotzer-Schrehardt, U. (2012). Evaluation of lamina cribrosa and peripapillary sclera stiffness in pseudoexfoliation and normal eyes by atomic force microscopy. Invest. Ophthalmol. Vis. Sci. 53, 2960–2967. doi: 10.1167/iovs.11-8409
Cashwell, L. F. Jr., and Shields, M. B. (1988). Exfoliation syndrome. Prevalence in a southeastern United States population. Arch. Ophthalmol. 106, 335–336. doi: 10.1001/archopht.1988.01060130361021
Chen, H., Cho, K. S., Vu, T. H. K., Shen, C. H., Kaur, M., Chen, G., et al. (2018). Commensal microflora-induced T cell responses mediate progressive neurodegeneration in glaucoma. Nat. Commun. 9:3209.
Coakes, R. L., and Brubaker, R. F. (1978). The mechanism of timolol in lowering intraocular pressure. In the normal eye. Arch. Ophthalmol. 96, 2045–2048. doi: 10.1001/archopht.1978.03910060433007
Crider, J. Y., and Sharif, N. A. (2002). Adenylyl cyclase activity mediated by beta-adrenoceptors in immortalized human trabecular meshwork and non-pigmented ciliary epithelial cells. J. Ocul. Pharmacol. Ther. 18, 221–230. doi: 10.1089/108076802760116142
Cvenkel, B., and Kolko, M. (2020). Current medical therapy and future trends in the management of glaucoma treatment. J. Ophthalmol. 2020:6138132.
Dailey, R. A., Brubaker, R. F., and Bourne, W. M. (1982). The effects of timolol maleate and acetazolamide on the rate of aqueous formation in normal human subjects. Am. J. Ophthalmol. 93, 232–237. doi: 10.1016/0002-9394(82)90419-6
Dervan, E. W., Chen, H., Ho, S. L., Brummel, N., Schmid, J., Toomey, D., et al. (2010). Protein macroarray profiling of serum autoantibodies in pseudoexfoliation glaucoma. Invest. Ophthalmol. Vis. Sci. 51, 2968–2975. doi: 10.1167/iovs.09-4898
Elhawy, E., Kamthan, G., Dong, C. Q., and Danias, J. (2012). Pseudoexfoliation syndrome, a systemic disorder with ocular manifestations. Hum. Genom. 6:22. doi: 10.1186/1479-7364-6-22
Feher, L. Z., Kalman, J., Puskas, L. G., Gyulveszi, G., Kitajka, K., Penke, B., et al. (2005). Impact of haloperidol and risperidone on gene expression profile in the rat cortex. Neurochem. Int. 47, 271–280. doi: 10.1016/j.neuint.2005.04.020
Founti, P., Haidich, A. B., Chatzikyriakidou, A., Salonikiou, A., Anastasopoulos, E., Pappas, T., et al. (2015). Ethnicity-based differences in the association of loxl1 polymorphisms with pseudoexfoliation/pseudoexfoliative glaucoma: a meta-analysis. Ann. Hum. Genet. 79, 431–450. doi: 10.1111/ahg.12128
Gaul, G. R., Will, N. J., and Brubaker, R. F. (1989). Comparison of a noncardioselective beta-adrenoceptor blocker and a cardioselective blocker in reducing aqueous flow in humans. Arch. Ophthalmol. 107, 1308–1311. doi: 10.1001/archopht.1989.01070020378039
Gonen, K. A., Gonen, T., and Gumus, B. (2013). Renal artery stenosis and abdominal aorta aneurysm in patients with pseudoexfoliation syndrome. Eye (Lond) 27, 735–741. doi: 10.1038/eye.2013.56
Gonzalez-Iglesias, H., Alvarez, L., Garcia, M., Escribano, J., Rodriguez-Calvo, P. P., Fernandez-Vega, L., et al. (2014). Comparative proteomic study in serum of patients with primary open-angle glaucoma and pseudoexfoliation glaucoma. J. Proteomics 98, 65–78. doi: 10.1016/j.jprot.2013.12.006
Heijl, A., Bengtsson, B., Hyman, L., and Leske, M. C., Early Manifest Glaucoma Trial G (2009). Natural history of open-angle glaucoma. Ophthalmology 116, 2271–2276. doi: 10.1016/j.ophtha.2009.06.042
Hohberger, B., Chaudhri, M. A., Michalke, B., Lucio, M., Nowomiejska, K., Schlotzer-Schrehardt, U., et al. (2018). Levels of aqueous humor trace elements in patients with open-angle glaucoma. J. Trace Elem. Med. Biol. 45, 150–155. doi: 10.1016/j.jtemb.2017.10.003
Hohberger, B., Kunze, R., Wallukat, G., Kara, K., Mardin, C. Y., Lammer, R., et al. (2019a). Autoantibodies activating the beta2-adrenergic receptor characterize patients with primary and secondary glaucoma. Front. Immunol. 10:2112.
