- 1Good Doctor Research Institute, College of Medicine, Korea University, Seoul, South Korea
- 2Department of Physiology, College of Medicine and Neuroscience Research Institute, Korea University, Seoul, South Korea
Methylene blue (MB) is a cationic thiazine dye, widely used as a biological stain and chemical indicator. Growing evidence have revealed that MB functions to restore abnormal vasodilation and notably it is implicated even in pain relief. Physicians began to inject MB into degenerated disks to relieve pain in patients with chronic discogenic low back pain (CDLBP), and some of them achieved remarkable outcomes. For osteoarthritis and colitis, MB abates inflammation by suppressing nitric oxide production, and ultimately relieves pain. However, despite this clinical efficacy, MB has not attracted much public attention in terms of pain relief. Accordingly, this review focuses on how MB lessens pain, noting three major actions of this dye: anti-inflammation, sodium current reduction, and denervation. Moreover, we showed controversies over the efficacy of MB on CDLBP and raised also toxicity issues to look into the limitation of MB application. This analysis is the first attempt to illustrate its analgesic effects, which may offer a novel insight into MB as a pain-relief dye.
Introduction
In 1876, German chemist Heinrich Caro synthesized methylene blue (MB) for the first time in history, which was basically applied for textiles as an aniline dye. Around the same time, it was found that MB is capable of staining cells by binding to their structures, in addition, sometimes inactivating bacteria. This discovery prepared the innovative ground for biological or medical studies related to MB. Numerous scientists applied it to a variety of animal and bacterial studies, importantly Paul Ehrlich introduced it to humans in 1891 as an anti-malarial agent. Indeed, this dye has been introduced to treat different diseases even including dementia, cancer, and depression (Wainwright and Crossley, 2002; Schirmer et al., 2003; Schirmer et al., 2011).
In the present day, MB is primarily known for a vasoconstrictor. It downregulates basically nitric oxide (NO), which is responsible for relaxing vascular smooth muscle, and leads to vasoconstriction (Wolin et al., 1990; Pan et al., 2019). However, under pathological conditions, NO is overexpressed and then contributes to inflammation as a pro-inflammatory mediator (Luo and Cizkova, 2000; Lundberg et al., 2008; Leiper and Nandi, 2011). Of note, MB suppresses the iNOS/NO-mediated inflammatory signaling by directly downregulating inducible NO synthase (iNOS) (Cohen et al., 2000). In addition, P2X receptor family, long non-coding RNA (lncRNA), and inflammasome are also involved in MB-mediated anti-inflammation (Ahn et al., 2017; Li et al., 2018; Zheng and Li, 2019). Accordingly, MB application can be an important strategy to reduce inflammation and pain.
In general, voltage-gated sodium channels (VGSCs) play an important role in evoking action potentials (APs) and, when activated, they consequently contribute to exciting neurons and thus facilitating communication with other ones. Interestingly, MB decreased significantly INA (voltage-gated sodium currents) in hippocampal CA1 neurons and, more importantly, attenuated markedly neural firing rates in the afferent nerve fibers (Zhang et al., 2010; Lee et al., 2021), which implies that MB may impede pain transmission by dampening neuronal excitability elicited by VGSCs.
In addition, MB may contribute to pain reduction by hindering or damaging nerve connection to tissues, which is referred to as denervation. Indeed, it can make affected nerve fibers or neurons incapable of sensing pain. Peng et al. (2007) conducted intradiscal MB injection in patients with chronic discogenic low back pain (CDLBP) to relieve pain for the first time. Most of patients showed encouraging results and this improvement lasted at least one year. Moreover, in a case, there were no noticeable side effects and complications in those patients even after prolonged follow-ups (Peng et al., 2010). However, as opposed to expectations, these outstanding outcomes faced a lot of challenges. We will deal with the controversies around the results in the relevant section.
Despite these remarkable reports, MB has not drawn much attention from the public specifically concerning pain. Thus, in this review, we will show MB-driven analgesic effects and their possible mechanisms along with the relevant experimental evidence and clinical cases. Finally, we will provide a novel insight into MB as a pain reliever.
MB and Anti-Inflammation
Blockade of NOS/NO-Mediated Inflammatory Signaling
Inflammation is tightly correlated with pain as a critical cause. It directly or indirectly induces nociceptive responses and in many cases underlies pain or pain-related diseases (Zhang H. et al., 2016; Harth and Nielson, 2019; Matsuda et al., 2019). It is well known that MB decreases NO formation by directly suppressing endothelial NOS (eNOS) expression, and blocks also the conversion of guanosine triphosphate (GTP) to cyclic guanosine monophosphate (cGMP) by suppressing soluble guanylate cyclase (sGC) expression in vascular smooth muscles, which in turn leads to vasoconstriction (Figure 1A; Wolin et al., 1990; Pan et al., 2019).
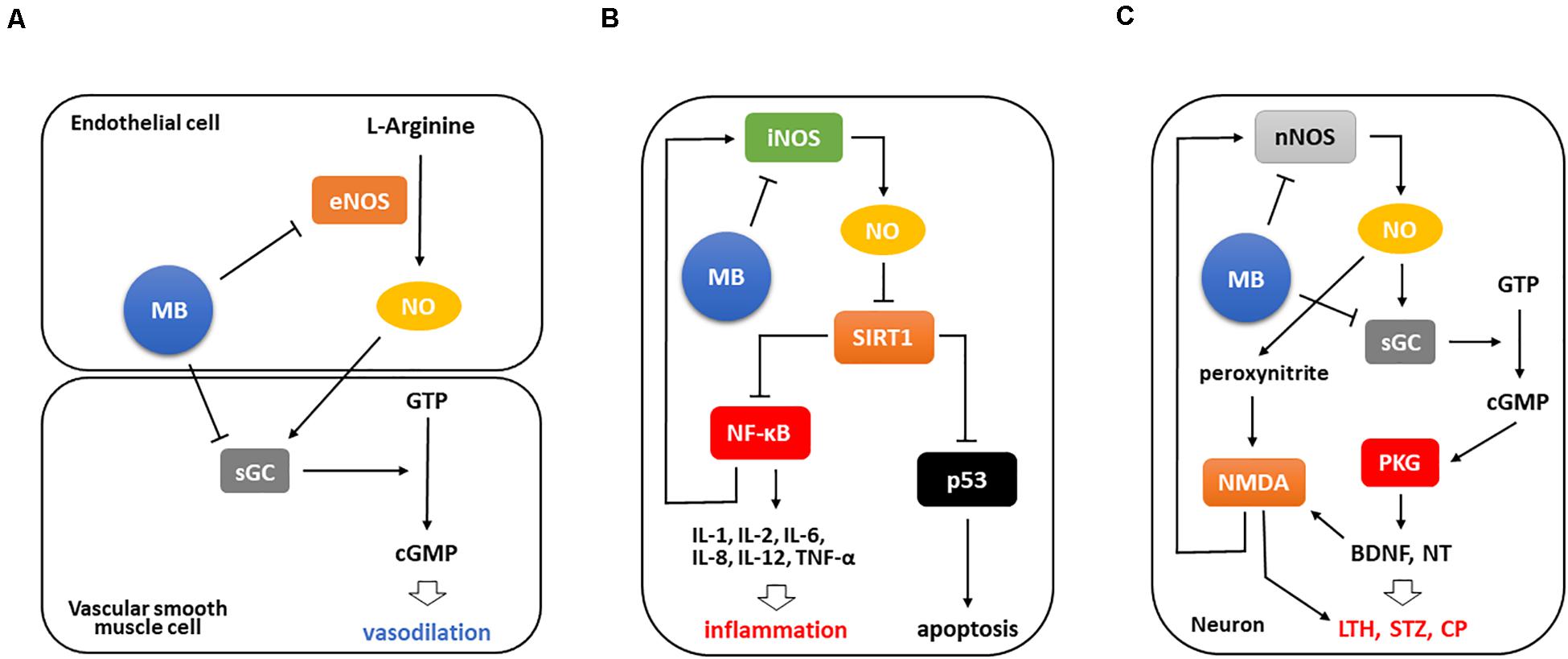
Figure 1. MB is involved in anti-inflammation by suppressing iNOS/NO-NF-κB pathway. (A) Basically, MB downregulates both eNOS and sGC that are major factors converting GTP to cGMP, ultimately leading to vasoconstriction. (B) Upon tissue injury, iNOS functions as a strong inflammatory mediator in different types of cells. It inhibits Sirt1 activation by NO-mediated S-nitrosylation, which in turn activates NF-κB and p53 to facilitate inflammatory cytokine expression and apoptosis, respectively. Of note, NF-κB activation intensifies these events by activating iNOS/NO-NF-κB pathway. Conversely, MB directly abates iNOS expression and moreover decreases the binding of NF-κB to iNOS promoter, which consequently interrupts this inflammatory signaling. (C) Meanwhile, NMDA receptors are activated during nerve injury and induces Ca2+ influx, which then results in the excessive expression of nNOS and markedly activates nNOS/NO signaling. The increased NO production stimulates NMDA receptors and triggers NO/cGMP/PKG cascade, which promotes the subsequent BNDF upregulation and neurotransmitter release and ultimately induces long-term hyperexcitability and central sensitization. Notably, BDNF and peroxynitrite potentiate NMDA receptors, which stimulate nNOS expression again. However, MB weakens these responses by inhibiting nNOS and sGC activation, thus may prevent the development of persistent pain.LTH, long-term hyperexcitability; STZ, sensitization; NT, neurotransmitter; CP, chronic pain.
