- 1UMR 1286, Laboratory of Nutrition and Integrative Neurobiology (NutriNeuro), INRA, Bordeaux, France
- 2UMR 1286, Laboratory of Nutrition and Integrative Neurobiology (NutriNeuro), Bordeaux University, Bordeaux, France
The prevalence of depressive disorders is growing worldwide, notably due to stagnation in the development of drugs with greater antidepressant efficacy, the continuous large proportion of patients who do not respond to conventional antidepressants, and the increasing rate of chronic medical conditions associated with an increased vulnerability to depressive comorbidities. Accordingly, better knowledge on the pathophysiology of depression and mechanisms underlying depressive comorbidities in chronic medical conditions appears urgently needed, in order to help in the development of targeted therapeutic strategies. In this review, we present evidence pointing to inflammatory processes as key players in the pathophysiology and treatment of depressive symptoms. In particular, we report preclinical and clinical findings showing that inflammation-driven alterations in specific metabolic pathways, namely kynurenine and tetrahydrobiopterin (BH4) pathways, leads to substantial alterations in the metabolism of serotonin, glutamate and dopamine that are likely to contribute to the development of key depressive symptom dimensions. Accordingly, anti-inflammatory interventions targeting kynurenine and BH4 pathways may be effective as novel treatment or as adjuvants of conventional medications rather directed to monoamines, notably when depressive symptomatology and inflammation are comorbid in treated patients. This notion is discussed in the light of recent findings illustrating the tight interactions between known antidepressant drugs and inflammatory processes, as well as their therapeutic implications. Altogether, this review provides valuable findings for moving toward more adapted and personalized therapeutic strategies to treat inflammation-related depressive symptoms.
Introduction
Depression currently represents a global public health concern. Not only its prevalence is steadily increasing worldwide but also it relates to a stronger risk of morbidity and death (World Health Organization, 2017). In addition, despite advances made in the treatment of depression, at least one third of depressed patients fail to respond to conventional medications (Rush et al., 2006). Further contributing to the global burden of depression, most of the chronic diseases whose prevalence is also continuously rising, including metabolic, autoimmune and cardiovascular diseases, are associated with an increased risk of depression (Luppino et al., 2010; Capuron et al., 2017; Zuzarte et al., 2018). The latter impairs the quality of life of affected patients and emerges as a potent contributor of subsequent medical complications (Penninx et al., 2013). Thus, a better knowledge of the pathophysiological bases of depression and identification of new targets relevant for therapeutic advances are still clearly needed.
While different neurobiological systems are likely involved in the pathophysiology of depression, this review will summarize evidence that supports a main role for inflammatory processes. First dedicated to decipher the intricate relationship between the innate immune system and the brain, initial research performed in the field of psychoneuroimmunology started to identify the molecular mechanisms underlying the behavioral and neuropsychiatric consequences of inflammation (for review Dantzer et al., 2008; Capuron and Miller, 2011). Meanwhile, converging clinical studies reported a link between increased circulating levels of inflammatory factors and greater risk of developing mood alterations (Capuron et al., 2002; Evans et al., 2005). Altogether, these findings have fostered still on-going investigations aiming to further identify the neurobiological pathways targeted by cytokines and in turn mediating their neuropsychiatric effects. Moreover, in line with recent data suggesting that elevated basal inflammation, as reported in obesity and metabolic syndrome, may contribute to impair the therapeutic efficiency of conventional antidepressant treatment (Kloiber et al., 2007; Toups et al., 2013), a corollary question arises as to whether inflammatory factors, their signaling pathways and/or neurobiological targets, may represent potential targets for new pharmacological interventions. This review will discuss those issues in the light of recent findings providing a deeper mechanistic understanding of the role of inflammation in the pathophysiology of depression and relevant insights for novel therapies.
The Inflammatory Hypothesis of Depression
Although progressively extended to several mental illnesses, including bipolar disorders, anxiety disorders or schizophrenia (Potvin et al., 2008; Hoge et al., 2009; Barbosa et al., 2013), the notion that inflammation may be involved in the pathophysiology of neuropsychiatric symptoms was particularly studied in the context of depression (Raison et al., 2006; Capuron and Miller, 2011). The first studies strongly supporting this notion report higher circulating levels of inflammatory mediators, including C-reactive protein (CRP), cytokines (particularly interleukin-6, IL-6) and different chemokines, in depressed individuals compared to healthy controls (Dowlati et al., 2010; Leighton et al., 2018). Moreover, longitudinal investigations reveal that higher inflammatory profiles predict the development of depressive symptoms (Valkanova et al., 2013; Smith et al., 2018). To move from correlation to causality, chronic cytokine administration has been shown to induce depression in up to 50% of medically ill patients undergoing cytokine immunotherapy (Musselman et al., 2001; Capuron et al., 2002; Kawase et al., 2016). Similarly, direct administration of cytokines, or cytokine inducers, such as lipopolysaccharide (LPS), to healthy volunteers triggers depressive symptoms (Schedlowski et al., 2014; Engler et al., 2017). Consistent with clinical findings, both acute or chronic immune challenges in rodents induce sustained depressive-like and anxiety-like behaviors (Merali et al., 2003; Frenois et al., 2007; Moreau et al., 2008; Klaus et al., 2016). Conversely, anti-inflammatory compounds reduce these behaviors in animal models of inflammatory diseases (Llorens-Martin et al., 2014; Zaminelli et al., 2014; Norden et al., 2015). Likewise, directly targeting specific inflammatory cytokines decreases mood alterations in both humans and rodents (Tyring et al., 2006; Kekow et al., 2010; Haji et al., 2012; Bayramgürler et al., 2013; Fleming et al., 2015).
Inflammation-induced behavioral changes have been shown to rely on a large communication network allowing inflammatory cytokines [e.g., IL-1β, IL-6, tumor necrosis factor (TNF)-α], which are released peripherally by activated immune cells, to reach the brain through humoral, nervous and chemical pathways and to locally induce production of brain cytokines by activated microglia, the immune cells of the brain. These cytokines in turn influence pathways involved in the regulation of behavior and mood, including neurotransmitter metabolism, neuroendocrine function and neural plasticity (Castanon et al., 2004; Frenois et al., 2007; Dantzer et al., 2008; Capuron and Miller, 2011). These neuroimmune interactions not only coordinate the immune response, but also the development of adaptive behavioral changes collectively referred to as sickness behavior. These changes, which include weakness, listlessness, malaise, anorexia and fatigue, have been shown to be necessary for recovery, by helping the body to actively fight against aggressions. Inflammatory activation is usually transient and controlled by anti-inflammatory mechanisms to warrant time-limitation and reversibility of sickness behavior. Conversely, failure of those control mechanisms that allows development of sustained inflammation has been shown to represent a major leading cause of inflammation-related mood alterations (Dantzer et al., 2008; Capuron and Miller, 2011; Castanon et al., 2014; Capuron and Castanon, 2017). Indeed, if inflammation initially serves a protective function in controlling infection and promoting tissue repair, it can in the long run interfere with brain neurotransmission and cause tissue damages, what ultimately contributes to promote sustained behavioral and mood alterations. Interestingly, compelling clinical studies revealed that patients under cytokine immunotherapy display symptoms spanning multiple dimensions from neurovegetative (e.g., fatigue, decreased tone and motivation) to neuropsychiatric (e.g., depressed mood, anxiety, cognitive alterations) levels (Musselman et al., 2001; Capuron and Miller, 2011; Capuron and Castanon, 2017). Importantly, these symptoms differ in their time-course and responsiveness to prophylactic treatment with classical antidepressant drugs, particularly those targeting serotonin neurotransmission. In particular, neurovegetative symptoms appear early after initiation of cytokine immunotherapy and in a large proportion of patients. On the contrary, neuropsychiatric symptoms progressively develop after several weeks of cytokine administration and only affect 30–50% of patients. These last symptoms can be prevented by antidepressant treatment, in contrast to neurovegetative symptoms that are not, or only minimally, responsive to this intervention (Musselman et al., 2001; Capuron and Miller, 2011). Altogether, these findings suggested the involvement of distinct underlying mechanisms.
