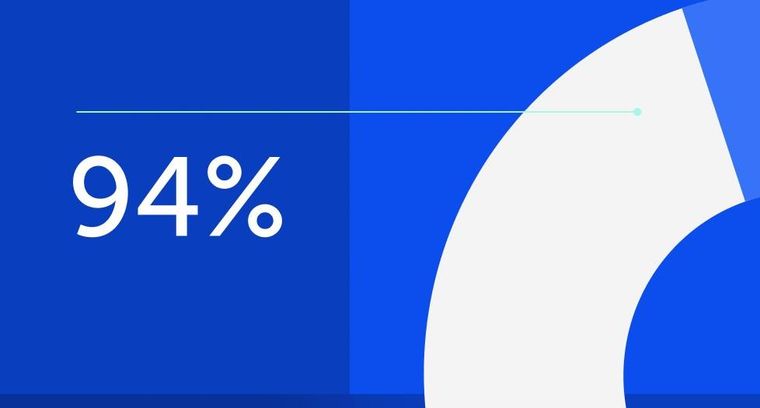
94% of researchers rate our articles as excellent or good
Learn more about the work of our research integrity team to safeguard the quality of each article we publish.
Find out more
ORIGINAL RESEARCH article
Front. Neurosci., 04 February 2021
Sec. Neuroendocrine Science
Volume 14 - 2020 | https://doi.org/10.3389/fnins.2020.583557
Background: Dilated perivascular spaces (dPVS) are considered to be a type of cerebral small vessel disease (CSVD) as well as an important part of the glymphatic system. Although obesity has been shown to play a significant role in the development of CSVD, there are no studies addressing the correlation between obesity and dPVS. We aimed to study the relationship between abdominal fat distribution and dPVS in neurologically healthy cohorts.
Methods: A total of 989 subjects, who were examined during a health examination project, were included in this study. We measured both visceral adipose tissue (VAT) and subcutaneous adipose tissue (SAT) areas using abdominal computed tomography. The dPVS scores were also evaluated in the basal ganglia (BG) and the centrum semiovale (CSO).
Results: In a multivariate ordinal regression analysis, the relationship between VAT area and CSO-dPVS scores remained significant (β [95% confidence interval {CI} = 0.00003395] [0.00001074–0.00005716], P = 0.004), especially in male cohorts (β [95% CI] = 0.00004325 [0.00001772–0.00006878], P = 0.001) after adjusting for age; sex; and glucose, creatinine, uric acid, high-density lipoprotein, and low-density lipoprotein levels, while no association was found between SAT area and dPVS scores. The effects of quartile VAT area on CSO-dPVS were also significant in male cohorts (odds ratio [95% CI] = 1.33 [1.139 – 1.557], P < 0.001).
Conclusion: We demonstrated a positive association between VAT and CSO-dPVS scores in a healthy cohort, which was more prominent in males.
The spaces surrounding small blood vessels in the brain are termed perivascular spaces. These include the periarteriolar, pericapillary, and perivenular spaces (Wardlaw et al., 2020). Perivascular spaces are the most important part of the brain glymphatic drainage system and function as a communication network between cellular fluid and cerebrospinal fluid to remove soluble proteins and other metabolic-waste-containing macromolecules such as glucose, lipids, and amino acids (Tarasoff-Conway et al., 2015; Bacyinski et al., 2017). It has been reported that reduced function of the glymphatic system leads to accumulation of toxic proteins or metabolic waste in the brain and results in a series of inflammatory reactions, leading to the occurrence of diseases such as Parkinson’s and Alzheimer’s diseases (Zou et al., 2019). When compensatory or pathologic enlargement occurs in perivascular spaces of the brain due to an impairment in the clearance of the glymphatic system in such a microenvironment, it manifests as dilated perivascular space (dPVS) and can be detected by both T1- and T2-weighted magnetic resonance imaging (MRI). To date, dPVS is considered a type of cerebral small vessel disease (CSVD), and the pathology of dPVS remains unclear.
