- 1Research Group on Biology of Exercise, Department of Health Sciences, Centro Araranguá, Federal University of Santa Catarina, Araranguá, Brazil
- 2Laboratório de Bioenergética e Estresse Oxidativo, Departamento de Bioquímica, Centro de Ciências Biológicas, Universidade Federal de Santa Catarina, Florianópolis, Brazil
In understanding the pathology of neurological diseases, the role played by brain energy metabolism is gaining prominence. Animal models have demonstrated that regular physical exercise improves brain energy metabolism while also providing antidepressant, anxiolytic, antioxidant and neuroprotective functions. This review summarizes the latest evidence on the roles played by peroxisome proliferator-activated receptor gamma (PPAR-γ) coactivator 1-alpha (PGC-1α) and mitochondrial uncoupling protein (UCP) in this scenario. The beneficial effects of exercise seem to depend on crosstalk between muscles and nervous tissue through the increased release of muscle irisin during exercise.
Introduction
A physically inactive lifestyle is associated with the development of non-communicable diseases (NCD), such as cardiovascular diseases, type 2 diabetes, some cancers, and an overall increased mortality rate (Booth et al., 2012; Koster et al., 2012; Biswas et al., 2015; Same et al., 2016; Patterson et al., 2018). Physical inactivity is also considered a risk factor for abdominal obesity, high serum triglyceride levels, low-density lipoprotein, cholesterol, hypertension, and hyperglycemia, which together characterize metabolic syndrome (Bankoski et al., 2011). Physical exercise has several benefits for physical and mental health (Aguiar et al., 2008; Garber et al., 2011; Esteban-Cornejo et al., 2015; Loprinzi, 2015), including increased physical and cardiorespiratory capacity (or fitness), improved body composition and balance (or fatness), and greater muscle strength and flexibility (Garber et al., 2011; Geneen et al., 2017). Physical exercise also improves the serum lipid profile, decreases glucose intolerance, and attenuates insulin resistance (Lin et al., 2015; Brymer and Davids, 2016; Qiu et al., 2018). The literature supports the fitness-fatness hypothesis, which suggests that a higher level of cardiorespiratory fitness will reduce the adverse effects of obesity on morbidity and mortality, making obesity a much less important factor for health than is generally believed (Hainer et al., 2009; Fogelholm, 2010; Barry et al., 2014). The data are mixed, but for many authors, fitness is more important than fatness for early mortality (Blair et al., 1989; Wei et al., 1999; McAuley et al., 2009; Barry et al., 2014). This is important for individuals who are unable to lose weight but are able to engage in a regular physical activity program.
The American College of Sports Medicine (ACSM) recommends increasing total energy expenditure (TEE; kcal/day) for health, with a minimum of 30 min of moderate physical exercise 5 days/week or 20 min of vigorous exercise 3 days/week (Haskell et al., 2007). The ACSM also recommends combining moderate (3–6 MET) and vigorous activities (>6 MET) (Haskell et al., 2007). The World Health Organization (2000) recommends that individuals participate in at least 150 or 75 min/week of moderate or vigorous physical activities, respectively. For overweight and obesity, the ACSM recommends increased physical activity, between 150 and 250 min/week to prevent weight gain or provide modest weight loss (Donnelly et al., 2009). Larger amounts of exercise (>250 min/week) are needed for clinically significant weight loss (Donnelly et al., 2009). However, physical activity for weight loss is controversial; the amount of weight lost due to an exercise intervention is often less than what is predicted to be lost based on the exercise workload, suggesting a smaller increase in TEE (and smaller energy imbalance) than expected (Thomas et al., 2012; Melanson et al., 2013; Flack et al., 2018). This reduced energy imbalance occurs through metabolic and behavioral modifications in humans (Pontzer, 2018) and reinforces the hypothesis that fitness is more important than fatness for health. In general, the 150 min/week of moderate physical activity or 60–75 min/week of vigorous activity recommendations of ACSM and WHO is effective for overall health.
Even single exercise sessions, which increases the production of endogenous opioids (Geneen et al., 2017), angiogenesis factors (such as vascular endothelial growth factor [VEGF], hypoxia-induced 1 alpha factor [HIF-1α] and erythropoietin [EPO]) (Ribeiro et al., 2017) appear to be healthy, and they protect against hyperglycemia peaks (Lang Lehrskov et al., 2018) in humans. A single exercise session increases the plasma endocannabinoid levels in mice (Fuss et al., 2015), which is a possible mechanism for the euphoric state (runner’s high) that occurs after long runs (Boecker et al., 2008).
The central nervous system (CNS) was the last physiological system approached by the exercise sciences. Lack of exercise is a major cause of chronic diseases (Booth et al., 2012), including brain diseases, such as depression (Farmer et al., 1988; Aguiar et al., 2014), and neurodegenerative diseases (Radak et al., 2010; Xu et al., 2010; Aguiar et al., 2016). However, physical exercise is a neuroprotective agent against depression (Schuch et al., 2017), anxiety disorders (Jayakody et al., 2014), cognitive decline/dementia in elderly people (Aguiar et al., 2011; Shen et al., 2016), Parkinson’s disease (Chen et al., 2018), and Alzheimer’s disease (Aguiar et al., 2016; Law et al., 2018). Animal studies have shown that physical exercise increases neuronal survival, cerebral vascularization, neurogenesis, and mitochondrial metabolism, while it decreases the effects of neurotoxins on the CNS (Aguiar et al., 2014; Zhang and Zhang, 2016). Iris and uncoupling proteins (U) are candidate mechanisms for these exercise-induced changes.
In mammals, transcriptional peroxisome proliferator-activated receptor gamma (PPAR-γ) coactivator 1-alpha (PGC-1α)/fibronectin type III domain-containing protein 5 (FNDC5, the precursor of irisin), which is secreted during exercise, promotes the browning of beige fat cells in white adipose tissue (Figure 1), resulting in enhanced thermogenesis and increased energy expenditure (Hofmann et al., 2014). In the CNS (Figure 2), FCDN5/irisin regulates central mechanisms that mediate adaptive responses by (a) improving neuronal mitochondrial decoupling and (b) increasing the expression of neurotrophins and neuroprotective proteins such as neuronal PAS domain protein 4 (NPAS4), cFOS, activity-regulated cytoskeleton-associated protein (ARC), and zinc finger protein 268 (ZIF268) (Figure 1; Wrann et al., 2013; Wrann, 2015). In brown adipose tissue, mitochondrial uncoupling is effected by a specific protein, referred to as uncoupling protein-1 (UCP1), in the inner mitochondrial membrane (Ricquier and Bouillaud, 2000). The cloning of UCP2 and UCP3, two homologs of UCP1, has boosted research into the importance of respiration control in metabolic processes, metabolic diseases and energy balance (Ricquier and Bouillaud, 2000). PPAR-γ/PGC-1α expression also improves mitochondrial decoupling, which reduces mitochondrial membrane potential and reactive oxygen species (ROS) production, oxidative damage, mitochondrial calcium overload and potential apoptotic events through the induction of uncoupling protein 2 (UCP2) (Andrews et al., 2005). Therefore, FND5/irisin is essential for processes involving neurotrophins and synaptic plasticity, mitochondrial biogenesis, and resistance to neuronal stress (Wrann et al., 2013; Marosi and Mattson, 2014; Raefsky and Mattson, 2017).
