- 1Department of Radiology, Beijing Children’s Hospital, Capital Medical University, National Center for Children’s Health, Beijing, China
- 2Key Laboratory of Cognition and Personality, Ministry of Education, Chongqing, China
- 3School of Psychology, Southwest University, Chongqing, China
- 4Department of Otolaryngology, Head and Neck Surgery, Children’s Hospital Capital Institute of Pediatrics, Beijing, China
- 5Department of Otolaryngology, Head and Neck Surgery, Beijing Children’s Hospital, Capital Medical University, National Center for Children’s Health, Beijing, China
Childhood obstructive sleep apnea (OSA) is a common chronic sleep-related breathing disorder in children, which leads to growth retardation, neurocognitive impairments, and serious complications. Considering the previous studies about brain structural abnormalities in OSA, in the present study, we aimed to explore the altered spontaneous brain activity among OSA patients, using amplitude of low-frequency fluctuation (ALFF), fractional ALFF (fALFF), and regional homogeneity (ReHo) methods based on resting-state functional magnetic resonance imaging (MRI). Thirty-one untreated OSA children and 33 age-and gender-matched healthy children (HC) were included in this study. Compared with controls, the OSA group showed significant lower ALFF in the right lingual gyrus, decreased fALFF in the left middle frontal gyrus (MFG), but increased fALFF in the left precuneus. Decreased ReHo was found in the left inferior frontal gyrus (orbital part) and left middle frontal gyrus. Notably, the mean fALFF value of left MFG was not only significantly related to multiple sleep parameters but also demonstrated the best performance in ROC curve analysis. These findings revealed OSA children were associated with dysfunctions in the default mode network, the frontal lobe, and the lingual gyrus, which may implicate the underlying neurophysiological mechanisms of intrinsic brain activity. The correlation between the altered spontaneous neuronal activity and the clinical index provides early useful diagnostic biomarkers for OSA children as well.
Introduction
Obstructive sleep apnea (OSA) is a common chronic sleep-related breathing disorder characterized by partial or complete closure of the upper airway during sleep, which results in recurrent intermittent hypoxia, carbon dioxide retention, and frequent awakening (Chen et al., 2016; Stevens et al., 2020). Nowadays, prevalence of OSA increased strikingly with the increasing population age and obesity. The total prevalence of OSA among children ranges from 1 to 4% (Kirk et al., 2017). Clinically, pediatric OSA always presents with loud and irregular snoring and sleep disorders, in contrast to adults, with a range of different symptoms including growth retardation, enuresis, and behavioral problems, such as attention deficit/hyperactivity (ADHD) disorders (Krysta et al., 2017). Besides, noteworthy neurocognitive impairments also occur in pediatric OSA, involving learning, memory, executive function, visuospatial function, and psychomotor development (Copes and Rosentswieg, 1972; Hamasaki et al., 2007; Krysta et al., 2017), which severely diminished academic performance, social adaptation, and even the quality of life in children. Nevertheless, compared with adults, the scholars have paid little attention to brain abnormalities of pediatric OSA before.
Several previous studies exhibited that cortical thinning or gray matter volume reductions occurred in the superior frontal, ventral medial prefrontal, the superior and lateral parietal cortices, the superior temporal lobe, and the brainstem in OSA children, which arose from delayed neuronal development, damage, or atrophy (Philby et al., 2017; Macey et al., 2018). However, unlike in adults, pediatric OSA observed cortical thickening, including the precentral gyrus, the mid-to-posterior insular cortices, and the right anterior insula cortex in OSA children, which might involve in hypoxia-induced inflammatory changes (Macey et al., 2018). Furthermore, entropy measures based on high-resolution T1-weighted imaging identified early damages of brain tissue integrity in pediatric OSA. The affected brain sites included the prefrontal cortex; corpus callosum; and insular, frontal, temporal, hippocampus, and cerebellar areas, which localized within regulated autonomic, respiratory, and cognitive functions (Kheirandish-Gozal et al., 2018). These findings indicated that pediatric OSA presented extensive injury to the brain structures, which might account for underlying executive and cognitive deficits in children.
Currently, resting-state functional magnetic resonance imaging (rs-fMRI) has been found to be a useful and non-invasive technique for detecting spontaneous neural activity. Using rs-fMRI, several studies have demonstrated that OSA patients have shown noteworthy resting-state functional connectivity (rsFC) deficits, especially in the default mode network (DMN), which plays a role in sustaining brain function in the resting-state (Prilipko et al., 2011; Chen et al., 2018). Zhang et al. (2013) reported that OSA patients showed significantly reduced rsFC within the anterior DMN and bilateral fronto-parietal network but increased rsFC between the posterior cingulate cortex and precuneus within the DMN. Similarly, decreased rsFC was observed in DMN subregions, including the medial prefrontal cortex, anterior cingulate, and posterior cingulate in patients with OSA (Chen et al., 2016). Li et al. (2016) found that patients with OSA displayed a dysfunction of rsFC between the right hippocampus formation and posterior cingulate cortex within the DMN and significant negative correlation with delayed memory. In addition, the frontal lobe as a crucial brain area involved in multiple cognitive functions has been increasingly reported. Yu H. et al. (2019) showed that OSA patients showed significantly increased rsFC between the left dorsal amygdala, the right ventrolateral amygdala, and the left inferior frontal gyrus. Regarding these findings, OSA may be related to the abnormal rsFC between distinct brain areas in DMN, fronto-parietal, and limbic system, whereas which area is more responsible for the observed abnormal connectivity was still unclear. It is meaningful to directly locate the abnormal regional spontaneous neural activity in OSA patients during resting state and their relationships with behavioral performances.
Amplitude of low-frequency fluctuation (ALFF), fractional ALFF (fALFF), and regional homogeneity (ReHo) are three major data-driven measures for quantification of spontaneous neural activity based on BOLD signals. ALFF detects the total power within the range between 0.01 and 0.10 Hz and positively correlates to the alterations of spontaneous neural activity (Zang et al., 2007; Zou et al., 2008). Moreover, fALFF measures the ratio of the specific power spectrum of low frequency to that of the total power in the entire frequency range (Qiu et al., 2019). Both ALFF and fALFF have been proven to exhibit greater test–retest reliability, especially in gray matter (Zuo et al., 2010). Notably, fALFF produces better effects in reducing the physiological noise than ALFF, and it can effectively suppress artifacts in non-specific brain regions, such as the ventricles and the vicinity of blood vessels (Zou et al., 2008). ReHo is a data-driven measure for the local measurement of spontaneous neural activity (Xia et al., 2018), and it can effectively evaluate resting-state brain activity based on the hypothesis that brain activity is more likely to occur in clusters rather than in a single voxel (Zhang et al., 2012). Recently, these methods have wide access to explore brain diseases with potential functional alterations, such as depression (Yu Y. et al., 2019), Alzheimer’s disease (Cheng et al., 2019), Tourette syndrome (Liu et al., 2017), and so on. Accordingly, the combination of the three may provide more detailed information about the intrinsic activity than each method alone.
In the present study, we not only investigated abnormal intensity of neural activity via ALFF/fALFF analysis but also investigated abnormal neural synchronization via ReHo analysis in OSA children. Based on previous studies, we hypothesized that (1) OSA children would show altered ALFF/fALFF and ReHo values in the DMN, frontal lobe, and lingual gyrus; (2) the alterations of the spontaneous brain activity would be related to sleep-related respiratory parameters in OSA children; and (3) abnormal spontaneous activity pattern might be utilized as diagnostic neuroimaging biomarkers to discriminate OSA from controls. We aim to take a crucial step towards identifying spontaneous brain activity abnormalities in OSA children and providing potential targets for better understanding and treatment of this neurologic and sleep dysfunction disorder.