Obesity is defined as the accumulation of excess fat and has been shown to play a significant role in the development of cerebrovascular diseases (Keys, 1980; Feinleib, 1985; Kannel, 1985; Wajchenberg, 2000). So far, the effect of obesity on the development of CSVD has been well established. Like white matter hyperintensity, lacunae and microbleeding are also associated with obesity as detected by imaging measurements (Karcher et al., 2013; Yamashiro et al., 2014). In addition, animal studies provide some insight on the potential impact of obesity on the occurrence of CSVD. For example, a previous study (Virdis et al., 2019) suggested that the pathology of endothelial dysfunction caused by obesity might be related to oxidative stress, inflammation, or enzyme-mediated pathways and may later manifest as CSVD. Interestingly, visceral adipose tissue (VAT) and subcutaneous adipose tissue (SAT) have different effects on disease formation (Gil et al., 2011). For example, a study demonstrated that VAT had a significant effect on atherosclerosis in obese patients, while SAT did not (Fantuzzi and Mazzone, 2007). Although numerous studies have been conducted on CSVD and obesity, the relationship between dPVS and obesity is not clear.
In this study, we focused on the relationship between abdominal fat distribution that was measured directly by abdominal computed tomography (CT), and dPVS in healthy cohorts. We also conducted laboratory examinations to predict factors that might lead to the formation of dPVS. We hypothesized that accumulation of adipose tissue might result in the formation of dPVS in one or the other pathological way.
We screened all the subjects (74,414) who participated in the health examination project at The First Affiliated Hospital of Wenzhou Medical University from April 2019 to November 2019. Among these subjects, we first searched for subjects whose examinations included abdominal CT images in the PACS system as well as laboratory examinations on the same day (N = 25,364). Furthermore, we searched for those who had brain MR examination within the following month. Also, we excluded subjects with a lifetime history of either neurological or psychiatric illnesses or a traumatic brain injury, as well as persons with obvious abnormality on their brain MRI (such as malformations and space-occupying lesions, infarcts, hematomas, and heterotopic white matter), leading to a total of 999 persons. Additionally, as MR images of 10 subjects were blurred, they were not included in the study. Finally, we included 989 subjects in this study (Figure 1). Our study was approved by the Medical Ethics Committee of The First Affiliated Hospital of Wenzhou Medical University. Since this was a retrospective cross-sectional study including both males and females, written informed consent for each subject was waived by the Medical Ethics Committee.
All 989 subjects underwent T1- and T2-weighted MRI, abdominal CT, and laboratory examinations for detecting the levels of the following: total protein (TP), urea nitrogen (UN), glucose, creatinine, triglycerides, total cholesterol (TC), high-density lipoprotein (HDL), low-density lipoprotein (LDL), and homocysteine.
The dPVS was defined and assessed using both axial T1- and T2-weighted images according to the Standards for Reporting Vascular Changes on Neuroimaging criteria by two trained neuroradiologists (YQ and YL with 7 and 4 years of experience, respectively) who were blinded to the clinical information. The dPVS had high intensity as seen in T2-weighted images, low intensity in T1-weighted images, and low intensity in fluid-attenuated inversion recovery images. It was necessary to distinguish the lacunar infarcts from dPVS, which are generally oval-shaped lesions greater than 3 mm. According to Doubal et al. (2010), a 4-point visual rating scale (0 = no dPVS, 1 = less than 10 dPVS, 2 = 11–20 dPVS, 3 = 21–40 dPVS, and 4 = greater than 40 dPVS) was used to evaluate dPVS in the centrum semiovale (CSO) area and the basal ganglia (BG). After examining all relevant levels, the score was assigned as per the abovementioned visual rating scale. The two brain hemispheres were evaluated separately, and the higher score was used as the subject’s final dPVS score. The dPVS scores of all the 989 participants were evaluated by the two radiologists. The inter-rater reliability was excellent for BG-dPVS scores (intraclass correlation coefficient = 0.80) and CSO-dPVS scores (intraclass correlation coefficient = 0.85). The dPVS rating scale of a senior radiologist was used for analysis. We obtained broad MR image acquisitions as follows: 1) T1-weighted images (repetition time [TR]/echo time [TE] = 2005/15 ms) and 2) T2-weighted images (TR/TE = 4900/120 ms).
