- 1Department of Cellular and Integrative Physiology, University of Texas Health Science Center at San Antonio, San Antonio, TX, United States
- 2Department of Pharmacology, University of Texas Health Science Center at San Antonio, San Antonio, TX, United States
Depression is a serious public health concern. Many patients are not effectively treated, but in children and adolescents this problem is compounded by limited pharmaceutical options. Currently, the Food and Drug Administration approves only two antidepressants for use in these young populations. Both are selective serotonin reuptake inhibitors (SSRIs). Compounding matters further, they are therapeutically less efficacious in children and adolescents than in adults. Here, we review clinical and preclinical literature describing the antidepressant efficacy of SSRIs in juveniles and adolescents. Since the high-affinity serotonin transporter (SERT) is the primary target of SSRIs, we then synthesize these reports with studies of SERT expression/function during juvenile and adolescent periods. Preclinical literature reveals some striking parallels with clinical studies, primary among them is that, like humans, juvenile and adolescent rodents show reduced antidepressant-like responses to SSRIs. These findings underscore the utility of preclinical assays designed to screen drugs for antidepressant efficacy across ages. There is general agreement that SERT expression/function is lower in juveniles and adolescents than in adults. It is well established that chronic SSRI treatment decreases SERT expression/function in adults, but strikingly, SERT expression/function in adolescents is increased following chronic treatment with SSRIs. Finally, we discuss a putative role for organic cation transporters and/or plasma membrane monoamine transporter in serotonergic homeostasis in juveniles and adolescents. Taken together, fundamental differences in SERT, and putatively in other transporters capable of serotonin clearance, may provide a mechanistic basis for the relative inefficiency of SSRIs to treat pediatric depression, relative to adults.
Child and Adolescent Depression: Overview
Major depressive disorder, one of the most common forms of depression, affects individuals of all ages with approximately 5.7% of children and 11.3% of adolescents experiencing major depressive episodes (Fuhrmann et al., 2014; Mojtabai et al., 2016). Hallmarks of major depressive disorder include feelings of sadness for long periods, anhedonia, significant increases or decreases in weight, sleeping disturbances, psychomotor retardation or agitation, lethargy, feelings of helplessness and hopelessness, difficulty concentrating and/or decision making, and suicidal thoughts (American Psychiatric Association, 2013). Diagnosis of major depressive disorder is based on an individual having at least five of these symptoms for two or more weeks. However, symptoms of depression do not present themselves the same in all ages. Children and adolescents have a more pernicious onset of symptoms and experience more irritability than adults (Hazell, 2009).
Depression is a serious public health concern. Many patients are not effectively treated, but in children and adolescents this problem is compounded by limited pharmaceutical options (for review, see Bylund and Reed, 2007). Only two selective serotonin reuptake inhibitors (SSRIs), fluoxetine (Prozac), and escitalopram (Lexapro), are currently approved by the United States Food and Drug Administration (FDA) for use in these young populations. However, children and adolescents are less effectively treated by these antidepressants compared with adults, further exacerbating the situation (Tsapakis et al., 2008; Hetrick et al., 2010, 2012). Moreover, treatment response to escitalopram is especially poor in children and adolescents carrying a common variant of the serotonin transporter (SERT) gene, which confers reduced SERT functionality (Kronenberg et al., 2007). Given the high prevalence of adolescent depression, affecting 4–8% of the population with a potential lifetime incidence as high as 25% (Kessler et al., 2001; Cheung et al., 2005; Bujoreanu et al., 2011; Thapar et al., 2012; Maughan et al., 2013) and, of great concern, the high incidence of suicide in this young population (“the third leading cause of death in the 15–19 years age group”; Reed et al., 2008), there is a clear and urgent need to establish the neural mechanisms underlying these differences between children and adolescents on the one hand and adults on the other, with the goal to discover novel targets for the development of improved treatments.
In spite of numerous reports documenting that the antidepressant response of children and adolescents is less than that of adults (Hazell et al., 2002; Bylund and Reed, 2007; Tsapakis et al., 2008; Hetrick et al., 2010, 2012), studies investigating the underlying mechanisms are lacking. That the brain is still undergoing rapid development during childhood and adolescent periods (Lenroot and Giedd, 2006) no doubt contributes, at least in part, to different treatment responses between children and adolescents, and adults. However, the neural reasons for the age-dependency of antidepressant response remain poorly understood. SSRIs, the most commonly prescribed antidepressants, and the only class approved for children and adolescents, act by inhibiting serotonin uptake via SERT, the high-affinity transporter for serotonin. This focused review aims to synthesize current clinical and preclinical literature investigating the utility of the SSRI class of antidepressant in the treatment of childhood and adolescent depression, together with what is known about SERT expression and function during these developmental periods. Importantly, we discuss findings from preclinical studies that are beginning to shed light on putative mechanisms accounting for the relatively poor response of children and adolescents to SSRIs relative to adults.
Available Treatments
The safety and effectiveness of many antidepressants for use in children and adolescents have been extensively studied and reviewed (e.g., Maneeton and Srisurapanont, 2000; Hazell et al., 2002; Courtney, 2004; Hazell, 2004; Whittington et al., 2004; Bhatia and Bhatia, 2007). Fluoxetine is FDA approved for children and adolescents as young as 8 years and older, and escitalopram is approved for children and adolescents age 12 and older. In addition to these, other antidepressants can be prescribed off label. For example, sertraline and fluvoxamine (also SSRIs) are approved to treat obsessive compulsive disorder in children and adolescents and therefore can be prescribed “off label” to treat depression in these young populations (Kodish et al., 2011). The numbers of prescriptions for antidepressants continues to increase in individuals over the age of six with approximately 3% of individuals under 18 years of age being prescribed an antidepressant (Kafali et al., 2018).
Whether these treatments yield clinical benefits remains controversial (see Table 1). For example, fluoxetine was originally found to be effective in treating children and adolescents with major depressive disorder (Emslie et al., 1997, 2002), but using fluoxetine as a positive control in later studies examining the efficacy of other SSRIs, the same investigators found no difference between fluoxetine and placebo treated groups (Atkinson et al., 2014; Emslie et al., 2014). As summarized in Table 1, randomized controlled trials conducted in children and adolescents have yielded variable results across studies and types of SSRIs, with roughly equal numbers reporting therapeutic benefit versus no difference from placebo. These mixed results are likely attributable, at least in part, to differences in population samples and symptomology scales used, with no clear pattern emerging with regard to dose or duration of treatment. In turn, this highlights a need to refine and standardize the diagnostic criterion for depression in juveniles and adolescents, which likely differs from that used to assess depression in adults. Regardless, based on current clinical literature it is difficult to conclude whether or not SSRIs are beneficial in the treatment of depressive disorders in children and adolescents. To this end, preclinical studies are beginning to provide insights into the utility of SSRIs as antidepressants in these young populations.
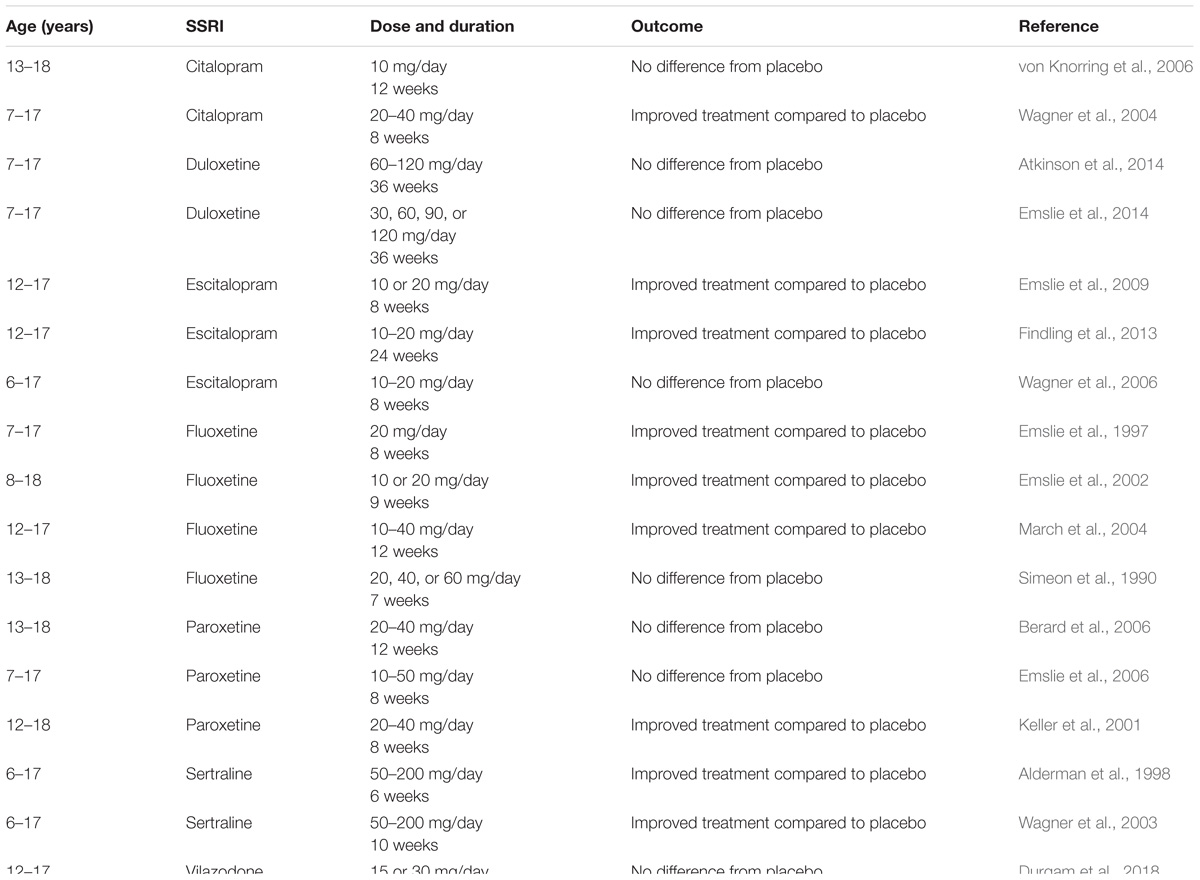
Table 1. Clinical studies examining the efficacy of antidepressant medications in children and adolescents.