The MB-Induced Interruption of iNOS/NO-NF-κB Signaling
Based on this pathway, MB weakens inflammation since NO plays a crucial role in the pathogenesis of inflammation as a pro-inflammatory mediator. This dye inhibits iNOS/NO pathway to affect nuclear factor kappa-light-chain-enhancer of activated B cells (NF-κB) activation. Silent information regulator 1 (Sirt1), which is a deacetylase and have a protective role under stress conditions, inactivates NF-κB and tumor protein p53 (p53) by deacetylating them. In contrast, iNOS/NO activates these two transcription factors by inactivating Sirt1 via S-nitrosylation (Nakazawa et al., 2017). NF-κB activation facilitates inflammatory cytokine expression, importantly, upregulates iNOS to enhance inflammatory signaling (Nakazawa et al., 2017; Jiang et al., 2020), and p53 activation induces cell death (Aubrey et al., 2018). However, MB prevents these events by downregulating iNOS in multiple inflammatory milieus. It directly inhibits iNOS expression and also attenuates the binding of NF-κB to iNOS promoter, thus resulting in the blockade of iNOS/NO-NF-κB signaling (Figure 1B; Huang et al., 2015).
Specifically, in human cartilage explants, MB decreased NO accumulation and iNOS expression in a dose dependent manner, and upregulated transforming growth factor beta (TGF-β) receptors, known as an important factor for cartilage matrix synthesis. As a result, it prevented the degradation of cartilage matrix and proteoglycan (Cohen et al., 2000). In ulcerative colitis rats, MB decreased remarkably NO production and inflammatory cytokine (IL-1β, IL-6, and TNF-α) levels. Functionally, with the alleviated tissue injury and edema in the submucosa, there was a significant improvement in intestinal permeability after MB application (Dinc et al., 2015). Accordingly, these studies demonstrated that MB has a critical role in negatively modulating NOS expression and NO production and ultimately is able to induce functional improvement in the inflamed tissues.
During nerve injury, Toll-like receptor 4 (TLR4) initiates neuroimmune activation in the nervous system. Notably, TLR4 activates NF-κB by mediating its nuclear localization, which in turn promotes pro-inflammatory cytokine expression and ultimately leads to inflammatory hyperalgesia (Lacagnina et al., 2018; Yadav and Surolia, 2019). Tanga et al. demonstrated for the first time that upon nerve injury (L5 nerve transection), behavioral hypersensitivity is induced via TLR4/NF-κB pathway, suggesting that TLR4 activation is a critical cause of the pathogenesis of neuropathic and chronic pain (Tanga et al., 2005). In this context, targeting TLR4/NF-κB pathway can be recommended as a decisive therapeutic strategy to relieve nerve injury–induced neuroinflammation and pain (Yadav and Surolia, 2019; Ye et al., 2020).
Interestingly, TLR4 is much involved in iNOS activation (Deng et al., 2015). This implies that MB may participate in suppressing the development and maintenance of neuropathic pain by downregulating directly iNOS and weakening NF-κB activation as previously described. A clinical study showed that systemic MB administration improved 10 patients with chronic refractory neuropathic pain, which was assumably due to the MB-mediated blockade of iNOS/NO pathway (Miclescu et al., 2015).
The MB-Induced Interruption of nNOS/cGMP/PKG Signaling
Lastly, neuronal NOS (nNOS), predominantly found in neurons, is also responsible for NO production and engaged in NO-mediated pathway (Kourosh-Arami et al., 2020). This enzyme has been much investigated specifically concerning chronic and inflammatory pain. In particular, nNOS has a critical role in the development of chronic and neuropathic pain upon nerve injury (Kim et al., 2011), while iNOS is partially related to it (Rocha et al., 2020). Interestingly, a study demonstrated that spinal nNOS, not eNOS and iNOS, is significantly upregulated after inflammatory pain induction and contributes to central sensitization (Chu et al., 2005). In this respect, nNOS has been highlighted as a target to dampen pain in both inflammatory and non-inflammatory milieus (Mukherjee et al., 2014; Demir et al., 2019). Moreover, it was found that NO/cGMP-mediated pathway, a downstream target of nNOS, is crucial for the development and maintenance of pain (Li et al., 2020), and cGMP protein kinase (PKG) is considered as a potent generator in this event since leading to long-term hyperexcitability and pain sensitization in neurons by inducing neurotransmitter release and upregulating brain-derived neurotrophic factor (BDNF) (Lewin and Walters, 1999; Sung et al., 2017; Wang et al., 2021). Furthermore, BDNF released during nerve injury and peroxynitrite activated via nNOS/NO signaling potentiate N-methyl-D-aspartate (NMDA) receptors, which then induces long-term hyperexcitability and upregulates nNOS, respectively (Pall, 2002; Chen et al., 2014; Figure 1C).
Importantly, MB is able to downregulate both nNOS and sGC, resulting in the inhibition of nNOS/NO-mediated signaling. Rey-Funes et al. (2016) demonstrated that MB protects retinal damage in rats with ischemic proliferative retinopathy, which is associated with local inflammation in the ischemic retina. MB suppressed the expression levels of nNOS and proangiogenic factors such as matrix metalloproteinase (MMP)-2 and MMP-9 and upregulated pigment epithelium-derived factor (PEDF), ultimately leading to the reduction of inner retinal thickness, gliosis, and retinal angiogenesis.
Inhibited NOS Activation Potentiates Opioidergic Effects
Opioids are substances with similar effects to those of morphine and used primarily for lessening pain. Basically, opioids act by binding to opioid receptors (ORs), which show analgesia by reducing cyclic adenosine monophosphate (cAMP) and causing the resultant decrease in the excitatory ion channels (Quirion et al., 2020; Sun et al., 2020; Kaski et al., 2021).
However, a number of issues concerning their addiction and adverse effects have been raised. In effect, morphine application may induce inflammatory cytokine expression such as IL-1β, IL-6, and TNF-α, ultimately contributing to inflammation and neurotoxic events. Importantly, it was found that reduced nNOS activation not only enhances the antinociception of morphine but also inhibits the morphine-induced neurotoxicity by downregulating inflammatory mediators (Machelska et al., 1997; Osmanlıoğlu et al., 2020). In this regard, MB may also contribute to these events as a potent NOS inhibitor. A clinical report showed that oral MB administration relieved oral mucositis-related intractable pain and more importantly reduced significantly morphine requirement in the relevant patients (Roldan et al., 2017).
There are some links between TLR4, OR, and cholecystokinin (CCK). CCK, an important neurohormone, plays a variety of roles in the nervous system and notably engaged in pain reduction mainly via CCK-B receptors (Roca-Lapirot et al., 2019; Keppel Hesselink, 2020). Interestingly, this peptide functions to inhibit OR activity and thus reduces the morphine-induced antinociception (Torres-López et al., 2007; Yang et al., 2018). Meanwhile, upon nerve injury, Tlr4 genes are significantly expressed in the midbrain and medulla of CCK-B receptor knockout mice compared to those of wild type, implying that CCK is involved in innate immunity (Kõks et al., 2008). This result is supported by recent studies that CCK has a pivotal role in anti-inflammation by decreasing inflammatory mediators (Funakoshi et al., 2019; Saia et al., 2020). It has not yet been confirmed that MB is related to these links. However, it is believed that MB possibly shows a synergistic effect with morphine and CCK, thus enhancing anti-inflammatory and analgesic effects.
Downregulation of P2XR and lncRNA
MB-Mediated P2XR Downregulation Induces Anti-inflammation and Pain Relief
Purinergic P2X receptor subtypes have been highlighted as a nociceptor that causes inflammation and pain. These receptors are characterized by ligand-gated ionotropic channels activated in response to the binding of adenosine 5′-triphosphate (ATP), exhibiting a non-selective cationic permeability such as K+, Na+, and Ca2+ that can contribute to opening voltage-gated channels on neurons by depolarizing membrane potential and activate a diversity of intracellular Ca2+-dependent signaling pathways (Surprenant and North, 2009; Schmid and Evans, 2019). In particular, P2 × 3 and P2 × 2/3 receptors among the subunits are distributed in the dorsal root ganglion (DRG) and the central terminal of primary afferent fibers, mainly small-diameter unmyelinated C-fibers, and play a critical role in pain transmission in the periphery (North, 2004; Bernier et al., 2018; Stephan et al., 2018). Interestingly, MB is highly correlated with this P2X receptor-mediated events.
Li et al. showed that MB alleviated inflammation and pain in osteoarthritis (OA) rabbits by negatively modulating P2 × 3 receptors. In this study, after intra-articular MB administration, weight distribution in the hind paw was significantly restored and the swelling ratio in the inflamed knees also markedly declined. Inflammatory cytokine levels (TNF-α, IL-1β, IL-6, and IL-8) were also remarkably reduced in the articular cartilage with a significant decrease in P2 × 3 receptor expression. Moreover, this event was reversed by P2 × 3 overexpression (Li et al., 2018).
Decreased lncRNA Contributes to Anti-inflammation
Meanwhile, lncRNA has been highlighted as a new player in gene regulation. Previous studies revealed that increased lncRNA is a critical cause of neuropathic pain, and interacts with P2XRs to initiate and maintain pain (Zhao et al., 2013; Li et al., 2017). Also, it is much implicated in inflammatory events (Chen et al., 2019).
Interestingly, MB may contribute to anti-inflammation and pain relief by downregulating this lncRNA. Zheng and Li demonstrated that MB negatively regulates a long non-coding RNA (lncRNA), specifically a chondrocyte inflammation–associated lncRNA (CILinc02), overexpressed in osteoarthritic cartilage tissues and cultured OA cells, and decreased inflammatory cytokine levels (IL-1, IL-6, and IL-17). Of note, MB suppressed even chondrocyte degradation. Conversely, CILinc02 overexpression resulted in inflammation and apoptosis (Zheng and Li, 2019).
Inhibition of Inflammasome Formation and Activation
Inflammasome is an intracellular multiprotein complex that functions to process cytokine precursors into their mature forms and induce an inflammatory form of programmed cell death termed pyroptosis, which, ultimately, has a crucial role in providing host defense against harmful intruders (Matsuda et al., 2019).