Materials and Methods
Subjects
Thirty-one OSA children (age: 5.65 ± 2.82 years, range: 3–10 years; 12 female) were recruited in Beijing Children’s Hospital from April 2016 to October 2019. We also included 33 age- (p = 0.585, two-sample t-test) and gender-matched (p = 0.431, chi-square test) healthy children (age: 6.01 ± 2.43 years; range: 2–11 years; 16 female) in our study (Table 1). All the participants were right-handed. The exclusion criteria included (1) history of brain structural injury, neurological or psychiatric disorders; (2) suffering from cardiovascular diseases, neuromuscular diseases, or defined genetic syndromes; (3) abnormal blood pressure, blood fat, and glucose; (4) being with any known acute or chronic illness; and (5) undergone treatment with drugs and surgery. Before the scan, children under 7 years old needed to take chloral hydrate for sedation. The dosage was 0.5 ml/kg and the maximum dose was 10 ml. This study was approved by the Medical Ethics Committee of Beijing Children’s Hospital, Beijing, China. The study was carried out in line with relevant guidelines by the Medical Ethics Committee of Beijing Children’s Hospital, which include MRI scan and clinical diagnosis.
Polysomnography
All subjects underwent a polysomnography (PSG) evaluation (Compumedics E; Compumedics, Melbourne, Australia; or ALICE 5; Philips Respironics, Amsterdam, Netherlands), which recorded a polysomnogram of more than 7.5 h. Simultaneous monitoring was included for EEG, bilateral electro-oculogram, electromyogram of mentalis activity and bilateral anterior tibialis, ECG, arterial oxyhemoglobin saturation and plethysmographic signal by pulse oximetry, heat-sensitive airflow and nasal pressure, chest and abdominal movements, snoring sensor, body position, and other indicators. All sleep monitoring results were scored manually by experienced professional pediatric PSG technicians according to the diagnostic criteria published by the American Academy of Sleep Medicine (AASM) (Berry et al., 2012). The criteria for the OSA group diagnosis consisted of obstructive apnea (OAI) > 1 times/h or apnea–hypopnea index (AHI) > 5 times/h and lowest oxygen saturation (LSaO2) < 92% by PSG.
Image Acquisition
Magnetic resonance imaging scanning was performed on a 3-T MR scanner (GE Medical Systems, Discovery MR750). Before the scan, all participants should keep respiration and heart rate in a normal state. All participants were required to be awake and quietly breathing until the end of the scan. The scanner parameters for fMRI data are TR/TE = 2000/24 ms, 240 time points, image matrix = 64 × 64, voxel size = 3.5 mm × 3.5 mm × 3.5 mm, field of view (FOV) = 224 mm × 224 mm. The scanner parameters for T1-weighted images are TR/TE = 8.19/3.78 ms, voxel size = 0.4688 mm × 0.4688 mm × 1 mm, matrix = 512 × 512, FOV = 240 mm × 240 mm. During MRI scanning, participants were asked to close their eyes and lie still in the scanner. Head positioning was standardized using canthomeatal landmarks. The head was stabilized with foam pads to minimize its movement.
Data Preprocessing
Resting-state fMRI data reprocessing was performed using the statistical parametric mapping (SPM8) and Data Processing & Analysis for Resting-state Brain Imaging (DPABI Version 2.1)1. The first 10 image volumes of functional images were removed for the signal equilibrium and subject’s adaptation to the scanning noise. Then, the functional images were corrected for time offsets between slices and geometrical displacements due to head motion. We further calculated the mean frame-wise displacement (FD) to measure voxel-wise differences in motion in its derivation (Jenkinson et al., 2002). None of the participants were excluded based on the excluding criteria of 3.0 mm and 3.0 degree in max head motion, with mean FD > 0.2 mm. The T1-weighted images were co-registered to the average functional images and then segmented into the white matter (WM), gray matter (GM), and cerebrospinal fluid (CSF) by using the New Segment tool in DPABI. We removed linear trends and regressed out several nuisance signals from each voxel’s time course, including 24-parameter head-motion profiles (Friston et al., 1996; Yan et al., 2013), mean WM, and cerebrospinal fluid (CSF) time series within the respective brain masks derived from prior probability maps in SPM8 (threshold = 0.8). All the corrected functional data were then normalized by DARTEL (Ashburner, 2007) to the Montreal Neurological Institute (MNI) space using an optimum 12-parameter affine transformation and non-linear deformations and then resampled to a 3-mm isotropic resolution.
Measurement of Amplitude of Low-Frequency Fluctuation/Fractional ALFF and Regional Homogeneity
To calculate ALFF, we firstly performed the spatial smoothing on the resampled images with a 4-mm full width at half maximum (FWHM) Gaussian kernel. We then converted the smoothed signal of each voxel from time domain to frequency domain via Fast Fourier Transform (FFT) to obtain the power spectrum. This power spectrum (frequency range: 0–0.25 Hz) was square-rooted at each frequency, and then averaged across 0.01–0.08 Hz at each voxel, which was taken as ALFF (Zang et al., 2007). To calculate fALFF, the sum of the amplitude (square root of power spectrum) across 0.01–0.08 Hz was divided by that of the entire frequency range (0–0.25 Hz) (Zou et al., 2008). ALFF/fALFF of each voxel was divided by the global mean ALFF/fALFF for standardization purpose, and mALFF/mfALFF was obtained as a parameter for further statistical comparison and analysis.
Regional homogeneity maps were generated before spatial smoothing. After normalization, the band-pass filtering (0.01–0.08 Hz) was performed on the normalized images to reduce the effects of low-frequency drift and high-frequency physiological noise. ReHo maps were conducted by calculating the Kendall coefficient of concordance (KCC) as synchronization of fMRI signals of nearest neighboring 27 voxels (Zang et al., 2004). For standardization purposes, the ReHo value of each voxel was divided by the whole brain mean ReHo value, and then smoothing was done with a 4 mm FWHM Gaussian kernel. The smReHo map was obtained as the ReHo parameter for further statistical comparison and analysis.
Receiver Operating Characteristic Curves Analysis
Once significantly altered ALFF/fALFF/ReHo areas were found between groups, they might be utilized as markers to discriminate OSA from controls, as useful diagnostic neuroimaging biomarkers (Li et al., 2015). To test this possibility, the mean ALFF/fALFF/ReHo values of significantly altered brain clusters were extracted and used for analysis of the receiver operating characteristic (ROC) curves, using the MedCalc Statistical Software2. To summarize the overall diagnostic ability of the tests, we computed the maximum Youden index (sensitivity + specificity − 1) (Fluss et al., 2005), and corresponding sensitivity, specificity, and 95% confidence intervals (CIs) for each cluster.
Statistical Analysis
Further statistical analysis was performed based on a 90% group mask (meaning 90% of subjects have this voxel) generated in DPABI toolbox to detect group differences. We conducted a two-sample t-test to compare whole-brain ALFF, ALFF and ReHo values between OSA children and controls, including age, gender, and mean FD (Jenkinson et al., 2002) as covariates. The Gaussian random field (GRF) correction (Bansal and Peterson, 2018) was used to correct for multiple comparisons, and the statistical threshold was set at a voxel level of p < 0.001 with a cluster-level p < 0.05 (two-tailed) in DPABI toolbox. All coordinates were reported in MNI space. Brain regions with significant intergroup differences in ALFF/fALFF/ReHo were defined as regions of interest (ROIs). We extracted the mean ALFF/fALFF/ReHo values of these ROIs from OSA children. Partial correlations controlling for age, gender, and FD values were applied in SPSS version 24.0 (SPSS Inc., Chicago, IL, United States) to identify mean ALFF/fALFF/ReHo values related to clinical parameters of OSAS children, using the statistical threshold of p < 0.05.
Results
Demographic and Clinical Characteristic
Demographic and clinical characteristics of each group are summarized in Table 1. The OSA had significantly higher scores for AHI, OAI, HI, SaO2 < 90%, and AI and significantly lower scores for LSaO2 and sleep efficiency than controls. No significant differences were found in age, gender, weight, BMI, and mean FD between the two groups. Of note, there is no significant Pearson correlation between mean FD and age for the OSA group (r = 0.197, p = 0.289) and control group (r = 0.204, p = 0.255).