Hohberger, B., Monczak, E., and Mardin, C. Y. (2019b). [26 years of the erlangen glaucoma registry: demographic and perimetric characteristics of patients through the ages]. Klin. Monbl. Augenheilkd 236, 691–698.
Hohberger, B., Worn, M., Lammer, R., Herrmann, M., Mardin, C. Y., Kruse, F. E., et al. (2020). Agonistic beta 2-adrenergic receptor autoantibodies characterize the aqueous humor of patients with primary and secondary open-angle glaucoma. Invest. Ophthalmol. Vis. Sci. 61:2112.
Huchzermeyer, C., Horn, F., Lammer, R., Mardin, C. Y., and Junemann, A. G. (2015). Short-term fluctuation of intraocular pressure is higher in patients with pseudoexfoliation syndrome despite similar mean intraocular pressure: a retrospective case-control study. Graefes Arch. Clin. Exp. Ophthalmol. 253, 107–114. doi: 10.1007/s00417-014-2821-1
Huflejt, M. E., Preiss, J. S., Thomson, J. E., Gils, I. M., and Vuskovic, M. I. (2014). Glycomics, extracellular matrix, and anti-glycan antibodies in exfoliation syndrome. J. Glaucoma 23, S24–S29.
Jeng, S. M., Karger, R. A., Hodge, D. O., Burke, J. P., Johnson, D. H., and Good, M. S. (2007). The risk of glaucoma in pseudoexfoliation syndrome. J. Glaucoma 16, 117–121. doi: 10.1097/01.ijg.0000243470.13343.8b
Joachim, S. C., Reinehr, S., Kuehn, S., Laspas, P., Gramlich, O. W., Kuehn, M., et al. (2013). Immune response against ocular tissues after immunization with optic nerve antigens in a model of autoimmune glaucoma. Mol. Vis. 19, 1804–1814.
Joachim, S. C., Wuenschig, D., Pfeiffer, N., and Grus, F. H. (2007). IgG antibody patterns in aqueous humor of patients with primary open angle glaucoma and pseudoexfoliation glaucoma. Mol. Vis. 13, 1573–1579.
Johnson, D. H., and Brubaker, R. F. (1982). Dynamics of aqueous humor in the syndrome of exfoliation with glaucoma. Am. J. Ophthalmol. 93, 629–634.