Strong support for this assumption came from studies performed in immune-challenged animals in which it is possible to experimentally dissociate sickness behavior, occurring early after the immune stimulation, from protracted depressive-like behaviors (Merali et al., 2003; Frenois et al., 2007; Moreau et al., 2008; Klaus et al., 2016). First attempts to assess the respective underlying neurobiological mechanisms have concentrated on the same preclinical models. In that context, it is worth mentioning that some of the behavioral changes characterizing sickness behavior, particularly motor slowing, could interfere with the measure of depressive-like behaviors when assessed in paradigms based on motor responses. This potential methodological bias has, however, been circumvented by using several reliable and complementary behavioral tests modeling different core symptoms of depression, and by testing mice in these tests only once they have totally recovered from sickness behavior (Frenois et al., 2007; Godbout et al., 2008; O’Connor et al., 2009c). Interestingly, this experimental design in LPS-challenged mice enabled to show a neuroanatomical dissociation between the brain structures that underlie LPS-induced sickness and depressive-like behaviors, respectively (Frenois et al., 2007). It could still be argued that some inconsistencies exist across the literature (see Biesmans et al., 2013) and that a model of acute immune activation with LPS is not necessarily relevant to the clinical situation. Several studies performed in models of chronic inflammation, which can be claimed as more suitable, however, confirmed the presence of sustained depressive-like behaviors while sickness behavior was not anymore detectable nor levels of circulating cytokines elevated (Moreau et al., 2008; O’Connor et al., 2009a,b). Together with clinical studies, all these models turned out to be very useful to progress in the identification of the neurobiological bases of inflammation-related depressive symptoms.
Neurobiological Bases of Inflammation-Related Depressive Symptoms
Mounting clinical and preclinical findings led us and others to propose that the multidimensional inflammation-related symptoms may rely on the ability of inflammatory cytokines to alter important metabolic pathways, namely kynurenine and tetrahydrobiopterin (BH4) pathways, which in turn can impair neurotransmission of monoamines, particularly serotonin, glutamate and dopamine, involved in mood regulation (Dantzer et al., 2008; Capuron and Miller, 2011; Capuron and Castanon, 2017; Haroon et al., 2017).
The Kynurenine Pathway: At the Crossroad Between Inflammation and Mood
The metabolism of the essential amino acid tryptophan produces an array of crucial factors able of regulating key physiological processes linked to behavior and mood, particularly serotonin. Tryptophan metabolism along the kynurenine pathway (KP) accounts for most of the tryptophan that is not used for protein synthesis, and ultimately leads to the production of several neuroactive metabolites, including 3-hydroxykynurenine (3-HK) and quinolinic acid (QA), which are able to stimulate N-methyl-D-aspartate (NMDA) glutamatergic receptors and promote oxidative stress, and kynurenic acid (KA) that rather displays neuroprotective properties (Figure 1) (Schwarcz and Stone, 2017). Since microglia preferentially produce QA, while KA is synthesized by astrocytes, neurotoxicity prevails in conditions of immune activation. Accordingly, increased plasma and/or cerebrospinal fluid QA levels have been reported in a plethora of conditions that encompass inflammatory and neurodegenerative damages, together with an increased prevalence of mood symptoms (Capuron et al., 2011; Bay-Richter et al., 2015; Lovelace et al., 2017; O’Farrell and Harkin, 2017; Schwarcz and Stone, 2017). More importantly, KP activation correlates with both stretch of brain damages and severity of neuropsychiatric symptoms (Capuron et al., 2002; Raison et al., 2010; Savitz et al., 2015a,b). These findings prompted a surge of interest for the involvement of KP enzymes and metabolites in inducing these symptoms, starting by the enzyme indoleamine 2,3-dioxygenase (IDO) that catalyzes the first and rate-limiting step of tryptophan metabolism along the KP (Lestage et al., 2002; Moreau et al., 2005; André et al., 2008). For example, it was shown in mice that inoculation with Bacillus Calmette-Guerin (BCG) chronically increases both peripheral and brain IDO activity, this activation paralleling development of sustained depressive-like behaviors (Moreau et al., 2008; O’Connor et al., 2009a,b). Aged mice (Godbout et al., 2008; Wynne et al., 2010), or mice exhibiting constitutive microglial over-activation (Corona et al., 2013) also display sustained cytokine production after an immune challenge, together with protracted brain IDO expression and depressive-like behavior (Godbout et al., 2008; Wynne et al., 2010; Corona et al., 2013). Similarly, a link between inflammation-related brain IDO activation and depressive-like behavior has been reported in other chronic inflammatory conditions, such as murine models of obesity (André et al., 2014; Dinel et al., 2014). Importantly, direct KP activation within the brain is sufficient to alter emotional behaviors in rodents, particularly when it occurs in key brain areas for behavior and mood, particularly the hippocampus (Henry et al., 2009; Fu et al., 2010; Park et al., 2011; Dobos et al., 2012; Lawson et al., 2013). By using mice with either genetic or systemic inhibition of IDO and submitted to an immune challenge, we and others described the causal role of IDO activation in the induction of depressive-like and anxiety-like behaviors (Godbout et al., 2008; O’Connor et al., 2009a,b,c; Salazar et al., 2012; Corona et al., 2013; Gibney et al., 2013; Xie et al., 2014; Castanon, 2015). This induction can be related to the potential for IDO to negatively impact serotonin synthesis by decreasing bioavailability of tryptophan. However, the fact that immune activation is actually associated with increased serotonin turnover (Godbout et al., 2008; O’Connor et al., 2009c; Gibney et al., 2013; Parrott et al., 2016a), regardless of concomitant IDO activation (O’Connor et al., 2009c), weakens this hypothesis. These findings may rather suggest intervention of other cytokine-induced alterations in serotonin neurotransmission, including modulation of serotonin transporter and receptors (Zhu et al., 2006). Follow-up work is therefore needed to deeply study the consequences of KP activation on the temporal and spatial patterns of serotonin-related processes.