Lessons Learned From Preclinical Studies
Preclinical studies using murine models afford well-controlled conditions to study normal brain development, as well as the neural and behavioral effects of SSRIs, and other classes of antidepressants, in juveniles and adolescents. While prior to 2008 only a handful of studies had examined antidepressant-like activity of SSRIs in adolescent rodents (and none in juveniles), research in this arena has been steadily increasing during the past decade (see Table 2). These studies are discussed in the following sections.
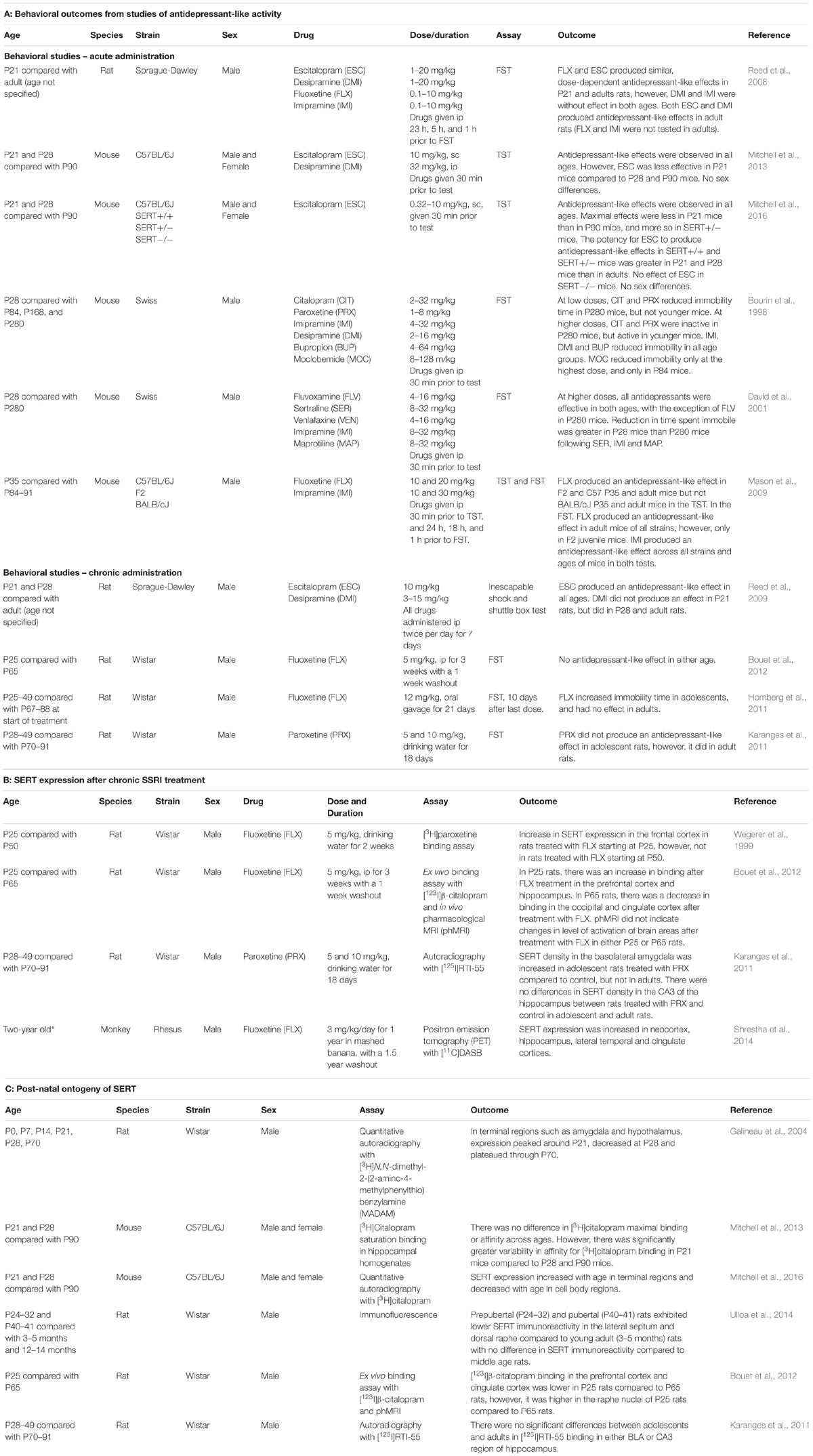
Table 2. Preclinical studies examining the antidepressant-like effects of SSRIs (2A), effect of chronic SSRI treatment on SERT expression (2B), and age-dependent shifts in SERT expression (2C) in juvenile and adolescent rodents, compared to adults.
Antidepressant-Like Efficacy of SSRIs in Juvenile and Adolescent Rodents
In rodents, postnatal days (P) 21–27 are considered the juvenile period, P28–42 early adolescence and P44–56 late adolescence (Spear, 2000; Bylund and Reed, 2007; Hefner and Holmes, 2007; Pechnick et al., 2008; Reed et al., 2008). Although there are still relatively few publications investigating antidepressant-like efficacy of SSRIs in juvenile and adolescent rodents, the literature is growing, and revealing some interesting patterns, discussed below (Table 2).
Acute Antidepressant-Like Effects of SSRIs
Studies examining the acute effects of antidepressant drugs have used the forced swim test (FST) and/or tail suspension test (TST), the gold standards for assaying antidepressant-like activity in rodents (Cryan and Holmes, 2005; Castagné et al., 2011). While there are some inconsistencies among reports (Table 2A), the most common finding is that SSRIs are less effective in juvenile and adolescent rodents, than in adults (Bourin et al., 1998; Mason et al., 2009; Mitchell et al., 2013, 2017). This is in line with clinical reports (Tsapakis et al., 2008; Hetrick et al., 2010, 2012), and underscores the utility of these preclinical tests as predictors of antidepressant-like efficacy in humans.
Interestingly, Mason et al. (2009) found that age-dependent differences in antidepressant-like response were also dependent on mouse strain and test used. For example, in the TST fluoxetine (20 mg/kg) produced an antidepressant-like effect in juvenile (P35) and adult C57BL/6J and F2 mice, but not in BALB/C mice. However, when using the FST, juvenile and adult C57BL/6 mice were insensitive to fluoxetine, but F2 and BALB/C mice responded. This is not surprising in view of elegant studies from Lucki’s group using adult mice to show clear strain-dependent differences in response to fluoxetine in the FST (Lucki et al., 2001) and to citalopram in the TST (Crowley et al., 2005). Regardless, in the Mason study, when antidepressant effects were detectable they were less in juveniles than in adults when using the FST, and less than or equivalent to those of adults when using the TST.
While the majority of studies have used mice, one study used rats (Reed et al., 2008). Using the FST, these investigators found that the SSRIs fluoxetine and escitalopram produced antidepressant-like effects that were of a similar magnitude in P21 and adult rats (Table 2A). Interestingly, they found that the tricyclic antidepressants, desipramine and imipramine, were ineffective in P21 rats, but produced robust antidepressant-like effects in adults. While the mechanistic underpinnings for these results are yet to be determined, a common view is that delayed maturation of the norepinephrine neurotransmitter system relative to serotonin is a factor (Bylund and Reed, 2007). However, in contrast to the rat study, mouse studies generally found tricyclic antidepressants to be either equally effective in producing antidepressant-like effects in the TST and FST in juveniles, adolescents, and adults (Bourin et al., 1998; Mason et al., 2009; Mitchell et al., 2013), or more efficacious in juveniles and adolescents than in adults (David et al., 2001; Mitchell et al., 2017), suggesting that in mice, the norepinephrine neurotransmitter system matures faster than in the rat. In humans, tricyclics are not prescribed for children and adolescents due to poor tolerability (e.g., vertigo, tremors, low blood pressure, and dry mouth; Andersen et al., 2009; Dell’Osso et al., 2011; Hazell and Mirzaie, 2013). However, preclinical findings from mouse studies indicate that the utility of NET, and/or dual NET and SERT blockers in the treatment of juvenile and adolescent depression warrants further investigation. It is possible that the development of new NET (and NET/SERT) targeting drugs that have fewer side effects than currently available drugs may be better suited to the treatment of pediatric depression (Mitchell et al., 2017).