Amplitude of Low-Frequency Fluctuation Results
Compared with controls, the OSA group showed significant lower ALFF in the cluster of the right lingual gyrus (Brodmann area 18). The details are presented in Table 2 and Figure 1.

Table 2. Two-sample t-tests demonstrated regions with significantly decreased ALFF in OSA children compared with controls (with GRF correction, voxel level p < 0.001, cluster level p < 0.05).
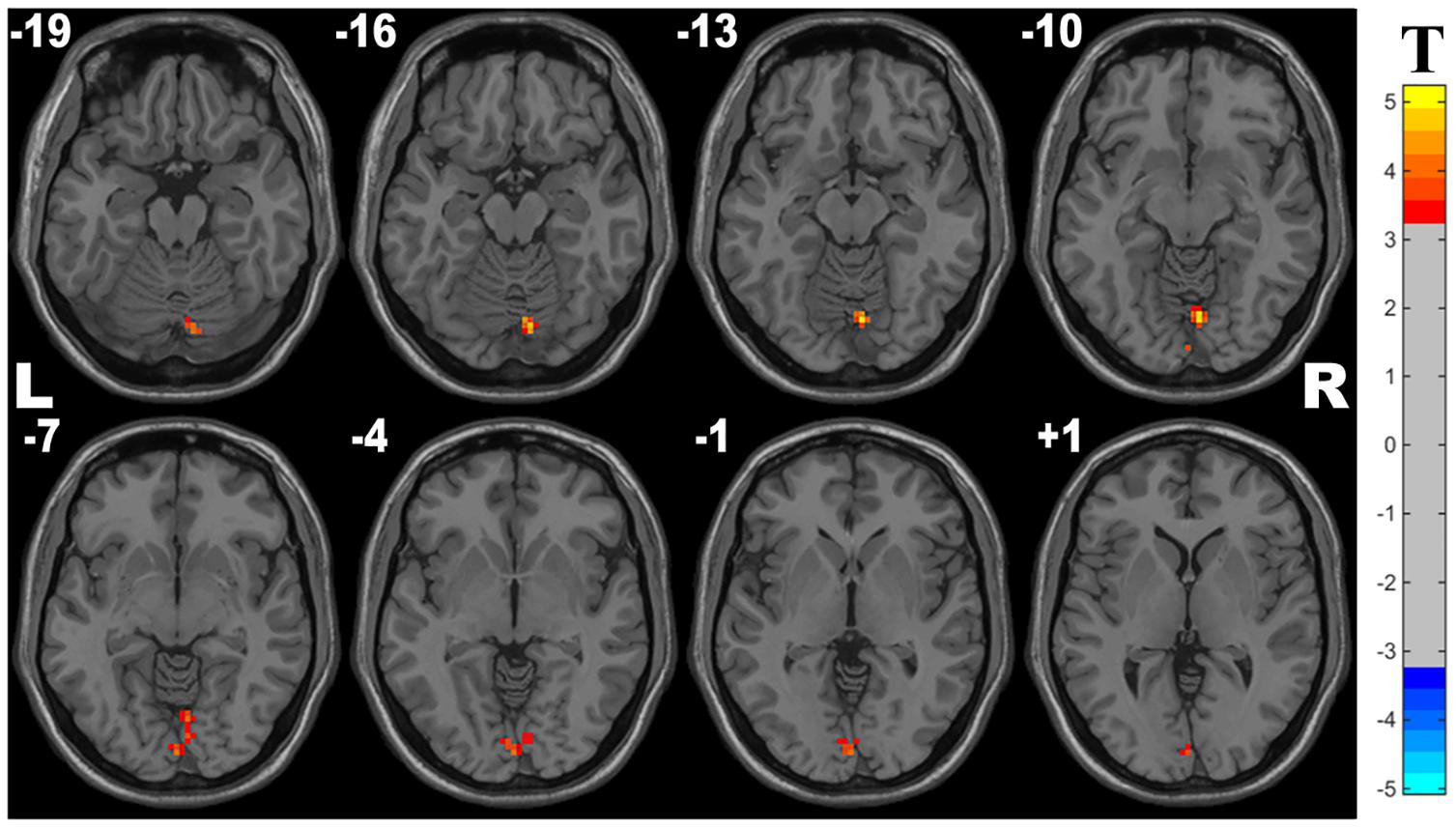
Figure 1. Cluster with significantly decreased ALFF in OSA children compared with controls (with GRF correction, voxel level p < 0.001, cluster level p < 0.05).
Fractional ALFF Results
Compared with controls, the OSA group showed significant lower fALFF in the cluster of the left middle frontal gyrus and higher fALFF in the left precuneus. The details are presented in Table 3 and Figure 2.

Table 3. Two-sample t-tests demonstrated regions with significantly altered fALFF in OSA children compared with controls (with GRF correction, voxel level p < 0.001, cluster level p < 0.05).
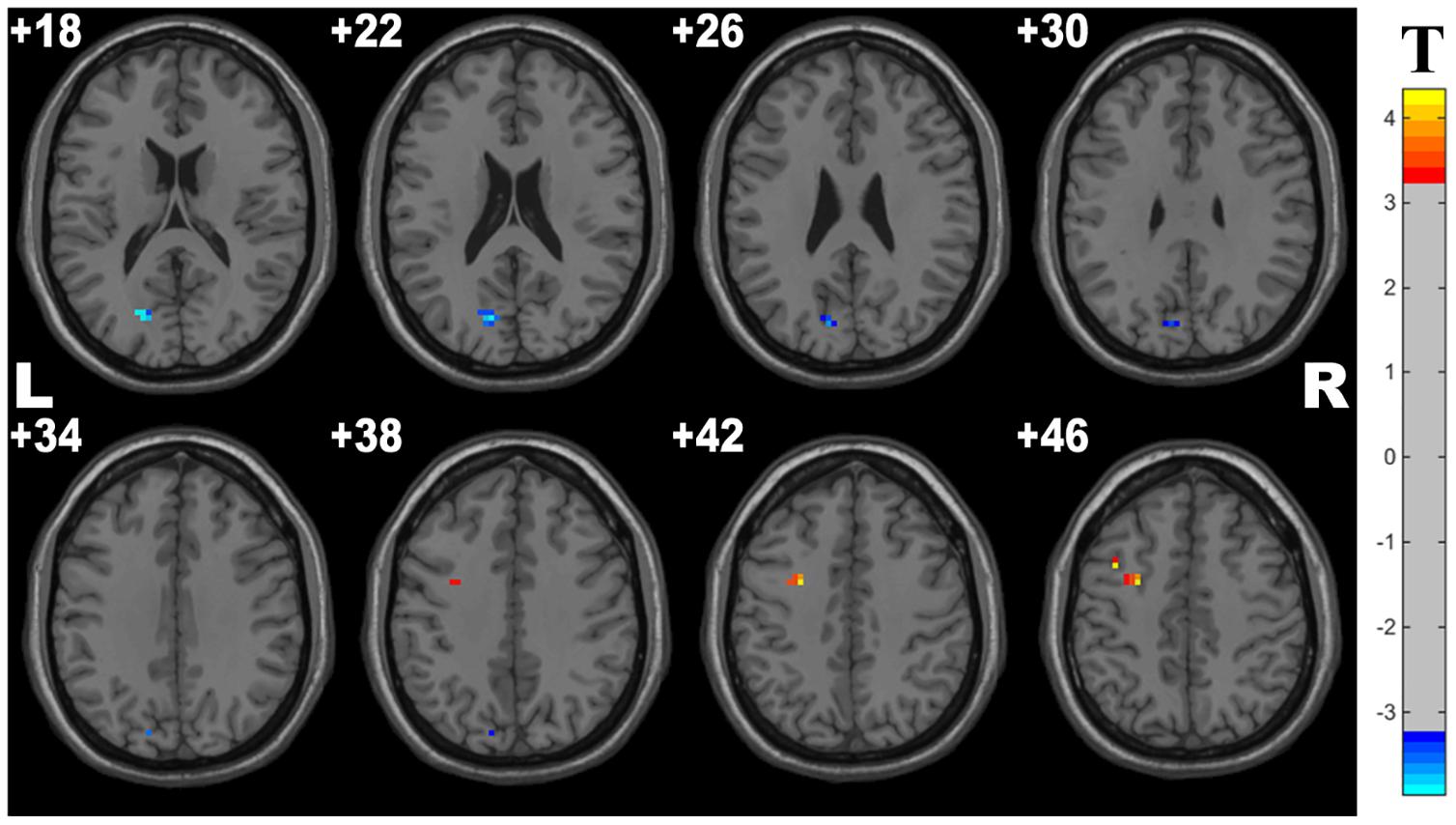
Figure 2. Cluster with significantly altered fALFF in OSA children compared with controls (with GRF correction, voxel level p < 0.001, cluster level p < 0.05). The blue and red areas denote higher and lower fALFF in OSA group.