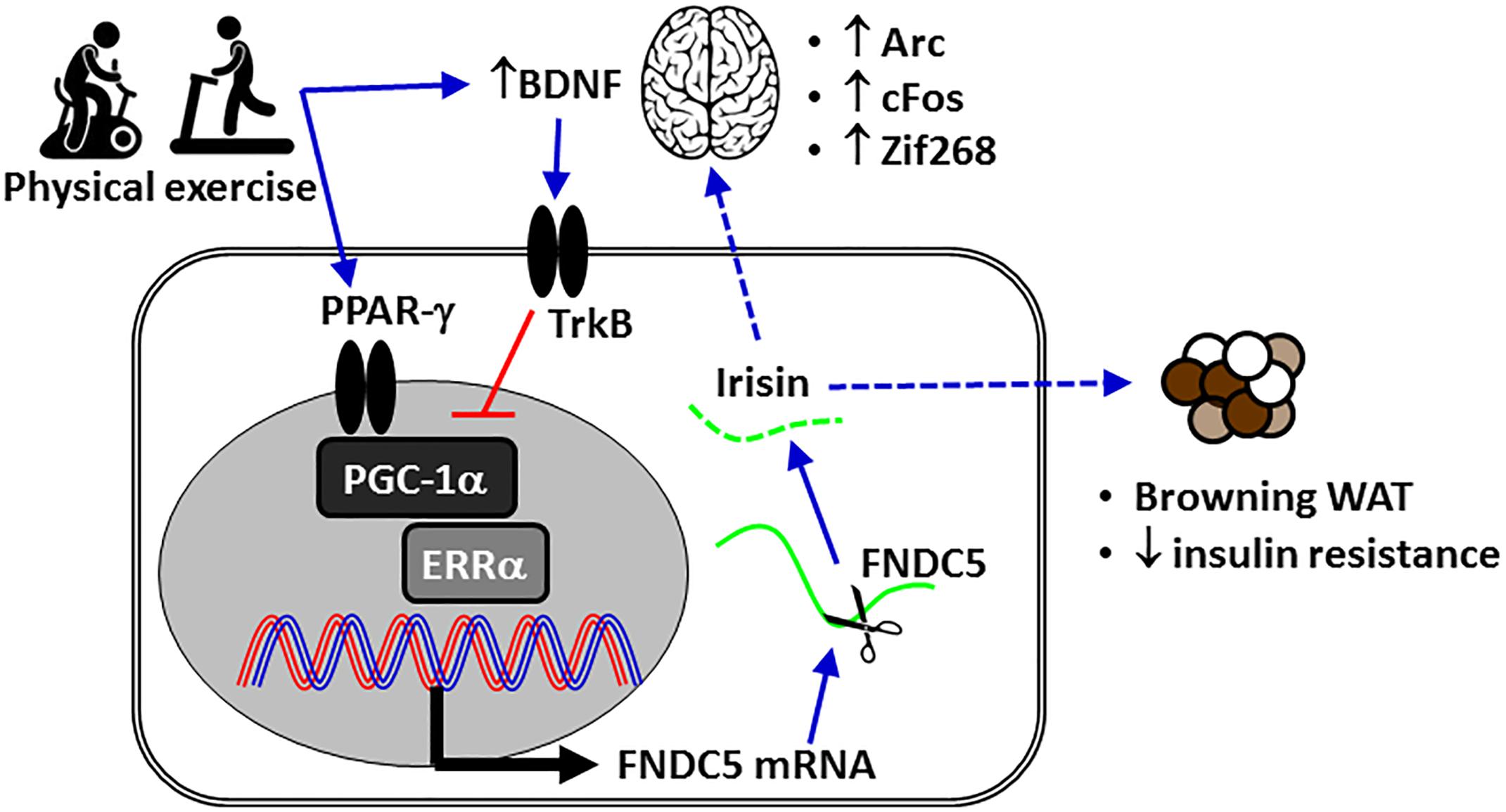
Figure 1. The mechanism of action of irisin in metabolism-associated health issues or metabolic diseases. The expression of Arc, cFos, and Zif268 is induced by neuronal activity. BDNF, brain-derived neurotrophic factor; ERRα, estrogen-related receptor alpha; FNDC5, fibronectin domain-containing protein 5; PGC-1α, peroxisome proliferator-activated receptor gamma coactivator-1-alpha; TrkB, tyrosine receptor kinase B; WAT, white adipose tissue.
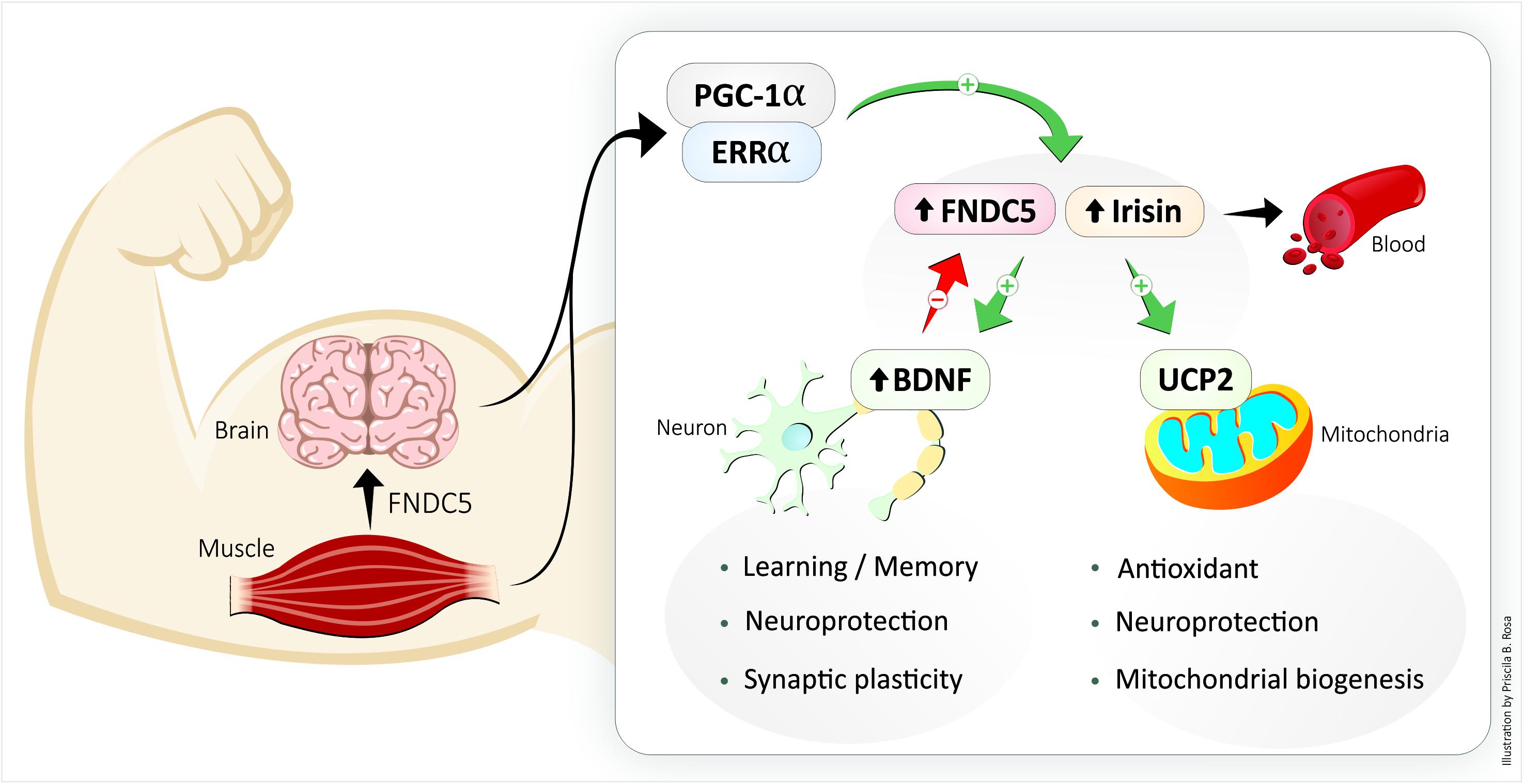
Figure 2. The exercise-increased circulating protein irisin links structural and functional modifications in muscle and brain. BDNF, brain-derived neurotrophic factor; FNDC5, fibronectin type III domain-containing protein 5; UCP2, uncoupling protein 2.
The purpose of this manuscript is to review the roles played by FND5/irisin and UCP2, which are important for energy metabolism, in the neuroprotective and antioxidant effects of physical activity in the CNS.