Clearly, differing rates of development of noradrenergic and serotonin systems, and the development of systems that impinge on them (e.g., histamine, see Munari et al., 2015) may also factor into the magnitude of antidepressant-response as a function of age. Rates of maturation also differ among species, and is a consideration when relating findings from rodents to outcomes in humans. The reader is directed to an excellent review by Murrin et al. (2007) for information on differential rates of development of noradrenergic and serotonergic systems among species. While studies in human are relatively scant, those that exist, together with studies in non-human primates, suggest that the serotonin system matures faster than the noradrenergic system (Murrin et al., 2007). It will be of interest for future studies to precisely map treatment response to different classes of antidepressant on developmental stage of the noradrenergic and serotonergic systems in humans.
Lastly, one study included constitutive SERT heterozygote mice as a murine model of humans carrying a common variant of the SERT gene, which confers reduced SERT functionality, and who respond less well to SSRIs than those without this variant (Kronenberg et al., 2007). Consistent with clinical literature, the maximal effect of escitalopram in juvenile SERT heterozygote mice was less than that in juvenile SERT wildtype mice. Moreover, in both SERT genotypes the maximal effect in juveniles was less than that in adults (Mitchell et al., 2016). These findings again underscore the utility of preclinical models of antidepressant-like activity in probing mechanisms contributing to age- and genotype-dependent responses to antidepressants (but see section “Caveats to Currently Available Behavioral Assays for Identifying Drugs With Antidepressant Potential”).
In sum, the most common finding from studies of the acute antidepressant-like effects of SSRIs in preclinical studies is that they are less effective in juveniles and adolescents than in adults, though some studies do report no difference in response among ages. With proper attention to mouse strain, and behavioral test employed, such preclinical models provide valuable tools to study the mechanistic basis for SSRIs being less effective in treating pediatric depression than in adults, as will be discussed further.
Chronic Antidepressant-Like Effects
While the TST and FST are currently the best tests available to quickly screen for drugs with antidepressant potential, for drugs to be useful clinically they must be effective after chronic administration. Numerous groups have reported antidepressant-like effects following chronic SSRI treatment in adult mice and rats, using tests such as the FST, TST, and novelty-induced hypophagia (Detke et al., 1997; Dulawa et al., 2004; Cryan et al., 2005; Holick et al., 2008; Miller et al., 2008; Balu et al., 2009; Jiao et al., 2011; Roohi-Azizi et al., 2018), with only one group reporting no effect (Griebel et al., 1999). However, only four studies have examined the antidepressant-like effect of chronic SSRI administration in juveniles and/or adolescents. All have used rats (Table 2A). Three used the FST, and though different SSRIs were used (fluoxetine in two studies [Homberg et al., 2011; Bouet et al., 2012] and paroxetine in the other [Karanges et al., 2011]), the overall findings were in general agreement. In the two studies where there was no washout period, chronic SSRI treatment either had no effect in young rats (Karanges et al., 2011), or increased immobility time (i.e., pro-depressive-like behavior; Homberg et al., 2011), whereas in adults there was either no effect (Homberg et al., 2011), or a robust antidepressant-like effect (Karanges et al., 2011), in agreement with published literature using adult rodents. These results are consistent with clinical literature reporting that children and adolescents respond poorly to SSRIs compared with adults, if at all (Tsapakis et al., 2008; Hetrick et al., 2010, 2012). In the one study where a 1-week washout period was allowed before testing in the FST, no antidepressant-like effect of fluoxetine was observed in either juvenile/adolescent or adult rats (Bouet et al., 2012).
Another study utilized inescapable shock and shuttle box tests to assay antidepressant-like activity following a week of twice daily injections of escitalopram or desipramine in rats. Escitalopram produced similar antidepressant-like effects in juveniles, adolescents, and adults, but desipramine was only effective in adolescent and adult rats, and ineffective in juvenile rats (Reed et al., 2009). This result is consistent with findings reported by the same group following acute administration of these drugs (Reed et al., 2008; see discussion above).
Overall, results from studies examining the chronic effects of SSRIs in rats again support the utility of preclinical assays such as these. However, given that mice seem to more reliably parallel human clinical literature, at least in the acute tests described above, it will be of interest to implement mice in studies of chronic SSRI administration as well.
Caveats to Currently Available Behavioral Assays for Identifying Drugs With Antidepressant Potential
Though the FST and TST are the current gold standards for assaying antidepressant-like activity in rodents (Cryan and Holmes, 2005), it is important to note that while the vast majority of antidepressant drugs reduce immobility time in these tests, not all drugs that reduce immobility time are antidepressants. Thus, these tests are valuable when comparing the sensitivity to drugs across ages, but they do not necessarily predict therapeutic effectiveness in the treatment of depression. In this realm, the field is in need of new tests (be they behavioral or biochemical) that can reliably predict therapeutic efficacy of novel drugs for the treatment of depression, while screening out false positives.
In addition, to date studies of juvenile and adolescent rodents in tests for antidepressant-like activity of drugs have used only naïve animals. Ideally, such tests should be performed in animal models of depression, such as those afforded by unpredictable chronic mild stress, or social defeat paradigms, to name a few (see O’Leary and Cryan, 2013 for review). While these models have been frequently applied to studies of depression in adult rodents, their application to juveniles (in particular), as well as adolescents, is impractical due to the shortness of these developmental periods (e.g., 7-day juvenile period, 14-day early adolescent, and 14-day late adolescent periods; Spear, 2000; Bylund and Reed, 2007; Hefner and Holmes, 2007; Pechnick et al., 2008; Reed et al., 2008). This is further compounded when the effects of chronic drug treatment need to be studied. While it is not within the scope of this focused review to discuss the pros and cons of existing models for depression research, the reader should be aware that there are limitations to those currently available (see O’Leary and Cryan, 2013 for review). Of course, recapitulating the heterogeneous nature of human depression, without yet fully understanding the etiologies of this complex disorder, makes developing improved animal models a challenging undertaking. However, as we learn more about the complexities of this disorder, new knowledge can be applied to the development of improved models to study depression and to screen novel compounds for therapeutic efficacy in its treatment. Importantly, models that can be applied to the study of juveniles and adolescents are sorely needed.
Expression and Function of SERT in Juvenile and Adolescent Rodents
Understanding how SERT expression and function varies across juvenile, adolescent and adult ages, and how chronic treatment with SSRIs influences SERT expression and function across these ages may lend insight into age-dependent differences in antidepressant-like responses to SSRIs. Studies investigating this subject are discussed below.
Effect of Chronic SSRI Treatment on SERT Expression and Function
In adult rodents, chronic treatment with SSRIs downregulates SERT expression (Benmansour et al., 1999; Gould et al., 2003, 2007; Mirza et al., 2007; Lin et al., 2017), which is associated with putatively reduced functionality of SERT, as demonstrated by in vivo chronoamperometry studies measuring clearance of serotonin from extracellular fluid in hippocampus (Benmansour et al., 1999). These decreases were not associated with reduced SERT gene expression or neurotoxicity (Benmansour et al., 1999). Several lines of evidence suggest that SSRI-induced downregulation of SERT function is attributable, at least in part, to internalization of SERT to the cytosolic compartment. For example, in vitro studies using Caco-2 cells transfected with human (h) SERT show that long-term exposure to fluoxetine causes internalization of hSERT, leaving less hSERT on the plasma membrane (Iceta et al., 2007). These studies showed no effect of fluoxetine treatment on either total hSERT protein or mRNA. Studies in rats found that chronic, but not acute fluoxetine treatment causes internalization of SERT in both cell bodies and terminals (Descarries and Riad, 2012). Similarly, translational approaches using stem cell-derived serotonergic neurons and a transgenic mouse expressing hSERT found that citalopram dose-dependently causes internalization of hSERT in both models (Matthäus et al., 2016). Such studies underscore the utility of complementary/translational approaches to understanding antidepressant response on a cellular and molecular level. While it remains to be determined if internalization of SERT following chronic SSRI treatment occurs in humans, and is temporally synced with therapeutic benefit, studies in adult rodents, and in vitro, suggests that downregulation of SERT, putatively by trafficking away from the plasma membrane, is at least in part required for ultimate therapeutic benefit following chronic treatment with SSRIs. Moreover, that it takes chronic exposure to SSRIs for this downregulation of SERT to occur, provides a potential mechanistic basis for the delay to onset of therapeutic benefit.