Regional Homogeneity Results
Compared with controls, OSA group showed significant lower ReHo in the cluster of the left inferior frontal gyrus (orbital part) and left middle frontal gyrus. The details are presented in Table 4 and Figure 3.

Table 4. Two-sample t-tests demonstrated regions with significantly decreased ReHo in OSA children compared with controls (with GRF correction, voxel level p < 0.001, cluster level p < 0.05).
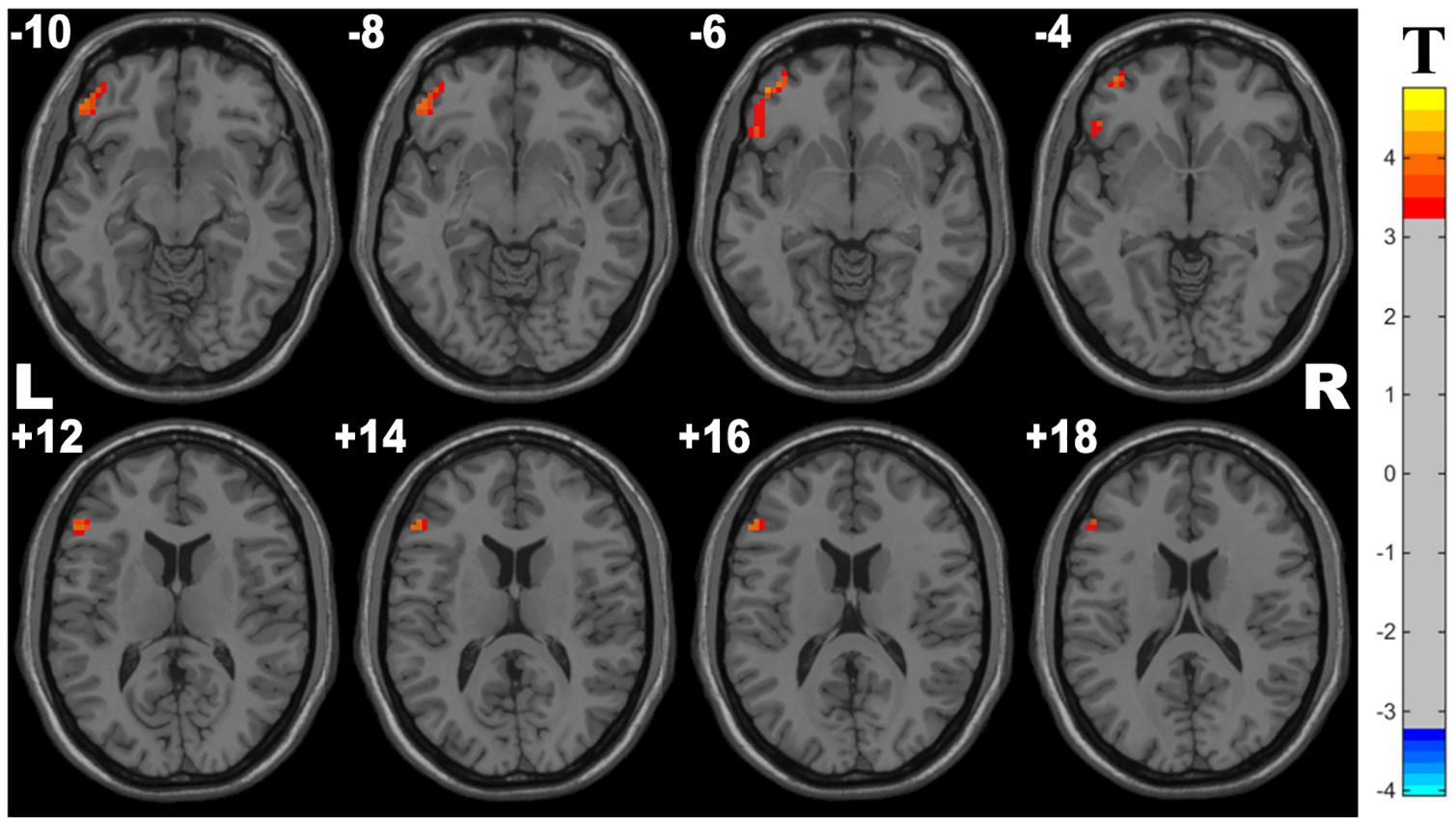
Figure 3. Cluster with significantly decreased ReHo in OSA children compared with controls (with GRF correction, voxel level p < 0.001, cluster level p < 0.05).
Receiver Operating Characteristic Curves Analysis Results
In the ROC curve analysis, the mean fALFF value of left middle frontal gyrus achieves the highest sensitivity, specificity, and area under the ROC curve (AUC). All the altered brain clusters achieve the significance level p < 0.001 of AUC, indicating these findings as potential useful diagnostic biomarkers. The details are presented in Table 5 and Figure 4.
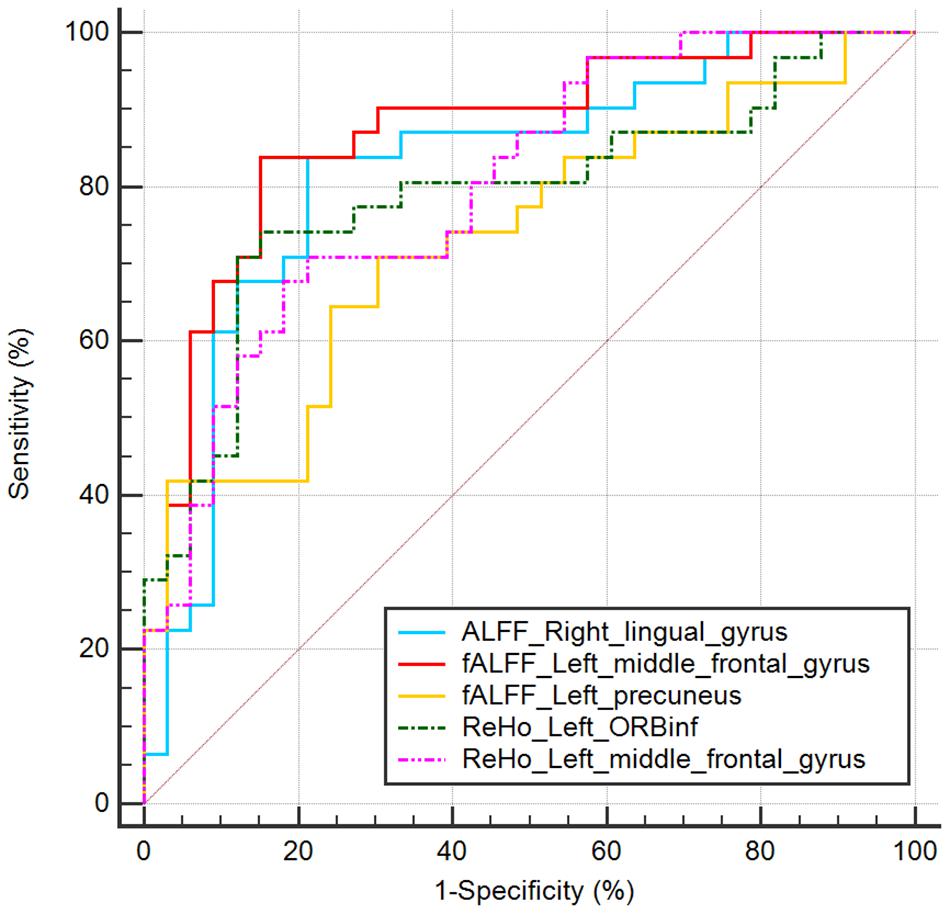
Figure 4. ROC curve analysis of mean ALFF/fALFF/ReHo signal values for altered brain clusters. AUC, area under the curve; ORBinf, inferior frontal gyrus, orbital part.