The Exercise-Induced Release of Irisin and Its Neuroprotective Effects
Because irisin is an exercise-induced hormone (or myokine), it is unclear whether the physical exercise-related CNS benefits are attributable to irisin. Irisin is a 112 amino acid peptide that is cleaved (by an unknown protease) from the glycosylated type I membrane protein FNDC5 and released into the bloodstream in a PGC-1α-dependent manner through a muscle contraction-mediated transcription mechanism (Bostrom et al., 2012). PGC-1α is a transcriptional coactivator and does not bind to DNA directly; it needs to interact with another transcription factor to induce neuronal FNDC5 gene expression (Xu, 2013). Several clues indicate that the PGC-1α binding partner is orphan nuclear estrogen-related receptor alpha (ERRα) (Kamei et al., 2003; Xu, 2013). Moreover, irisin enhances PGC-1α expression in the hippocampus and prefrontal cortex of mice in a positive feedback loop (Siteneski et al., 2018). The irisin released from muscles is a myokine that acts preferentially on the subcutaneous ‘beige’ fat and causes it to ‘brown’ by increasing the expression of UCP1 and other thermogenic genes (Bostrom et al., 2012). Irisin is involved in human biological adaptations such as increased muscle strength, decreased obesity and insulin resistance, and also has physical and psychological benefits (Bostrom et al., 2012; Ghahrizjani et al., 2015). Currently, it is hypothesized that irisin circulates in the blood in vesicles containing other molecules, such as proteins, miRNA and nucleic acids, until reaching the target tissues, which include adipose tissue and the brain (Safdar et al., 2016). The exercise-induced release of peptides and nucleic acids from skeletal muscle (collectively termed ‘exerkines’) has been implicated in mediating systemic biological adaptations (Safdar et al., 2016).
The contraction of large muscle groups increases the muscle-specific expression of PGC1-α and FNDC5 and consequent release of irisin (Bostrom et al., 2012). In humans, blood irisin levels reach approximately 3.6 ng/ml in sedentary individuals and 4.3 ng/ml in active individuals after 12 weeks of regular aerobic exercise (Jedrychowski et al., 2015). Irisin contributes to exercise-induced physiological adaptations in the cardiovascular, immune, digestive, and adipose systems (Bostrom et al., 2012; Zhang et al., 2015; Mazur-Bialy et al., 2017). In obese adult humans, exercise combined with caloric restriction improves health (Mozaffarian et al., 2011) and increases concentrations of circulating irisin (Huang et al., 2017). Although skeletal muscle is the major source of exercise-induced irisin that is released into the plasma (Roca-Rivada et al., 2013), it remains unclear whether neuronal irisin is derived from muscles or is produced in neurons. In neurons, PGC-1α interacts with estrogen-related receptor alpha (ERRα) to regulate the expression of FNDC5 (Figure 1; Wrann et al., 2013). Moreover, the increased expression of FNDC5 promotes neuronal development and differentiation (Forouzanfar et al., 2015; Ghahrizjani et al., 2015). FNDC5 can be found in the cerebrospinal fluid, cortical neurons, paraventricular neurons in the hippocampus, Purkinje cells in the cerebellum, hypothalamus, multipolar neurons in the anterior nerve of the spinal cord, and in astrocytes and microglia in the cerebral tissue (Dun et al., 2013; Moon et al., 2013; Piya et al., 2014; Albayrak et al., 2015). In the rat H19-7HN cell line, irisin (50–100 nmol/l) increased the proliferation of hippocampal cells, thus reinforcing its role in neurogenesis (Moon et al., 2013).
Wrann et al. (2013) demonstrated that the expression of PGC-1α and FNDC5 in the hippocampal neurons was enhanced after the mice spent 2 weeks running in exercise wheels. Higher FNDC5 expression also increased the expression of the genes BDNF, Arc, cFos, and Zif268, which is induced by neuronal activity. FNDC5 expression is counterbalanced by BDNF expression in a negative feedback mechanism (Figure 1; Wrann et al., 2013). It is possible that this feedback loop is a CNS detraining mechanism that requires regular exercise to maintain its neurological benefits. This evidence suggests that the induction of FNDC5 is part of the transcriptional response to exercise, including neuroplasticity and neuroprotection, in the CNS. Exercise-induced PGC-1α and irisin reduced ischemia-induced neuronal injury (Zhang et al., 2012) via activation of the Akt and ERK1/2 signaling pathways in mice (Li et al., 2017). Exercise-induced irisin also reduced the brain infarct volume, neurological deficits, brain edema and the body weight decline of mice subjected to middle cerebral artery occlusion (MCAO) (Li et al., 2017). Since BDNF is a critical regulator of neural plasticity, irisin may act as a key regulator of neuronal survival following cerebral ischemia. Physical activity (running wheel, 12 weeks) increases levels of circulating irisin and BDNF even in 20-month-old female rats (Belviranli and Okudan, 2018), increases the expression of BDNF and decreases neuroinflammation in the hippocampus of aged rats and mice, and has motor and cognitive benefits (Aguiar et al., 2011; Dallagnol et al., 2017).
Exercise is an antidepressant (Blumenthal et al., 1999; Cunha et al., 2013), and irisin has been linked to the antidepressant effects of exercise. Reduced irisin levels are associated with mood impairment and reduced BDNF levels in humans (Papp et al., 2017; Szilasi et al., 2017), and increased circulating concentrations of irisin have been shown to have antidepressant effects in mice (Siteneski et al., 2018). A possible mechanism for the antidepressant effect is the activation of the PGC-1α/BDNF pathway by irisin after exercise (Wrann et al., 2013). As previously mentioned, BDNF is a critical neurotrophin involved in the differentiation, survival, maintenance, and function of neurons; it is also involved in learning and memory processes (Wrann et al., 2013). Torma et al. (2014) demonstrated that the neurotrophic role of BDNF is dependent on PGC-1α.
In humans, the increased plasma levels of FNDC-5, irisin and BDNF seem to depend on exercising large muscle groups, as can be achieved with regular Nordic walking training (Gmiat et al., 2018), aquarobics (16 weeks) (Kim and Kim, 2018), and CrossFit training (12 weeks) (Murawska-Cialowicz et al., 2015). The electrical stimulation of small muscle groups increases BDNF but not irisin in the hippocampus of rats (Maekawa et al., 2018). In healthy elderly women, Nordic walking training improved body composition, anaerobic capacity and cardiovascular fitness (Gmiat et al., 2018), and CrossFit training improved psychological (Quality-of-Life Assessment and The Beck Depression Inventory-2) and cognitive functions (D2 test of attention and Trail Making Test A&B).
Neuronal UCP2 –An Antioxidant Mechanism of Exercise
The expression of neuronal uncoupling proteins (UCP) is induced by metabolic and oxidative challenges such as physical exercise and caloric restriction (Liu et al., 2015). UCP facilitates proton flux through the internal mitochondrial membrane, thereby dissociating the oxidative phosphorylation of ATP synthesis (Wei et al., 2009). The enhanced proton flux process reduces the mitochondrial membrane potential, increases mitochondrial respiration, decreases the ATP/ADP ratio, and dissipates chemical energy in the form of heat (Chu et al., 2009). Acute mitochondrial decoupling reduces mitochondrial ATP production; however, chronic mitochondrial decoupling promotes an increase in the number of mitochondria and an increased level of ATP production (Coppola et al., 2007).
Initially, UCP1, which functions in heat production, was identified in brown adipose tissue (Geisler et al., 2017). UCP2 is found in organs and tissues such as the liver, kidney, pancreas, endothelium, immune cells, and the CNS (Pecqueur et al., 2001; Chu et al., 2009). UCP2, UCP4, and UCP5 are expressed in the CNS, are referred to as neuronal U, and are involved in the adaptation to cellular stress (Chu et al., 2009). The distribution of neuronal UCPs demonstrates the relevance of mitochondrial decoupling in the CNS to the control of neuronal, neuroendocrine, and autonomic responses (Richard et al., 1998). UCP2 is expressed in the hypothalamus, especially in the arcuate nucleus, limbic system, cerebellum, choroid plexus, and encephalic trunk (Richard et al., 1998; Arsenijevic et al., 2000). UCP4 is detected in most brain tissues, but it is expressed at lower levels in the spinal cord and Substantia nigra (Mao et al., 1999). UCP5 is expressed in the cerebral cortex, hippocampus, thalamus, hypothalamus, amygdala, basal ganglia, and spinal cord (Kwok et al., 2010).