Currently there is no literature reporting effects of chronic SSRI treatment in juvenile rodents. This is almost certainly due to the very narrow juvenile window in rodents (i.e., P21–27). There is remarkably sparse literature on the effect of chronic SSRI treatment on SERT expression in adolescent rodents (reviewed in Daws and Gould, 2011). However, a consistent finding is that chronic treatment with SSRIs, beginning at either P25 or P28 in rodents, increases SERT expression in a number of brain regions (Wegerer et al., 1999; Karanges et al., 2011; Bouet et al., 2012) (Table 2B). These findings in rodents are further supported by a study in juvenile rhesus macaque monkeys, which found that chronic treatment with fluoxetine increased SERT expression in several brain regions, including neocortex and hippocampus (Shrestha et al., 2014) (Table 2B). With more SERT putatively becoming available to take up serotonin as SSRI treatment continues, the increases in extracellular serotonin that are thought to be needed to trigger the downstream cascade of events leading to ultimate therapeutic benefit could be greatly diminished. Thus, the clinical implications of increased SERT expression in children and adolescents could include a need to increase dose of SSRI in order to fully occupy SERT. It is interesting to note that initiating dosing of SSRIs for pediatric depression is generally less than that for adults. For example, initiating low doses of fluoxetine and citalopram to 5- to 12-year olds is less than 10 mg/d, whereas in adults (18–64 years) the dose is less than 20 mg/d (Bushnell et al., 2016). Before the black-box warning on all antidepressants was issued by the FDA in 2004, the percent of patients initiating low dose SSRI treatment was similar across ages (∼15%), with the exception of 10- to 12-year olds where the percent was much lower (5%). In contrast, following the black-box warning the percent of patients initiating low dose SSRI (relevant to their age) increased markedly (5–9 years to ∼28%, 13–17 years to ∼38%, 18–24 years to ∼25%, 25–64 years to ∼20%, and 10–12 years to ∼11%; see Bushnell et al., 2016). In the context of the present review, this raises the possibility that increasing initial dosing in pediatric depression may be therapeutically beneficial, though clearly with this comes the risk of increased, potentially life-threatening, side-effects. It must also be kept in mind that of the clinical studies summarized in Table 1, SSRI doses much higher than these initiating low doses (e.g., 60 mg/day for fluoxetine; 200 mg/d sertraline) yielded mixed outcomes, some reporting therapeutic efficacy, and others no difference from placebo.
Clearly it is difficult to draw conclusions from existing literature. The important take home message here is that increased SERT expression following chronic SSRI treatment in children and adolescents could account, at least in part, for their therapeutic efficacy being less than that in adults. Though there are no studies of SERT in brain of children and adolescents, one study examining platelet SERT expression before and after treatment with the SSRI sertraline, provides support for this premise. Sertraline treatment was found to decrease [3H]paroxetine binding affinity. Notably increased maximal binding of [3H]paroxetine to SERT was associated with non-response (Sallee et al., 1998). Further study into the benefits of more rapidly ramping SSRI dosing in pediatric depression may therefore be warranted.
To stay within the scope of this focused review, we have discussed only the consequences of chronic SSRI treatment for their primary target, SERT. Of course this is an overly simplistic view of the neural consequences of chronic SSRI treatment in juveniles and adolescents, which has also been shown to impact glycogen synthase kinase-3 (GSK3; Beurel et al., 2012), extracellular signal-regulated protein kinase 1/2 (ERK) and cAMP response element binding protein (CREB; Alcantara et al., 2014), brain derived neurotrophic factor (BDNF) and TrkB receptors (Kozisek et al., 2008), genes associated with cell cycle and survival (Tsapakis et al., 2014), and neuroplasticity (Bastos et al., 1999; Norrholm and Ouimet, 2000; Bock et al., 2013; Klomp et al., 2014). Some of the effects of chronic SSRI treatment on these factors in juveniles and adolescents are in parallel with those reported in adults (Bastos et al., 1999), while others are not (Kozisek et al., 2008; Beurel et al., 2012; Bock et al., 2013; Alcantara et al., 2014; Klomp et al., 2014). A deeper focus on SSRI-induced changes that are specific to juveniles and adolescents will help guide future research to develop improved antidepressants for these young populations.
Expression of SERT in Juveniles, Adolescents, and Adults
Prior to 2011, remarkably little was known about the ontogeny of SERT expression during juvenile and adolescent periods (reviewed in Daws and Gould, 2011). The first paper to describe postnatal ontogeny of SERT found that in terminal regions, including amygdala and hypothalamus, expression was maximal around P21, decreased at P28, before rising again to stabilize through P70, the oldest age tested (Galineau et al., 2004; Daws and Gould, 2011). Subsequent studies of SERT expression from P21 through adulthood reported either no change across those ages (Karanges et al., 2011; Mitchell et al., 2013), or a steady increase from P21 through adulthood in terminal fields (Bouet et al., 2012; Ulloa et al., 2014; Mitchell et al., 2016), and a decrease from P21 in cell body regions (Bouet et al., 2012; Mitchell et al., 2016) (Table 2C). Of particular interest, Mitchell et al. (2013) using [3H]citalopram saturation binding assays in hippocampal homogenates, provided evidence that SERT in juveniles may be functionally less able to transport serotonin than in adults. The modest variation in results among these studies is likely due to nuanced differences in the assays used, ages studies, and species used. However, taken together current evidence suggests that SERT expression and/or function in terminal fields is lower in juveniles than in adults, whereas expression in cell bodies is greater (Table 2C).
It is difficult to speculate on how these postnatal shifts in SERT expression and function ultimately play into therapeutic responsiveness to SSRIs, but this is clearly a line of investigation that warrants further research. Likewise, it will be important to determine the relationship between receptor systems that are well-known to be influenced by SSRIs (e.g., Artigas, 2013; Kulikov et al., 2018), SERT, and therapeutic response in juveniles and adolescents.
Alternative Transporter Targets for Therapeutic Intervention in Juveniles and Adolescents
In recent times, there has been increasing recognition of transporter “promiscuity” (Daws, 2009). High-affinity monoamine transporters are not loyal to their specific monoamine, and will take up other species, sometimes with greater efficiency than their own (Daws, 2009). Moreover, there is increasing awareness of “uptake-2” transporters in monoamine clearance (Gasser and Daws, 2017a,b). These are classified as transporters with low-affinity, but high-capacity to take up monoamines, and include organic cation transporters (OCTs) and the plasma membrane monoamine transporter (PMAT).
Of the three OCT subtypes (OCT1, OCT2, OCT3), OCT2 and OCT3 are most widely expressed in brain (Amphoux et al., 2006; Vialou et al., 2008; Gasser et al., 2009; Couroussé and Gautron, 2015). PMAT is also widely expressed in brain, including limbic regions (Engel et al., 2004; Dahlin et al., 2007). Each of these transporters is capable of clearing serotonin, norepinephrine, and dopamine from extracellular fluid, albeit with varying affinities (Koepsell et al., 2007; Duan and Wang, 2010; Wang, 2016). Emerging evidence indicates that, in adult mice, OCT3 may be a novel target for the development of new antidepressants. For example, the non-selective OCT/PMAT blocker decynium-22 produced robust antidepressant-like effects in adult SERT heterozygous and knockout mice, which have upregulated OCT3 expression, but was without effect in SERT wildtype mice (Baganz et al., 2008). Subsequently, Horton et al. (2013) showed that decynium-22 augmented the antidepressant-like effect of a sub-effective dose of the SSRI fluvoxamine, and that this effect was attenuated in OCT3 knockout mice. Recently, novel decynium-22 analogs were synthesized and characterized. Unlike decynium-22, which lacks activity at SERT, NET, and DAT, analogs with activity at OCT3, and modest activity at SERT, were found to produce stand-alone antidepressant-like effects in wildtype mice. Together with the findings of Horton et al. (2013), these results support the notion that dual SERT/OCT3 blockers may be more effective antidepressants than SSRIs alone (Krause-Heuer et al., 2017; Fraser-Spears et al., 2019). In addition, studies using OCT2 knockout mice provide some evidence that OCT2 may also be a new pharmacological target for mood disorders (Bacq et al., 2012).
These findings in adult mice raise the possibility that OCTs and/or PMAT may play a mechanistic role in poor response of children and adolescents to SSRIs relative to adults. For example, based on SERT expression studies described earlier, it appears that SERT expression and perhaps function is lower in juveniles and adolescents than in adults. Putatively, serotonin clearance might be driven more by OCTs/PMAT during these post-natal periods, or perhaps these “uptake-2” transporters could be transiently expressed at greater functional levels than in adults. Though no data currently exists regarding the ontogeny of OCTs and PMAT in brain, in the periphery, OCT1 mRNA expression reaches adult levels by P15 in kidney and by P22 in liver (Alnouti et al., 2006). While it is difficult to translate these findings to changes that may occur in brain, these data support the idea that in juveniles and adolescents, OCTs and/or PMAT may already be at adult levels, or perhaps transiently expressed at higher levels. In this scenario, OCTs/PMAT could conceivably buffer any increase in serotonin following an SSRI, thereby reducing, or preventing therapeutic benefit (Daws and Gould, 2011). Studies using adult SERT heterozygote mice, which afford a murine model of humans carrying a common low expressing variant of the SERT gene, lend support to this premise. OCT3 expression is increased in these mice, presumably to compensate for reduced SERT expression/function (Baganz et al., 2008). As in humans carrying this low expressing SERT gene variant (Kronenberg et al., 2007), SERT heterozygote mice are less responsive to the antidepressant-like effect of escitalopram than SERT wildtype mice (Mitchell et al., 2016). However, SERT heterozygote mice are responsive to the antidepressant-like effect of decynium-22 (OCT/PMAT blocker), whereas SERT wildtype mice are not.