Correlation Results
In the OSA group, the mean fALFF value in the left middle frontal gyrus showed significantly negative correlations with HI (r = −0.385, p = 0.043), SaO2 < 90% (r = −0.381, p = 0.046), AI (r = −0.391, p = 0.040), and a significantly positive correlation with LSaO2 (r = 0.378, p = 0.047). The mean fALFF value in the left precuneus showed a significantly negative correlation with LSaO2 (r = −0.506, p = 0.006) and a significantly positive correlation with SaO2 < 90% (r = 0.396, p = 0.037) (shown in Figure 5). All the p-values were uncorrected for multiple comparisons.
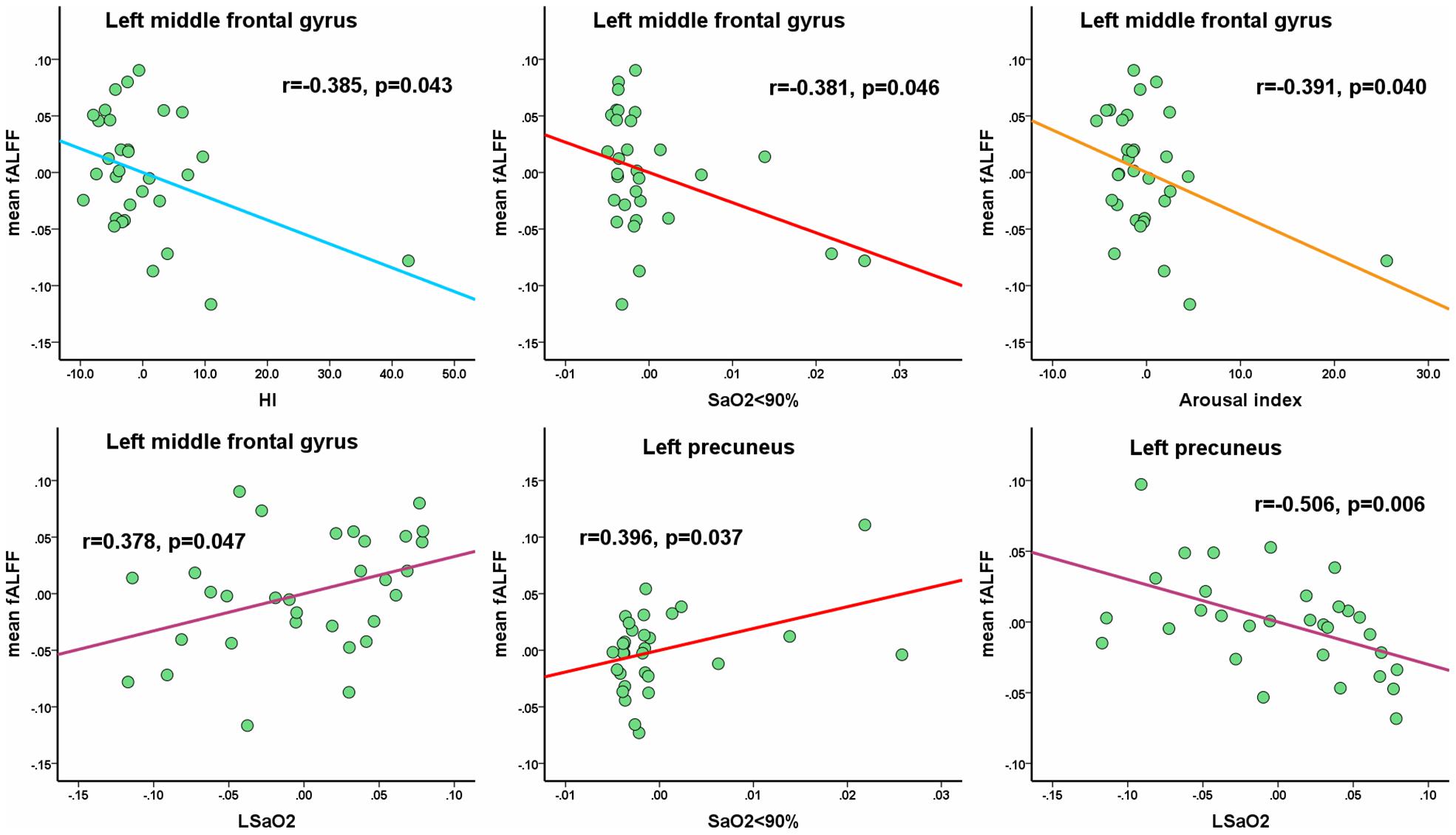
Figure 5. The mean fALFF values in altered clusters show the significantly partial correlations with clinical parameters. Of note, the coordinate value of both X-axis (clinical parameter) and Y-axis (fALFF value) do not reflect the initial values of these variables, while considering age, gender and FD as covariates. All the p-values were uncorrected for multiple comparisons.
Discussion
Amplitude of Low-Frequency Fluctuation/Fractional ALFF and ReHo approaches are used to investigate the intrinsic brain activities in OSA, as effective noninvasive imaging tools. These methods are based on different neurophysiological mechanisms, ALFF/fALFF analysis represents neural intensity, whereas ReHo reflects neural coherence (Liu et al., 2017). Our study is the first to utilize the combination of three methods to detect abnormal neural activities in certain brain regions in pediatric OSA, which contributed to the exhibition of more comprehensive functional alterations. Compared to previous study (Li et al., 2015), we applied more clinical parameters analysis and obtained more outstanding correlations with functional parameters. Moreover, the ROC curve analysis was demonstrated in detail, along with sensitivity and specificity, which provided remarkable guidance for clinical diagnosis. In addition, a large sample size was enrolled with 31 OSA individuals in this study. Taken together, we believe our study contributes to obtain more reliable results for revealing the abnormalities of neural activity in OSA children.
Compared with healthy controls, the OSA group showed significant lower ALFF in the right lingual gyrus (Brodmann area 18). In the OSA group, fALFF was significantly decreased in the left middle frontal gyrus as well as increased in the left precuneus. We also observed that the OSA group showed a significant decrease of ReHo in the left inferior frontal gyrus (orbital part) and left middle frontal gyrus. These results revealed the changes of spontaneous brain activities were closely associated with the DMN, the frontal lobe, and the right lingual gyrus. Furthermore, in the OSA group, the mean fALFF value in the left middle frontal gyrus showed significantly negative correlations with HI, SaO2 < 90%, and AI and a significantly positive correlation with LSaO2. The mean fALFF value in the left precuneus showed a significantly negative correlation with LSaO2 and a significantly positive correlation with SaO2 < 90%. Additionally, in the previous studies (Zang et al., 2007; Zou et al., 2008), scholars have found the ALFF method may be affected by physiological noise irrelevant to brain activity. However, the artifacts from non-specific brain areas (the ventricles and the vicinity of blood vessels) were significantly reduced by the fALFF approach, while signals from cortical regions correlated with brain activity were enhanced, which contributed to the superior sensitivity and specificity in detecting spontaneous brain activities (Zou et al., 2008). In line with previous researches, in our study, we also noted the fALFF approach showed more rewarding results among these approaches. The mean fALFF value not only significantly associated with the sleep parameters but also demonstrated the best performance in ROC curve analysis.