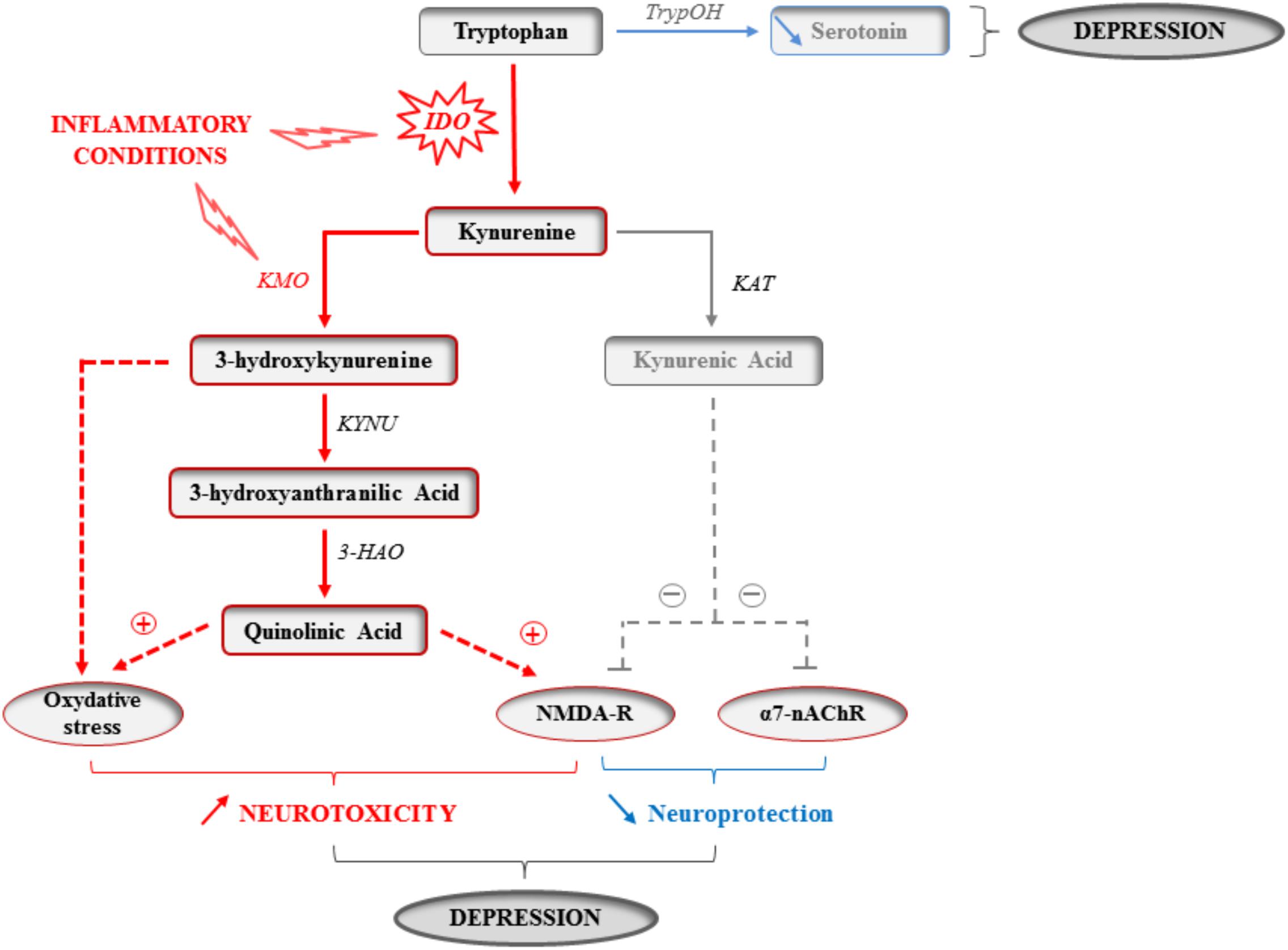
FIGURE 1. Kynurenine pathway activation in inflammatory conditions. The enzymatic activity of the indoleamine 2,3-dioxygenase (IDO) is increased by inflammatory cytokines in activated monocytes, macrophages and brain microglia in inflammatory conditions. The essential amino acid tryptophan is therefore used for the synthesis of kynurenine, and this at the expense of the synthesis of the monoamine serotonin that directly depends on the availability of its precursor and limiting factor tryptophan. IDO activation might therefore impair serotonin neurotransmission. Kynurenine is then used to produce different neuroactive glutamatergic metabolites, including kynurenic acid, which is neuroprotective by acting on both glutamatergic NMDA and α7-nicotinic acetylcholine (α7-nAChR) receptors, and 3-hydroxykynurenine and quinolinic acid that are rather neurotoxic by promoting oxidative stress and/or activating NMDA receptors. As the activity of the kynurenine monooxygenase (KMO) that synthesizes 3-hydroxykynurenine is increased in activated microglia by inflammatory cytokines, increased production of kynurenine is ultimately associated with skewing of the kynurenine metabolic balance toward increased neurotoxicity. By impairing serotonin neurotransmission and promoting neuronal damages, cytokine-induced kynurenine pathway activation can therefore contribute to the development of inflammation-driven depressive symptoms. TrypOH, tryptophan hydroxylase; KAT, kynurenine aminotransferase; KYNU, kynureninase; 3-HAO, 3-hydroxyanthranilic acid oxygenase.
An alternative explanation for the involvement of KP activation in inflammation-related mood symptoms is the generation of neurotoxic glutamatergic kynurenine metabolites, as they have been clinically related to the severity of mood alterations (Capuron et al., 2011; Bay-Richter et al., 2015; Haroon et al., 2017; Schwarcz and Stone, 2017). In line with the well-established role of altered neuron integrity and/or function in subtending the clinical outcomes of neurodegenerative diseases, neuronal damages have been also linked to the development of inflammation-induced depressive symptoms (Dantzer and Walker, 2014). This may particularly involve the NMDA receptors, which have recently drawn much attention in the field of depression research (Dantzer and Walker, 2014; Haroon et al., 2017). Supporting further this notion, preclinical studies report that depressive-like behaviors induced by LPS challenge (Parrott et al., 2016a,b) or related to neuropathic pain (Laumet et al., 2017) are associated with skewing of the kynurenine metabolic balance toward production of neurotoxic metabolites, and this in a region-specific manner with the hippocampus being particularly affected. Moreover, systemic administration of kynurenine (O’Connor et al., 2009c; Salazar et al., 2012; Agudelo et al., 2014) or 3-HK (Parrott et al., 2016a,b) dose-dependently impairs emotional behaviors, whereas mice deficient for IDO are protected against NMDA receptor-mediated excitotoxicity (Mazarei et al., 2013). Of note, NMDA receptor blockade abrogates inflammation-induced depressive-like behavior (Walker et al., 2013), along with selective inhibition of downstream KP enzymes, i.e., kynurenine 3-monooxygenase (KMO) or 3-hydroxyanthranilic acid dioxygenase (HAAO) that ultimately synthesize the NMDA receptor agonist QA (Parrott et al., 2016b; Laumet et al., 2017). Interestingly, this especially improves depressive-like behaviors related to clinical behavioral despair (i.e., immobility in the tail suspension test), while being less efficient on behavioral changes rather modeling anhedonia (i.e., sucrose preference) (Parrott et al., 2016b). These results are particularly relevant to help identifying the neurobiological mechanisms that, respectively, underlie the different inflammation-related depressive symptoms. As each behavior/symptom is functionally subtended by preferential activation of selective neuronal pathways in discrete brain regions, such dimensional dissociation may rely on the regional differences of KP activation reported in those experimental studies (Parrott et al., 2016a,b; Laumet et al., 2017). While LPS-induced brain expression of cytokines is largely region-independent, the 3-HK/KA ratio is significantly increased in the hippocampus, but not central amygdala or nucleus accumbens (Parrott et al., 2016a). Consistent with these preclinical findings, post-mortem studies of depressed patients link severe depression with increased microglial QA detection in selective cortical subregions (Steiner et al., 2011). Conversely, a higher neuroprotective index, as reflected by elevated KA/QA ratio, positively correlates with hippocampal volume in clinically depressed but non-medicated patients (Savitz et al., 2015a). Otherwise, the above-mentioned dissociation between KP activation and development of specific depressive symptoms may rather reflect involvement of other metabolic pathways and/or neurotransmission systems (Capuron and Castanon, 2017). This assumption is nicely supported by a recent study showing that inflammation-induced impairment of motivation-driven behaviors is independent from IDO activation since it persists in LPS-treated IDO deficient mice (Vichaya et al., 2018). With regard to inflammation-related motivational deficits, the BH4 pathway that can ultimately impair dopamine neurotransmission appears as a likely candidate (Felger and Treadway, 2017). This is supported by data obtained in mice deficient in BH4, which exhibit dopamine-related behavioral alterations (Choi and Tarazi, 2010). Both alternatives are not necessarily disconnected since KA has been shown to modulate striatal dopaminergic tone, by acting on α7-nicotinic acetylcholine receptors (Wu et al., 2007), while pharmacological activation of these receptors mitigates anhedonia in a mouse model of chronic stress (Zhao et al., 2017). Moreover, clinical data report an inverse association between circulating KA/QA ratio and the degree of anhedonia in depressed patients (Savitz et al., 2015a).