Neuronal UCPs influence the regulation of mitochondrial biogenesis, calcium flux, ROS production, and local temperature (Teshima et al., 2003). Neuronal UCPs play an important role in the reduction of ROS production and consequent reduction in oxidative stress without compromising the production of ATP (Arsenijevic et al., 2000). Exposure of cultured neurons to decoupling agents, such as carbonyl cyanide-4-(trifluoromethoxy) phenylhydrazone (FCCP) or 2,4-dinitrophenol (2,4-DNP or simply DNP), reduces the mitochondrial membrane potential and inhibits mitochondrial calcium absorption, and thus prevents cell death (Stout et al., 1998). Neuronal UCPs also influence the temperature of neuronal microenvironments and thus contribute to the dynamics of neuronal activity through greater synaptic plasticity and neuronal transmission (Arsenijevic et al., 2000). Some studies have suggested that mitochondrial decoupling is linked to neuroprotection against physiological processes and pathological mechanisms including aging, Alzheimer’s and Parkinson’s diseases, neuronal hypoxia and ischemia, and epilepsy (Bechmann et al., 2002; Dietrich et al., 2008).
Among the neuronal UCPs, UCP2 is involved in central autonomic, endocrine, and metabolic regulation and is thus associated with cognition, mood, and behavior (Diano et al., 2000; Wang et al., 2014). UCP2 in the ventromedial nucleus restores glucose tolerance and regulates insulin sensitivity mediated by glucose-excited neurons, which is important for the physiological control of systemic glucose metabolism (Toda et al., 2016). In the arcuate nucleus, UCP2 is associated with mitochondrial fission, increased mitochondrial density and diminished mitochondrial size (Toda et al., 2016). UCP2 shows increased expression after neuronal injury (Bechmann et al., 2002). UCP2 induces mitochondrial decoupling in nigral neurons of the substantia nigra pars compacta (SNpc) and can prevent the loss of dopaminergic cells after 1-methyl-4-phenyl-1,2,5,6-tetrahydropyridine (MPTP)-induced toxicity, which is an essential effect to delay Parkinson’s disease pathophysiology (Echtay et al., 2001; Horvath et al., 2003). The relation of UCP2 to exercise occurs through the PGC-1α/PPARα pathway, which can regulate neuronal UCP2 (Wu et al., 2014) and BDNF (Gomez-Pinilla et al., 2008). Physical activity (running wheel, 4 weeks) increased UCP2 expression and mitochondrial oxygen consumption in coupled and uncoupled mitochondria in the hippocampus of mice (Dietrich et al., 2008). Moreover, physical activity (running wheel, 1 week) and exercise (treadmill, 12 weeks) increased UCP2 levels in the hippocampus, cerebellum and brain cortex mitochondria of adult rats (Gomez-Pinilla et al., 2008; Marques-Aleixo et al., 2015). The exercise-induced (running wheel, 1 week) increase in UCP2 correlated with increased BDNF in the hippocampus of rats (Gomez-Pinilla et al., 2008). These changes in BDNF content and mitochondrial metabolism (Vaynman et al., 2006; Aguiar et al., 2007, 2014) coincided with an increase in the number of mitochondria and dendritic spine synapses in the granule cells of the dentate gyrus and the stratum radiatum of the CA1 region and were dependent on UCP2 expression because such changes were not observed in UCP2 knockout mice (Dietrich et al., 2008). The absence of proper mitochondrial decoupling reduced the number of synapses in hippocampal neurons due to the increase in free radical production in response to exercise, thus demonstrating the characteristic protective effect of UCP2 in this knockout mouse model (Dietrich et al., 2008). For example, doxorubicin is an effective antineoplastic agent that is limited by mitochondrial toxicity in non-target tissues, including the brain (Marques-Aleixo et al., 2016; Flanigan et al., 2018). Doxorubicin (2 mg/kg, i.p.) impaired spatial learning/memory and decreased UCP2 protein content in cerebellum and brain cortex mitochondria of adult rats, both of which were prevented by physical activity (treadmill, 12 weeks) (Marques-Aleixo et al., 2016). The UCP2-related nuclear respiration factor 1 (NRF1) and mitochondrial transcription factor A (TFAM) genes, which are involved in mitochondrial biogenesis, are associated with synaptic plasticity and decreased neuronal vulnerability to cellular stress (Simon-Areces et al., 2012). In an animal model of Parkinson’s disease, 8 weeks of treadmill exercise stimulated mitochondrial biogenesis and increased NRF2 and TFAM expression in the striatum of mice, which protected against neuronal death caused by the neurotoxin 6-OHDA (Aguiar et al., 2016). The mitochondrial mechanism related to UCP2 function is essential for the appropriate bioenergetic adaptation of neurons to increased neuronal activity and synaptic plasticity in response to physical activity.
Conclusion
Exercise improves the PGC-1α/BDNF pathway (muscle/brain) through the signaling of circulating irisin, which strengthens synapses and exhibits neuroprotective and antidepressant effects. These neuroprotective effects of exercise are enhanced by the antioxidant effects of UCP2, which is expressed at increased levels in neurons in response to exercise. Therefore, the evidence suggests a role for irisin/UCP2 in the mechanism underlying the benefits of physical exercise on the CNS. Consequently, irisin/UCP2 might be a potential therapeutic target to improve brain function and prevent or treat neurological and neurodegenerative diseases.
Author Contributions
All authors listed have made a substantial, direct and intellectual contribution to the work, and approved it for publication.
Funding
The work was supported by CAPES (Coordenação de Aperfeiçoamento de Pessoal de Nível Superior), CNPq (Conselho Nacional de Desenvolvimento Científico e Tecnológico), and FAPESC (Fundação de Amparo à Pesquisa e Inovação do Estado de Santa Catarina). AA was a CNPq fellow.
Conflict of Interest Statement
The authors declare that the research was conducted in the absence of any commercial or financial relationships that could be construed as a potential conflict of interest.