Johnson, M. (2006). Molecular mechanisms of beta(2)-adrenergic receptor function, response, and regulation. J. Allergy Clin. Immunol. 117, 18–24. doi: 10.1016/j.jaci.2005.11.012
Johnstone, M., Xin, C., Tan, J., Martin, E., Wen, J., and Wang, R. K. (2020). Aqueous outflow regulation – 21st century concepts. Prog Retin Eye Res. 83:100917. doi: 10.1016/j.preteyeres.2020.100917
Jonas, J. B., Gusek, G. C., and Naumann, G. O. (1988). Optic disc morphometry in chronic primary open-angle glaucoma. I. Morphometric intrapapillary characteristics. Graefes Arch. Clin. Exp. Ophthalmol. 226, 522–530. doi: 10.1007/bf02169199
Junemann, A., Hohberger, B., Rech, J., Sheriff, A., Fu, Q., Schlotzer-Schrehardt, U., et al. (2018). Agonistic autoantibodies to the beta2-adrenergic receptor involved in the pathogenesis of open-angle glaucoma. Front. Immunol. 9:145. doi: 10.3389/fimmu.2018.00145
Kamat, S. S., Gregory, M. S., and Pasquale, L. R. (2016). The role of the immune system in glaucoma: bridging the divide between immune mechanisms in experimental glaucoma and the human disease. Semin. Ophthalmol. 31, 147–154. doi: 10.3109/08820538.2015.1114858
Katz, I. M., Hubbard, W. A., Getson, A. J., and Gould, A. L. (1976). Intraocular pressure decrease in normal volunteers following timolol ophthalmic solution. Invest Ophthalmol 15, 489–492.
Kaufman, P. L. (2020). Deconstructing aqueous humor outflow – The last 50 years. Exp. Eye Res. 197:108105. doi: 10.1016/j.exer.2020.108105
Koliakos, G. G., Schlotzer-Schrehardt, U., Konstas, A. G., Bufidis, T., Georgiadis, N., and Dimitriadou, A. (2001). Transforming and insulin-like growth factors in the aqueous humour of patients with exfoliation syndrome. Graefes Arch. Clin. Exp. Ophthalmol. 239, 482–487. doi: 10.1007/s004170100287
Koz, O. G., Turkcu, M. F., Yarangumeli, A., Koz, C., and Kural, G. (2009). Normotensive glaucoma and risk factors in normotensive eyes with pseudoexfoliation syndrome. J. Glaucoma 18, 684–688. doi: 10.1097/ijg.0b013e31819c4311
Kozobolis, V. P., Paschalis, E. I., Labiris, G., Foudoulakis, N. C., Konstantinidis, A., and Koukoula, S. C. (2012). Tonography assessment using quantitative and qualitative analysis of the aqueous humor outflow mechanism. Eur. J. Ophthalmol. 22, 726–733. doi: 10.5301/ejo.5000107
Krishnadas, R., Nirmalan, P. K., Ramakrishnan, R., Thulasiraj, R. D., Katz, J., Tielsch, J. M., et al. (2003). Pseudoexfoliation in a rural population of southern India: the Aravind Comprehensive Eye Survey. Am. J. Ophthalmol. 135, 830–837. doi: 10.1016/s0002-9394(02)02271-7
Krumbiegel, M., Pasutto, F., Mardin, C. Y., Weisschuh, N., Paoli, D., Gramer, E., et al. (2009). Exploring functional candidate genes for genetic association in german patients with pseudoexfoliation syndrome and pseudoexfoliation glaucoma. Invest. Ophthalmol. Vis. Sci. 50, 2796–2801. doi: 10.1167/iovs.08-2339
Larsson, L. I. (2001). Aqueous humor flow in normal human eyes treated with brimonidine and timolol, alone and in combination. Arch. Ophthalmol. 119, 492–495. doi: 10.1001/archopht.119.4.492
Lee, K. Y., Ho, S. L., Thalamuthu, A., Venkatraman, A., Venkataraman, D., Pek, D. C., et al. (2009). Association of LOXL1 polymorphisms with pseudoexfoliation in the Chinese. Mol. Vis. 15, 1120–1126.