Although a role for OCTs/PMAT in antidepressant-like response of juveniles and adolescents awaits empirical verification, evidence in hand supports further investigation of these transporters in regulating homeostasis of serotonin neurotransmission (as well as that of other monoamines), during juvenile and adolescent periods.
Conclusion
This review of current literature examining clinical and preclinical antidepressant efficacy of SSRIs in juveniles and adolescents reveals many knowledge gaps. These range from a need to better understand the ontogeny of neural systems known to be involved in antidepressant action in adults (in both humans and rodents), to a need for improved preclinical models in which to study antidepressant efficacy (across all ages). Although the utility of SSRIs in the treatment of pediatric depression is not clear from clinical studies, preclinical research (in spite of its current limitations) is providing valuable insights. Results from preclinical studies examining the acute and chronic antidepressant-like effects of SSRIs share remarkable similarities with clinical outcomes, supporting the use of paradigms such as the TST and FST as valuable tools to screen novel compounds for their potential therapeutic utility in these young populations. Studies of SERT expression and function, though few, are revealing a striking dichotomy in the effect of chronic SSRI treatment on SERT expression. In adults SERT is downregulated, whereas in juveniles and adolescents, SERT is upregulated. Moreover, there is growing evidence for a role of OCTs and PMAT in regulating monoamine neurotransmission. Literature reviewed in this focal area of “transporters for serotonin” encourages further research into what appears to be fundamental differences in SERT, and putatively other transporters capable of serotonin uptake, between juvenile and adolescents on the one hand, and adults on the other. In doing so, novel targets for the improved treatment of depression in children and adolescents should be revealed.
Author Contributions
LD conceived the content. LD and MB wrote the manuscript.
Funding
This work was supported by R01 MH093320, R01 MH106978, and R21 DA046044 to LD.
Conflict of Interest Statement
The authors declare that the research was conducted in the absence of any commercial or financial relationships that could be construed as a potential conflict of interest.
References
Alcantara, L., Warren, B. L., Parise, E. M., Iñiguez, S. D., and Bolaños-Guzmán, C. A. (2014). Effects of psychotropic drugs on second messenger signaling and preference for nicotine in juvenile male mice. Psychopharmacology 231, 1479–1492. doi: 10.1007/s00213-014-3434-4
Alderman, J., Wolkow, R., Chung, M., and Johnston, H. F. (1998). Sertraline treatment of children and adolescents with obsessive-compulsive disorder or depression: pharmacokinetics, tolerability, and efficacy. J. Am. Acad. Child Adolesc. Psychiatry 37, 386–394. doi: 10.1097/00004583-199804000-00016
Alnouti, Y., Petrick, J. S., and Klaassen, C. D. (2006) Tissue distribution and ontogeny of organic cation transporters in mice. Drug Metabol. Disposit. 34, 477–482.
American Psychiatric Association (2013). Diagnostic and Statistical Manual of Mental Disorders, 5th Edn. Arlington, VA: American Psychiatric Publishing. doi: 10.1176/appi.books.9780890425596
Amphoux, A., Vialou, V., Drescher, E., Brüss, M., La Cour, C. M., and Gautron, S. (2006). Differential pharmacological in vitro properties of organic cation transporters and regional distribution in rat brain. Neuropharmacology 50, 941–952. doi: 10.1016/j.neuropharm.2006.01.005
Andersen, J., Kirstensen, A. S., Bang-Andersen, B., and Stromgaard, K. (2009). Recent advances in the understanding of the interaction of antidepressant drugs with serotonin and norepinephrine transporters. Chem. Commun. 25, 3677–3692. doi: 10.1039/b903035m
Artigas, F. (2013). Serotonin receptors involved in antidepressant effects. Pharmacol. Therap. 137, 119–131. doi: 10.1016/j.pharmthera.2012.09.006
Atkinson, S. D., Prakash, A., Zhang, Q., Pangallo, B. A., Bangs, M. E., Emslie, G. J., et al. (2014). A double-blind efficacy and safety study of duloxetine flexible dosing in children and adolescents with major depressive disorder. J. Child Adolesc. Psychopharmacol. 24, 180–189. doi: 10.1089/cap.2013.0146
Bacq, A., Balasse, L., Biala, G., Guiard, B., Gardier, A. M., Schinkel, A., et al. (2012). Organic cation transporter 2 controls brain norepinephrine and serotonin clearance and antidepressant response. Mol. Psychiatry 17, 926–939. doi: 10.1038/mp.2011.87
Baganz, N. L., Horton, R. E., Calderon, A. S., Owens, W. A., Munn, J. L., Watts, L. T., et al. (2008). Organic cation transporter 3: keeping the brake on extracellular serotonin in serotonin-transporter deficit mice. Proc. Natl. Acad. Sci. U.S.A. 105, 18976–18981. doi: 10.1073/pnas.0800466105
Balu, D. T., Hodes, G. E., Anderson, B. T., and Lucki, I. (2009). Enhanced sensitivity of the MRL/MpJ mouse to the neuroplastic and behavioral effects of chronic antidepressant treatments. Neuropsychopharmacology 34, 1764–1773. doi: 10.1038/npp.2008.234
Bastos, E. F., Marcelino, L. S., Amaral, A. R., and Serfaty, C. A. (1999). Fluoxetine-induced plasticity in the rodent visual system. Brain Res. 824, 28–35. doi: 10.1016/S0006-8993(99)01184-1
Benmansour, S., Cecchi, M., Morilak, D. A., Gerhardt, G. A., Javors, M. A., Gould, G. G., et al. (1999). Effects of chronic antidepressant treatments on serotonin transporter function, density, and mRNA level. J. Neurosci. 19, 10494–10501. doi: 10.1523/JNEUROSCI.19-23-10494.1999
Berard, R., Fong, R., Carpenter, D. J., Thomason, C., and Wilkinson, C. (2006). An international, multicenter, placebo-controlled trial of paroxetine in adolescents with major depressive disorder. J. Child Adolesc. Psychopharmacol. 16, 59–75. doi: 10.1089/cap.2006.16.59
Beurel, E., Mines, M. A., Song, L., and Jope, R. S. (2012). Glycogen synthase kinase-3 levels and phosphorylation undergo large fluctuations in mouse brain during development. Bipolar Disord. 14, 822–830. doi: 10.1111/bdi.12023
Bhatia, S. K., and Bhatia, S. C. (2007). Childhood and adolescent depression. Am. Fam. Phys. 75, 73–80.