References
Aguiar, A. S. Jr., Castro, A. A., Moreira, E. L., Glaser, V., Santos, A. R., Tasca, C. I., et al. (2011). Short bouts of mild-intensity physical exercise improve spatial learning and memory in aging rats: involvement of hippocampal plasticity via AKT, CREB and BDNF signaling. Mech. Ageing Dev. 132, 560–567. doi: 10.1016/j.mad.2011.09.005
Aguiar, A. S. Jr., Duzzioni, M., Remor, A. P., Tristao, F. S., Matheus, F. C., Raisman-Vozari, R., et al. (2016). Moderate-intensity physical exercise protects against experimental 6-hydroxydopamine-induced hemiparkinsonism through Nrf2-antioxidant response element pathway. Neurochem. Res. 41, 64–72. doi: 10.1007/s11064-015-1709-8
Aguiar, A. S. Jr., Stragier, E., da Luz, Scheffer, D., Remor, A. P., Oliveira, P. A., et al. (2014). Effects of exercise on mitochondrial function, neuroplasticity and anxio-depressive behavior of mice. Neuroscience 271, 56–63. doi: 10.1016/j.neuroscience.2014.04.027
Aguiar, A. S. Jr., Tuon, T., Albuquerque, M. M., Rocha, G. S., Speck, A. E., Araujo, J. C., et al. (2008). The exercise redox paradigm in the Down’s syndrome: improvements in motor function and increases in blood oxidative status in young adults. J. Neural Transm. 115, 1643–1650. doi: 10.1007/s00702-008-0120-x
Aguiar, A. S. Jr., Tuon, T., Pinho, C. A., Silva, L. A., Andreazza, A. C., Kapczinski, F., et al. (2007). Mitochondrial IV complex and brain neurothrophic derived factor responses of mice brain cortex after downhill training. Neurosci. Lett. 426, 171–174. doi: 10.1016/j.neulet.2007.08.058
Albayrak, S., Atci, I. B., Kalayci, M., Yilmaz, M., Kuloglu, T., Aydin, S., et al. (2015). Effect of carnosine, methylprednisolone and their combined application on irisin levels in the plasma and brain of rats with acute spinal cord injury. Neuropeptides 52, 47–54. doi: 10.1016/j.npep.2015.06.004
Andrews, Z. B., Diano, S., and Horvath, T. L. (2005). Mitochondrial uncoupling proteins in the CNS: in support of function and survival. Nat. Rev. Neurosci. 6, 829–840. doi: 10.1038/nrn1767
Arsenijevic, D., Onuma, H., Pecqueur, C., Raimbault, S., Manning, B. S., Miroux, B., et al. (2000). Disruption of the uncoupling protein-2 gene in mice reveals a role in immunity and reactive oxygen species production. Nat. Genet. 26, 435–439. doi: 10.1038/82565
Bankoski, A., Harris, T. B., McClain, J. J., Brychta, R. J., Caserotti, P., Chen, K. Y., et al. (2011). Sedentary activity associated with metabolic syndrome independent of physical activity. Diabetes Care 34, 497–503. doi: 10.2337/dc10-0987
Barry, V. W., Baruth, M., Beets, M. W., Durstine, J. L., Liu, J., and Blair, S. N. (2014). Fitness vs. fatness on all-cause mortality: a meta-analysis. Prog. Cardiovasc. Dis. 56, 382–390. doi: 10.1016/j.pcad.2013.09.002
Bechmann, I., Diano, S., Warden, C. H., Bartfai, T., Nitsch, R., and Horvath, T. L. (2002). Brain mitochondrial uncoupling protein 2 (UCP2): a protective stress signal in neuronal injury. Biochem. Pharmacol. 64, 363–367. doi: 10.1016/S0006-2952(02)01166-8
Belviranli, M., and Okudan, N. (2018). Exercise training increases cardiac, hepatic and circulating levels of brain-derived neurotrophic factor and irisin in young and aged rats. Horm. Mol. Biol. Clin. Investig. 36:20180053. doi: 10.1515/hmbci-2018-0053
Biswas, A., Oh, P. I., Faulkner, G. E., Bajaj, R. R., Silver, M. A., Mitchell, M. S., et al. (2015). Sedentary time and its association with risk for disease incidence, mortality, and hospitalization in adults: a systematic review and meta-analysis. Ann. Intern. Med. 162, 123–132. doi: 10.7326/M14-1651
Blair, S. N., Kohl, H. W. III, Paffenbarger, R. S. Jr., Clark, D. G., Cooper, K. H., and Gibbons, L. W. (1989). Physical fitness and all-cause mortality. A prospective study of healthy men and women. JAMA 262, 2395–2401. doi: 10.1001/jama.1989.03430170057028
Blumenthal, J. A., Babyak, M. A., Moore, K. A., Craighead, W. E., Herman, S., Khatri, P., et al. (1999). Effects of exercise training on older patients with major depression. Arch. Intern. Med. 159, 2349–2356. doi: 10.1001/archinte.159.19.2349
Boecker, H., Sprenger, T., Spilker, M. E., Henriksen, G., Koppenhoefer, M., Wagner, K. J., et al. (2008). The runner’s high: opioidergic mechanisms in the human brain. Cereb. Cortex 18, 2523–2531. doi: 10.1093/cercor/bhn013
Booth, F. W., Roberts, C. K., and Laye, M. J. (2012). Lack of exercise is a major cause of chronic diseases. Compr. Physiol. 2, 1143–1211. doi: 10.1002/cphy.c110025
Bostrom, P., Wu, J., Jedrychowski, M. P., Korde, A., Ye, L., Lo, J. C., et al. (2012). A PGC1-alpha-dependent myokine that drives brown-fat-like development of white fat and thermogenesis. Nature 11, 463–468. doi: 10.1038/nature10777
Brymer, E., and Davids, K. (2016). Designing environments to enhance physical and psychological benefits of physical activity: a multidisciplinary perspective. Sports Med. 46, 925–926. doi: 10.1007/s40279-016-0535-8
Chen, Y. H., Kuo, T. T., Kao, J. H., Huang, E. Y., Hsieh, T. H., Chou, Y. C., et al. (2018). Exercise ameliorates motor deficits and improves dopaminergic functions in the rat hemi-Parkinson’s model. Sci. Rep. 8:3973. doi: 10.1038/s41598-018-22462-y
Chu, A. C., Ho, P. W., Kwok, K. H., Ho, J. W., Chan, K. H., Liu, H. F., et al. (2009). Mitochondrial UCP4 attenuates MPP+ - and dopamine-induced oxidative stress, mitochondrial depolarization, and ATP deficiency in neurons and is interlinked with UCP2 expression. Free Radic. Biol. Med. 46, 810–820. doi: 10.1016/j.freeradbiomed.2008.12.015
Coppola, A., Liu, Z. W., Andrews, Z. B., Paradis, E., Roy, M. C., Friedman, J. M., et al. (2007). A central thermogenic-like mechanism in feeding regulation: an interplay between arcuate nucleus T3 and UCP2. Cell Metab. 5, 21–33. doi: 10.1016/j.cmet.2006.12.002
Cunha, M. P., Oliveira, A., Pazini, F. L., Machado, D. G., Bettio, L. E., Budni, J., et al. (2013). The antidepressant-like effect of physical activity on a voluntary running wheel. Med. Sci. Sports Exerc. 45, 851–859. doi: 10.1249/MSS.0b013e31827b23e6
Dallagnol, K. M. C., Remor, A. P., da Silva, R. A., Prediger, R. D., Latini, A., and Aguiar, A. S. Jr. (2017). Running for REST: physical activity attenuates neuroinflammation in the hippocampus of aged mice. Brain Behav. Immun. 61, 31–35. doi: 10.1016/j.bbi.2016.07.159
Diano, S., Urbanski, H. F., Horvath, B., Bechmann, I., Kagiya, A., Nemeth, G., et al. (2000). Mitochondrial uncoupling protein 2 (UCP2) in the nonhuman primate brain and pituitary. Endocrinology 141, 4226–4238. doi: 10.1210/endo.141.11.7740
Dietrich, M. O., Andrews, Z. B., and Horvath, T. L. (2008). Exercise-induced synaptogenesis in the hippocampus is dependent on UCP2-regulated mitochondrial adaptation. J. Neurosci. 28, 10766–10771. doi: 10.1523/JNEUROSCI.2744-08.2008
Donnelly, J. E., Blair, S. N., Jakicic, J. M., Manore, M. M., Rankin, J. W., and Smith, B. K. (2009). American college of sports medicine position stand. Appropriate physical activity intervention strategies for weight loss and prevention of weight regain for adults. Med. Sci. Sports Exerc. 41, 459–471. doi: 10.1249/MSS.0b013e3181949333