Upon stimulation, inflammasome initiates the expression of cytosolic sensing proteins, called a priming step, which include nucleotide-binding oligomerization domain (NOD), leucine-rich repeat (LRR), pyrin domain-containing protein 3 (NLRP3), NLR family caspase recruitment domain (CARD)-containing protein 4 (NLRC4), absent in melanoma 2 (AIM2), and cytokine precursors. And inflammasome requires the second phase triggered by pathogen- and danger-associated molecular patterns, an activation step, to assemble a cytosolic sensor, an adaptor [apoptosis-associated speck-like protein containing a CARD (APC)], and an effector (pro-caspase-1) into inflammasome complex, and to secrete IL-1β and IL-18 (Kanneganti, 2015; Rathinam and Fitzgerald, 2016; Swanson et al., 2019; Yang et al., 2019).
Interestingly, it was found that MB inhibits inflammasome activation by interrupting both steps. Ahn et al. elucidated the relation between MB and inflammasome for the first time, demonstrating that MB suppresses both canonical and non-canonical processes by decreasing dose-dependently the expression of NLRP3, pro-IL-1β and caspase-1, as well as reactive oxygen species (ROS) production (Ahn et al., 2017).
Similarly, neuroinflammation triggered by spinal cord injury (SCI) was alleviated by the MB-mediated inhibition of NLRP3 and NLRC4 inflammasome formation in microglia. In SCI animals, following MB administration, their locomotive activities were ameliorated and inflammatory cytokine levels (IL-1β, IL-6, and TNF-α) were also diminished (Lin et al., 2017). Overall MB-mediated anti-inflammation pathways are summarized in Table 1.
MB and Sodium Current Reduction
Early Studies for MB and INA
It is an old story that MB is involved in the reduction of excitatory synaptic currents. In earlier periods, Armstrong, Croop, and Starkus conducted pioneering experiments to explore the inactivation of sodium channels and their electrophysiological profiles using an artificial inactivation model established by administering MB into squid giant axons. The dyes blocked efficiently both the Ig (gate current) and INA, as well as simulated the channel inactivation even after pronase, an agent of eliminating normal inactivation, treatment (Armstrong and Croop, 1982). Similarly, a subsequent study showed that MB weakened sodium currents in both normal and pronase-treated crayfish giant axons (Starkus et al., 1984). In the two studies, MB was believed to be a gate-immobilizing open-pore blocker rather than an inactivation enhancer, since directly blocking sodium pores rather than promoting or speeding the inactivation process (Figure 2; Armstrong and Croop, 1982; Starkus et al., 1984).
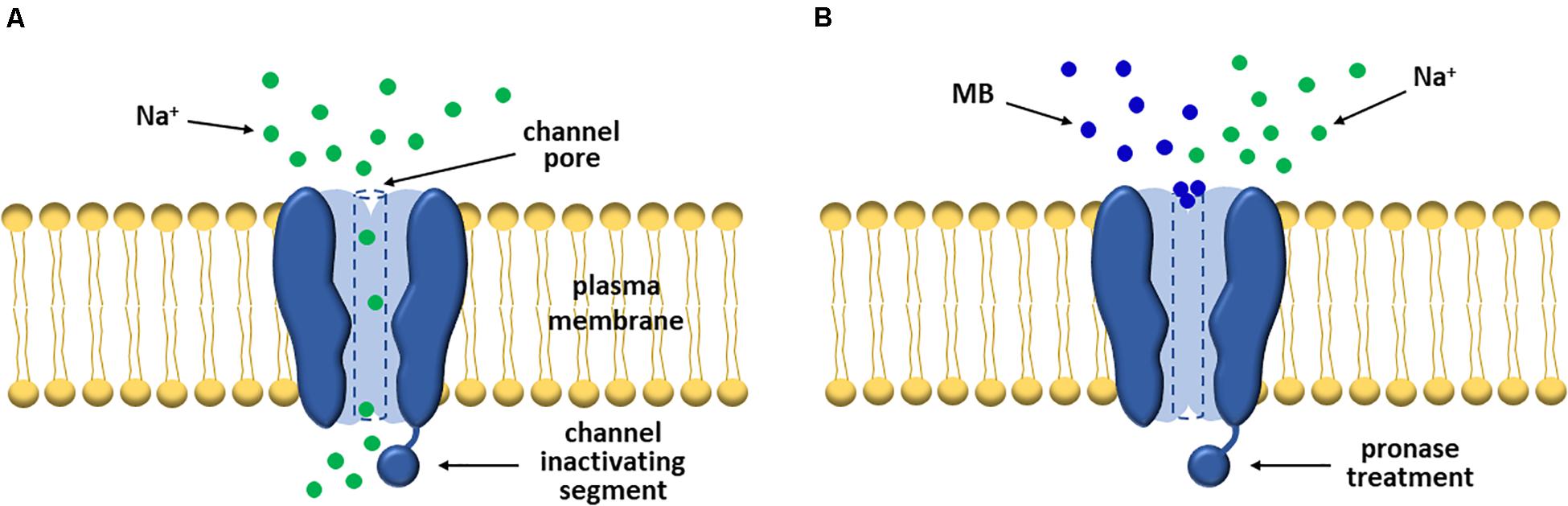
Figure 2. MB significantly attenuates sodium currents by blocking VGSCs. (A) In general, VGSCs allow sodium ions to flow into the cell in the activated state. (B) However, early researchers found that the gate and sodium currents of the channels were markedly suppressed post-MB treatment. And notably, this event was maintained even after pronase treatment. Thus, they interpreted this event as MB functions as a pore blocker rather than an inactivation enhancer.
Altered VGSC-Dependent Currents and Neural Firing Rates
Zhang et al. (2010) explored the effect of MB on sodium currents and found that MB alters VGSC-mediated electrophysiological events. MB application diminished the peak amplitude of INA up to 45% at 100 μM, number of repetitive firings, and Vmax (AP upstroke velocity) in rat hippocampal slices. In addition, it accelerated INA inactivation and even retarded the shift from inactivation to recovery state.
Importantly, Lee et al. (2021) performed the first in vivo experiments to investigate the link between MB-mediated neural firing patterns and pain reduction in rats using in vivo single nerve recordings and behavioral studies. This study showed dramatic results that neural firing rates significantly decreased in a dose-dependent manner after MB administration and, notably, this event lasted longer than that of lidocaine, showing anesthetic-like firing patterns. Ultimately, MB improved pain behaviors in rodents. These results demonstrate that MB abates pain by markedly suppressing neural excitability (Lee et al., 2021).
Silenced Excitable Neurons as a Critical Cause of Pain Relief
Exciting neurons is the most fundamental step to convey signals and to relay or cause several subsequent responses. Moreover, VGSCs are directly engaged in AP generation and affect the resultant reactions including signal propagation, opening other channels, vesicle release, cell-to-cell communication, and other related responses (Kruger and Isom, 2016; Wang et al., 2017). Accordingly, it is natural to postulate that VGSCs and AP generation play a crucial role even in pain transmission (Dubin and Patapoutian, 2010; Bennett et al., 2019). Interestingly, multiple evidence demonstrates that MB silences excitable cells (or nerves) by significantly attenuating INA and AP production, which ultimately leads to pain relief (Armstrong and Croop, 1982; Starkus et al., 1984, 1993; Zhang et al., 2010; Lee et al., 2021). Therefore, these two crucial electrophysiological activities, weakened sodium currents and decreased neural firing rates, are possible causes that can elucidate how MB contributes to pain relief.
MB and Denervation
Early Cases for MB Application
Denervation is defined as loss or interruption of nerve connection to an organ, which thereby may contribute to pain relief by making nerves shut off sensory transmission. In history, MB began to rear its head for denervation firstly to treat itch. In 1968, Professor Rygick in the Moscow University reported for the first time an effect of MB on chronic pruritus ani (PA), characterized by severe itching in the perianal area, noting that patients with chronic PA was significantly improved after subcutaneous MB administration (Rygick, 1968). In 1979, Wollock and Dintsman inspired by Rygick’s work conducted also an intervention to treat intractable PA locally administering MB into the perianal skin and produced remarkable results that eight out of nine patients were completely improved post-MB treatment. Importantly, Eusebio et al. conducted an electron microscopic investigation aimed at the perianal skin of intractable PA patients treated with subcutaneous MB and no distinct nerve endings were detected in the samples even after a long-term (7 years) follow-up. Thus, they interpreted that this improvement was attributed to death of nerve endings connected to the perianal skin, that is, denervation (Eusebio et al., 1990). Most recently, PA patients treated with MB showed satisfactory scores at 6-week, as well as 3-year, follow-ups. The recurrence rate was low (7.5%) even three years after MB treatment. In the study, MB was also considered as a critical agent to sever nerve endings (Kim et al., 2019).
Intradiscal MB Injection and Debates
The Novel Intervention to Treat Chronic Pain
Similar to the itching cases, MB was applied to inflamed intervertebral disks (IVDs) in CDLBP patients. In 2007, Peng et al. (2007) reported encouraging outcomes in the treatment of CDLBP using a minimal invasive method, intradiscal MB injection. They believed that MB has a capacity to mitigate pain in the patients by blocking nerve conduction or destroying nerve endings around the painful disks that may be vascularized and extensively innervated owing to disk degeneration. This was a first prospective clinical trial conducted for examining the pain-relieving effect of MB in human subjects. In this study, MB was administered into the affected disks of CDLBP patients using a discographic needle (1 ml of 1% MB), then pain intensity and disability of the patients were measured by visual analog scale (VAS) and oswestry disability index (ODI). As a result, most patients were evidently or completely improved and there were no side effects or complications during long-term follow-up periods (12-23 months) (Peng et al., 2007). After four years, Peng et al. (2010) conducted a randomized placebo-controlled trial in 72 eligible participants, who accepted intradiscal MB injection or placebo treatment. For numerical rating scale (NRS) and ODI scores, there were dramatic or obvious improvements in most patients treated with MB. Of note, these events lasted even to the subsequent follow-up visits at 12 and 25 months.