Leske, M. C., Heijl, A., Hussein, M., Bengtsson, B., Hyman, L., Komaroff, E., et al. (2003). Factors for glaucoma progression and the effect of treatment: the early manifest glaucoma trial. Arch. Ophthalmol. 121, 48–56. doi: 10.1001/archopht.121.1.48
Mantyh, P. W., Rogers, S. D., Allen, C. J., Catton, M. D., Ghilardi, J. R., Levin, L. A., et al. (1995). Beta 2-adrenergic receptors are expressed by glia in vivo in the normal and injured central nervous system in the rat, rabbit, and human. J. Neurosci. 15, 152–164. doi: 10.1523/jneurosci.15-01-00152.1995
Mazeikaite, G., Kuzmiene, L., Daveckaite, A., Siaudvytyte, L., Nedzelskiene, I., Siesky, B., et al. (2016). Serum antiphospholipid antibody level and ocular haemodynamics in eyes with pseudoexfoliation syndrome. Acta Ophthalmol. 94, e165–e166.
Mccannel, C. A., Heinrich, S. R., and Brubaker, R. F. (1992). Acetazolamide but not timolol lowers aqueous-humor flow in sleeping humans. Graefes Arch. Clin. Exp. Ophthalmol. 230, 518–520. doi: 10.1007/bf00181771
Mitchell, P., Wang, J. J., and Hourihan, F. (1999). The relationship between glaucoma and pseudoexfoliation: the Blue Mountains Eye Study. Arch. Ophthalmol. 117, 1319–1324. doi: 10.1001/archopht.117.10.1319
Mitchell, P., Wang, J. J., and Smith, W. (1997). Association of pseudoexfoliation syndrome with increased vascular risk. Am. J. Ophthalmol. 124, 685–687. doi: 10.1016/s0002-9394(14)70908-0
Musch, D. C., Gillespie, B. W., Niziol, L. M., Lichter, P. R., Varma, R., and Group, C. S. (2011). Intraocular pressure control and long-term visual field loss in the collaborative initial glaucoma treatment study. Ophthalmology 118, 1766–1773. doi: 10.1016/j.ophtha.2011.01.047
Praveen, M. R., Shah, S. K., Vasavada, A. R., Diwan, R. P., Shah, S. M., Zumkhawala, B. R., et al. (2011). Pseudoexfoliation as a risk factor for peripheral vascular disease: a case-control study. Eye 25, 174–179. doi: 10.1038/eye.2010.175
Puska, P. M. (2002). Unilateral exfoliation syndrome: conversion to bilateral exfoliation and to glaucoma: a prospective 10-year follow-up study. J. Glaucoma 11, 517–524. doi: 10.1097/00061198-200212000-00012
Rieck, J. (2013). The pathogenesis of glaucoma in the interplay with the immune system. Invest. Ophthalmol. Vis. Sci. 54, 2393–2409. doi: 10.1167/iovs.12-9781
Ritch, R. (1994). Exfoliation syndrome-the most common identifiable cause of open-angle glaucoma. J. Glaucoma 3, 176–177.
Ritch, R., and Schlotzer-Schrehardt, U. (2001). Exfoliation syndrome. Surv. Ophthalmol. 45, 265–315.
Scharfenberg, E., and Schlotzer-Schrehardt, U. (2012). [PEX syndrome. Clinical diagnosis and systemic manifestations]. Ophthalmologe 109, 952–961.
Schlotzer-Schrehardt, U. (2010). [Oxidative stress and pseudoexfoliation glaucoma]. Klin. Monbl. Augenheilkd. 227, 108–113.
Schlotzer-Schrehardt, U., and Khor, C. C. (2020). Pseudoexfoliation syndrome and glaucoma: from genes to disease mechanisms. Curr. Opin. Ophthalmol. 32, 118–128. doi: 10.1097/icu.0000000000000736
Schlotzer-Schrehardt, U., and Khor, C. C. (2021). Pseudoexfoliation syndrome and glaucoma: from genes to disease mechanisms. Curr. Opin. Ophthalmol. 32, 118–128. doi: 10.1097/icu.0000000000000736
Schlotzer-Schrehardt, U., Kuchle, M., Hofmann-Rummelt, C., Kaiser, A., and Kirchner, T. (2000). [Latent TGF-beta 1 binding protein (LTBP-1); a new marker for intra-and extraocular PEX deposits]. Klin. Monbl. Augenheilkd. 216, 412–419.