Dun, S. L., Lyu, R. M., Chen, Y. H., Chang, J. K., Luo, J. J., and Dun, N. J. (2013). Irisin-immunoreactivity in neural and non-neural cells of the rodent. Neuroscience 240, 155–162. doi: 10.1016/j.neuroscience.2013.02.050
Echtay, K. S., Winkler, E., Frischmuth, K., and Klingenberg, M. (2001). Uncoupling proteins 2 and 3 are highly active H(+) transporters and highly nucleotide sensitive when activated by coenzyme Q (ubiquinone). Proc. Natl. Acad. Sci. U.S.A. 98, 1416–1421. doi: 10.1073/pnas.98.4.1416
Esteban-Cornejo, I., Tejero-Gonzalez, C. M., Sallis, J. F., and Veiga, O. L. (2015). Physical activity and cognition in adolescents: a systematic review. J. Sci. Med. Sport 18, 534–539. doi: 10.1016/j.jsams.2014.07.007
Farmer, M. E., Locke, B. Z., Moscicki, E. K., Dannenberg, A. L., Larson, D. B., and Radloff, L. S. (1988). Physical activity and depressive symptoms: the NHANES I epidemiologic follow-up study. Am. J. Epidemiol. 128, 1340–1351. doi: 10.1093/oxfordjournals.aje.a115087
Flack, K. D., Ufholz, K., Johnson, L., Fitzgerald, J. S., and Roemmich, J. N. (2018). Energy compensation in response to aerobic exercise training in overweight adults. Am. J. Physiol. Regul. Integr. Comp. Physiol. 315, R619–R626. doi: 10.1152/ajpregu.00071.2018
Flanigan, T. J., Anderson, J. E., Elayan, I., Allen, A. R., and Ferguson, S. A. (2018). Effects of cyclophosphamide and/or doxorubicin in a murine model of postchemotherapy cognitive impairment. Toxicol. Sci. 162, 462–474. doi: 10.1093/toxsci/kfx267
Fogelholm, M. (2010). Physical activity, fitness and fatness: relations to mortality, morbidity and disease risk factors. A systematic review. Obes. Rev. 11, 202–221. doi: 10.1111/j.1467-789X.2009.00653.x
Forouzanfar, M., Rabiee, F., Ghaedi, K., Beheshti, S., Tanhaei, S., Shoaraye Nejati, A., et al. (2015). Fndc5 overexpression facilitated neural differentiation of mouse embryonic stem cells. Cell Biol. Int. 39, 629–637. doi: 10.1002/cbin.10427
Fuss, J., Steinle, J., Bindila, L., Auer, M. K., Kirchherr, H., Lutz, B., et al. (2015). A runner’s high depends on cannabinoid receptors in mice. Proc. Natl. Acad. Sci. U.S.A. 112, 13105–13108. doi: 10.1073/pnas.1514996112
Garber, C. E., Blissmer, B., Deschenes, M. R., Franklin, B. A., Lamonte, M. J., Lee, I. M., et al. (2011). American college of sports medicine position stand. Quantity and quality of exercise for developing and maintaining cardiorespiratory, musculoskeletal, and neuromotor fitness in apparently healthy adults: guidance for prescribing exercise. Med. Sci. Sports Exerc. 43, 1334–1359. doi: 10.1249/MSS.0b013e318213fefb
Geisler, J. G., Marosi, K., Halpern, J., and Mattson, M. P. (2017). DNP, mitochondrial uncoupling, and neuroprotection: a little dab’ll do ya. Alzheimers Dement. 13, 582–591. doi: 10.1016/j.jalz.2016.08.001
Geneen, L. J., Moore, R. A., Clarke, C., Martin, D., Colvin, L. A., and Smith, B. H. (2017). Physical activity and exercise for chronic pain in adults: an overview of cochrane reviews. Cochrane Database Syst. Rev. 1:CD011279. doi: 10.1002/14651858.CD011279.pub3
Ghahrizjani, F. A., Ghaedi, K., Salamian, A., Tanhaei, S., Nejati, A. S., Salehi, H., et al. (2015). Enhanced expression of FNDC5 in human embryonic stem cell-derived neural cells along with relevant embryonic neural tissues. Gene 557, 123–129. doi: 10.1016/j.gene.2014.12.010
Gmiat, A., Jaworska, J., Micielska, K., Kortas, J., Prusik, K., Lipowski, M., et al. (2018). Improvement of cognitive functions in response to a regular Nordic walking training in elderly women - a change dependent on the training experience. Exp. Gerontol. 104, 105–112. doi: 10.1016/j.exger.2018.02.006
Gomez-Pinilla, F., Vaynman, S., and Ying, Z. (2008). Brain-derived neurotrophic factor functions as a metabotrophin to mediate the effects of exercise on cognition. Eur. J. Neurosci. 28, 2278–2287. doi: 10.1111/j.1460-9568.2008.06524.x
Hainer, V., Toplak, H., and Stich, V. (2009). Fat or fit: what is more important? Diabetes Care 32(Suppl. 2), S392–S397. doi: 10.2337/dc09-S346
Haskell, W. L., Lee, I. M., Pate, R. R., Powell, K. E., Blair, S. N., Franklin, B. A., et al. (2007). Physical activity and public health: updated recommendation for adults from the American college of sports medicine and the American heart association. Med. Sci. Sports Exerc. 39, 1423–1434. doi: 10.1249/mss.0b013e3180616b27
Hofmann, T., Elbelt, U., and Stengel, A. (2014). Irisin as a muscle-derived hormone stimulating thermogenesis–a critical update. Peptides 54, 89–100. doi: 10.1016/j.peptides.2014.01.016
Horvath, T. L., Diano, S., Leranth, C., Garcia-Segura, L. M., Cowley, M. A., Shanabrough, M., et al. (2003). Coenzyme Q induces nigral mitochondrial uncoupling and prevents dopamine cell loss in a primate model of Parkinson’s disease. Endocrinology 144, 2757–2760. doi: 10.1210/en.2003-0163
Huang, J., Wang, S., Xu, F., Wang, D., Yin, H., Lai, Q., et al. (2017). Exercise training with dietary restriction enhances circulating irisin level associated with increasing endothelial progenitor cell number in obese adults: an intervention study. PeerJ 5:e3669. doi: 10.7717/peerj.3669
Jayakody, K., Gunadasa, S., and Hosker, C. (2014). Exercise for anxiety disorders: systematic review. Br. J. Sports Med. 48, 187–196. doi: 10.1136/bjsports-2012-091287
Jedrychowski, M. P., Wrann, C. D., Paulo, J. A., Gerber, K. K., Szpyt, J., Robinson, M. M., et al. (2015). Detection and quantitation of circulating human irisin by tandem mass spectrometry. Cell Metab. 22, 734–740. doi: 10.1016/j.cmet.2015.08.001
Kamei, Y., Ohizumi, H., Fujitani, Y., Nemoto, T., Tanaka, T., Takahashi, N., et al. (2003). PPARgamma coactivator 1beta/ERR ligand 1 is an ERR protein ligand, whose expression induces a high-energy expenditure and antagonizes obesity. Proc. Natl. Acad. Sci. U.S.A. 100, 12378–12383. doi: 10.1073/pnas.2135217100
Kim, J. H., and Kim, D. Y. (2018). Aquarobic exercises improve the serum blood irisin and brain-derived neurotrophic factor levels in elderly women. Exp. Gerontol. 104, 60–65. doi: 10.1016/j.exger.2018.01.024
Koster, A., Caserotti, P., Patel, K. V., Matthews, C. E., Berrigan, D., Van Domelen, D. R., et al. (2012). Association of sedentary time with mortality independent of moderate to vigorous physical activity. PLoS One 7:e37696. doi: 10.1371/journal.pone.0037696
Kwok, K. H., Ho, P. W., Chu, A. C., Ho, J. W., Liu, H. F., Yiu, D. C., et al. (2010). Mitochondrial UCP5 is neuroprotective by preserving mitochondrial membrane potential, ATP levels, and reducing oxidative stress in MPP+ and dopamine toxicity. Free Radic. Biol. Med. 49, 1023–1035. doi: 10.1016/j.freeradbiomed.2010.06.017
Lang Lehrskov, L., Lyngbaek, M. P., Soederlund, L., Legaard, G. E., Ehses, J. A., Heywood, S. E., et al. (2018). Interleukin-6 delays gastric emptying in humans with direct effects on glycemic control. Cell Metab. 27, 1201.e3–1211.e3. doi: 10.1016/j.cmet.2018.04.008
Law, L. L., Rol, R. N., Schultz, S. A., Dougherty, R. J., Edwards, D. F., Koscik, R. L., et al. (2018). Moderate intensity physical activity associates with CSF biomarkers in a cohort at risk for Alzheimer’s disease. Alzheimers Dement. 10, 188–195. doi: 10.1016/j.dadm.2018.01.001