Controversies and Refutations for Intradiscal MB Application
However, their outcomes were immediately challenged and debated over a more fundamental issue. Bogduk (2010) explained that cultural factors may affect the manner in which patients report their outcomes, and Chinese patients may report their physical conditions more favorably to physicians or assessors than United States or British patients do. Another commentary was about the safety of MB. MB has a potent neurotoxic effect and can be leaked to the spinal canal through annular tears during intradiscal administration since discogenic pain is associated with annular tears (Levine and Richeimer, 2011). Lastly, a researcher argued that there is no LBP treatment that can immunize against new LBP episodes, emphasizing that discography accelerates disk degeneration after years (Schiltenwolf et al., 2011).
In 2019, an article directly refuted the previous results of Peng et al. (2010) designing a study protocol almost identical to their previous study to verify their outcomes (Kallewaard et al., 2019). As a result, there were no significant differences between MB plus lidocaine treatment group and placebo plus lidocaine group after NRS, ODI, quality of life (QOL), and VAS measurement. They concluded that the outcomes of the previous study were not able to be reproduced (Kallewaard et al., 2019).
Short-Term Effect of Intradiscal MB Application on Chronic Low Back Pain
Over years after Peng et al. (2010), there have been multiple attempts to perform intradiscal MB injection in CDLBP patients. Kim et al. revealed that following intradiscal MB injection, there was a significant decrease in VAS (1, 3, and 6 months) and ODI (one and three months) scores in 20 CDLBP patients, but, one year after intervention, such outcomes were reproduced only in five patients (Kim et al., 2012). Accordingly, the patients showed short-term improvement (three or six months) after intervention. Gupta et al. showed a relatively low success rate (13%) in eight CDLBP patients after a single intradiscal MB injection (Gupta et al., 2012). Levi et al. revealed that there were very limited benefits in the VAS and ODI scores in 16 CDLBP patients. Only a few patients (25% or less) met the criteria for success at the follow-up periods (Levi et al., 2014). Lastly, Zhang X. et al. (2016) reported the short-term clinical outcomes in CDLBP patients. Their NRS and ODI scores were significantly decreased at 1- to 6-month follow-ups. Imaging experiments showed that the mean apparent diffusion coefficient (ADC) and T2 values significantly increased at 6- and 12-month, but not 3-month, follow-ups.
Collectively, it was found that MB is effective in CDLBP patients at least in a short period (until 3 or 6 months after treatment), which is also supported by the most recent review report (Deng et al., 2021). Similarly, radiofrequency (RF) denervation also resulted in short-term improvement (4 weeks or 6 months) in patients with chronic LBP (Leclaire et al., 2001; Nath et al., 2008; Al-Najjim et al., 2018). In addition, epidermal nerve fibers were regenerated in healthy humans about 100 days after capsaicin-induced denervation (Polydefkis et al., 2004). Regarding knee osteoarthritis, RF denervation contributed to pain reduction in patients with inflamed knee joints and their joint function was ameliorated. This improvement lasted 6 and 3 months, respectively (Wang R. et al., 2019). Based on these results, it is believed that denervating effects do not exceed 6 months. Accordingly, the short-term improvement observed in CDLBP patients is interpreted reasonable. Overall results of the studies were summarized in Table 2.
MB and Toxicity Issues
Serotonin Toxicity
The Contribution of MB to Serotonin Toxicity as a Potent MAO Inhibitor
In the last 20 years, it has been reported that depressive or anxiety patients taking serotonergic medications experienced ST, also referred to as serotonin syndrome, after MB administration. The investigators and medical doctors have believed that the toxicity is deeply linked with the synergism of MB and the medications, and that such patients may be more vulnerable to ST (Ng et al., 2008; Ng and Cameron, 2010; Kapadia et al., 2016; Wolvetang et al., 2016).
ST is characterized by the excessive accumulation of serotonin into the body, which, ultimately, leads to neuromuscular hyperexcitability by the excessive serotonergic agonism of the central and peripheral nervous system, whose clinical findings include agitation, tremor, inducible and ocular clonus, diaphoresis, hyperreflexia, hypertonia, and hyperthermia (over 38°C) (Boyer and Shannon, 2005). A great number of case reports showed that these events occurred predominantly in mental patients who had been taking serotonergic antidepressant medications including fluoxetine [a selective serotonin reuptake inhibitor (SSRI)] (Martindale and Stedeford, 2003; Kapadia et al., 2016), paroxetine, a SSRI (Bach et al., 2004; Mihai et al., 2007; Ng et al., 2008; Shanmugam et al., 2008; Schwiebert et al., 2009; Wolvetang et al., 2016), venlafaxine [a selective serotonin and norepinephrine reuptake inhibitor (SSNRI)] (Majithia and Stearns, 2006), citalopram, a SSRI (Mathew et al., 2006; Pollack et al., 2009), duloxetine, a SSNRI (Rowley et al., 2009), and clomipramine [a serotonin reuptake inhibitor (SRI)] (Khan et al., 2007), and have been commonly interpreted to be attributed to the reaction of MB as a potent monoamine oxidase A (MAO-A) inhibitor (Gillman, 2011; Top et al., 2014). In effect, MAO has a part in the degradation process of a diversity of monoamines such as serotonin, epinephrine, norepinephrine, dopamine, and histamine (Yeung et al., 2019; Floris et al., 2020). In this regard, MB augments serotonin levels in the synaptic cleft by suppressing the activity of MAO, which, furthermore, may lead to ST along with the use of SRIs, SSRIs, and SSNRIs due to over-enhanced serotonergic transmission (Zuschlag et al., 2018).
The Risk of Intravenous MB Application in Patients Taking Serotonergic Medications
Previous studies showed that ST was precipitated after intravenous MB administration predominantly at doses of 5-7.5 mg/kg except for the case of a 65-year-old woman (1.75 mg/kg) (Mihai et al., 2007; Ng et al., 2008). More importantly, Gillman reported that even a low dose of MB (0.75 mg/kg) administered via the intravenous route may reach peak plasma concentration and cause CNS toxicity (or ST) in those being treated with the medications facilitating serotonergic transmission (Schwiebert et al., 2009; Gillman, 2011). The drug safety update, a newsletter issued by the Medicines and Healthcare products Regulatory Agency (MHRA), also informed that patients who have recently treated with serotonergic antidepressants should avoid intravenous MB and be closely observed if administered with MB, and that intravenous MB can be approved only for patients with drug-induced methemoglobinemia at a dose of 1–2 mg/kg (MHRA, 2009). Oral administration of MB is not likely to cause ST since the MB concentration in blood and brain was significantly higher after intravenous, compared to oral, administration (Peter et al., 2000; Top et al., 2014). Therefore, we need to avoid intravenous route and consider about proper dosage to prevent this toxicity event.
Other Issues
A previous study demonstrated that MB deteriorated the dendritic arbor of isolated neurons and was engaged in cell death in a dose-dependent manner (no neurotoxic effects at concentrations of 0.25 μM or less) (Vutskits et al., 2008). More recently, it was found that MB has a detrimental effect on the viability of nucleus pulposus and annulus fibrosus cells. But MB at lower doses had little effect on both (Wang X. et al., 2019; Zhang et al., 2019). Thus, these results imply that high-dose MB may impair them, thus we need to consider about proper dosage in the clinical setting.
Discussion
In the present review, we illustrated MB-driven analgesic effects and their possible mechanisms based on key clinical and experimental studies. In effect, MB is much closely involved in pain relief via the following major actions: anti-inflammation, sodium current reduction, and denervation (Figure 3).