Schlotzer-Schrehardt, U., Kuchle, M., Junemann, A., and Naumann, G. O. (2002). [Relevance of the pseudoexfoliation syndrome for the glaucomas]. Ophthalmologe 99, 683–690.
Schlotzer-Schrehardt, U., and Naumann, G. O. (1995). Trabecular meshwork in pseudoexfoliation syndrome with and without open-angle glaucoma. A morphometric, ultrastructural study. Invest. Ophthalmol. Vis. Sci. 36, 1750–1764.
Schlotzer-Schrehardt, U., and Naumann, G. O. (2006). Ocular and systemic pseudoexfoliation syndrome. Am J Ophthalmol 141, 921–937. doi: 10.1016/j.ajo.2006.01.047
Schlotzer-Schrehardt, U., Zenkel, M., Kuchle, M., Sakai, L. Y., and Naumann, G. O. (2001). Role of transforming growth factor-beta1 and its latent form binding protein in pseudoexfoliation syndrome. Exp. Eye Res. 73, 765–780. doi: 10.1006/exer.2001.1084
Siordia, J. A., Franco, J., Golden, T. R., and Dar, B. (2016). Ocular Pseudoexfoliation Syndrome Linkage to Cardiovascular Disease. Curr. Cardiol. Re.p 18:61.
Sunderland, D. K., and Sapra, A. (2021). Physiology, Aqueous Humor Circulation. Treasure Island (FL): StatPearls.
Szentiványi, A. (1968). The beta-adrenergic theory of the atopic abnormality in bronchial asthma. J. Allergy 42, 203–232. doi: 10.1016/s0021-8707(68)90117-2
Tezel, G., Fourth ARVO/Pfizer Ophthalmics Research Institute Conference Working Group (2009). The role of glia, mitochondria, and the immune system in glaucoma. Invest. Ophthalmol. Vis. Sci. 50, 1001–1012. doi: 10.1167/iovs.08-2717
Tezel, G., Yang, X., Luo, C., Peng, Y., Sun, S. L., and Sun, D. (2007). Mechanisms of immune system activation in glaucoma: oxidative stress-stimulated antigen presentation by the retina and optic nerve head glia. Invest. Ophthalmol. Vis. Sci. 48, 705–714. doi: 10.1167/iovs.06-0810
Thorleifsson, G., Magnusson, K. P., Sulem, P., Walters, G. B., Gudbjartsson, D. F., Stefansson, H., et al. (2007). Common sequence variants in the LOXL1 gene confer susceptibility to exfoliation glaucoma. Science 317, 1397–1400. doi: 10.1126/science.1146554
Topouzis, F., Founti, P., Yu, F., Wilson, M. R., and Coleman, A. L. (2019). Twelve-year incidence and baseline risk factors for pseudoexfoliation: the thessaloniki eye study (An American Ophthalmological Society Thesis). Am. J. Ophthalmol. 206, 192–214. doi: 10.1016/j.ajo.2019.05.005
Topouzis, F., Wilson, M. R., Harris, A., Founti, P., Yu, F., Anastasopoulos, E., et al. (2011). Risk factors for primary open-angle glaucoma and pseudoexfoliative glaucoma in the Thessaloniki eye study. Am. J. Ophthalmol. 152, 219–228.e211.
Topper, J. E., and Brubaker, R. F. (1985). Effects of timolol, epinephrine, and acetazolamide on aqueous flow during sleep. Invest. Ophthalmol. Vis. Sci. 26, 1315–1319.
Tsai, T., Reinehr, S., Maliha, A. M., and Joachim, S. C. (2019). Immune mediated degeneration and possible protection in Glaucoma. Front. Neurosci. 13:931.
Von Thun Und Hohenstein-Blaul, N., Bell, K., Pfeiffer, N., and Grus, F. H. (2016). Autoimmune aspects in glaucoma. Eur. J. Pharmacol. 787, 105–118.