The BH4 Pathway: A Key Player in Neurobiological Modulation of Depressive Symptoms
BH4 is a pivotal cofactor for the optimal functioning of the nitric oxide synthase isoforms (NOS) and three aromatic amino acid hydroxylases: phenylalanine hydroxylase (PAH), tryptophan hydroxylase (trypOH) and tyrosine hydroxylase (TH) (Thöny et al., 2000; Sumi-Ichinose et al., 2001). Accordingly, it is required for the synthesis of dopamine, serotonin and nitric oxide from the essential amino acids tyrosine, tryptophan and arginine, respectively (Sumi-Ichinose et al., 2001). BH4 de novo synthesis involves the sequential activation of three enzymes, the first one, GTP-cyclohydrolase 1 (GCH1) being the rate-limiting step (Thöny et al., 2000) (Figure 2). The activity of this enzyme, which is co-localized with the monoamine-containing cells, has been shown to differ according to the brain area (Sawada et al., 1986; Nagatsu et al., 1995; Dassesse et al., 1997). Inflammatory cytokines, including IL-1β, interferon-γ and TNF-α, are able to induce both GCH1 expression and activity, therefore increasing BH4 synthesis (Shi et al., 2004). At the post-translational level, GCH1 is inhibited by BH4 and stimulated by phenylalanine, through its complex formation with the cyclohydrolase feedback regulatory protein (GFRP) (Neurauter et al., 2008). This process ensures tightly keeping BH4 levels within a physiological range in the body. BH4 is also oxidized to BH2, which can, however, be conversely converted back to BH4 by the dihydrofolate reductase (DHFR) (Harada et al., 1993). In fine, the net cellular bioavailability of BH4 likely results therefore from the balance between its de novo synthesis, its oxidation to BH2, and its regeneration by DHFR.
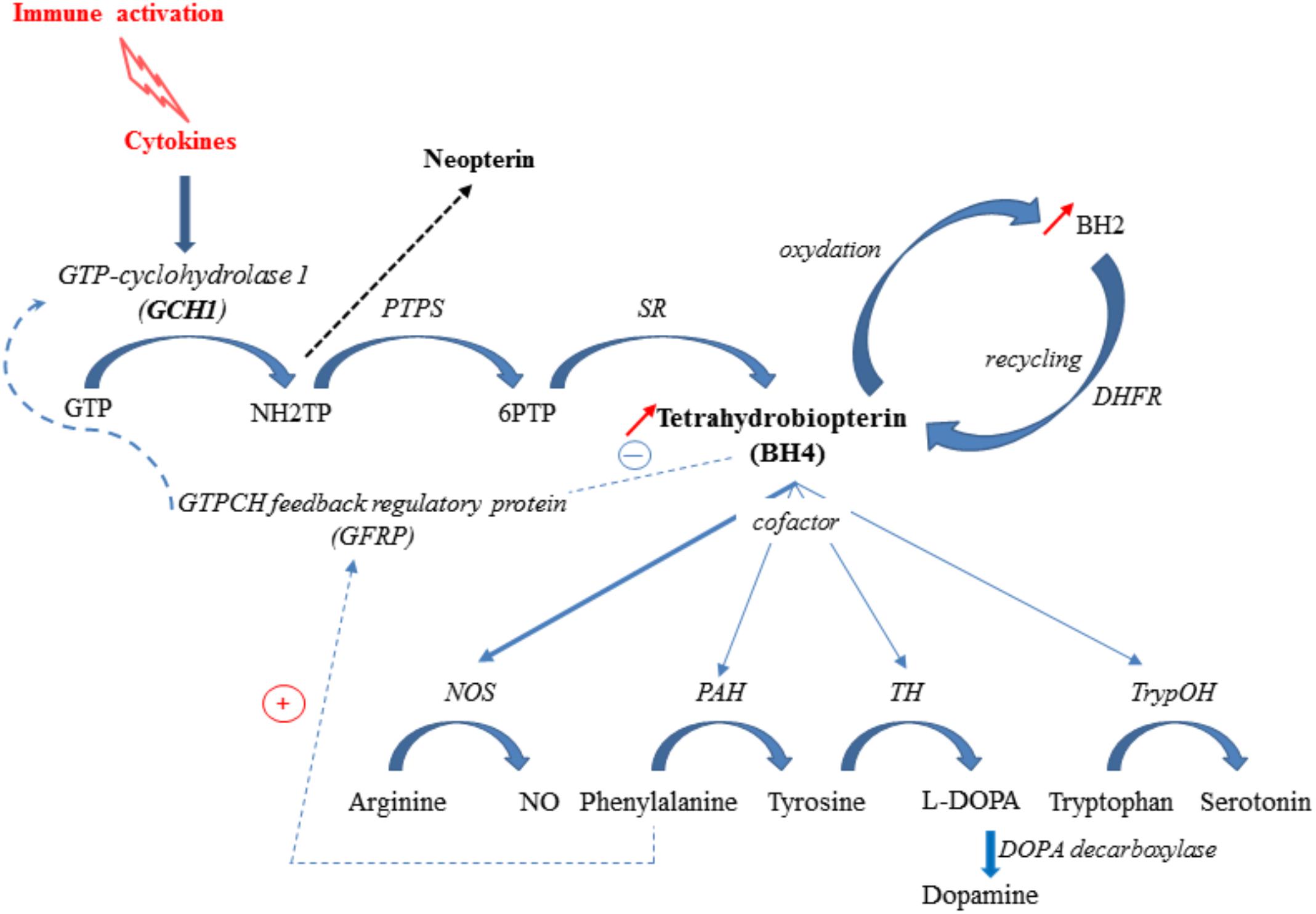
FIGURE 2. Inflammation-associated BH4 pathway. Cytokine production elicited in response to an immune challenge induces GTP-cyclohydrolase-1 (GCH1), 6-pyruvoyl-tetrahydropterin synthase (PTPS) and sepiapterin reductase (SR) activation (de novo synthesis pathway), leading to tetrahydrobiopterin (BH4) synthesis. GCH1 activity is modulated by the interaction of GTP-cyclohydrolase feedback protein (GFRP) and effectors molecules, BH4 and phenylalanine. BH4 is rapidly oxidized to BH2, which is subsequently reduced back to BH4 by the enzyme DHFR, representing a salvage pathway. In Human, PTPS becomes rate-limiting under inflammatory challenge and drives neopterin formation to the detriment of BH4. Under inflammatory conditions, BH4 is preferentially used as cofactor for NOS (nitric oxide synthase) activity, for synthesis of nitric oxide (NO), leading to a lower availability for PAH (phenylalanine hydroxylase), TH (tyrosine hydroxylase) and TrypOH (tryptophan hydroxylase) activities and reduced synthesis of monoamines. NH2TP: 7,8Dihydroneopterin triphosphate; 6PTP: 6Pyruvoyl-tetrahydrobiopterin.