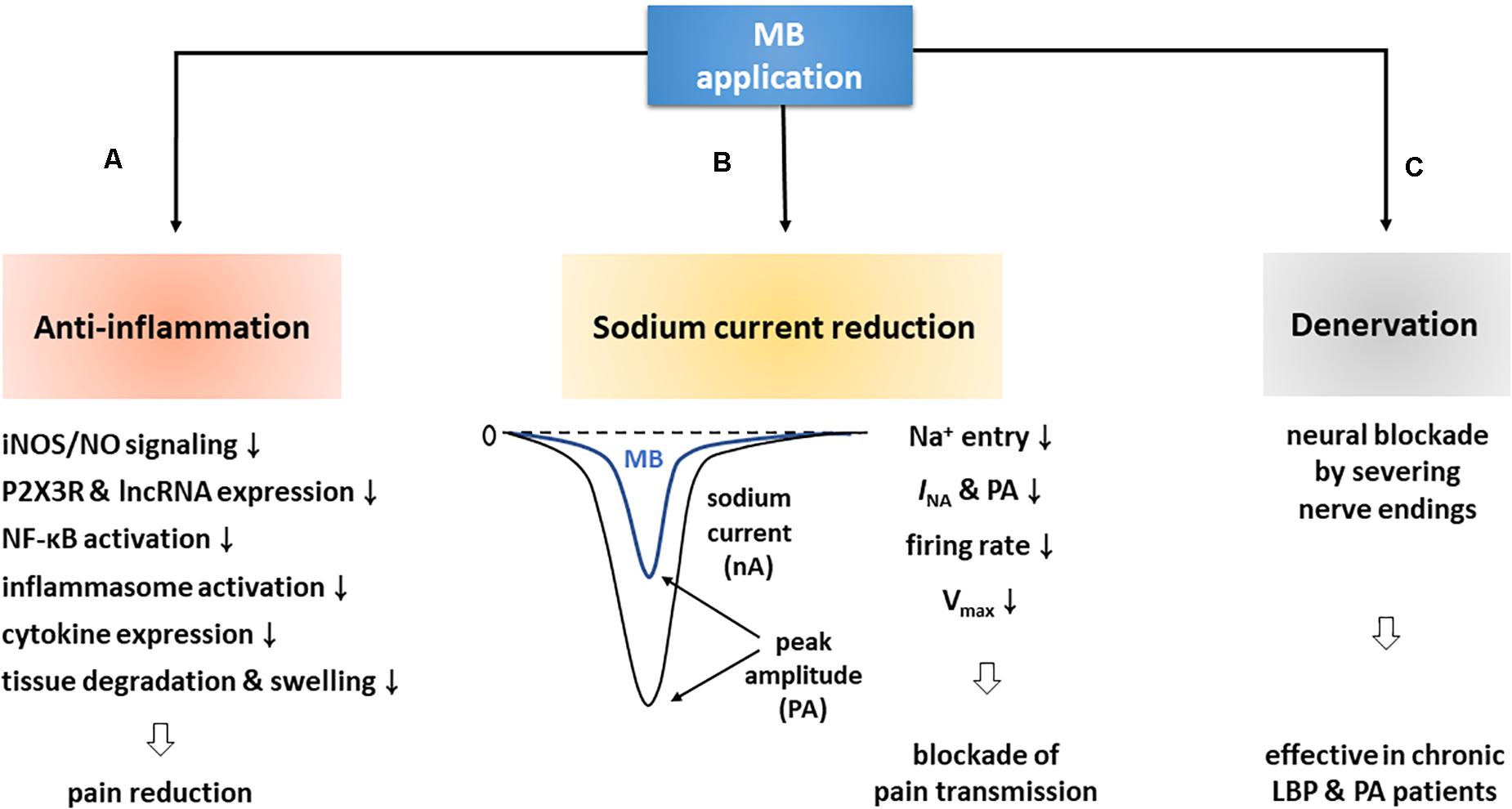
Figure 3. MB contributes to pain reduction via three major routes. (A) First of all, MB is deeply involved in anti-inflammation. MB application blocks iNOS/NO signaling by downregulating iNOS, and suppresses P2 × 3R and lncRNA expression, NF-κB activation, and inflammasome formation, which thereby decreases inflammatory cytokine levels. These events are ultimately followed by pain reduction with the prevention of tissue degradation and swelling. (B) In addition, MB application attenuates neuronal excitability by decreasing INA and firing rates. These altered electrophysiological properties may contribute to pain relief by blocking synaptic transmission. (C) Lastly, MB application improved chronic PA and LBP. An electron microscopic experiment demonstrated that such efficacy was due to the death of nerve endings.
First of all, MB is deeply related to anti-inflammation. Notably, it triggers a variety of anti-inflammatory pathways and functions to decrease the expression level of pro-inflammatory cytokines in a variety of ways, including inhibition of iNOS/NO signaling, downregulation of P2XR and lncRNA, and interruption of priming and activation steps required for inflammasome complex formation and activation. Ultimately, these anti-inflammatory responses contribute to restoring inflamed tissues and lessening pain (Table 1; Vutskits et al., 2008; Dinc et al., 2015; Ahn et al., 2017; Li et al., 2018; Zheng and Li, 2019). In addition, MB reduces the amplitude of INA, accelerates the INA inactivation, delays the shift from inactivation to recovery state in neurons, and importantly decreases neural firing rates, which can therefore alleviate pain by impeding VGSC-mediated neuronal excitability (Zhang et al., 2010; Lee et al., 2021). Lastly, MB is involved in denervation. MB has been believed to have a neurolytic effect and thus to destroy dermal nerve endings for years. Of note, this effect was confirmed by an electron microscopic experiment (Eusebio et al., 1990; Etter and Myers, 2002). In clinical cases, CDLBP patients were improved for at least 3 or 6 months after intradiscal MB injection and RF denervation (Kim et al., 2012; Maas et al., 2015; Zhang X. et al., 2016; Al-Najjim et al., 2018).
However, there are toxicity problems with MB application. Patients being treated with serotonergic medications are likely to suffer ST after intravenous MB administration (mainly at doses of 5-7.5 mg/kg) due to the synergistic effect of MB and the medications. In addition, MB is able to damage cultured neurons (Vutskits et al., 2008) and NP and AF cells in a dose-dependent manner (Wang X. et al., 2019; Zhang et al., 2019). Therefore, we should find proper dose levels and administration routes to protect MB-induced adverse events.
MB is a blue dye that had been used at first for textile manufacturing. Similarly, at the present day, it is applied as a dye to mark and visualize a certain tissue or region in the clinical and experimental settings. However, astonishingly, MB has been also used in biological and medical studies for a long time. Importantly, this review highlighted the relation between MB and pain reduction and made an effort to inform the relevant mechanisms. MB is engaged in pain reduction via diverse routes, but there is a still lack of scientific evidence. There are still a lot of blanks to be filled in the anti-inflammatory pathways and remain possible links to be uncovered between MB and pain-related receptors.
Modern medicine lies in the positivist tradition, which implies that medicine must be based on a solid foundation and the foundation can be constructed by the faithful observation of life phenomena (Cabanis, 1803). In addition, the certainty of medical facts can be guaranteed by that of experimental science such as experimental certainty and logical certainty. The former can be obtained by senses of observant subjects, the latter by intellectual action of humans (Bouillaud, 1836). With regard to the relation between MB and pain control, a solid foundation has not been established yet, although MB intercalates into critical signal pathways to reduce inflammation and pain. Thus, we need still further investigations to build the foundation and reach the certainty of medical facts.
Lessening pain is one of the salient issues in the medical profession, which may also determine our QoL. A great number of medications have been explored and are now applied to patients to relieve pain. Given the actions of MB in pathological or inflammatory milieus, this review offers a strong possibility that this dye may be developed as a new therapeutic agent for pain patients.
Author Contributions
First of all, HH and SL set up the concept of this study, were involved in its overall design, and wrote this manuscript. Moreover, SL put a lot of effort into acquiring and analyzing the relevant articles and data, and made tables and figures to illustrate more clearly methylene blue-mediated events and mechanisms. Both authors contributed to completing this manuscript.
Funding
This research was supported by Basic Science Research Program through the National Research Foundation of Korea (NRF) funded by the Ministry of Education (grant number: NRF-2019R1I1A1A01063196) and supported by BMI Korea Inc. (grant no: Q1829241). BMI Korea Inc. (http://www.bmikr.co.kr), which funded partially this study, is a biotech company and mainly involved in the research and production of medicines and medical instruments. This company always supports basic medical sciences for universities and other institutes. However, this company has never been involved in the study design, collection, analysis, interpretation of data, the writing of this article, or the decision to submit it for publication.
Conflict of Interest
The authors declare that the research was conducted in the absence of any commercial or financial relationships that could be construed as a potential conflict of interest.
References
Ahn, H., Kang, S. G., Yoon, S. I., Ko, H. J., Kim, P. H., Hong, E. J., et al. (2017). Methylene blue inhibits NLRP3, NLRC4, AIM2, and non-canonical inflammasome activation. Sci. Rep. 7:12409.
Al-Najjim, M., Shah, R., Rahuma, M., and Gabbar, O. A. (2018). Lumbar facet joint injection in treating low back pain: radiofrequency denervation versus SHAM procedure. Systematic review. J. Orthop. 15, 1–8. doi: 10.1016/j.jor.2017.10.001
Armstrong, C. M., and Croop, R. S. (1982). Simulation of Na channel inactivation by thiazine dyes. J Gen Physiol 80, 641–662. doi: 10.1085/jgp.80.5.641
Aubrey, B. J., Kelly, G. L., Janic, A., Herold, M. J., and Strasser, A. (2018). How does p53 induce apoptosis and how does this relate to p53-mediated tumour suppression? Cell Death Differ. 25, 104–113. doi: 10.1038/cdd.2017.169
Bach, K. K., Lindsay, F. W., Berg, L. S., and Howard, R. S. (2004). Prolonged postoperative disorientation after methylene blue infusion during parathyroidectomy. Anesth Analg 99, 1573–1574. doi: 10.1213/01.ane.0000134860.73875.cf
Bennett, D. L., Clark, A. J., Huang, J., Waxman, S. G., and Dib-Hajj, S. D. (2019). The role of voltage-gated sodium channels in pain signaling. Physiol. Rev, 99, 1079–1151. doi: 10.1152/physrev.00052.2017
Bernier, L. P., Ase, A. R., and Seguela, P. (2018). P2X receptor channels in chronic pain pathways. Br. J. Pharmacol. 175, 2219–2230. doi: 10.1111/bph.13957
Bouillaud, J. (1836). Essai sur la Philosophie Médicale et sur les Généralités de la Clinique Médicale. Oxford: Université d’Oxford. De Just Rouvier et E. Le Bouvier.
Chen, J., Ao, L., and Yang, J. (2019). Long non-coding RNAs in diseases related to inflammation and immunity. Ann. Transl. Med. 7:494. doi: 10.21037/atm.2019.08.37
Chen, W., Walwyn, W., Ennes, H. S., Kim, H., Mcroberts, J. A., and Marvizón, J. C. (2014). BDNF released during neuropathic pain potentiates NMDA receptors in primary afferent terminals. Eur. J. Neurosci. 39, 1439–1454. doi: 10.1111/ejn.12516
Chu, Y.-C., Guan, Y., Skinner, J., Raja, S. N., Johns, R. A., and Tao, Y.-X. (2005). Effect of genetic knockout or pharmacologic inhibition of neuronal nitric oxide synthase on complete Freund’s adjuvant-induced persistent pain. Pain 119, 113–123. doi: 10.1016/j.pain.2005.09.024
Cohen, N., Robinson, D., Ben-Ezzer, J., Hemo, Y., Hasharoni, A., Wolmann, Y., et al. (2000). Reduced NO accumulation in arthrotic cartilage by exposure to methylene blue. Acta Orthop. Scand. 71, 630–636. doi: 10.1080/000164700317362299
Demir, I. E., Heinrich, T., Carty, D. G., Saricaoglu, ØC., Klauss, S., Teller, S., et al. (2019). Targeting nNOS ameliorates the severe neuropathic pain due to chronic pancreatitis. EBioMedicine 46, 431–443. doi: 10.1016/j.ebiom.2019.07.055
Deng, M., Huang, H., Ma, Y. G., Zhou, Y., Chen, Q., and Xie, P. (2021). Intradiskal injection of methylene blue for discogenic back pain: a meta-analysis of randomized controlled trials. J. Neurol. Surg. A Cent Eur. Neurosurg. 82, 161–165. doi: 10.1055/s-0040-1721015
Deng, S., Yu, K., Zhang, B., Yao, Y., Wang, Z., Zhang, J., et al. (2015). Toll-like receptor 4 promotes NO synthesis by upregulating GCHI expression under oxidative stress conditions in sheep Monocytes/Macrophages. Oxid. Med. Cell.Longev. 2015:359315.