Our study revealed the increase of fALFF in the left precuneus. The precuneus is located in the posteromedial cortex of the parietal lobe and plays a key role in a range of highly integrated tasks, including visuospatial imagery, self-processing operations, and episodic memory retrieval, namely, first-person perspective taking and an experience of agency (Cavanna and Trimble, 2006). Peng et al. (2014) found that patients with OSA showed decreased ReHo in the precuneus, besides the significant negative correlation between altered ReHo in the precuneus with sleep time, suggesting decreased sleep time might be a key factor for dysfunction in the precuneus. An 18F-fluoro-2-deoxy-D-Glucose positron emission tomography study showed a decrease of brain metabolism in the precuneus (Yaouhi et al., 2009). Another functional imaging study displayed lower ALFF in the right precuneus; in addition, a significant positive correlation was found in the right precuneus between MoCA score and the ALFF value, which indicated the abnormalities of the precuneus may be associated with a cognitive dysfunction (Li et al., 2015).
As is well known, the precuneus is a vital node in the default mode networks (DMN), and others include the posterior cingulate cortex; medial prefrontal cortex; and medial, lateral, and inferior parietal regions. These brain regions are highly interconnected to form DMN, a large-scale network, which is thought to be involved in an array of advanced cognitive functions, such as visuospatial imagery, consciousness, attention, adaptation, episodic memory, executive cognitive control, and behavioral inhibition (Andrews-Hanna et al., 2010; Wilson et al., 2010). As similarly reported in the past, abnormal inactivation in the DMN among patients with OSA was found during working memory tasks and also significantly positively correlated with behavioral performance, which may imply that the inhibition of activity in the DMN plays a role in cognitive impairment (Prilipko et al., 2011). Specifically, reduced functional connectivity is related to cognitive dysfunction in the DMN at rest and the enhanced functional connectivity of the OSA may be a compensatory mechanism for the decrease of cognition (Chen et al., 2016). Chen et al. (2018) found that the OSA group showed significantly decreased FC of the anterior–posterior DMN and within the posterior DMN and was associated with the MoCA score, using graph theory approaches. Furthermore, they found abnormal FC within the DMN may contribute to the topological reconfiguration of the DMN in patients with OSA, which illuminated the cognitive dysfunction and topological reconfiguration in OSA. Nevertheless, based on these findings, we found that patients with OSA had increased fALFF in the left precuneus, which may imply an adaptive compensatory response in the DMN. Furthermore, our study also found a significant negative correlation between the altered fALFF in the local region of the DMN and the LSaO2, suggesting that intermittent hypoxia may be a principal element for the DMN abnormity in OSA.
Our study showed fALFF and ReHo were decreased in the left middle frontal gyrus and the left inferior frontal gyrus (orbital part), and left middle frontal gyrus, respectively. To our knowledge, the frontal lobe is the higher cortex of cognitive executive function and also plays an important role in emotion, language, attention, working memory, problem solving, impulse control, and social behavior (Stern et al., 2001; Johnson et al., 2009; Nie et al., 2017). Huang et al. (2019) found both decreased gray matter volume (GMV) and functional response in the orbital frontal cortex (OFC) in patients with OSA, which may indicate that OFC is a vulnerable and sensitive area in the brain. Another study demonstrated that patients with OSA showed significantly lower ReHo in the right medial frontal gyrus and right superior frontal gyrus (Peng et al., 2014). Ji et al. (2021) also found significant lower ReHo in the left medial superior frontal gyrus and was positively associated with VIQ, which reflected that such brain area may play a crucial role in the cognitive processing related to VIQ. The inferior frontal gyrus was attributed to the language function, decision making under risk, and the regulation of cognitive control (Foland-Ross and Gotlib, 2012; Luo et al., 2015). Song et al. (2018) found that the impaired FC between the caudate and inferior frontal gyrus may become the basis of the cognitive regulation defects of emotion, which leads to comorbid mood disorders in OSA.
Consistent with these findings, in our study, we found that patients with OSA showed significantly altered brain activities in the left frontal gyrus when compared with controls. Notably, the mean fALFF value of left middle frontal gyrus achieves the highest sensitivity, specificity, and AUC value in ROC curve analysis. Also, significant correlations were found between the mean fALFF values of the left middle frontal gyrus with the more clinical parameters, suggesting the middle frontal gyrus might be a sensitive region in the brain among OSA children, which might turn into the potential useful diagnostic biomarkers.
In the present study, we also observed significant lower ALFF in the right lingual gyrus among patients with OSA. The lingual gyrus of the occipital lobe is located between the calcarine sulcus and the posterior portion of the collateral sulcus and then extends to the tentorial surface of the temporal lobe and joins the hippocampus (Joo et al., 2007). A study by Luo et al. (2015) found that the OSA showed a tendency of decreased degree in the right lingual gyrus, as a topological alteration in and regional properties in patients with OSA. Our study is also consistent with their results of different modalities. Besides, Joo et al. (2007) found that the cerebral blood flow of parahippocampal and lingual gyrus were reduced in OSA during wakefulness, using the 99mTc-ethyl cysteinate dimer (ECD) single photon emission computed tomography method, which partly indicated memory impairment and spatial learning deficits in patients with severe OSA. The lingual gyrus has been believed to be involved in visual recognition and episodic memory consolidation (Kukolja et al., 2016) and are considered to play a role in the process of generating and recalling dreams as well (Bischof and Bassetti, 2004). Therefore, the decreased metrics of lingual gyrus possibly account for certain deficits in visual memory and learning.
Limitations
Several limitations deserve to be mentioned in the present study. Firstly, our study obtained a larger sample size compared with previous neuroimaging researches in pediatric OSA (Luo et al., 2015; Ji et al., 2021). However, the sample size was still relatively small, which implies an urgent need for expanding data to validate the results of future studies. Secondly, the p-values of the correlations between network properties and clinical measurements are not corrected for multiple comparisons in this study, as this is currently a preliminary exploratory research. We will reveal the correlations corrected for multiple comparisons based on more subjects in future research, which obtains the more reliable mechanisms of brain activity in OSA children. Thirdly, the previous study found that the test–retest reliability of PerAF is better than ALFF and fALFF, and the test–retest reliability between machines is better (Zhao et al., 2018). We will investigate this using perAF to measure the spontaneous brain activity of OSA children in our future study.
Conclusion
We have investigated the alterations of spontaneous neural activity in OSA, based on the ALFF/fALFF and ReHo approaches on rs-fMRI data. Abnormal regions with the altered neural activity in OSA children include the precuneus, the middle and inferior frontal gyrus, and the lingual gyrus. Moreover, we also found that the altered fALFF in the precuneus was negatively correlated with the LSaO2 in OSA. These results expounded the underlying neurophysiological mechanisms of altered spontaneous brain activity and revealed the correlation between the changes of sleep function and functional activity in brain.
Data Availability Statement
The original contributions presented in the study are included in the article/supplementary material, further inquiries can be directed to the corresponding author/s.
Ethics Statement
The studies involving human participants were reviewed and approved by the Medical Ethics Committee of Beijing Children’s Hospital, Beijing, China. Written informed consent to participate in this study was provided by the participants’ legal guardian/next of kin.
Author Contributions
JB: study concept and design, investigation, acquisition of data, analysis and interpretation of data, and drafting of the original manuscript. HW: study concept and design, methodology, software, analysis and interpretation of data, and writing – review and editing. JT: collect clinical information and access cognitive function. YP and XN: study supervision and project administration. HL, LM, TJ, and XL: collect clinical information and access cognitive function. YaL: acquisition of data. YuL: study concept and design, and writing – review and editing. All authors contributed to the article and approved the submitted version.