Bock, N., Koc, E., Alter, H., Roessner, V., Becker, A., Rothenberger, A., et al. (2013). Chronic fluoxetine treatment changes S100B expression during postnatal rat brain development. J. Child Adolesc. Psychopharmacol. 23, 481–489. doi: 10.1089/cap.2011.0065
Bouet, V., Klomp, A., Freret, T., Wylezinska-Arridge, M., Lopez-Tremoleda, J., Dauphin, F., et al. (2012). Age-dependent effects of chronic fluoxetine treatment on the serotonergic system one week following treatment. Psychopharmacology 221, 321–339. doi: 10.1007/s00213-011-2580-1
Bourin, M., Colombel, M. C., Redrobe, J. P., Nizard, J., Hascoët, M., and Baker, G. B. (1998). Evaluation of efficacies of different classes of antidepressants in the forced swimming test in mice at different ages. Progr. Neuro-Psychopharmacol. Biol. Psychiatry 22, 343–351. doi: 10.1016/S0278-5846(98)00009-8
Bujoreanu, S., Benhayon, D., and Szigethy, E. (2011). Treatment of depression in children and adolescents. Pediatr. Ann. 40, 548–555. doi: 10.3928/00904481-20111007-05
Bushnell, G. A., Stürmer, T., Swanson, S. A., White, A., Azrael, D., Pate, V., et al. (2016). Dosing of selective serotonin reuptake inhibitors among children and adults before and after the FDA black-box warning. Psychiat. Serv. 67, 302–309. doi: 10.1176/appi.ps.201500088
Bylund, D. B., and Reed, A. L. (2007). Childhood and adolescent depression: why do children and adults respond differently to antidepressant drugs? Neurochemistry Int. 51, 246–253. doi: 10.1016/j.neuint.2007.06.025
Castagné, V., Moser, P., Roux, S., and Porsolt, R. D. (2011). Rodent models of depression: forced swim and tail suspension behavioral despair tests in rats and mice. Curr. Protoc. Neurosci. 8:10A. doi: 10.1002/0471142301.ns0810as55
Cheung, A. H., Emslie, G. J., and Mayes, T. L. (2005). Review of the efficacy and safety of antidepressants in youth depression. J. Child Psychol. Psychiatry 46, 735–754. doi: 10.1111/j.1469-7610.2005.01.01467
Colman, R. J., Anderson, R. M., and Johnson, S. C., Kastman, E. K., Kosmatka, K. J., Beasley, T., et al. (2009). Caloric restriction delays disease onset and mortality in rhesus monkeys. Science 325, 201–204. doi: 10.1126/science.1173635
Couroussé, T., and Gautron, S. (2015). Role of organic cation transporters (OCTs) in the brain. Pharmacol. Toxicol. 146, 94–103. doi: 10.1016/j.pharmthera.2014.09.008
Courtney, D. B. (2004). Selective serotonin reuptake inhibitor and venlafaxine use in children and adolescents with major depressive disorder: a systematic review of published randomized controlled trials. Can. J. Psychiatry 49, 557–563. doi: 10.1177/070674370404900807
Crowley, J. J., Blendy, J. A., and Lucki, I. (2005). Strain-dependent antidepressant-like effects of citalopram in the mouse tail suspension test. Psychopharmacology 183, 257–264. doi: 10.1007/s00213-005-0166-5
Cryan, J. F., and Holmes, A. (2005). The ascent of mouse: advances in modelling human depression and anxiety. Nat. Rev. Drug Discov. 4, 775–790. doi: 10.1038/nrd1825
Cryan, J. F., Page, M. E., and Lucki, I. (2005). Differential behavioral effects of the antidepressants reboxetine, fluoxetine, and moclobemide in a modified forced swim test following chronic treatment. Psychopharmacology 182, 335–344. doi: 10.1007/s00213-005-0093-5
Dahlin, A., Xia, L., Kong, W., Hevner, R., and Wang, J. (2007). Expression and immunolocalization of the plasma membrane monoamine transporter in the brain. Neuroscience 146, 1193–1211. doi: 10.1016/j.neuroscience.2007.01.072
David, D. J., Bourin, M., Hascoët, M., Colombel, M. C., Baker, G. B., and Jolliet, P. (2001). Comparison of antidepressant activity in 4- and 40-week-old male mice in the forced swimming test: involvement of 5-HT1A and 5-HT1B receptors in old mice. Psychopharmacology 153, 443–449. doi: 10.1007/s002130000588
Daws, L. C. (2009). Unfaithful neurotransmitter transporters: focus on serotonin uptake and implications for antidepressant efficacy. Pharmacol. Ther. 121, 89–99. doi: 10.1016/j.pharmthera.2008.10.004
Daws, L. C., and Gould, G. G. (2011). Ontogeny and regulation of the serotonin transporter: providing insights into human disorders. Pharmacol. Ther. 131, 61–79. doi: 10.1016/j.pharmthera.2011.03.013
Dell’Osso, B., Palazzo, M. C., Oldani, L., and Altamura, A. C. (2011). The noradrenergic action in antidepressant treatments: pharmacological and clinical aspects. CNS Neurosci. Ther. 17, 723–732. doi: 10.1111/j.1755-5949.2010.00217.x
Descarries, L., and Riad, M. (2012). Effects of the antidepressant fluoxetine on the subcellular localization of 5-HT1A receptors and SERT. Philos. Trans. R. Soc. Lond. Ser. B Biol. Sci. 367, 2416–2425. doi: 10.1098/rstb.2011.0361
Detke, M. J., Johnson, J., and Lucki, I. (1997). Acute and chronic antidepressant drug treatment in the rat forced swimming test model of depression. Exp. Clin. Psychopharmacol. 5, 107–112. doi: 10.1037/1064-1297.5.2.107
Duan, H., and Wang, J. (2010). Selective transport of monoamine neurotransmitters by human plasma membrane monoamine transporter and organic cation transporter 3. J. Pharmacol. Exp. Ther. 335, 743–753. doi: 10.1124/jpet.110.170142
Dulawa, S. C., Holick, K. A., Gundersen, B., and Hen, R. (2004). Effects of chronic fluoxetine in animal models of anxiety and depression. Neuropsychopharmacology 29, 1321–1330. doi: 10.1038/sj.npp.1300433
Durgam, S., Chen, C., Migliore, R., Prakash, C., Edwards, J., and Findling, R. L. (2018). A phase 3, double-blind, randomized, placebo-controlled study of vilazodone in adolescents with major depressive disorder. Paediatr. Drugs 20, 353–363. doi: 10.1007/s40272-018-0290-4
Emslie, G. J., Heiligenstein, J. H., Wagner, K. D., Hoog, S. L., Ernest, D. E., Brown, E., et al. (2002). Fluoxetine for acute treatment of depression in children and adolescents: a placebo-controlled, randomized clinical trial. J. Am. Acad. Child Adolesc. Psychiatry 41, 1205–1215. doi: 10.1097/01.CHI.0000024834.94814.9A
Emslie, G. J., Prakash, A., Zhang, Q., Pangallo, B. A., Bangs, M. E., and March, J. S. (2014). A double-blind efficacy and safety study of duloxetine fixed doses in children and adolescents with major depressive disorder. J. Child Adolesc. Psychopharmacol. 24, 170–179. doi: 10.1089/cap.2013.0096
Emslie, G. J., Rush, A. J., Weinberg, W. A., Kowatch, R. A., Hughes, C. W., Carmody, T., et al. (1997). A double-blind, randomized, placebo-controlled trial of fluoxetine in children and adolescents with depression. Arch. Gen. Psychiatry 54, 1031–1037. doi: 10.1001/archpsyc.1997.01830230069010
Emslie, G. J., Ventura, D., Korotzer, A., and Tourkodimitris, S. (2009). Escitalopram in the treatment of adolescent depression: a randomized placebo-controlled multisite trial. J. Am. Acad. Child Adolesc. Psychiatry 48, 721–729. doi: 10.1097/CHI.0b013e3181a2b304
Emslie, G. J., Wagner, K. D., Kutcher, S., Krulewicz, S., Fong, R., Carpenter, D. J., et al. (2006). Paroxetine treatment in children and adolescents with major depressive disorder: a randomized, multicenter, double-blind, placebo-controlled trial. J. Am. Acad. Child Adolesc. Psychiatry 45, 709–719. doi: 10.1097/01.chi.0000214189.73240.63
Engel, K., Zhou, M., and Wang, J. (2004). Identification and characterization of a novel monoamine transporter in the human brain. J. Biol. Chem. 279, 50042–50049. doi: 10.1074/jbc.M407913200
Findling, R. L., Robb, A., and Bose, A. (2013). Escitalopram in the treatment of adolescent depression: a randomized, double-blind, placebo-controlled extension trial. J. Child Adolesc. Psychopharmacol. 23, 468–480. doi: 10.1089/cap.2012.0023
Fraser-Spears, R., Krause-Heuer, A. M., Basiouny, M., Mayer, F. P., Manishimwe, R., Wyatt, N. A., et al. (2019). Comparative analysis of novel decynium-22 analogs to inhibit transport by the low-affinity, high-capacity biogenic amine transporters, organic cation transporters 2 and 3, and plasma membrane monoamine transporter. Eur. J. Pharmacol. 842, 351–364. doi: 10.1016/j.ejphar.2018.10.028
Fuhrmann, P., Equit, M., Schmidt, K., and von Gontard, A. (2014). Prevalence of depressive symptoms and associated developmental disorders in preschool children: a population-based study. Eur. Child Adolesc. Psychiatry 23, 219–224. doi: 10.1007/s00787-013-0452-4
Galineau, L., Kodas, E., Guilloteau, D., Vilar, M. P., and Chalon, S. (2004). Ontogeny of the dopamine and serotonin transporters in the rat brain: an autoradiographic study. Neurosci. Lett. 363, 266–271. doi: 10.1016/j.neulet.2004.04.007