Several clinical conditions have been associated with a defect in the BH4-pathway, mainly linked to alterations affecting the different enzymatic steps. Mutation of GCH1 results in greatly reduced BH4 level, which has been shown to cause neurological diseases, such as dopamine-responsive dystonia (Ichinose et al., 1994; Müller et al., 2002). Mice genetically deficient for BH4 (hph1 model) display decreased dopamine levels and increased depressive-like behaviors (Nasser et al., 2014). Supporting preclinical findings, patients with GCH1 deficiency have been shown to exhibit an increased frequency of psychiatric disorders, including depression and anxiety (Van Hove et al., 2006; Trender-Gerhard et al., 2009). Moreover, we have recently reported that variations in markers of GCH1 activity correlate with neurovegetative symptoms and inflammatory factors in elderly persons (Capuron et al., 2011). Low BH4 levels have also been found in postmortem brains of subjects with a history of severe depression (Blair et al., 1984). Conversely, raised urine and plasma total biopterin levels are measured in depressed patients (Duch et al., 1984; Garbutt et al., 1985; Knapp and Irwin, 1989; Hashimoto et al., 1994; Abou-Saleh et al., 1995), suggesting impaired BH4 metabolism. In addition, reduced GCH1 activity, assessed through the increased phenylalanine/tyrosine ratio, has been reported in depressive patients responding to electroconvulsive therapy (Anderson et al., 1994). This phenylalanine/tyrosine ratio is also used as indicator of BH4 availability and PAH activity and may serve therefore as an indirect biomarker of dopamine and norepinephrine synthesis (Neurauter et al., 2008; Capuron et al., 2011).
In Human, low PTPS activity directs the production of neopterin at the expense of BH4 in conditions of GCH1 stimulation, such as inflammation. Neopterin is therefore considered as a marker of cell-mediated immunity in inflammatory conditions (Murr et al., 2002). Increased phenylalanine levels and phenylalanine/tyrosine ratio is reported in patients suffering from chronic inflammatory conditions, this increase being correlated with neopterin concentrations (Neurauter et al., 2008; Ploder et al., 2008; Zangerle et al., 2010; Murr et al., 2014; Hirayama et al., 2016; Ormstad et al., 2016). It is well known that inflammatory stimulation activates the inducible NOS, which considerably increases the use of BH4 for optimal enzymatic activity, and induces formation of large amounts of oxygen radicals that in turn contribute to the oxidative loss of BH4 (Werner et al., 2003). Both increased use and loss of BH4 driven by a chronic inflammatory state may synergistically act to alter the function of BH4-dependent enzymes and then compromise the biosynthesis of monoamines, which may contribute to development of mood disorders (Neurauter et al., 2008; Felger et al., 2013a,b).
Preclinical studies show changes in brain dopamine and serotonin, and/or respective metabolite levels after an immune stimulation (Kamata et al., 2000; Kumai et al., 2000; Kitagami et al., 2003; Sato et al., 2006). Interestingly, inflammation-induced impairment of dopamine neurotransmission, which may involve induction of oxidative damages, has been proposed as a potential mechanism underlying motivational changes reported in LPS-challenged mice (Vichaya et al., 2018). Clinical studies also reveal some functional alterations of the dopaminergic reward system, in association with impairment in motivation and motor slowing (Capuron et al., 2007; Eisenberger et al., 2010; Capuron et al., 2012). Similar blunting of neural responses to reward has been observed in condition of dietary depletion of amino acid precursors for dopamine synthesis (Bjork et al., 2014). Decreased dopamine synthesis and release have been confirmed using microdialysis in monkeys and mice under inflammatory conditions (Felger and Treadway, 2017 for review). Some evidence also shows that inflammatory cytokines may alter the presynaptic dopamine storage through changes in expression and function of the vesicular monoamine transporter (VMAT2) (Kazumori et al., 2004). Moreover, functional changes of the dopamine reuptake pump, DAT, have been suggested in neuroinflammatory conditions associated to HIV infection or prenatal LPS challenge (Gelman et al., 2006; Tien et al., 2013). Then, a reduced presynaptic vesicular storage and/or altered DAT-reuptake of dopamine could together lead to reduced dopamine turnover in inflammatory conditions.
Based on these data, the BH4 pathway is emerging as an important regulator for a number of symptoms and pathologies associated with over-production of inflammatory mediators. Moreover, as previously mentioned recent data suggest a link between KP and BH4 pathways that could act synergistically upon inflammatory conditions to compromise monoamine synthesis. Thus, increased xanthurenic acid, a metabolite of 3-HK, has been shown to directly lower BH4 biosynthesis by inhibiting sepiapterin reductase (Haruki et al., 2016). Similarly, it has been suggested that a concurrent upregulation of kynurenines and BH2 production may lead to a combined up-regulated activity of NOS (by kynurenines) and decreased availability of BH4, as NOS cofactor (Oxenkrug, 2011). Such a combination results in an uncoupling of NOS and consequently reduced production of NO in favor of “inflammogenic” reactive oxygen species (ROS), which cause further oxidative BH4 loss. Altogether, these data strongly support the role of both kynurenine and BH4 pathways in development of inflammatory-related depressive symptoms.
Therapeutic Implications
One of the major issues when dealing with depressive disorders is the heightened resistance to standard antidepressant therapy (Rush et al., 2006). In that context, the possibility of alternatively and/or concomitantly targeting inflammatory processes to improve clinical outcomes has recently received particular attention (Schmidt et al., 2016; Colpo et al., 2018; Kappelmann et al., 2018; Shariq et al., 2018; Zuzarte et al., 2018). It has been postulated that most classical antidepressants known to primary act on monoamine neurotransmission [i.e., selective serotonin reuptake blockers: SSRIs, tricyclic antidepressants (TCA) acting on norepinephrine reuptake, and antidepressants acting on both serotonin and norepinephrine reuptake: SNRI] may also act on inflammation since they display anti-inflammatory properties both peripherally and within the brain (Lanquillon et al., 2000; Tynan et al., 2012; Strawbridge et al., 2015; Wiêdłocha et al., 2018). Importantly, they not only reduce circulating cytokine levels, but also downstream activation of the KP (Ara and Bano, 2012; Zoga et al., 2014; Reus et al., 2015), ultimately correcting the imbalance between neuroprotective and neurotoxic kynurenine metabolites (Kocki et al., 2012; Eskelund et al., 2017). Hence, sustained SSRI treatment in rodent models of depression reduces QA levels in different brain regions known to be involved in mood regulation (Eskelund et al., 2017). Moreover, variations in the genes coding for IDO and GCH1 have been shown to predict SSRI treatment outcome in depressive patients (Cutler et al., 2012; Kishi et al., 2012), further supporting a role for both pathways in the therapeutic response. Of note, the immune-modulatory impact of antidepressants differs depending on their class, with SSRI and SNRI drugs being mostly anti-inflammatory, while TCAs rather displaying in some studies pro-inflammatory properties (Hamer et al., 2011; Vogelzangs et al., 2012; Nazimek et al., 2016; Chen et al., 2018). Further highlighting the complexity of the relationship between antidepressants and inflammation, a given antidepressant drug can display both pro- or anti-inflammatory properties depending on what immune parameter is assessed (e.g., cytokine release or activation of specific intracellular signaling pathways) (Horowitz et al., 2014). Mounting evidence suggests that treatment resistance might be predicted by elevated inflammation, whether linked to specific gene variants (Baune et al., 2010), activation of selective intracellular pathways (Horowitz et al., 2014) or the presence of chronic inflammatory conditions/diseases (Luppino et al., 2010; Vogelzangs et al., 2012; Hughes and Kumari, 2017). In support of this notion, conditions such as overweight or obesity, which are characterized by a chronic low-grade inflammatory state together with a higher prevalence of depression, have been recently shown to relate to a greater risk of non-response to conventional antidepressants (Kloiber et al., 2007; Toups et al., 2013; Woo et al., 2016; Jantaratnotai et al., 2017). Interestingly, in those conditions, systemic inflammation is associated with both KP activation, as revealed by increased circulating kynurenine levels and expression of the KP enzymes – notably neurotoxic metabolites – in the adipose tissue of obese subjects (Favennec et al., 2015; Alemán et al., 2017), and mood symptoms (Capuron et al., 2008; Daly, 2013). Similar results were obtained in preclinical models of obesity (Dinel et al., 2011, 2014; André et al., 2014; Boitard et al., 2014; Castanon et al., 2015; Almeida-Suhett et al., 2017; de Cossio et al., 2017). Interestingly, weight loss that reduces inflammation and KP activation (Cancello and Clement, 2006; Alemán et al., 2017) correlates with significant mood improvement (mery et al., 2007; Capuron et al., 2011). Beside KP activation, studies reporting increased neopterin levels in obese (Brandacher et al., 2006; Oxenkrug et al., 2011; Mangge et al., 2014) also suggest the potential involvement of BH4 pathway in obesity-related depressive comorbidity, consistent with impairment of dopamine neurotransmission reported in obesity (Sharma and Fulton, 2013; Krishna et al., 2015).