Funding
This work was supported by the National Natural Science Foundation of China (32100902 and 82000991), the Fundamental Research Funds for the Central Universities (SWU118065), and the Beijing Hospitals Authority Youth Programme (code: QML20201206).
Conflict of Interest
The authors declare that the research was conducted in the absence of any commercial or financial relationships that could be construed as a potential conflict of interest.
Publisher’s Note
All claims expressed in this article are solely those of the authors and do not necessarily represent those of their affiliated organizations, or those of the publisher, the editors and the reviewers. Any product that may be evaluated in this article, or claim that may be made by its manufacturer, is not guaranteed or endorsed by the publisher.
Acknowledgments
The authors would like to thank all the subjects and their families for the time and effort they dedicated to our research and all the experts who helped to complete this research.
Footnotes
References
Andrews-Hanna, J. R., Reidler, J. S., Sepulcre, J., Poulin, R., and Buckner, R. L. (2010). Functional-anatomic fractionation of the brain’s default network. Neuron 65, 550–562. doi: 10.1016/j.neuron.2010.02.005
Ashburner, J. (2007). A fast diffeomorphic image registration algorithm. Neuroimage 38, 95–113. doi: 10.1016/j.neuroimage.2007.07.007
Bansal, R., and Peterson, B. S. (2018). Cluster-level statistical inference in fMRI datasets: the unexpected behavior of random fields in high dimensions. Magn. Reson. Imaging 49, 101–115. doi: 10.1016/j.mri.2018.01.004
Berry, R. B., Budhiraja, R., Gottlieb, D. J., Gozal, D., Iber, C., Kapur, V. K., et al. (2012). Rules for scoring respiratory events in sleep: update of the 2007 AASM manual for the scoring of sleep and associated events. Deliberations of the sleep apnea definitions task force of the American Academy of Sleep Medicine. J. Clin. Sleep Med. 8, 597–619. doi: 10.5664/jcsm.2172
Bischof, M., and Bassetti, C. L. (2004). Total dream loss: a distinct neuropsychological dysfunction after bilateral PCA stroke. Ann. Neurol. 56, 583–586. doi: 10.1002/ana.20246
Cavanna, A. E., and Trimble, M. R. (2006). The precuneus: a review of its functional anatomy and behavioural correlates. Brain 129, 564–583. doi: 10.1093/brain/awl004
Chen, L., Fan, X., Li, H., Ye, C., Yu, H., Gong, H., et al. (2018). Topological reorganization of the default mode network in severe male obstructive sleep apnea. Front. Neurol. 9:363. doi: 10.3389/fneur.2018.00363
Chen, T., Yang, M., Liu, B., Liu, Y. T., Zhang, H. X., Liu, C. C., et al. (2016). The resting-state functional connectivity of the default mode networks in patients with obstructive sleep apnea-hypopnea syndrome. CNS Neurol. Disord. Drug Targets. doi: 10.2174/187152731601170111222000
Cheng, J., Yang, H., and Zhang, J. (2019). Donepezil’s effects on brain functions of patients with Alzheimer disease: a regional homogeneity study based on resting-state functional magnetic resonance imaging. Clin. Neuropharmacol. 42, 42–48. doi: 10.1097/WNF.0000000000000324
Copes, K., and Rosentswieg, J. (1972). The effects of sleep deprivation upon motor performance of ninth-grade students. J. Sports Med. Phys. Fitness 12, 47–53.
Fluss, R., Faraggi, D., and Reiser, B. (2005). Estimation of the Youden Index and its associated cutoff point. Biom. J. 47, 458–472. doi: 10.1002/bimj.200410135
Foland-Ross, L. C., and Gotlib, I. H. (2012). Cognitive and neural aspects of information processing in major depressive disorder: an integrative perspective. Front. Psychol. 3:489. doi: 10.3389/fpsyg.2012.00489
Friston, K. J., Williams, S., Howard, R., Frackowiak, R. S., and Turner, R. (1996). Movement-related effects in fMRI time-series. Magn. Reson. Med. 35, 346–355. doi: 10.1002/mrm.1910350312
Hamasaki, U. S., Nagata, P. S., Fujita, R. R., Moreira, G. A., Pradella-Hallinan, M., and Weckx, L. (2007). Assessment of cognitive learning function in children with obstructive sleep breathing disorders. Braz. J. Otorhinolaryngol. 73, 315–320. doi: 10.1016/S1808-8694(15)30074-4
Huang, X., Tang, S., Lyu, X., Yang, C., and Chen, X. (2019). Structural and functional brain alterations in obstructive sleep apnea: a multimodal meta-analysis. Sleep Med. 54, 195–204. doi: 10.1016/j.sleep.2018.09.025
Jenkinson, M., Bannister, P., Brady, M., and Smith, S. (2002). Improved optimization for the robust and accurate linear registration and motion correction of brain images. Neuroimage 17, 825–841. doi: 10.1006/nimg.2002.1132
Ji, T., Li, X., Chen, J., Ren, X., Mei, L., Qiu, Y., et al. (2021). Brain function in children with obstructive sleep apnea: a resting-state fMRI study. Sleep 44:zsab047. doi: 10.1093/sleep/zsab047
Johnson, S. B., Blum, R. W., and Giedd, J. N. (2009). Adolescent maturity and the brain: the promise and pitfalls of neuroscience research in adolescent health policy. J. Adolesc. Health 45, 216–221. doi: 10.1016/j.jadohealth.2009.05.016
Joo, E. Y., Tae, W. S., Han, S. J., Cho, J. W., and Hong, S. B. (2007). Reduced cerebral blood flow during wakefulness in obstructive sleep apnea-hypopnea syndrome. Sleep 30, 1515–1520. doi: 10.1093/sleep/30.11.1515
Kheirandish-Gozal, L., Sahib, A. K., Macey, P. M., Philby, M. F., Gozal, D., and Kumar, R. (2018). Regional brain tissue integrity in pediatric obstructive sleep apnea. Neurosci. Lett. 682, 118–123. doi: 10.1016/j.neulet.2018.06.002
Kirk, V., Baughn, J., D’Andrea, L., Friedman, N., Galion, A., Garetz, S., et al. (2017). American academy of sleep medicine position paper for the use of a home sleep apnea test for the diagnosis of OSA in children. J. Clin. Sleep Med. 13, 1199–1203. doi: 10.5664/jcsm.6772
Krysta, K., Bratek, A., Zawada, K., and Stepanczak, R. (2017). Cognitive deficits in adults with obstructive sleep apnea compared to children and adolescents. J. Neural Transm. (Vienna) 124, 187–201. doi: 10.1007/s00702-015-1501-6
Kukolja, J., Goreci, D. Y., Onur, O. A., Riedl, V., and Fink, G. R. (2016). Resting-state fMRI evidence for early episodic memory consolidation: effects of age. Neurobiol. Aging 45, 197–211. doi: 10.1016/j.neurobiolaging.2016.06.004
Li, H. J., Dai, X. J., Gong, H. H., Nie, X., Zhang, W., and Peng, D. C. (2015). Aberrant spontaneous low-frequency brain activity in male patients with severe obstructive sleep apnea revealed by resting-state functional MRI. Neuropsychiatr. Dis. Treat. 11, 207–214. doi: 10.2147/NDT.S73730
Li, H. J., Nie, X., Gong, H. H., Zhang, W., Nie, S., and Peng, D. C. (2016). Abnormal resting-state functional connectivity within the default mode network subregions in male patients with obstructive sleep apnea. Neuropsychiatr. Dis. Treat. 12, 203–212. doi: 10.2147/NDT.S97449