Dinc, S., Caydere, M., Akgul, G., Yenidogan, E., Hucumenoglu, S., and Rajesh, M. (2015). Methylene Blue inhibits the inflammatory process of the acetic acid-induced colitis in the rat colonic mucosa. Int. Surg. 100, 1364–1374. doi: 10.9738/intsurg-d-15-00118.1
Dubin, A. E., and Patapoutian, A. (2010). Nociceptors: the sensors of the pain pathway. J. Clin. Invest. 120, 3760–3772. doi: 10.1172/jci42843
Etter, L., and Myers, S. A. (2002). Pruritus in systemic disease: mechanisms and management. Dermatol. Clin. 20, 459–472. doi: 10.1016/s0733-8635(02)00011-6
Eusebio, E. B., Graham, J., and Mody, N. (1990). Treatment of intractable pruritus ani. Dis Colon Rectum. 33, 770–772. doi: 10.1007/bf02052324
Floris, G., Cadeddu, R., and Bortolato, M. (2020). “The effects of serotonin degradation on psychopathology: role of monoamine oxidase,” in Handbook of the Behavioral Neurobiology of Serotonin, eds C. P. Müller and K. A. Cunningham (Cambridge, MA: Academic Press), 267–278. doi: 10.1016/b978-0-444-64125-0.00014-1
Funakoshi, A., Tatsuno, K., Shimauchi, T., Fujiyama, T., Ito, T., and Tokura, Y. (2019). Cholecystokinin downregulates psoriatic inflammation by its possible self-regulatory effect on epidermal keratinocytes. J. Immunol. 202:2609. doi: 10.4049/jimmunol.1801426
Gillman, P. K. (2011). CNS toxicity involving methylene blue: the exemplar for understanding and predicting drug interactions that precipitate serotonin toxicity. J. Psychopharmacol. 25, 429–436. doi: 10.1177/0269881109359098
Gupta, G., Radhakrishna, M., Chankowsky, J., and Asenjo, J. F. (2012). Methylene blue in the treatment of discogenic low back pain. Pain Physician 15, 333–338. doi: 10.36076/ppj.2012/15/333
Harth, M., and Nielson, W. R. (2019). Pain and affective distress in arthritis: relationship to immunity and inflammation. Expert Rev. Clin. Immunol. 15, 541–552. doi: 10.1080/1744666x.2019.1573675
Huang, C., Tong, L., Lu, X., Wang, J., Yao, W., Jiang, B., et al. (2015). Methylene blue attenuates iNOS induction through suppression of transcriptional factor binding amid iNOS mRNA transcription. J. Cell Biochem. 116, 1730–1740. doi: 10.1002/jcb.25132
Jiang, M., Wang, H., Liu, Z., Lin, L., Wang, L., Xie, M., et al. (2020). Endoplasmic reticulum stress-dependent activation of iNOS/NO-NF-κB signaling and NLRP3 inflammasome contributes to endothelial inflammation and apoptosis associated with microgravity. FASEB J. 34, 10835–10849. doi: 10.1096/fj.202000734r
Kallewaard, J. W., Wintraecken, V. M., Geurts, J. W., Willems, P. C., Van Santbrink, H., Terwiel, C. T. M., et al. (2019). A multicenter randomized controlled trial on the efficacy of intradiscal methylene blue injection for chronic discogenic low back pain: the IMBI study. Pain 160, 945–953. doi: 10.1097/j.pain.0000000000001475
Kanneganti, T. D. (2015). The inflammasome: firing up innate immunity. Immunol. Rev. 265, 1–5. doi: 10.1111/imr.12297
Kapadia, K., Cheung, F., Lee, W., Thalappillil, R., Florence, F. B., and Kim, J. (2016). Methylene blue causing serotonin syndrome following cystocele repair. Urol. Case Rep. 9, 15–17. doi: 10.1016/j.eucr.2016.07.012
Kaski, S. W., White, A. N., Gross, J. D., and Siderovski, D. P. (2021). Potential for kappa-opioid receptor agonists to engineer nonaddictive analgesics: a narrative review. Anesth. Anal. 132, 406–419. doi: 10.1213/ane.0000000000005309
Keppel Hesselink, J. M. (2020). Rediscovery of ceruletide, a CCK agonist, as an analgesic drug. J. Pain Res. 13, 123–130. doi: 10.2147/jpr.s232714
Khan, M. A., North, A. P., and Chadwick, D. R. (2007). Prolonged postoperative altered mental status after methylene blue infusion during parathyroidectomy: a case report and review of the literature. Ann. R. Coll. Surg. Engl. 89, W9–W11.
Kim, J. H., Kim, D. H., and Lee, Y. P. (2019). Long-term follow-up of intradermal injection of methylene blue for intractable, idiopathic pruritus ani. Tech. Coloproctol. 23, 143–149. doi: 10.1007/s10151-019-01934-x
Kim, K. H., Kim, J. I., Han, J. A., Choe, M. A., and Ahn, J. H. (2011). Upregulation of neuronal nitric oxide synthase in the periphery promotes pain hypersensitivity after peripheral nerve injury. Neuroscience 190, 367–378. doi: 10.1016/j.neuroscience.2011.05.064
Kim, S. H., Ahn, S. H., Cho, Y. W., and Lee, D. G. (2012). Effect of intradiscal methylene blue injection for the chronic discogenic low back pain: one year prospective follow-up study. Ann. Rehabil. Med. 36, 657–664. doi: 10.5535/arm.2012.36.5.657
Kõks, S., Fernandes, C., Kurrikoff, K., Vasar, E., and Schalkwyk, L. C. (2008). Gene expression profiling reveals upregulation of Tlr4 receptors in Cckb receptor deficient mice. Behav. Brain Res. 188, 62–70. doi: 10.1016/j.bbr.2007.10.020
Kourosh-Arami, M., Hosseini, N., Mohsenzadegan, M., Komaki, A., and Joghataei, M. T. (2020). Neurophysiologic implications of neuronal nitric oxide synthase. Rev. Neurosci. 31, 617–636. doi: 10.1515/revneuro-2019-0111
Kruger, L. C., and Isom, L. L. (2016). Voltage-Gated Na+ Channels: not just for conduction. Cold Spring Harbor Perspect. Biol. 8:a029264. doi: 10.1101/cshperspect.a029264
Lacagnina, M. J., Watkins, L. R., and Grace, P. M. (2018). Toll-like receptors and their role in persistent pain. Pharmacol. Ther. 184, 145–158. doi: 10.1016/j.pharmthera.2017.10.006
Leclaire, R., Fortin, L., Lambert, R., Bergeron, Y. M., and Rossignol, M. (2001). Radiofrequency facet joint denervation in the treatment of low back pain: a placebo-controlled clinical trial to assess efficacy. Spine 26, 1411–1416. discussion 1417, doi: 10.1097/00007632-200107010-00003
Lee, S. W., Moon, S. W., Park, J. S., Suh, H. R., and Han, H. C. (2021). Methylene blue induces an analgesic effect by significantly decreasing neural firing rates and improves pain behaviors in rats. Biochem. Biophys. Res. Commun. 541, 36–42. doi: 10.1016/j.bbrc.2021.01.008
Leiper, J., and Nandi, M. (2011). The therapeutic potential of targeting endogenous inhibitors of nitric oxide synthesis. Nat. Rev. Drug Discov. 10, 277–291. doi: 10.1038/nrd3358
Levi, D. S., Horn, S., and Walko, E. (2014). Intradiskal methylene blue treatment for diskogenic low back pain. PM R 6, 1030–1037. doi: 10.1016/j.pmrj.2014.04.008
Levine, R., and Richeimer, S. H. (2011). Spinal methylene blue is hazardous. PAIN 152, 952–953. doi: 10.1016/j.pain.2011.01.009
Lewin, M. R., and Walters, E. T. (1999). Cyclic GMP pathway is critical for inducing long–term sensitization of nociceptive sensory neurons. Nat. Neurosci. 2, 18–23. doi: 10.1038/4520
Li, G., Jiang, H., Zheng, C., Zhu, G., Xu, Y., Sheng, X., et al. (2017). Long noncoding RNA MRAK009713 is a novel regulator of neuropathic pain in rats. PAIN 158, 2042–2052. doi: 10.1097/j.pain.0000000000001013
Li, H., Liu, S., Wang, Z., Zhang, Y., and Wang, K. (2020). Hydrogen sulfide attenuates diabetic neuropathic pain through NO/cGMP/PKG pathway and μ-opioid receptor. Exp. Biol. Med. 245, 823–834. doi: 10.1177/1535370220918193
Li, X., Tang, C., Wang, J., Guo, P., Wang, C., Wang, Y., et al. (2018). Methylene blue relieves the development of osteoarthritis by upregulating lncRNA MEG3. Exp. Ther. Med. 15, 3856–3864.