Compelling evidence supports the notion that anti-inflammatory interventions may be effective as novel antidepressants or adjuvants of conventional antidepressants, as long as inflammation and depressive symptoms are comorbid in treated patients (Strawbridge et al., 2015; Kappelmann et al., 2018; Köhler et al., 2016; Schmidt et al., 2016; Jantaratnotai et al., 2017; Jha and Trivedi, 2018) (Table 1). For example, non-steroidal anti-inflammatory drugs (NSAIDs) improve antidepressant treatment outcomes in patients with depressive disorders (Müller et al., 2006; Akhondzadeh et al., 2009). Similarly, NSAIDs administration decreases severity of emotional alterations in several animal models of inflammatory diseases, such as cancer (Norden et al., 2015), Alzheimer’s disease (Llorens-Martin et al., 2014), and Parkinson’s disease (Zaminelli et al., 2014). Significant antidepressant effects of the tetracycline antibiotic minocycline have also been reported in depressed patients compared to placebo (for review Rosenblat and McIntyre, 2018). It is also worth mentioning that natural anti-inflammatory agents, such as ω-3 polyunsaturated fatty acids (PUFAs), have also shown promising results on mood, particularly as add-on therapy with conventional antidepressants (for review Layé et al., 2018). Supporting the link between obesity and depression, this strategy revealed to be particularly effective in depressed patients with low-grade basal inflammation (Rapaport et al., 2016), which is linked to elevated body mass index (BMI). Interestingly, supplementation with ω-3 PUFAs in obese subjects also accentuates weight loss induced by low-calorie diet (Kunesová et al., 2006), weight loss in the context of obesity being associated – as mentioned earlier – with reduced inflammation (Lasselin et al., 2014) and improved depressive symptoms (Emery et al., 2007; Capuron et al., 2011). Current knowledge on the implication of specific inflammatory pathways in driving neuropsychiatric symptoms also offers the potential for targeted anti-inflammatory interventions. Thus, monoclonal antibodies against IL-6, IL-17, and TNF-α display antidepressant effects in chronically inflamed patients with significant depressive symptoms, as well as depressed patients with basal low-grade inflammation (Traki et al., 2014; Gossec et al., 2015; Griffiths et al., 2017; Jha and Trivedi, 2018; Kappelmann et al., 2018). Abundant literature also reports antidepressant effects of anti-TNF-α drugs, such as etanercept or infliximab, in clinical trials (Kekow et al., 2010; Arisoy et al., 2013; Raison et al., 2013; Fleming et al., 2015) and murine models of inflammatory diseases (Haji et al., 2012; Bayramgürler et al., 2013). Of note, targeting TNF-α in treatment-resistant depressed patients was found to improve depressive symptomatology particularly in those with higher baseline inflammation (Raison et al., 2013), which tended to be those with higher BMI. In addition, chronic stress-induced depressive-like behaviors are reduced by TNF-α antagonism through decreased IDO activation (Fu et al., 2016).
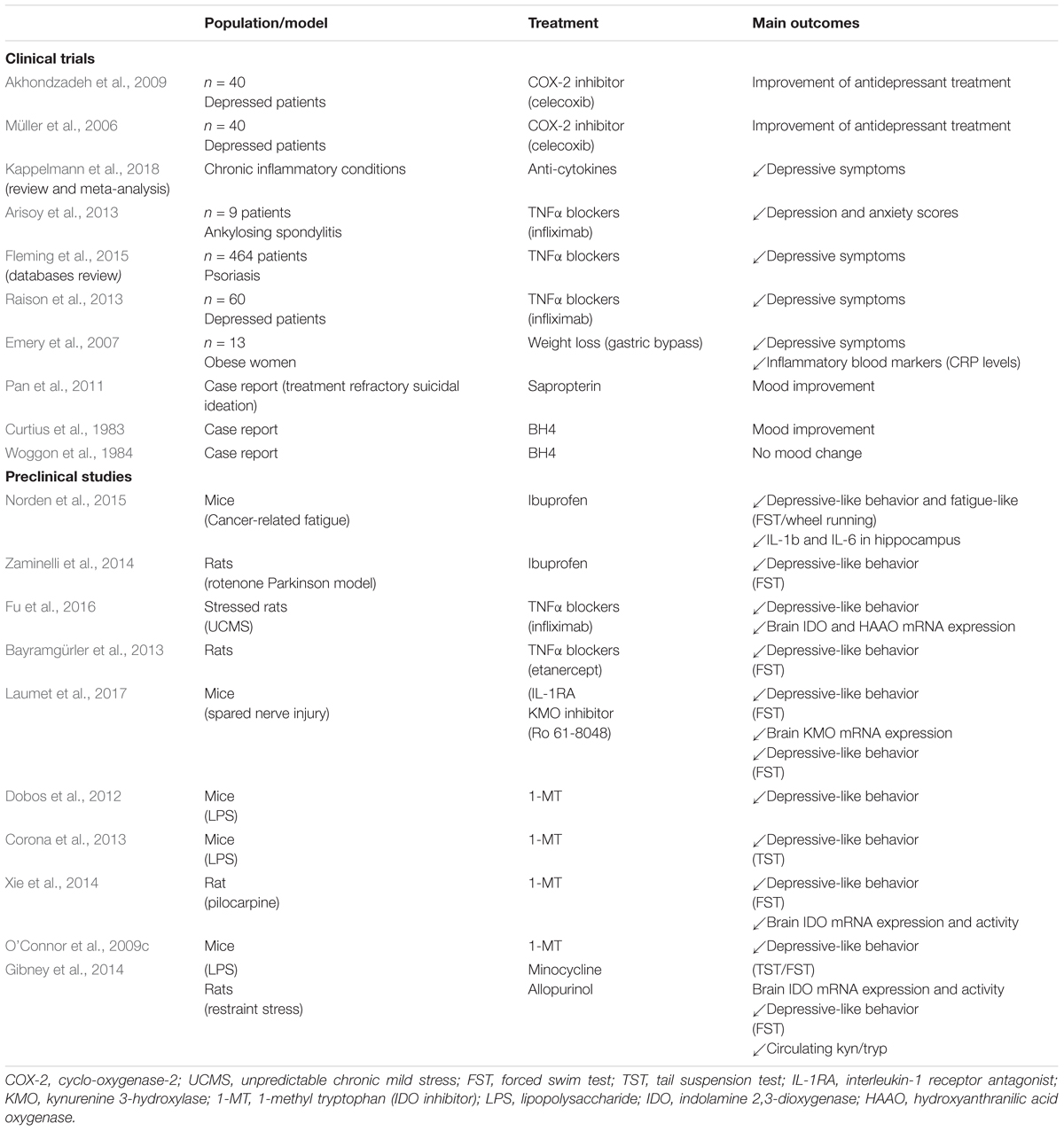
TABLE 1. Studies investigating antidepressant interventions targeting inflammation, kynurenine or BH4 pathways.