Liu, Y., Wang, J., Zhang, J., Wen, H., Zhang, Y., Kang, H., et al. (2017). Altered spontaneous brain activity in children with early tourette syndrome: a resting-state fMRI Study. Sci. Rep. 7:4808. doi: 10.1038/s41598-017-04148-z
Luo, Y. G., Wang, D., Liu, K., Weng, J., Guan, Y., Chan, K. C., et al. (2015). Brain structure network analysis in patients with obstructive sleep apnea. PLoS One 10:e139055. doi: 10.1371/journal.pone.0139055
Macey, P. M., Kheirandish-Gozal, L., Prasad, J. P., Ma, R. A., Kumar, R., Philby, M. F., et al. (2018). Altered regional brain cortical thickness in pediatric obstructive sleep apnea. Front. Neurol. 9:4. doi: 10.3389/fneur.2018.00004
Nie, S., Peng, D. C., Gong, H. H., Li, H. J., Chen, L. T., and Ye, C. L. (2017). Resting cerebral blood flow alteration in severe obstructive sleep apnoea: an arterial spin labelling perfusion fMRI study. Sleep Breath. 21, 487–495. doi: 10.1007/s11325-017-1474-9
Peng, D. C., Dai, X. J., Gong, H. H., Li, H. J., Nie, X., and Zhang, W. (2014). Altered intrinsic regional brain activity in male patients with severe obstructive sleep apnea: a resting-state functional magnetic resonance imaging study. Neuropsychiatr. Dis. Treat. 10, 1819–1826. doi: 10.2147/NDT.S67805
Philby, M. F., Macey, P. M., Ma, R. A., Kumar, R., Gozal, D., and Kheirandish-Gozal, L. (2017). Reduced regional grey matter volumes in pediatric obstructive sleep apnea. Sci. Rep. 7:44566. doi: 10.1038/srep44566
Prilipko, O., Huynh, N., Schwartz, S., Tantrakul, V., Kim, J. H., Peralta, A. R., et al. (2011). Task positive and default mode networks during a parametric working memory task in obstructive sleep apnea patients and healthy controls. Sleep 34, 293–301. doi: 10.1093/sleep/34.3.293
Qiu, H., Li, X., Luo, Q., Li, Y., Zhou, X., Cao, H., et al. (2019). Alterations in patients with major depressive disorder before and after electroconvulsive therapy measured by fractional amplitude of low-frequency fluctuations (fALFF). J. Affect. Disord. 244, 92–99. doi: 10.1016/j.jad.2018.10.099
Song, X., Roy, B., Kang, D. W., Aysola, R. S., Macey, P. M., Woo, M. A., et al. (2018). Altered resting-state hippocampal and caudate functional networks in patients with obstructive sleep apnea. Brain Behav. 8:e994. doi: 10.1002/brb3.994
Stern, C. E., Sherman, S. J., Kirchhoff, B. A., and Hasselmo, M. E. (2001). Medial temporal and prefrontal contributions to working memory tasks with novel and familiar stimuli. Hippocampus 11, 337–346. doi: 10.1002/hipo.1048
Stevens, D., Jackson, B., Carberry, J., McLoughlin, J., Barr, C., Mukherjee, S., et al. (2020). The impact of obstructive sleep apnoea on balance, gait and falls risk: a narrative review of the literature. J. Gerontol. A Biol. Sci. Med. Sci. 75, 2450–2460. doi: 10.1093/gerona/glaa014
Wilson, C. R., Gaffan, D., Browning, P. G., and Baxter, M. G. (2010). Functional localization within the prefrontal cortex: missing the forest for the trees? Trends Neurosci. 33, 533–540. doi: 10.1016/j.tins.2010.08.001
Xia, W., Chen, Y. C., Luo, Y., Zhang, D. F., Chen, H., Ma, J., et al. (2018). Decreased spontaneous brain activity and functional connectivity in type 1 diabetic patients without microvascular complications. Cell. Physiol. Biochem. 51, 2694–2703. doi: 10.1159/000495960
Yan, C. G., Cheung, B., Kelly, C., Colcombe, S., Craddock, R. C., Di Martino, A., et al. (2013). A comprehensive assessment of regional variation in the impact of head micromovements on functional connectomics. Neuroimage 76, 183–201. doi: 10.1016/j.neuroimage.2013.03.004
Yaouhi, K., Bertran, F., Clochon, P., Mezenge, F., Denise, P., Foret, J., et al. (2009). A combined neuropsychological and brain imaging study of obstructive sleep apnea. J. Sleep Res. 18, 36–48. doi: 10.1111/j.1365-2869.2008.00705.x
Yu, H., Chen, L., Li, H., Xin, H., Zhang, J., Wei, Z., et al. (2019). Abnormal resting-state functional connectivity of amygdala subregions in patients with obstructive sleep apnea. Neuropsychiatr. Dis. Treat. 15, 977–987. doi: 10.2147/NDT.S191441
Yu, Y., Li, Z., Lin, Y., Yu, J., Peng, G., Zhang, K., et al. (2019). Depression affects intrinsic brain activity in patients with mild cognitive impairment. Front. Neurosci. 13:1333. doi: 10.3389/fnins.2019.01333
Zang, Y., Jiang, T., Lu, Y., He, Y., and Tian, L. (2004). Regional homogeneity approach to fMRI data analysis. Neuroimage 22, 394–400. doi: 10.1016/j.neuroimage.2003.12.030
Zang, Y. F., He, Y., Zhu, C. Z., Cao, Q. J., Sui, M. Q., Liang, M., et al. (2007). Altered baseline brain activity in children with ADHD revealed by resting-state functional MRI. Brain Dev. 29, 83–91. doi: 10.1016/j.braindev.2006.07.002
Zhang, Q., Wang, D., Qin, W., Li, Q., Chen, B., Zhang, Y., et al. (2013). Altered resting-state brain activity in obstructive sleep apnea. Sleep 36, 651–659. doi: 10.5665/sleep.2620
Zhang, Z., Liu, Y., Jiang, T., Zhou, B., An, N., Dai, H., et al. (2012). Altered spontaneous activity in Alzheimer’s disease and mild cognitive impairment revealed by Regional Homogeneity. Neuroimage 59, 1429–1440. doi: 10.1016/j.neuroimage.2011.08.049
Zhao, N., Yuan, L. X., Jia, X. Z., Zhou, X. F., Deng, X. P., He, H. J., et al. (2018). Intra- and inter-scanner reliability of voxel-wise whole-brain analytic metrics for resting state fMRI. Front. Neuroinform. 12:54. doi: 10.3389/fninf.2018.00054
Zou, Q. H., Zhu, C. Z., Yang, Y., Zuo, X. N., Long, X. Y., Cao, Q. J., et al. (2008). An improved approach to detection of amplitude of low-frequency fluctuation (ALFF) for resting-state fMRI: fractional ALFF. J. Neurosci. Methods 172, 137–141. doi: 10.1016/j.jneumeth.2008.04.012
Keywords: obstructive sleep apnea, children, amplitude of low-frequency fluctuation fractional, regional homogeneity, functional magnetic resonance imaging, resting state, spontaneous activity, cognitive impairment
Citation: Bai J, Wen H, Tai J, Peng Y, Li H, Mei L, Ji T, Li X, Li Y, Ni X and Liu Y (2021) Altered Spontaneous Brain Activity Related to Neurologic and Sleep Dysfunction in Children With Obstructive Sleep Apnea Syndrome. Front. Neurosci. 15:595412. doi: 10.3389/fnins.2021.595412
Received: 16 August 2020; Accepted: 27 September 2021;
Published: 15 November 2021.
Edited by:
Tim B. Dyrby, Technical University of Denmark, DenmarkCopyright © 2021 Bai, Wen, Tai, Peng, Li, Mei, Ji, Li, Li, Ni and Liu. This is an open-access article distributed under the terms of the Creative Commons Attribution License (CC BY). The use, distribution or reproduction in other forums is permitted, provided the original author(s) and the copyright owner(s) are credited and that the original publication in this journal is cited, in accordance with accepted academic practice. No use, distribution or reproduction is permitted which does not comply with these terms.
*Correspondence: Yue Liu, bGl1eXVlMjAxMzZAMTYzLmNvbQ==; Xin Ni, bml4aW5AYmNoLmNvbS5jbg==
†These authors have contributed equally to this work and share first authorship