We calculated SAT and VAT using CT images of the abdomen at the horizontal level of the third lumbar vertebra. Based on the specifications by Kim et al. (2017), we identified the tissues within the parietal peritoneum, except the spine and paravertebral muscles, as VAT, and the tissues outside the fascia of the abdominal wall muscles as SAT.
We used post-processing software (version 4.6; GE Healthcare) to set the CT value range from –150 to –40 Hounsfield units, automatically marked the tissue within the CT value range on the image level of the third lumbar vertebra and manually circled the SAT and VAT areas to finally show the output of SAT and VAT areas. Figure 2 shows CT images of the third lumbar vertebral level of a subject and the SAT and VAT area ranges. CT scans were performed using a standard clinical protocol: tube voltage = 120 kV(p), automatically adjusted tube current, and axial section thickness = 5 mm.
Figure 2. The diagram of adipose tissue segment. Blue area represented the selected zone within the CT value range from –150 to –40 Hounsfield units. (A) The blue area represented for the subcutaneous adipose tissue we circled, and 9965.81 mm2 was automatically calculated as the area. (B) The blue area represented for visceral adipose tissue and 10042.93 mm2 was automatically calculated as the area.
First, the data are shown as the mean ± standard deviation of the normally distributed continuous variables (determined by the Kolmogorov–Smirnov test) and the number of binary variables. In addition, the values were presented separately for males and females.
Second, the associations between dPVS scores and possible predictors were analyzed by univariate ordinal regression analysis.
Third, multivariate ordinal regression analysis with dPVS scores as the dependent variable was performed to assess the association with VAT area and VAT/SAT ratio separately, by adjusting for variables with P < 0.05 in univariate linear regression.
Certain previous studies demonstrated that the adipocyte activity appeared to be rather different between males and females (Fox et al., 2004; Porter et al., 2009; Bouchi et al., 2015). To confirm this sexual difference regarding the effects of adipose tissues on dPVS scores, we performed stratified multivariate ordinal regression analysis by sex. The adjusted variables were determined using univariate ordinal regression analysis. On the basis of the results of sex-stratified multivariate ordinal regression analysis, we divided the VAT area into 4 grades according to quartiles (Degree 1: VAT area < 9621.5 mm2; Degree 2: 9621.5 mm2 < VAT area < 14056.5 mm2; Degree 3: 14056 mm2 < VAT area < 18920.5 mm2; Degree 4: VAT area > 18920.5 mm2) to show the correlation clearly.
SPSS version 25 (IBM SPSS, Chicago, IL, United States) was used for all statistical analyses in this study. P < 0.05 was considered statistically significant.
The demographic data of 989 subjects are shown in Table 1. The mean BG-dPVS and CSO-dPVS scores (± SD) were 1.01 ± 0.12 and 1.42 ± 0.666, and the mean areas of SAT and VAT (± SD) were 12177 ± 4914 mm2 and 12043 ± 6910.2 mm2, respectively.
In univariate regression analysis (Table 2), CSO-dPVS scores were significantly associated with age; sex; levels of glucose, creatinine, UA, HDL, and LDL; VAT area; and VAT/SAT ratio. Meanwhile, BG-dPVS scores were significantly associated with age, sex, creatinine levels, VAT area, and VAT/SAT ratio. Tolerance (TC) = 0.095 and variance inflation factor (VIF) = 10.577 were collinear with other indicators; hence, they were not considered in this study.
Table 2. Univariate ordinal regression analysis between dPVS scores and demographic, laboratory and radiological factors.
After adjusting for age; sex; and levels of glucose, creatinine, UA, HDL, and LDL, the association between CSO-dPVS scores and VAT area remained significant (P = 0.004), while no association was found between BG-dPVS scores and adipose tissue indicators after adjusting for age, sex, and creatinine levels (Table 3).
In the sex-stratified multivariate ordinal regression analysis, only significant association was found only between VAT area and CSO-dPVS scores of males (P = 0.001). When the VAT area data were divided into 4 parts, the OR (95% CI) value was 1.33 (1.139–1.557) (P < 0.001) between VAT area levels and CSO-dPVS scores of males.