Lin, Z. H., Wang, S. Y., Chen, L. L., Zhuang, J. Y., Ke, Q. F., Xiao, D. R., et al. (2017). Methylene blue mitigates acute neuroinflammation after spinal cord injury through inhibiting nlrp3 inflammasome activation in microglia. Front. Cell Neurosci. 11:391. doi: 10.3389/fncel.2017.00391
Lundberg, J. O., Weitzberg, E., and Gladwin, M. T. (2008). The nitrate-nitrite-nitric oxide pathway in physiology and therapeutics. Nat. Rev. Drug Discov. 7, 156–167. doi: 10.1038/nrd2466
Luo, Z. D., and Cizkova, D. (2000). The role of nitric oxide in nociception. Curr. Rev. Pain 4, 459–466. doi: 10.1007/s11916-000-0070-y
Maas, E. T., Ostelo, R. W., Niemisto, L., Jousimaa, J., Hurri, H., Malmivaara, A., et al. (2015). Radiofrequency denervation for chronic low back pain. Cochrane Database Syst. Rev. CD008572.
Machelska, H., Labuz, D., Przewłocki, R., and Przewłocka, B. (1997). Inhibition of nitric oxide synthase enhances antinociception mediated by mu, delta and kappa opioid receptors in acute and prolonged pain in the rat spinal cord. J. Pharmacol. Exp. Ther. 282, 977–984.
Majithia, A., and Stearns, M. P. (2006). Methylene blue toxicity following infusion to localize parathyroid adenoma. J. Laryngol. Otol. 120, 138–140. doi: 10.1017/s0022215105005098
Martindale, S. J., and Stedeford, J. C. (2003). Neurological sequelae following methylene blue injection for parathyroidectomy. Anaesthesia 58, 1041–1042. doi: 10.1046/j.1365-2044.2003.03415_23.x
Mathew, S., Linhartova, L., and Raghuraman, G. (2006). Hyperpyrexia and prolonged postoperative disorientation following methylene blue infusion during parathyroidectomy. Anaesthesia 61, 580–583. doi: 10.1111/j.1365-2044.2006.04619.x
Matsuda, M., Huh, Y., and Ji, R. R. (2019). Roles of inflammation, neurogenic inflammation, and neuroinflammation in pain. J. Anesth. 33, 131–139. doi: 10.1007/s00540-018-2579-4
MHRA, (2009). Methylthioninium Chloride (methylene blue): Update on Central Nervous System (CNS) Toxicity. London: Medicines and Healthcare products Regulatory Agency, 2.
Miclescu, A. A., Svahn, M., and Gordh, T. E. (2015). Evaluation of the protein biomarkers and the analgesic response to systemic methylene blue in patients with refractory neuropathic pain: a double-blind, controlled study. J. Pain Res. 8, 387–397. doi: 10.2147/jpr.s84685
Mihai, R., Mitchell, E. W., and Warwick, J. (2007). Dose-response and postoperative confusion following methylene blue infusion during parathyroidectomy. Can. J. Anaesth. 54, 79–81. doi: 10.1007/bf03021907
Mukherjee, P., Cinelli, M. A., Kang, S., and Silverman, R. B. (2014). Development of nitric oxide synthase inhibitors for neurodegeneration and neuropathic pain. Chem. Soc. Rev. 43, 6814–6838. doi: 10.1039/c3cs60467e
Nakazawa, H., Chang, K., Shinozaki, S., Yasukawa, T., Ishimaru, K., Yasuhara, S., et al. (2017). iNOS as a driver of inflammation and apoptosis in mouse skeletal muscle after burn injury: possible involvement of sirt1 s-nitrosylation-mediated acetylation of p65 NF-κB and p53. PLoS One 12:e0170391. doi: 10.1371/journal.pone.0170391
Nath, S., Nath, C. A., and Pettersson, K. (2008). Percutaneous lumbar zygapophysial (Facet) joint neurotomy using radiofrequency current, in the management of chronic low back pain: a randomized double-blind trial. Spine 33, 1291–1297. doi: 10.1097/brs.0b013e31817329f0
Ng, B. K. W., and Cameron, A. J. D. (2010). The role of methylene blue in serotonin syndrome: a systematic review. Psychosomatics 51, 194–200. doi: 10.1016/s0033-3182(10)70685-x
Ng, B. K. W., Cameron, A. J. D., Liang, R., and Rahman, H. (2008). Serotonin syndrome following methylene blue infusion during parathyroidectomy: a case report and literature review. Can. J. Anesth. 55, 36–41. doi: 10.1007/bf03017595
Osmanlıoğlu, H. Ø, Yıldırım, M. K., Akyuva, Y., Yıldızhan, K., and Nazıroğlu, M. (2020). Morphine induces apoptosis, inflammation, and mitochondrial oxidative stress via activation of TRPM2 Channel and nitric oxide signaling pathways in the hippocampus. Mol. Neurobiol. 57, 3376–3389. doi: 10.1007/s12035-020-01975-6
Pall, M. L. (2002). NMDA sensitization and stimulation by peroxynitrite, nitric oxide, and organic solvents as the mechanism of chemical sensitivity in multiple chemical sensitivity. Faseb J. 16, 1407–1417. doi: 10.1096/fj.01-0861hyp
Pan, P. F., Wang, Y., Li, X. P., Yang, C. B., Zhong, H., Du, X. X., et al. (2019). Effects of methylene blue on the nitric oxide-soluble guanylate cyclase-cyclic guanylyl monophosphate pathway and cytokine levels in rats with sepsis. Int. J. Clin. Exp. Med. 12, 12203–12211.
Peng, B., Pang, X., Wu, Y., Zhao, C., and Song, X. (2010). A randomized placebo-controlled trial of intradiscal methylene blue injection for the treatment of chronic discogenic low back pain. Pain 149, 124–129. doi: 10.1016/j.pain.2010.01.021
Peng, B., Zhang, Y., Hou, S., Wu, W., and Fu, X. (2007). Intradiscal methylene blue injection for the treatment of chronic discogenic low back pain. Eur. Spine J. 16, 33–38. doi: 10.1007/s00586-006-0076-1
Peter, C., Hongwan, D., Küpfer, A., and Lauterburg, B. H. (2000). Pharmacokinetics and organ distribution of intravenous and oral methylene blue. Eur. J. Clin. Pharmacol. 56, 247–250. doi: 10.1007/s002280000124
Pollack, G., Pollack, A., Delfiner, J., and Fernandez, J. (2009). Parathyroid surgery and methylene blue: a review with guidelines for safe intraoperative use. Laryngosc. 119, 1941–1946. doi: 10.1002/lary.20581
Polydefkis, M., Hauer, P., Sheth, S., Sirdofsky, M., Griffin, J. W., and Mcarthur, J. C. (2004). The time course of epidermal nerve fibre regeneration: studies in normal controls and in people with diabetes, with and without neuropathy. Brain 127, 1606–1615. doi: 10.1093/brain/awh175
Quirion, B., Bergeron, F., Blais, V., and Gendron, L. (2020). The delta-opioid receptor; a target for the treatment of pain. Front. Mol. Neurosci. 13:52. doi: 10.3389/fnmol.2020.00052
Rathinam, V. A., and Fitzgerald, K. A. (2016). Inflammasome complexes: emerging mechanisms and effector functions. Cell 165, 792–800. doi: 10.1016/j.cell.2016.03.046
Rey-Funes, M., Larrayoz, I. M., Fernández, J. C., Contartese, D. S., Rolón, F., Inserra, P. I. F., et al. (2016). Methylene blue prevents retinal damage in an experimental model of ischemic proliferative retinopathy. Am. J. Physiol. Regul. Integr. Comp. Physiol. 310, R1011–R1019.
Roca-Lapirot, O., Fossat, P., Ma, S., Egron, K., Trigilio, G., López-González, M. J., et al. (2019). Acquisition of analgesic properties by the cholecystokinin (CCK)/CCK2 receptor system within the amygdala in a persistent inflammatory pain condition. Pain 160, 345–357. doi: 10.1097/j.pain.0000000000001408
Rocha, P. A., Ferreira, A. F. B., Da Silva, J. T., Alves, A. S., Martins, D. O., Britto, L. R. G., et al. (2020). Effects of selective inhibition of nNOS and iNOS on neuropathic pain in rats. Mol. Cell. Neurosci. 105:103497. doi: 10.1016/j.mcn.2020.103497
Roldan, C. J., Nouri, K., Chai, T., and Huh, B. (2017). Methylene blue for the treatment of intractable pain associated with oral mucositis. Pain Pract. 17, 1115–1121. doi: 10.1111/papr.12566
Rowley, M., Riutort, K., Shapiro, D., Casler, J., Festic, E., and Freeman, W. D. (2009). Methylene blue-associated serotonin syndrome: a ‘Green’ encephalopathy after parathyroidectomy. Neurocrit. Care 11, 88–93. doi: 10.1007/s12028-009-9206-z
Saia, R. S., Ribeiro, A. B., and Giusti, H. (2020). Cholecystokinin modulates the mucosal inflammatory response and prevents the lipopolysaccharide-induced intestinal epithelial barrier dysfunction. Shock 53, 242–251. doi: 10.1097/shk.0000000000001355
Schiltenwolf, M., Fischer, C., and Kunz, P. (2011). How perfect studies may be? Comment on Peng et al. A randomized placebo-controlled trial of intradiscal methylene blue injection for the treatment of chronic discogenic low back pain. Pain 2010;149:124-9. Pain 152:954. author reply 954-955, doi: 10.1016/j.pain.2011.01.008
Schirmer, R. H., Coulibaly, B., Stich, A., Scheiwein, M., Merkle, H., Eubel, J., et al. (2003). Methylene blue as an antimalarial agent. Redox Rep. 8, 272–275. doi: 10.1179/135100003225002899
Schirmer, R. H., Adler, H., Pickhardt, M., and Mandelkow, E. (2011). Lest we forget you–methylene blue. Neurobiol. Aging 32:2325.e7–16. doi: 10.1016/j.neurobiolaging.2010.12.012
Schmid, R., and Evans, R. J. (2019). ATP-Gated P2X receptor channels: molecular insights into functional roles. Annu. Rev. Physiol. 81, 43–62. doi: 10.1146/annurev-physiol-020518-114259
Schwiebert, C., Irving, C., and Gillman, P. K. (2009). Small doses of methylene blue, previously considered safe, can precipitate serotonin toxicity. Anaesthesia 64, 924–924. doi: 10.1111/j.1365-2044.2009.06029.x
Shanmugam, G., Kent, B., Alsaiwadi, T., and Baskett, R. (2008). Serotonin syndrome following cardiac surgery. Interact. Cardiovasc. Thorac. Surg. 7, 656–657. doi: 10.1510/icvts.2007.173104
Starkus, J. G., Heggeness, S. T., and Rayner, M. D. (1984). Kinetic analysis of sodium channel block by internal methylene blue in pronased crayfish giant axons. Biophys. J. 46, 205–218. doi: 10.1016/s0006-3495(84)84014-x
Starkus, J. G., Rayner, M. D., Fleig, A., and Ruben, P. C. (1993). Fast and slow inactivation of sodium channels: effects of photodynamic modification by methylene blue. Biophys. J. 65, 715–726. doi: 10.1016/s0006-3495(93)81098-1
Stephan, G., Huang, L., Tang, Y., Vilotti, S., Fabbretti, E., Yu, Y., et al. (2018). The ASIC3/P2X3 cognate receptor is a pain-relevant and ligand-gated cationic channel. Nat. Commun. 9:1354.