Gasser, P. J., and Daws, L. C. (2017a). Editorial for the special issue: monoamine transporters in health and disease. J. Chem. Neuroanat. 8, 1–2. doi: 10.1016/j.chemneu.2017.07.008
Gasser, P. J., and Daws, L. C. (2017b). Extending the family: roles of uptake2 transporters in regulation of monoaminergic signaling. J. Chem. Neuroanat. 8, 107–108. doi: 10.1016/j.chemneu.2017.07.009
Gasser, P. J., Orchinik, M., Raju, I., and Lowry, C. A. (2009). Distribution of organic cation transporter 3, a corticosterone-sensitive monoamine transporter, in the rat brain. J. Compar. Neurol. 512, 529–555. doi: 10.1002/cne.21921
Gould, G. G., Brooks, B. W., and Frazer, A. (2007). [(3)H] citalopram binding to serotonin transporter sites in minnow brains. Basic Clin. Pharmacol. Toxicol. 101, 203–210. doi: 10.1111/j.1742-7843.2007.00100.x
Gould, G. G., Pardon, M. C., Morilak, D. A., and Frazer, A. (2003). Regulatory effects of reboxetine treatment alone, or following paroxetine treatment, on brain noradrenergic and serotonergic systems. Neuropsychopharmacology 28, 1633–1641. doi: 10.1038/sj.npp.1300236
Griebel, G., Cohen, C., Perrault, G., and Sanger, D. J. (1999). Behavioral effects of acute and chronic fluoxetine in Wistar-Kyoto rats. Physiol. Behav. 67, 315–320. doi: 10.1016/S0031-9384(98)00298-4
Hazell, P., and Mirzaie, M. (2013). Tricyclic drugs for depression in children and adolescents. Cochr. Datab. Systemat. Rev. 18:CD002317. doi: 10.1002/14651858.CD002317.pub2
Hazell, P., O’Connell, D., Heathcote, D., and Henry, D. (2002). Tricyclic drugs for depression in children and adolescents. Cochr. Datab. Syst. Rev. 2:CD002317. doi: 10.1002/14651858.CD002317
Hefner, K., and Holmes, A. (2007). Ontogeny of fear-, anxiety-, and depression-related behavior across adolescence in C57BL/6J mice. Behav. Brain Res. 176, 210–215. doi: 10.1016/j.bbr.2006.10.001
Hetrick, S. E., McKenzie, J. E., Cox, G. R., Simmons, M. B., and Merry, S. N. (2012). Newer generation antidepressants for depressive disorders in children and adolescents (review). Cochr. Datab. Syst. Rev. 11, 1–137. doi: 10.1002/14651858.CD004851.phb3
Hetrick, S. E., McKenzie, J. E., and Merry, S. N. (2010). The use of SSRIs in children and adolescents. Curr. Opin. Psychiatry 23, 53–57. doi: 10.1097/yco.0b013e328334bc92
Holick, K. A., Lee, D. C., Hen, R., and Dulawa, S. C. (2008). Behavioral effects of chronic fluoxetine in BALB/cJ mice do not require adult hippocampal neurogenesis or the serotonin 1A receptor. Neuropsychopharmacology 33, 406–417. doi: 10.1038/sj.npp.1301399
Homberg, J. R., Olivier, J. D. A., Blom, T., Arentsen, T., van Brunschot, C., Schipper, P., et al. (2011). Fluoxetine exerts age-dependent effects on behavior and amygdala neuroplasticity in the rat. PLoS One 6:e16646. doi: 10.1371/journal.pone.0016646
Horton, R. E., Apple, D. M., Owens, W. A., Baganz, N. L., Cano, S., Mitchell, N. C., et al. (2013). Decynium-22 enhances SSRI-induced antidepressant-like effects in mice: uncovering novel targets to treat depression. J. Neurosci. 33, 10534–10543. doi: 10.1523/jneurosci.5687-11.2013
Iceta, R., Mesonero, J. E., and Alcalde, A. I. (2007). Effect of long-term fluoxetine treatment on the human serotonin transporter in Caco-2 cells. Life Sci. 80, 1517–1524. doi: 10.1016/j/lfs.2007.01.020
Jiao, J., Nitzke, A. M., Doukas, D. G., Seiglie, M. P., and Dulawa, S. C. (2011). Antidepressant response to chronic citalopram treatment in eight inbred mouse strains. Psychopharmacology 213, 509–520. doi: 10.1007/s00213-010-2140-0
Kafali, N., Progovac, A., Hou, S. S., and Cook, B. L. (2018). Long-run trends in antidepressant use among youths after the FDA black box warning. Psychiatr. Serv. 69, 389–395. doi: 10.1176/appi.ps.201700089
Karanges, E., Li, K. M., Motbey, C., Callaghan, P. D., Katsifis, A., and McGregor, I. S. (2011). Differential behavioural and neurochemical outcomes from chronic paroxetine treatment in adolescent and adult rats: a model of adverse antidepressant effects in human adolescents? Int. J. Neuropsychopharmacol. 14, 491–504. doi: 10.1017/S146114571100006X
Keller, M. B., Ryan, N. D., Strober, M., Klein, R. G., Kutcher, S. P., Birmaher, B., et al. (2001). Efficacy of paroxetine in the treatment of adolescent major depression: a randomized, controlled trial. J. Am. Acad. Child Adolesc. Psychiatry 40, 762–772. doi: 10.1097/00004583-200107000-00010
Kessler, R. C., Avenevoli, S., and Ries Merikangas, K. (2001). Mood disorders in children and adolescents: an epidemiologic perspective. Biol. Psychiatry 49, 1002–1014. doi: 10.1016/S0006-3223(01)01129-5
Klomp, A., Václavu, L., Meerhoff, G. F., Reneman, L., and Lucassen, P. (2014). Effects of chronic fluoxetine treatment on neurogenesis and tryptophan hydroxylase expression in adolescent and adult rats. PLoS One 9:e97603. doi: 10.1371/journal.pone.0097603
Kodish, I., Rockhill, C., and Varley, C. (2011). Pharmacotherapy for anxiety disorders in children and adolescents. Dial. Clin. Neurosci. 13, 439–452. doi: 10.1016/j.pcl.2010.10.002
Koepsell, H., Lips, K., and Volk, C. (2007). Polyspecific organic cation transporters: structure, function, physiological roles, and biopharmaceutical implications. Pharm. Res. 24, 1227–1251. doi: 10.1007/s11095-007-9254-z
Kozisek, M. E., Middlemas, D., and Bylund, D. B. (2008). The differential regulation of BDNF and TrkB levels in juvenile rats after four days of escitalopram and desipramine treatment. Neuropharmacology 54, 251–257. doi: 10.1016/j.neuropharm.2007.08.001
Krause-Heuer, A. M., Fraser-Spears, R., Dobrowolski, J. C., Ashford, M. E., Wyatt, N. A., Roberts, M. P., et al. (2017). Evaluation of the antidepressant therapeutic potential of isocyanine and pseudoisocyanine analogues of the organic cation decynium-22. Eur. J. Med. Chem. 137, 476–487. doi: 10.1016/j.ejmech.2017.06.011
Kronenberg, S., Apter, A., Brent, D., Schirman, S., Melhem, N., Pick, N., et al. (2007). Serotonin transporter polymorphism (5-HTTLPR) and citalopram effectiveness and side effects in children with depression and/or anxiety disorders. J. Child Adolesc. Psychopharmacol. 17, 741–750. doi: 10.1089/cap.2006.0144
Kulikov, A. V., Gainetdinov, R. R., Ponimaskin, E., Kalueff, A. V., Naumenko, V. S., and Popova, N. K. (2018). Interplay between key proteins of serotonin system in SSRI antidepressant efficacy. Exp. Opin. Therapeut. Targets 22, 319–330. doi: 10.1080/14728222.2018.1452912
Lenroot, R. K., and Giedd, J. N. (2006). Brain development in children and adolescents: insights from anatomical magnetic resonance imaging. Neurosci. Biobehav. Rev. 30, 718–729. doi: 10.1016/j.neubiorev.2006.06.001
Lin, W. H., Li, X. F., Lin, M. X., Zhou, Y., and Huang, H. P. (2017). Novel insights into the effect of paroxetine administration in pilocarpine-induced chronic epileptic rats. Mol. Med. Rep. 16, 8245–8252. doi: 10.3892/mmr.2017.7659
Lucki, I., Dalvi, A., and Mayorga, A. J. (2001). Sensitivity to the effects of pharmacologically selective antidepressants in different strains of mice. Psychopharmacology 155, 315–322. doi: 10.1007/s002130100694
Maneeton, N., and Srisurapanont, M. (2000). Tricyclic antidepressants for depressive disorders in children and adolescents: a meta-analysis of randomized-controlled trials. J. Med. Assoc. Thailand 83, 1367–1374.
March, J., Silva, S., Petrycki, S., Curry, J., Wells, K., Fairbank, J., …Treatment for Adolescents with Depression Study (TADS) Team (2004). Fluoxetine, cognitive-behavioral therapy, and their combination for adolescents with depression: treatment for adolescents with depression study (TADS) randomized controlled trial. J. Am. Med. Assoc. 292, 807–820. doi: 10.1001/jama.292.7.807
Mason, S. S., Baker, K. B., Davis, K. W., Pogorelov, V. M., Malbari, M. M., Ritter, R., et al. (2009). Differential sensitivity to SSRI and tricyclic antidepressants in juvenile and adult mice of three strains. Eur. J. Pharmacol. 602, 306–315. doi: 10.1016/j.ejphar.2008.11.010
Matthäus, F., Haddjeri, N., Sánchez, C., Martí, Y., Bahri, S., Rovera, R., et al. (2016). The allosteric citalopram binding site differentially interferes with neuronal firing rate and SERT trafficking in serotonergic neurons. Eur. Neuropsychopharmacol. 26, 1806–1817. doi: 10.1016/j.euroneuro.2016.09.001
Maughan, B., Collishaw, S., and Stringaris, A. (2013). Depression in childhood and adolescence. J. Can. Acad. Child Adolesc. Psychiatry 22, 35–40.