In this study, we demonstrated that the VAT area was positively associated with CSO-dPVS scores in a neurologically healthy cohort, while SAT did not show a positive association. Because other obesity parameters showed no statistical significance, VAT was acknowledged as the most potent predictor of dPVS scores in our cohort. Furthermore, this association was more prominent in male subjects.
The exact underlying pathophysiologic mechanisms of the relationship between adipose tissue and dPVS are not clear. The positive association of VAT area and CSO-dPVS scores can be attributed to the following reasons. First, obesity is thought to be a systemic disease that affects every cell in the body through endocrine, metabolic, and inflammatory activities of adipocytes (Mazurek et al., 2003; Raggi, 2013). Large amounts of VAT in the body have been linked to cerebrovascular risk factors in numerous studies (Finelli et al., 2013). Second, the basic pathology of CSVD has been reported to originate from vascular endothelial cells (Wardlaw et al., 2019), while vascular endothelial cells are also affected by pro-inflammatory cytokines secreted by adipocytes, such as plasminogen activator inhibitor type-1, tumor necrosis factor-alpha, and interleukin 1 and 6 (Schafer and Konstantinides, 2011). In addition, some studies suggested that VAT mainly secreted pro-inflammatory factors, while SAT mainly secreted beneficial factors, such as leptin (Ibrahim, 2010). This explains why we found a negative correlation between SAT and dPVS scores in the ordinal regression analysis, although it was not statistically significant. The finding of a positive association between CSO-dPVS and VAT area has not been reported in previous studies, but it has been reported that other types of CSVD such as white matter hyperintensity, lacunae, and microbleeding (Karcher et al., 2013; Yamashiro et al., 2014) are related to VAT, so we believe that our findings are consistent with the conclusions of previous literature.
Interestingly, the effects of VAT were more prominent in males than in females. Sex differences were always a confounding factor. The findings of the present study might be explained by the difference of fat distribution in males and females (Golan et al., 2012; Bouchi et al., 2015). Also, males tend to accumulate fat at the visceral depot at any age (Matsuzawa et al., 1995) which causes males to have more VAT than females. Moreover, as previously discussed, VAT, as an endocrine organ (Wajchenberg, 2000), had more negative effects than SAT, which may lead to vascular endothelial damage (Virdis et al., 2019).
Despite the novel findings of this study, there were some limitations. First, there were differences in the proportion of males and females, and although we corrected for the sex factor in the statistical model, the non-linear effect might still be present as a confounding factor. Second, this study was a cross-sectional analysis, so we were unable to provide evidence of causality. Further prospective studies are needed to identify the underlying pathophysiological mechanisms. Finally, our study was designed as a single-center, retrospective observational study. Analyses were limited to persons with no obvious abnormalities on their brain MRI (participants with brain infarcts or hematomas were excluded). Hence, our study population is not representative of the general population in this age range, and a selection bias cannot be excluded.
We demonstrated a positive association between VAT and CSO-dPVS scores in a healthy cohort, which was more prominent in males. Considering that VAT had a significant association with various risk factors associated with the development of dPVS, we speculated that obese men might have poorer clearance of the glymphatic system in the brain, and further animal experiments are needed to confirm it.
The raw data supporting the conclusions of this article will be made available by the authors, without undue reservation.
The studies involving human participants were reviewed and approved by the Medical Ethics Committee of The First Affiliated Hospital of Wenzhou Medical University. Written informed consent from the patients/participants are not required to participate in this study in accordance with the national legislation and the institutional requirements.
YL and YY directed the experiment’s overall design and revised the manuscript. YQ and ML collected the data. YL and YQ evaluated the image data. YL conceived of the design’ details and analytic plan, then performed the statistical analyses, and drafted the manuscript. All authors contributed to the article and approved the submitted version.
The authors declare that the research was conducted in the absence of any commercial or financial relationships that could be construed as a potential conflict of interest.