Li, D. J., Li, Y. H., Yuan, H. B., Qu, L. F., and Wang, P. (2017). The el exercise-induced hormone irisin protects against neuronal injury via activation of the Akt and ERK1/2 signaling pathways and contributes to the neuroprotection of physical exercise in cerebral ischemia. Metabolism 68, 31–42. doi: 10.1016/j.metabol.2016.12.003
Lin, X., Zhang, X., Guo, J., Roberts, C. K., McKenzie, S., Wu, W. C., et al. (2015). Effects of exercise training on cardiorespiratory fitness and biomarkers of cardiometabolic health: a systematic review and meta-analysis of randomized controlled trials. J. Am. Heart Assoc. 4:e002014. doi: 10.1161/JAHA.115.002014
Liu, D., Zhang, Y., Gharavi, R., Park, H. R., Lee, J., Siddiqui, S., et al. (2015). The mitochondrial uncoupler DNP triggers brain cell mTOR signaling network reprogramming and CREB pathway up-regulation. J. Neurochem. 134, 677–692. doi: 10.1111/jnc.13176
Loprinzi, P. D. (2015). Dose-response association of moderate-to-vigorous physical activity with cardiovascular biomarkers and all-cause mortality: considerations by individual sports, exercise and recreational physical activities. Prev. Med. 81, 73–77. doi: 10.1016/j.ypmed.2015.08.014
Maekawa, T., Ogasawara, R., Tsutaki, A., Lee, K., Nakada, S., Nakazato, K., et al. (2018). Electrically evoked local muscle contractions cause an increase in hippocampal BDNF. Appl. Physiol. Nutr. Metab. 43, 491–496. doi: 10.1139/apnm-2017-0536
Mao, W., Yu, X. X., Zhong, A., Li, W., Brush, J., Sherwood, S. W., et al. (1999). UCP4, a el brain-specific mitochondrial protein that reduces membrane potential in mammalian cells. FEBS Lett. 443, 326–330. doi: 10.1016/S0014-5793(98)01713-X
Marosi, K., and Mattson, M. P. (2014). BDNF mediates adaptive brain and body responses to energetic challenges. Trends Endocrinol. Metab. 25, 89–98. doi: 10.1016/j.tem.2013.10.006
Marques-Aleixo, I., Santos-Alves, E., Balca, M. M., Moreira, P. I., Oliveira, P. J., Magalhaes, J., et al. (2016). Physical exercise mitigates doxorubicin-induced brain cortex and cerebellum mitochondrial alterations and cellular quality control signaling. Mitochondrion 26, 43–57. doi: 10.1016/j.mito.2015.12.002
Marques-Aleixo, I., Santos-Alves, E., Balca, M. M., Rizo-Roca, D., Moreira, P. I., Oliveira, P. J., et al. (2015). Physical exercise improves brain cortex and cerebellum mitochondrial bioenergetics and alters apoptotic, dynamic and auto(mito)phagy markers. Neuroscience 301, 480–495. doi: 10.1016/j.neuroscience.2015.06.027
Mazur-Bialy, A. I., Pochec, E., and Zarawski, M. (2017). Anti-inflammatory properties of irisin, mediator of physical activity, are connected with TLR4/MyD88 signaling pathway activation. Int. J. Mol. Sci. 18:E701. doi: 10.3390/ijms18040701
McAuley, P., Pittsley, J., Myers, J., Abella, J., and Froelicher, V. F. (2009). Fitness and fatness as mortality predictors in healthy older men: the veterans exercise testing study. J. Gerontol. A Biol. Sci. Med. Sci. 64, 695–699. doi: 10.1093/gerona/gln039
Melanson, E. L., Keadle, S. K., Donnelly, J. E., Braun, B., and King, N. A. (2013). Resistance to exercise-induced weight loss: compensatory behavioral adaptations. Med. Sci. Sports Exerc. 45, 1600–1609. doi: 10.1249/MSS.0b013e31828ba942
Moon, H. S., Dincer, F., and Mantzoros, C. S. (2013). Pharmacological concentrations of irisin increase cell proliferation without influencing markers of neurite outgrowth and synaptogenesis in mouse H19-7 hippocampal cell lines. Metabolism 62, 1131–1136. doi: 10.1016/j.metabol.2013.04.007
Mozaffarian, D., Hao, T., Rimm, E. B., Willett, W. C., and Hu, F. B. (2011). Changes in diet and lifestyle and long-term weight gain in women and men. N. Engl. J. Med. 364, 2392–2404. doi: 10.1056/NEJMoa1014296
Murawska-Cialowicz, E., Wojna, J., and Zuwala-Jagiello, J. (2015). Crossfit training changes brain-derived neurotrophic factor and irisin levels at rest, after wingate and progressive tests, and improves aerobic capacity and body composition of young physically active men and women. J. Physiol. Pharmacol. 66, 811–821.
Papp, C., Pak, K., Erdei, T., Juhasz, B., Seres, I., Szentpeteri, A., et al. (2017). Alteration of the irisin-brain-derived neurotrophic factor axis contributes to disturbance of mood in COPD patients. Int. J. Chron. Obstruct. Pulmon. Dis. 12, 2023–2033. doi: 10.2147/COPD.S135701
Patterson, R., McNamara, E., Tainio, M., de Sá, T. H., Smith, A. D., Sharp, S. J., et al. (2018). Sedentary behaviour and risk of all-cause, cardiovascular and cancer mortality, and incident type 2 diabetes: a systematic review and dose response meta-analysis. Eur. J. Epidemiol. 33, 811–829. doi: 10.1007/s10654-018-0380-1
Pecqueur, C., Alves-Guerra, M. C., Gelly, C., Levi-Meyrueis, C., Couplan, E., Collins, S., et al. (2001). Uncoupling protein 2, in vivo distribution, induction upon oxidative stress, and evidence for translational regulation. J. Biol. Chem. 276, 8705–8712. doi: 10.1074/jbc.M006938200
Piya, M. K., Harte, A. L., Sivakumar, K., Tripathi, G., Voyias, P. D., James, S., et al. (2014). The identification of irisin in human cerebrospinal fluid: influence of adiposity, metabolic markers, and gestational diabetes. Am. J. Physiol. Endocrinol. Metab. 306, E512–E518. doi: 10.1152/ajpendo.00308.2013
Pontzer, H. (2018). Energy constraint as a el mechanism linking exercise and health. Physiology 33, 384–393. doi: 10.1152/physiol.00027.2018
Qiu, S., Cai, X., Yin, H., Sun, Z., Zügel, M., Steinacker, J. M., et al. (2018). Exercise training and endothelial function in patients with type 2 diabetes: a meta-analysis. Cardiovasc. Diabetol. 17:64. doi: 10.1186/s12933-018-0711-2
Radak, Z., Hart, N., Sarga, L., Koltai, E., Atalay, M., Ohno, H., et al. (2010). Exercise plays a preventive role against Alzheimer’s disease. J. Alzheimers Dis. 20, 777–783. doi: 10.3233/JAD-2010-091531
Raefsky, S. M., and Mattson, M. P. (2017). Adaptive responses of neuronal mitochondria to bioenergetic challenges: roles in neuroplasticity and disease resistance. Free Radic. Biol. Med. 102, 203–216. doi: 10.1016/j.freeradbiomed.2016.11.045
Ribeiro, F., Ribeiro, I. P., Gonçalves, A. C., Alves, A. J., Melo, E., Fernandes, R., et al. (2017). Effects of resistance exercise on endothelial progenitor cell mobilization in women. Sci. Rep. 7:17880. doi: 10.1038/s41598-017-18156-6
Richard, D., Rivest, R., Huang, Q., Bouillaud, F., Sanchis, D., Champigny, O., et al. (1998). Distribution of the uncoupling protein 2 mRNA in the mouse brain. J. Comp. Neurol. 397, 549–560. doi: 10.1002/(SICI)1096-9861(19980810)397:4<549::AID-CNE7>3.0.CO;2-1
Ricquier, D., and Bouillaud, F. (2000). Mitochondrial uncoupling proteins: from mitochondria to the regulation of energy balance. J. Physiol. 529(Pt 1), 3–10. doi: 10.1111/j.1469-7793.2000.00003.x
Roca-Rivada, A., Castelao, C., Senin, L. L., Landrove, M. O., Baltar, J., Belen Crujeiras, A., et al. (2013). FNDC5/irisin is not only a myokine but also an adipokine. PLoS One 8:e60563. doi: 10.1371/journal.pone.0060563