Miller, B. H., Schultz, L. E., Gulati, A., Cameron, M. D., and Pletcher, M. T. (2008). Genetic regulation of behavioral and neuronal responses to fluoxetine. Neuropsychopharmacology 33, 1312–1322. doi: 10.1083/sj.npp.1301497
Mirza, N. R., Nielsen, E. O., and Troelsen, K. B. (2007). Serotonin transporter density and anxiolytic-like effects of antidepressants in mice. Progr. Neuro-psychopharmacol. Biol. Psychiatry 31, 858–866. doi: 10.1016/j.pnpbp.2007.01.020
Mitchell, N. C., Bowman, M. A., Gould, G. G., Koek, W., and Daws, L. C. (2017). Ontogeny of norepinephrine transporter expression and antidepressant-like response to desipramine in wild-type and serotonin transporter mutant mice. J. Pharmacol. Exp. Ther. 360, 84–94. doi: 10.1124/jpet.116.237305
Mitchell, N. C., Gould, G. G., Koek, W., and Daws, L. C. (2016). Ontogeny of SERT expression and antidepressant-like response to escitalopram in wild-type and SERT mutant mice. J. Pharmacol. Exp. Ther. 358, 271–281. doi: 10.1124/jpet.116.233338
Mitchell, N. C., Gould, G. G., Smolik, C. M., Koek, W., and Daws, L. C. (2013). Antidepressant-like drug effects in juvenile and adolescent mice in the tail suspension test: relationship with hippocampal serotonin and norepinephrine transporter expression and function. Front. Pharmacol. 4:131. doi: 10.3389/fphar.2013.00131
Mojtabai, R., Olfson, M., and Han, B. (2016). National trends in the prevalence and treatment of depression in adolescents and young adults. Pediatrics 138:e20161878. doi: 10.1542/peds.2016-1878
Munari, L., Provensi, G., Passani, M. B., Galeotti, N., Cassano, T., Benetti, F., et al. (2015). Brain histamine is crucial for selective serotonin reuptake inhibitors’ behavioral and neurochemical effects. Int. J. Neuropsychopharmacol. 18:pyv045. doi: 10.1093/ijnp/pyv045
Murrin, L. C., Sanders, J. D., and Bylund, D. B. (2007). Comparison of the maturation of the adrenergic and serotonergic neurotransmitter systems in the brain: implications for differential drug effects on juveniles and adults. Biochem. Pharmacol. 73, 1225–1236. doi: 10.1016/j.bcp.2007.01.028
Norrholm, S. D., and Ouimet, C. C. (2000). Chronic fluoxetine administration to juvenile rats prevents age-associated dendritic spine proliferation in hippocampus. Brain Res. 883, 205–215. doi: 10.1016/S0006-8993(00)02909-7
O’Leary, O. F., and Cryan, J. F. (2013). Towards translational rodent models of depression. Cell Tissue Res. 354, 141–153. doi: 10.1007/s00441-013-1587-9
Pechnick, R. N., Bresee, C. J., Manalo, C. M., and Poland, R. E. (2008). Comparison of the effects of desmethylimipramine on behavior in the forced swim test in peripubertal and adult rats. Behav. Pharmacol. 19, 81–84. doi: 10.1097/fbp.0b013e3282f3d07f
Reed, A. L., Anderson, J. C., Bylund, D. B., Petty, F., Refaey, H. E., and Happe, H. K. (2009). Treatment with escitalopram but not desipramine decreases escape latency times in a learned helplessness model using juvenile rats. Psychopharmacology 205, 249–259. doi: 10.1007/s00213-009-1535-2
Reed, A. L., Happe, H. K., Petty, F., and Bylund, D. B. (2008). Juvenile rats in the forced-swim test model the human response to antidepressant treatment for pediatric depression. Psychopharmacology 197, 433–441. doi: 10.1007/s00213-007-1052-0
Roohi-Azizi, M., Torkaman-Boutorabi, A., Akhondzadeh, S., Nejatisafa, A. A., Sadat-Shirazi, M. S., and Zarrindast, M. R. (2018). Influence of citicoline on citalopram-induced antidepressant activity in depressive-like symptoms in male mice. Physiol. Behav. 195, 151–157. doi: 10.1016/j.physbeh.2018.08.002
Sallee, F. R., Hilal, R., Dougherty, D., Beach, K., and Nesbitt, L. (1998). Platelet serotonin transporter in depressed children and adolescents: 3H-paroxetine platelet binding before and after sertraline. J. Am. Acad. Child Adolesc. Psychiatry 37, 777–784. doi: 10.1097/00004583-199807000-00018
Shrestha, S. S., Nelson, E. E., Liow, J. S., Gladding, R., Lyoo, C. H., Noble, P. L., et al. (2014). Fluoxetine administration to juvenile monkeys: effects on the serotonin transporter and behavior. Am. J. Psychiatry 171, 323–331. doi: 10.1176/appi.ajp.2013.13020183
Simeon, J. G., Dinicola, V. F., Ferguson, H. B., and Copping, W. (1990). Adolescent depression: a placebo-controlled fluoxetine treatment study and follow-up. Progr. Neuro-psychopharmacol. Biol. Psychiatry 14, 791–795. doi: 10.1016/0278-5846(90)90050-Q
Spear, L. P. (2000). The adolescent brain and age-related behavioral manifestations. Neurosci. Biobehav. Rev. 24, 417–463. doi: 10.1016/S0149-7634(00)00014-2
Thapar, A., Collishaw, S., Pine, D. S., and Thapar, A. K. (2012). Depression in adolescence. Lancet 379, 1056–1067. doi: 10.1016/S0140-6736(11)60871-4
Tsapakis, E. M., Fernandes, C., Moran-Gates, T., Basu, A., Sugden, K., Aitchison, K. J., et al. (2014). Effects of antidepressant drug exposure on gene expression in the developing cerebral cortex. Synapse 68, 209–220. doi: 10.1002/syn.21732
Tsapakis, E. M., Soldani, F., Tondo, L., and Baldessarini, R. J. (2008). Efficacy of antidepressants in juvenile depression: meta-analysis. Br. J. Psychiatry 193, 10–17. doi: 10.1192/bjp.bp.106/031088
Ulloa, R.-E., Díaz-Valderrama, A., Herrera-Pérez, J., León-Olea, M., and Martínez-Mota, L. (2014). Age differences in the impact of forced swimming test on serotonin transporter levels in lateral septum and dorsal raphe. Behav. Brain Funct. 10, 1–8. doi: 10.1186/1744-9081-10-3
Vialou, V., Balasse, L., Callebert, J., Launay, J.-M., Giros, B., and Gautron, S. (2008). Altered aminergic neurotransmission in the brain of organic cation transporter 3-deficit mice. J. Neurochemistry 106, 1471–1482. doi: 10.1111/j.1471-4159.2008.05506.x
von Knorring, A. L., Olsson, G. I., Thomsen, P. H., Lemming, O. M., and Hultén, A. (2006). A randomized, double-blind, placebo-controlled study of citalopram in adolescents with major depressive disorder. J. Clin. Psychopharmacol. 26, 311–315. doi: 10.1097/01.jcp.0000219051.40632.d5
Wagner, K. D., Ambrosini, P., Rynn, M., Wohlberg, C., Yang, R., Greenbaum, M. S., et al. (2003). Efficacy of sertraline in the treatment of children and adolescents with major depressive disorder: two randomized controlled trials. J. Am. Med. Assoc. 290, 1033–1041. doi: 10.1001/jama.290.8.1033
Wagner, K. D., Jonas, J., Findling, R. L., Ventura, D., and Saikali, K. (2006). A double-blind, randomized, placebo-controlled trial of escitalopram in the treatment of pediatric depression. J. Am. Acad. Child Adolesc. Psychiatry 45, 280–288. doi: 10.1097/01.chi.0000192250.38400.9e
Wagner, K. D., Robb, A. S., Findling, R. L., Jin, J., Gutierrez, M. M., and Heydorn, W. E. (2004). A randomized, placebo-controlled trial of citalopram for the treatment of major depression in children and adolescents. Am. J. Psychiatry 161, 1079–1083. doi: 10.1176/appi.ajp.161.6.1079
Wang, J. (2016). The plasma membrane monoamine transporter (PMAT): structure, function, and role in organic cation disposition. Clin. Pharmacol. Therapeut. 100, 489–499. doi: 10.1002/cpt.442
Wegerer, V., Moll, G. H., Bagli, M., Rothenberger, A., Rüther, E., and Huether, G. (1999). Persistently increased density of serotonin transporters in the frontal cortex of rats treated with fluoxetine during early juvenile life. J. Child Adolesc. Psychopharmacol. 9, 13–24. doi: 10.1089/cap.1999.9.13
Keywords: serotonin, transporters, depression, antidepressants, development, children, juveniles, adolescents
Citation: Bowman MA and Daws LC (2019) Targeting Serotonin Transporters in the Treatment of Juvenile and Adolescent Depression. Front. Neurosci. 13:156. doi: 10.3389/fnins.2019.00156
Received: 03 November 2018; Accepted: 11 February 2019;
Published: 27 February 2019.
Edited by:
Guillaume Lucas, INSERM U1215 Neurocentre Magendie, FranceReviewed by:
Renato Corradetti, Università degli Studi di Firenze, ItalyThorsten Lau, Central Institute for Mental Health, Germany
Ove Wiborg, Aalborg University, Denmark
Copyright © 2019 Bowman and Daws. This is an open-access article distributed under the terms of the Creative Commons Attribution License (CC BY). The use, distribution or reproduction in other forums is permitted, provided the original author(s) and the copyright owner(s) are credited and that the original publication in this journal is cited, in accordance with accepted academic practice. No use, distribution or reproduction is permitted which does not comply with these terms.
*Correspondence: Lynette C. Daws, ZGF3c0B1dGhzY3NhLmVkdQ==