CSVD, cerebral small vessel disease; CT, computed tomography; dPVS, dilated perivascular spaces; HDL, high-density lipoprotein; LDL, low-density lipoprotein; MR, magnetic resonance; SAT, subcutaneous adipose tissue; TC, total cholesterol; TP, total protein; UN, urea nitrogen; VAT, visceral adipose tissue.
Bacyinski, A., Xu, M., Wang, W., and Hu, J. (2017). The paravascular pathway for brain waste clearance: current understanding, significance and controversy. Front. Neuroanat. 11:101. doi: 10.3389/fnana.2017.00101
Bouchi, R., Takeuchi, T., Akihisa, M., Ohara, N., Nakano, Y., Nishitani, R., et al. (2015). High visceral fat with low subcutaneous fat accumulation as a determinant of atherosclerosis in patients with type 2 diabetes. Cardiovasc. Diabetol. 14:136.
Doubal, F. N., MacLullich, A. M. J., Ferguson, K. J., Dennis, M. S., and Wardlaw, J. M. (2010). Enlarged perivascular spaces on MRI are a feature of cerebral small vessel disease. Stroke 41, 450–454. doi: 10.1161/STROKEAHA.109.564914
Fantuzzi, G., and Mazzone, T. (2007). Adipose tissue and atherosclerosis: exploring the connection. Arterioscler. Thromb. Vasc. Biol. 27, 996–1003. doi: 10.1161/atvbaha.106.131755
Feinleib, M. (1985). Epidemiology of obesity in relation to health hazards. Ann. Intern. Med. 103, 1019–1024. doi: 10.7326/0003-4819-103-6-1019
Finelli, C., Sommella, L., Gioia, S., La Sala, N., and Tarantino, G. (2013). Should visceral fat be reduced to increase longevity? Ageing Res. Rev. 12, 996–1004. doi: 10.1016/j.arr.2013.05.007
Fox, C. S., Massaro, J. M., Hoffmann, U., Pou, K., Maurovich-Horvat, P., and Liu, C. (2004). Abdominal visceral and subcutaneous adipose tissue compartments: association with metabolic risk factors in the framingham heart study. Circulation 116, 39–48. doi: 10.1161/circulationaha.106.675355
Gil, A., Olza, J., Gil-Campos, M., Gomez-Llorente, C., and Aguilera, C. M. (2011). Is adipose tissue metabolically different at different sites? Int. J. Pediatr. Obes. 6, 13–20. doi: 10.3109/17477166.2011.604326
Golan, R., Shelef, I., Rudich, A., Gepner, Y., Shemesh, E., Chassidim, Y., et al. (2012). Abdominal superficial subcutaneous fat: a putative distinct protective fat subdepot in type 2 diabetes. Diabetes Care 35, 640–647. doi: 10.2337/dc11-1583
Ibrahim, M. M. (2010). Subcutaneous and visceral adipose tissue: structural and functional differences. Obes. Rev. 11, 11–18. doi: 10.1111/j.1467-789x.2009.00623.x
Kannel, W. B. (1985). Lipids, diabetes, and coronary heart disease: insights from the Framingham Study. Am. Heart J. 110, 1100–1107. doi: 10.1016/0002-8703(85)90224-8
Karcher, H.-S., Holzwarth, R., Mueller, H.-P., Ludolph, A. C., Huber, R., Kassubek, J., et al. (2013). Body fat distribution as a risk factor for cerebrovascular disease: an MRI-based body fat quantification study. Cerebrovasc. Dis. 35, 341–348. doi: 10.1159/000348703
Keys, A. B. (1980). Overweight, obesity, coronary heart disease, and mortality. Nutr. Today 15, 16–22. doi: 10.1097/00017285-198007000-00004
Kim, K. W., Seo, H., Kwak, M. S., and Kim, D. (2017). Visceral obesity is associated with white matter hyperintensity and lacunar infarct. Int. J. Obes. 41, 683–688. doi: 10.1038/ijo.2017.13
Matsuzawa, Y., Nakamura, T., Shimomura, I., and Kotani, K. (1995). Visceral fat accumulation and cardiovascular disease. Obes. Res. 3, 645S–647S.