Wallukat, G., and Wollenberger, A. (1991). Autoantibodies to beta 2-adrenergic receptors with antiadrenergic activity from patients with allergic asthma. J. Allergy Clin. Immunol. 88, 581–587. doi: 10.1016/0091-6749(91)90151-d
Wallukat, G. N. E., Müller, J., Brinckmann, R., Schimke, J., and Kunze, R. (2002). The Pathophysiological Role of Autoantibodies Directed to G-protein Coupled Receptors and Therapeutic Strategies of Antibody Removal. Lengerich: Pabst Science Publishers.
Wax, M. B., and Molinoff, P. B. (1987). Distribution and properties of beta-adrenergic receptors in human iris-ciliary body. Invest Ophthalmol Vis Sci 28, 420–430.
Wayman, L., Larsson, L. I., Maus, T., Alm, A., and Brubaker, R. (1997). Comparison of dorzolamide and timolol as suppressors of aqueous humor flow in humans. Arch. Ophthalmol. 115, 1368–1371. doi: 10.1001/archopht.1997.01100160538002
Yablonski, M. E., Zimmerman, T. J., Waltman, S. R., and Becker, B. (1978). A fluorophotometric study of the effect of topical timolol on aqueous humor dynamics. Exp. Eye Res. 27, 135–142. doi: 10.1016/0014-4835(78)90083-0
Yokusoglu, M. (2012). Is pseudoexfoliation syndrome a risk factor for cardiovascular diseases? Anadolu Kardiyol. Derg. 12, 488–489.
Zenkel, M., Lewczuk, P., Junemann, A., Kruse, F. E., Naumann, G. O., and Schlotzer-Schrehardt, U. (2010). Proinflammatory cytokines are involved in the initiation of the abnormal matrix process in pseudoexfoliation syndrome/glaucoma. Am. J. Pathol. 176, 2868–2879. doi: 10.2353/ajpath.2010.090914
Zenkel, M., and Schlotzer-Schrehardt, U. (2014). The composition of exfoliation material and the cells involved in its production. J. Glaucoma 23, S12–S14.
Keywords: autoantibodies, glaucoma, pseudoexfoliation, β2-adrenergic receptor, imbalanced autonomic theory
Citation: Hohberger B, Schlötzer-Schrehard U, Mardin C, Lämmer R, Munoz L, Kunze R, Herrmann M and Wallukat G (2021) Inhibitory and Agonistic Autoantibodies Directed Against the β2-Adrenergic Receptor in Pseudoexfoliation Syndrome and Glaucoma. Front. Neurosci. 15:676579. doi: 10.3389/fnins.2021.676579
Received: 05 March 2021; Accepted: 02 June 2021;
Published: 06 August 2021.
Edited by:
Eric Boué-Grabot, UMR 5293 Institut des Maladies Neurodégénératives (IMN), FranceReviewed by:
Madeleine W. Cunningham, University of Oklahoma Health Sciences Center, United StatesCopyright © 2021 Hohberger, Schlötzer-Schrehard, Mardin, Lämmer, Munoz, Kunze, Herrmann and Wallukat. This is an open-access article distributed under the terms of the Creative Commons Attribution License (CC BY). The use, distribution or reproduction in other forums is permitted, provided the original author(s) and the copyright owner(s) are credited and that the original publication in this journal is cited, in accordance with accepted academic practice. No use, distribution or reproduction is permitted which does not comply with these terms.
*Correspondence: Bettina Hohberger, YmV0dGluYS5ob2hiZXJnZXJAdWstZXJsYW5nZW4uZGU=
†These authors have contributed equally to this work
Disclaimer: All claims expressed in this article are solely those of the authors and do not necessarily represent those of their affiliated organizations, or those of the publisher, the editors and the reviewers. Any product that may be evaluated in this article or claim that may be made by its manufacturer is not guaranteed or endorsed by the publisher.
Research integrity at Frontiers
Learn more about the work of our research integrity team to safeguard the quality of each article we publish.