Safdar, A., Saleem, A., and Tarnopolsky, M. A. (2016). The potential of endurance exercise-derived exosomes to treat metabolic diseases. Nat. Rev. Endocrinol. 12, 504–517. doi: 10.1038/nrendo.2016.76
Same, R. V., Feldman, D. I., Shah, N., Martin, S. S., Al Rifai, M., Blaha, M. J., et al. (2016). Relationship between sedentary behavior and cardiovascular risk. Curr. Cardiol. Rep. 18:6. doi: 10.1007/s11886-015-0678-5
Schuch, F., Vancampfort, D., Firth, J., Rosenbaum, S., Ward, P., Reichert, T., et al. (2017). Physical activity and sedentary behavior in people with major depressive disorder: a systematic review and meta-analysis. J. Affect. Disord. 210, 139–150. doi: 10.1016/j.jad.2016.10.050
Shen, X., Wong-Yu, I. S., and Mak, M. K. (2016). Effects of exercise on falls, balance, and gait ability in Parkinson’s disease: a meta-analysis. Neurorehabil. Neural Repair 30, 512–527. doi: 10.1177/1545968315613447
Simon-Areces, J., Dietrich, M. O., Hermes, G., Garcia-Segura, L. M., Arevalo, M. A., and Horvath, T. L. (2012). UCP2 induced by natural birth regulates neuronal differentiation of the hippocampus and related adult behavior. PLoS One 7:e42911. doi: 10.1371/journal.pone.0042911
Siteneski, A., Cunha, M. P., Lieberknecht, V., Pazini, F. L., Gruhn, K., Brocardo, P. S., et al. (2018). Central irisin administration affords antidepressant-like effect and modulates neuroplasticity-related genes in the hippocampus and prefrontal cortex of mice. Prog. Neuropsychopharmacol. Biol. Psychiatry 84, 294–303. doi: 10.1016/j.pnpbp.2018.03.004
Stout, A. K., Raphael, H. M., Kanterewicz, B. I., Klann, E., and Reynolds, I. J. (1998). Glutamate-induced neuron death requires mitochondrial calcium uptake. Nat. Neurosci. 1, 366–373. doi: 10.1038/1577
Szilasi, M. E., Pak, K., Kardos, L., Varga, V. E., Seres, I., Mikaczo, A., et al. (2017). The alteration of irisin-brain-derived neurotrophic factor axis parallels severity of distress disorder in bronchial asthma patients. Front. Neurosci. 11:653. doi: 10.3389/fnins.2017.00653
Teshima, Y., Akao, M., Jones, S. P., and Marbán, E. (2003). Uncoupling protein-2 overexpression inhibits mitochondrial death pathway in cardiomyocytes. Circ. Res. 93, 192–200. doi: 10.1161/01.RES.0000085581.60197.4D
Thomas, D. M., Bouchard, C., Church, T., Slentz, C., Kraus, W. E., Redman, L. M., et al. (2012). Why do individuals not lose more weight from an exercise intervention at a defined dose? An energy balance analysis. Obes. Rev. 13, 835–847. doi: 10.1111/j.1467-789X.2012.01012.x
Toda, C., Kim, J. D., Impellizzeri, D., Cuzzocrea, S., Liu, Z. W., and Diano, S. (2016). UCP2 regulates mitochondrial fission and ventromedial nucleus control of glucose responsiveness. Cell 164, 872–883. doi: 10.1016/j.cell.2016.02.010
Torma, F., Bori, Z., Koltai, E., Felszeghy, K., Vacz, G., Koch, L., et al. (2014). Eating habits modulate short term memory and epigenetical regulation of brain derived neurotrophic factor in hippocampus of low- and high running capacity rats. Brain Res. Bull. 107, 54–60. doi: 10.1016/j.brainresbull.2014.07.003
Vaynman, S., Ying, Z., Wu, A., and Gomez-Pinilla, F. (2006). Coupling energy metabolism with a mechanism to support brain-derived neurotrophic factor-mediated synaptic plasticity. Neuroscience 139, 1221–1234. doi: 10.1016/j.neuroscience.2006.01.062
Wang, D., Zhai, X., Chen, P., Yang, M., Zhao, J., Dong, J., et al. (2014). Hippocampal UCP2 is essential for cognition and resistance to anxiety but not required for the benefits of exercise. Neuroscience 277, 36–44. doi: 10.1016/j.neuroscience.2014.06.060
Wei, M., Kampert, J. B., Barlow, C. E., Nichaman, M. Z., Gibbons, L. W., Paffenbarger, R. S. Jr., et al. (1999). Relationship between low cardiorespiratory fitness and mortality in normal-weight, overweight, and obese men. JAMA 282, 1547–1553. doi: 10.1001/jama.282.16.1547
Wei, Z., Chigurupati, S., Bagsiyao, P., Henriquez, A., and Chan, S. L. (2009). The brain uncoupling protein UCP4 attenuates mitochondrial toxin-induced cell death: role of extracellular signal-regulated kinases in bioenergetics adaptation and cell survival. Neurotox. Res. 16, 14–29. doi: 10.1007/s12640-009-9039-8
World Health Organization (2010). Global Recommendations on Physical Activity for Health. Available at: http://whqlibdoc.who.int/publications/2010/9789241599979_eng.pdf
World Health Organization (2000). Obesity: Preventing and Managing the Global Epidemic: Report of a WHO Consultation. World Health Organ Tech Rep Ser. 894. Geneva: World Health Organization, 1–253.
Wrann, C. D. (2015). FNDC5/irisin - their role in the nervous system and as a mediator for beneficial effects of exercise on the brain. Brain Plast. 1, 55–61. doi: 10.3233/BPL-150019
Wrann, C. D., White, J. P., Salogiannnis, J., Laznik-Bogoslavski, D., Wu, J., Ma, D., et al. (2013). Exercise induces hippocampal BDNF through a PGC-1alpha/FNDC5 pathway. Cell Metab. 18, 649–659. doi: 10.1016/j.cmet.2013.09.008
Wu, R. M., Sun, Y. Y., Zhou, T. T., Zhu, Z. Y., Zhuang, J. J., Tang, X., et al. (2014). Arctigenin enhances swimming endurance of sedentary rats partially by regulation of antioxidant pathways. Acta Pharmacol. Sin. 35, 1274–1284. doi: 10.1038/aps.2014.70
Xu, B. (2013). BDNF (I)rising from exercise. Cell Metab. 18, 612–614. doi: 10.1016/j.cmet.2013.10.008
Xu, Q., Park, Y., Huang, X., Hollenbeck, A., Blair, A., Schatzkin, A., et al. (2010). Physical activities and future risk of Parkinson disease. Neurology 75, 341–348. doi: 10.1212/WNL.0b013e3181ea1597
Zhang, J., and Zhang, W. (2016). Can irisin be a linker between physical activity and brain function? Biomol. Concepts 7, 253–258. doi: 10.1515/bmc-2016-0012
Zhang, Q., Wu, Y., Sha, H., Zhang, P., Jia, J., Hu, Y., et al. (2012). Early exercise affects mitochondrial transcription factors expression after cerebral ischemia in rats. Int. J. Mol. Sci. 13, 1670–1679. doi: 10.3390/ijms13021670
Keywords: physical exercise, mitochondria, irisin, FNDC5, PGC-1α, UCP2
Citation: de Oliveira Bristot VJ, de Bem Alves AC, Cardoso LR, da Luz Scheffer D and Aguiar AS Jr (2019) The Role of PGC-1α/UCP2 Signaling in the Beneficial Effects of Physical Exercise on the Brain. Front. Neurosci. 13:292. doi: 10.3389/fnins.2019.00292
Received: 29 September 2018; Accepted: 13 March 2019;
Published: 29 March 2019.
Edited by:
Sebastien Talbot, Université de Montréal, CanadaReviewed by:
Sung Ung Kang, Johns Hopkins University, United StatesMatthew Lawrence Goodwin, Johns Hopkins Medicine, United States
Copyright © 2019 de Oliveira Bristot, de Bem Alves, Cardoso, da Luz Scheffer and Aguiar. This is an open-access article distributed under the terms of the Creative Commons Attribution License (CC BY). The use, distribution or reproduction in other forums is permitted, provided the original author(s) and the copyright owner(s) are credited and that the original publication in this journal is cited, in accordance with accepted academic practice. No use, distribution or reproduction is permitted which does not comply with these terms.
*Correspondence: Aderbal Silva Aguiar Jr., aderbal.aguiar@ufsc.br; aderbalaguiar@gmail.com