Mazurek, T., Zhang, L., Zalewski, A., Mannion, J. D., Diehl, J. T., Arafat, H., et al. (2003). Human epicardial adipose tissue is a source of inflammatory mediators. Circulation 108, 2460–2466. doi: 10.1161/01.cir.0000099542.57313.c5
Porter, S. A., Massaro, J. M., Hoffmann, U., Vasan, R. S., O’Donnel, C. J., and Fox, C. S. (2009). Abdominal subcutaneous adipose tissue: a protective fat depot? Diabetes Care 32, 1068–1075. doi: 10.2337/dc08-2280
Raggi, P. (2013). Epicardial adipose tissue as a marker of coronary artery disease risk. J. Am. Coll. Cardiol. 61, 1396–1397. doi: 10.1016/j.jacc.2012.12.028
Schafer, K., and Konstantinides, S. V. (2011). Update on the cardiovascular risk in obesity: endocrine and paracrine role of the adipose tissue. Hellenic J. Cardiol. 52, 327–336.
Tarasoff-Conway, J. M., Carare, R. O., Osorio, R. S., Glodzik, L., Butler, T., Fieremans, E., et al. (2015). Clearance systems in the brain-implications for Alzheimer disease. Nat. Rev. Neurol. 11, 457–470. doi: 10.1038/nrneurol.2015.119
Virdis, A., Masi, S., Colucci, R., Chiriacò, M., Uliana, M., Puxeddu, I., et al. (2019). Microvascular endothelial dysfunction in patients with obesity. Curr. Hypertens. Rep. 21:32.
Wajchenberg, B. L. (2000). Subcutaneous and visceral adipose tissue: their relation to the metabolic syndrome. Endocr. Rev. 21, 697–738. doi: 10.1210/edrv.21.6.0415
Wardlaw, J. M., Benveniste, H., Nedergaard, M., Zlokovic, B. V., Mestre, H., Lee, H., et al. (2020). Perivascular spaces in the brain: anatomy, physiology and pathology. Nat. Rev. Neurol. 16, 137–153.
Wardlaw, J. M., Smith, C., and Dichgans, M. (2019). Small vessel disease: mechanisms and clinical implications. Lancet Neurol. 18, 684–696. doi: 10.1016/s1474-4422(19)30079-1
Yamashiro, K., Tanaka, R., Tanaka, Y., Miyamoto, N., Shimada, Y., Ueno, Y., et al. (2014). Visceral fat accumulation is associated with cerebral small vessel disease. Eur. J. Neurol. 21, 667–673. doi: 10.1111/ene.12374
Keywords: cerebral small vessel disease, abdominal obesity, visceral adipose tissue, MRI, dilated perivascular space
Citation: Qi Y, Lin M, Yang Y and Li Y (2021) Relationship of Visceral Adipose Tissue With Dilated Perivascular Spaces. Front. Neurosci. 14:583557. doi: 10.3389/fnins.2020.583557
Received: 16 July 2020; Accepted: 23 December 2020;
Published: 04 February 2021.
Edited by:
Riccarda Granata, University of Turin, ItalyReviewed by:
Zhendong Liu, Shandong First Medical University, ChinaCopyright © 2021 Qi, Lin, Yang and Li. This is an open-access article distributed under the terms of the Creative Commons Attribution License (CC BY). The use, distribution or reproduction in other forums is permitted, provided the original author(s) and the copyright owner(s) are credited and that the original publication in this journal is cited, in accordance with accepted academic practice. No use, distribution or reproduction is permitted which does not comply with these terms.
*Correspondence: Yanxuan Li, MjU4MjI3NDY0QHFxLmNvbQ==; Yunjun Yang, eXlqdW5qaW1AMTYzLmNvbQ==
†These authors have contributed equally to this work
Disclaimer: All claims expressed in this article are solely those of the authors and do not necessarily represent those of their affiliated organizations, or those of the publisher, the editors and the reviewers. Any product that may be evaluated in this article or claim that may be made by its manufacturer is not guaranteed or endorsed by the publisher.
Research integrity at Frontiers
Learn more about the work of our research integrity team to safeguard the quality of each article we publish.