The possibility of acting on brain neurobiological targets of inflammation, such as the KP, rather than on inflammation itself has also drawn much attention, particularly since global anti-inflammatory strategies are often accompanied by important side effects. Thus, compelling preclinical studies report that reducing KP activation promotes antidepressant effects (Reus et al., 2015; Remus and Dantzer, 2016; Jeon and Kim, 2017; Lovelace et al., 2017; O’Farrell and Harkin, 2017). First promising on-trial strategies include blockade of KP enzymes, particularly IDO and KMO, which has been shown to display antidepressant-like properties whose efficiency varies, however, depending on what symptom domains were assessed (Toledo-Sherman et al., 2015; Laumet et al., 2017; Lovelace et al., 2017; Tashiro et al., 2017). Instead of directly targeting KP enzymes, other studies have rather investigated the possibility of reducing the peripheral kynurenine transport across the blood–brain barrier, as the majority of brain kynurenine comes from the periphery. This procedure has been indeed shown to prevent production of QA by activated microglia (Carrillo-Mora et al., 2010), as well as LPS-induced depressive-like behavior (Remus and Dantzer, 2016). Alternatively, it may be worthwhile to increase synthesis/availability of KA, the neuroprotective kynurenine metabolite (Vécsei et al., 2013). By counteracting the neurotoxic effect of QA and other NMDA receptor agonists, increasing KA formation has been shown to reduce neuronal damages and associated experimentally-induced seizures (Russi et al., 1992; Silva-Adaya et al., 2011). Similar beneficial effects still need to be confirmed regarding inflammation-related depressive symptoms, but these data are already very encouraging. Lastly, in agreement with the literature that links glutamate with mood disorders and highlights antidepressant effects of NMDA receptor antagonists such as ketamine (see for review Haroon et al., 2017), several interesting studies suggest that targeting glutamate activity or preventing QA from activating NMDA receptors also offer additional therapeutic opportunities (Dantzer and Walker, 2014; Reus et al., 2015). Blocking activation of those receptors, which was already shown to protect against chronic stress-induced depressive-like behavior (Li et al., 2011), are similarly effective regarding LPS-induced behavioral alterations (Walker et al., 2013). Moreover, IDO deficient mice are less sensitive to QA-induced neuronal damages (Mazarei et al., 2013). Further supporting the role of NMDA receptor activation as potential therapeutic targets to improve inflammation-related depressive symptoms, antidepressant treatment was recently shown to reduce both stress-induced activation of the KP and changes of NMDA receptor expression (Martín-Hernández et al., 2018). Similarly, reducing NMDA receptor activation by enhancing brain KYNA levels has been proposed as a promising way of counteracting amyloid beta-related neurodegeneration (Carrillo-Mora et al., 2010).
Even if the potential antidepressant effect of targeting the BH4 pathway, for example with oral BH4 supplementation, has been only poorly studied so far and with inconsistent results (Curtius et al., 1983; Woggon et al., 1984), supplementation with synthetic BH4 is already used to treat patients suffering from phenylketonuria (Blau, 2013). Moreover, an interesting case report shows that administrating a BH4 replacement protein improves depressive symptoms in a patient suffering from major depression (Pan et al., 2011). Preclinical data also highlight the possibility of increasing brain BH4 levels through its peripheral administration (Ohashi et al., 2016), which consequently changes serotonin and dopamine metabolism (Brand et al., 1996), TH protein content (Homma et al., 2013), dopamine levels and neuronal activities in the brain (Koshimura et al., 2000). More studies are necessary to test if such BH4-induced changes in dopamine and norepinephrine neurotransmission might underlie the expected behavioral improvement, particularly regarding depression-related anhedonic symptoms.
Altogether, these findings clearly show that targeting, either directly or indirectly, inflammation and/or neurobiological mediators shown to underlie inflammation-driven depressive symptoms represents promising new therapeutic strategies (Figure 3). These strategies may not only rely on pharmacological approaches using currently available drugs prescribed for their antidepressant properties, but also on the use of still to discover/validate drugs. Regarding the KP, the potential beneficial effects of KP enzyme inhibitors are currently considered in other medical fields, particularly in oncology (see for review Prendergast et al., 2018). Studies carried out in the context of inflammation-driven depressive symptoms should likely benefit therefore from findings reported in other fields. Beside pharmacological approaches, non-pharmacological therapeutic strategies, such as nutritional interventions, may represent a promising alternative associated with reduced risk of complications and economic cost. This particularly includes diet supplementation with natural agents such as ω-3 PUFAs, amino acids or antioxidants, namely compounds known to ultimately protect against inflammation, oxidative stress and/or other neurotoxic insults. Importantly, the opportunity to combine these different therapeutic strategies or rather favor one of them according to the type of depressive symptoms to treat, should broaden the spectrum of action of currently used antidepressant interventions and therefore help improving the management and/or treatment of depressive symptoms associated with inflammation.
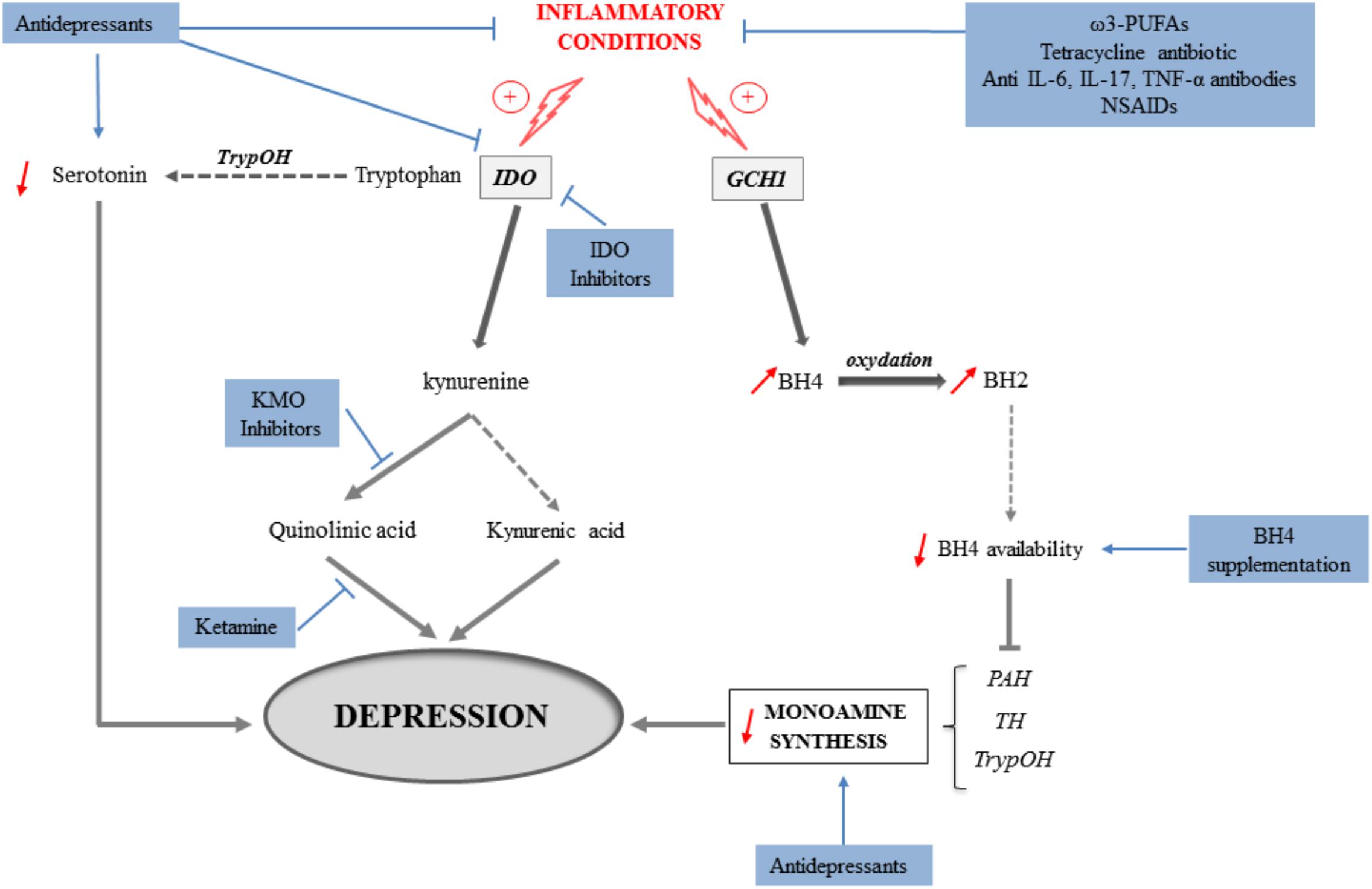
FIGURE 3. Mechanisms involved in the induction of depressive symptoms in inflammatory conditions (gray arrows) and potential therapeutic implications following pharmacological or nutritional interventions (blue arrows). Activation of the indoleamine 2,3-dioxygenase (IDO) in inflammatory conditions leads to the production of kynurenine from tryptophan, at the expense of serotonin production. The synthesis of serotonin and other monoamines is also impaired because of reduced bioavailability of tetrahydrobiopterin (BH4) that results from the induction of GTP-cyclohydrolase-1 (GCH1) by cytokines. By concomitantly activating the kynurenine monooxygenase (KMO), inflammation promotes the production of the neurotoxic metabolite quinolinic acid, while synthesis of kynurenic acid, rather neuroprotective, is reduced. Both inflammation-induced reduction of monoamine production and increase of neuronal damages ultimately contribute to the development of depressive symptoms. In that context, different therapeutic strategies targeting these mechanisms can be identified. They include: classical antidepressants that aim to increase monoamine synthesis, but also able of reducing inflammation or kynurenine pathway activation; anti-inflammatory interventions with antibiotics, ω3-PUFAs, anti-cytokine antibodies or NSAIDs; IDO or KMO inhibitors; antagonists of the NMDA receptors such as ketamine, and BH4 supplementation. PAH, phenylalanine hydroxylase; TH, tyrosine hydroxylase; TrypOH, tryptophan hydroxylase.