Sun, J., Chen, S.-R., and Pan, H.-L. (2020). μ-Opioid receptors in primary sensory neurons are involved in supraspinal opioid analgesia. Brain Res. 1729:146623. doi: 10.1016/j.brainres.2019.146623
Sung, Y.-J., Sofoluke, N., Nkamany, M., Deng, S., Xie, Y., Greenwood, J., et al. (2017). A novel inhibitor of active protein kinase G attenuates chronic inflammatory and osteoarthritic pain. Pain 158, 822–832. doi: 10.1097/j.pain.0000000000000832
Surprenant, A., and North, R. A. (2009). Signaling at purinergic P2X receptors. Annu. Rev. Physiol. 71, 333–359. doi: 10.1146/annurev.physiol.70.113006.100630
Swanson, K. V., Deng, M., and Ting, J. P. (2019). The NLRP3 inflammasome: molecular activation and regulation to therapeutics. Nat. Rev. Immunol. 19, 477–489. doi: 10.1038/s41577-019-0165-0
Tanga, F. Y., Nutile-Mcmenemy, N., and Deleo, J. A. (2005). The CNS role of Toll-like receptor 4 in innate neuroimmunity and painful neuropathy. Proc. Natl. Acad. Sci. U.S.A. 102, 5856–5861. doi: 10.1073/pnas.0501634102
Top, W. M., Gillman, P. K., De Langen, C. J., and Kooy, A. (2014). Fatal methylene blue associated serotonin toxicity. Neth. J. Med. 72, 179–181.
Torres-López, J. E., Juárez-Rojop, I. E., Granados-Soto, V., Diaz-Zagoya, J. C., Flores-Murrieta, F. J., Ortíz-López, J. U., et al. (2007). Peripheral participation of cholecystokinin in the morphine-induced peripheral antinociceptive effect in non-diabetic and diabetic rats. Neuropharmacology 52, 788–795. doi: 10.1016/j.neuropharm.2006.09.015
Vutskits, L., Briner, A., Klauser, P., Gascon, E., Dayer, A. G., Kiss, J. Z., et al. (2008). Adverse effects of methylene blue on the central nervous system. Anesthesiology 108, 684–692. doi: 10.1097/aln.0b013e3181684be4
Wainwright, M., and Crossley, K. B. (2002). Methylene Blue–a therapeutic dye for all seasons? J. Chemother. 14, 431–443. doi: 10.1179/joc.2002.14.5.431
Wang, F., Ma, S.-B., Tian, Z.-C., Cui, Y.-T., Cong, X.-Y., Wu, W.-B., et al. (2021). Nociceptor-localized cGMP-dependent protein kinase I is a critical generator for central sensitization and neuropathic pain. PAIN 162, 135–151. doi: 10.1097/j.pain.0000000000002013
Wang, J., Ou, S.-W., and Wang, Y.-J. (2017). Distribution and function of voltage-gated sodium channels in the nervous system. Channels 11, 534–554. doi: 10.1080/19336950.2017.1380758
Wang, R., Ma, C., Han, Y., Tan, M., and Lu, L. (2019). Effectiveness of denervation therapy on pain and joint function for patients with refractory knee osteoarthritis: a systematic review and meta-analysis. Pain Physician 22, 341–352. doi: 10.36076/ppj/2019.22.341
Wang, X., Zhang, S., Xie, Z., Chen, L., Yang, B., and Wu, X. (2019). deleterious effects of methylene blue on rat nucleus pulposus cell in vitro: changes in cell viability and secretory phenotype in exposed cells. J. Neurol. Surg. A Cent. Eur. Neurosurg. 80, 174–179. doi: 10.1055/s-0038-1670638
Wolin, M. S., Cherry, P. D., Rodenburg, J. M., Messina, E. J., and Kaley, G. (1990). Methylene blue inhibits vasodilation of skeletal muscle arterioles to acetylcholine and nitric oxide via the extracellular generation of superoxide anion. J. Pharmacol. Exp. Ther. 254, 872–876.
Wolvetang, T., Janse, R., and Ter Horst, M. (2016). Serotonin syndrome after methylene blue administration during cardiac surgery: a case report and review. J. Cardiothorac. Vasc. Anesth. 30, 1042–1045. doi: 10.1053/j.jvca.2015.11.019
Yadav, S., and Surolia, A. (2019). Lysozyme elicits pain during nerve injury by neuronal Toll-like receptor 4 activation and has therapeutic potential in neuropathic pain. Sci. Transl. Med. 11:eaav4176. doi: 10.1126/scitranslmed.aav4176
Yang, Y., Li, Q., He, Q.-H., Han, J.-S., Su, L., and Wan, Y. (2018). Heteromerization of μ-opioid receptor and cholecystokinin B receptor through the third transmembrane domain of the μ-opioid receptor contributes to the anti-opioid effects of cholecystokinin octapeptide. Exp. Mol. Med. 50, 1–16. doi: 10.1038/s12276-018-0090-5
Yang, Y., Wang, H., Kouadir, M., Song, H., and Shi, F. (2019). Recent advances in the mechanisms of NLRP3 inflammasome activation and its inhibitors. Cell Death Dis. 10:128.
Ye, Y., Wang, Y., Yang, Y., and Tao, L. (2020). Aloperine suppresses LPS-induced macrophage activation through inhibiting the TLR4/NF-κB pathway. Inflammation Res. 69, 375–383. doi: 10.1007/s00011-019-01313-0
Yeung, A. W. K., Georgieva, M. G., Atanasov, A. G., and Tzvetkov, N. T. (2019). Monoamine oxidases (MAOs) as privileged molecular targets in neuroscience: research literature analysis. Front. Mol. Neurosci. 12:143. doi: 10.3389/fnmol.2019.00143
Zhang, H., Li, F., Li, W. W., Stary, C., Clark, J. D., Xu, S., et al. (2016). The inflammasome as a target for pain therapy. Br. J. Anaesth. 117, 693–707. doi: 10.1093/bja/aew376
Zhang, X., Hao, J., Hu, Z., and Yang, H. (2016). Clinical evaluation and magnetic resonance imaging assessment of intradiscal methylene blue injection for the treatment of discogenic low back pain. Pain Physician 19, E1189–E1195.
Zhang, L., Liu, Y., Huang, Z., Nan, L., Wang, F., Zhou, S., et al. (2019). Toxicity effects of methylene blue on rat intervertebral disc annulus fibrosus cells. Pain Physician 22, 155–164. doi: 10.36076/ppj/2019.22.155
Zhang, Y., Zhao, J., Zhang, T., and Yang, Z. (2010). In vitro assessment of the effect of methylene blue on voltage-gated sodium channels and action potentials in rat hippocampal CA1 pyramidal neurons. Neurotoxicology 31, 724–729. doi: 10.1016/j.neuro.2010.07.001
Zhao, X., Tang, Z., Zhang, H., Atianjoh, F. E., Zhao, J.-Y., Liang, L., et al. (2013). A long noncoding RNA contributes to neuropathic pain by silencing Kcna2 in primary afferent neurons. Nat. Neurosci. 16, 1024–1031. doi: 10.1038/nn.3438
Zheng, J., and Li, Q. (2019). Methylene blue regulates inflammatory response in osteoarthritis by noncoding long chain RNA CILinc02. J. Cell Biochem. 120, 3331–3338. doi: 10.1002/jcb.27602
Keywords: methylene blue, pain, anti-inflammation, sodium current, denervation
Citation: Lee SW and Han HC (2021) Methylene Blue Application to Lessen Pain: Its Analgesic Effect and Mechanism. Front. Neurosci. 15:663650. doi: 10.3389/fnins.2021.663650
Received: 03 February 2021; Accepted: 16 April 2021;
Published: 17 May 2021.
Edited by:
Claudia Bregonzio, CCT CONICET Córdoba, ArgentinaReviewed by:
Cristiane Salum, Federal University of ABC, BrazilSulev Kõks, University of Tartu, Estonia
Copyright © 2021 Lee and Han. This is an open-access article distributed under the terms of the Creative Commons Attribution License (CC BY). The use, distribution or reproduction in other forums is permitted, provided the original author(s) and the copyright owner(s) are credited and that the original publication in this journal is cited, in accordance with accepted academic practice. No use, distribution or reproduction is permitted which does not comply with these terms.
*Correspondence: Hee Chul Han, aGVlaGFuQGtvcmVhLmFjLmty