Conclusion
Given the steadily rising prevalence of depression and inflammation-based chronic diseases, their combined negative impact on the etiology of other severe diseases, and the elevated resistance to conventional antidepressants, a better understanding of the pathophysiological mechanisms of inflammation-driven depressive symptoms is urgently required for the identification of new and efficacious therapeutic strategies. Altogether, findings discussed in the present review show that strategies directed to inflammatory processes might be particularly promising, because of their pivotal role in the pathophysiology of depression and their substantial impact on the metabolism of monoamines involved in the regulation of mood. Despite the important advances made over the last decade, several issues are still at stake, from the identification of the best candidates to be targeted within the inflammatory pathways to the determination of the most suitable protocol of treatment (i.e., anti-inflammatory strategies as treatment or co-treatment with conventional antidepressants). Resolving these issues, which clearly depend on the patient’s individual characteristics, constitutes a crucial challenge for the future, as it should allow personalizing antidepressant prescription, and in turn contributing to the development of a precision medicine in psychiatry.
Author Contributions
All authors listed have made a substantial, direct and intellectual contribution to the work, and approved it for publication.
Funding
This work was supported by subventions from the Institut National de la Recherche Agronomique (INRA), the Region Aquitaine (2013-13-03-001; NC), the Société Francophone du Diabète (SFD) (220009S1; NC), the Fondation pour la Recherche Médicale (FRM) (DPP20151033943; LC) and ERANET-NEURON (ANR-13-NEUR-0004-03; LC).
Conflict of Interest Statement
The authors declare that the research was conducted in the absence of any commercial or financial relationships that could be construed as a potential conflict of interest.
References
Abou-Saleh, M., Anderson, D. N., Collins, J., Hughes, K., Cattell, R. J., Hamon, C. G., et al. (1995). The role of pterins in depression and the effects of antidepressive therapy. Biol. Psychiatry 38, 458–463. doi: 10.1016/0006-3223(94)00323-U
Agudelo, L. Z., Femenia, T., Orhan, F., Porsmyr-Palmertz, M., Goiny, M., Martinez-Redondo, V., et al. (2014). Skeletal muscle PGC-1alpha1 modulates kynurenine metabolism and mediates resilience to stress-induced depression. Cell 159, 33–45. doi: 10.1016/j.cell.2014.07.051
Akhondzadeh, S., Jafari, S., Raisi, F., Nasehi, A. A., Ghoreishi, A., Salehi, B., et al. (2009). Clinical trial of adjunctive celecoxib treatment in patients with major depression: a double blind and placebo controlled trial. Depress. Anxiety 26, 607–611. doi: 10.1002/da.20589
Alemán, J. O., Iyengar, N. M., Walker, J. M., Milne, G. L., Da Rosa, J. C., Liang, Y., et al. (2017). Effects of rapid weight loss on systemic and adipose tissue inflammation and metabolism in obese postmenopausal women. J. Endocr. Soc. 1, 625–637. doi: 10.1210/js.2017-00020
Almeida-Suhett, C. P., Graham, A., Chen, Y., and Deuster, P. (2017). Behavioral changes in male mice fed a high-fat diet are associated with IL-1beta expression in specific brain regions. Physiol. Behav. 169, 130–140. doi: 10.1016/j.physbeh.2016.11.016
Anderson, D. N., Wilkinson, A. M., Abou-Saleh, M. T., and Blair, J. A. (1994). Recovery from depression after electroconvulsive therapy is accompanied by evidence of increased tetrahydrobiopterin-dependent hydroxylation. Acta Psychiatr. Scand. 90, 10–13. doi: 10.1111/j.1600-0447.1994.tb01547.x
André, C., Dinel, A. L., Ferreira, G., Layé, S., and Castanon, N. (2014). Diet-induced obesity progressively alters cognition, anxiety-like behavior and lipopolysaccharide-induced depressive-like behavior: focus on brain indoleamine 2,3-dioxygenase activation. Brain Behav. Immun. 41, 10–21. doi: 10.1016/j.bbi.2014.03.012
André, C., O’Connor, J. C., Kelley, K. W., Lestage, J., Dantzer, R., and Castanon, N. (2008). Spatio-temporal differences in the profile of murine brain expression of proinflammatory cytokines and indoleamine 2,3-dioxygenase in response to peripheral lipopolysaccharide administration. J. Neuroimmunol. 200, 90–99. doi: 10.1016/j.jneuroim.2008.06.011
Ara, I., and Bano, S. (2012). Citalopram decreases tryptophan 2,3-dioxygenase activity and brain 5-HT turnover in swim stressed rats. Pharmacol. Rep. 64, 558–566. doi: 10.1016/S1734-1140(12)70851-4
Arisoy, O., Bes, C., Cifci, C., Sercan, M., and Soy, M. (2013). The effect of TNF-alpha blockers on psychometric measures in ankylosing spondylitis patients: a preliminary observation. Rheumatol. Int. 33, 1855–1864. doi: 10.1007/s00296-013-2671-x
Barbosa, I. G., Rocha, N. P., Bauer, M. E., de Miranda, A. S., Huguet, R. B., and Reis, H. J. (2013). Chemokines in bipolar disorder: trait or state? Eur. Arch. Psychiatry Clin. Neurosci. 263, 159–165. doi: 10.1007/s00406-012-0327-6
Baune, B. T., Dannlowski, U., Domschke, K., Janssen, D. G., Jordan, M. A., Ohrmann, P., et al. (2010). The interleukin 1 beta (IL1B) gene is associated with failure to achieve remission and impaired emotion processing in major depression. Biol. Psychiatry 67, 543–549. doi: 10.1016/j.biopsych.2009.11.004
Bayramgürler, D., Karson, A., Ozer, C., and Utkan, T. (2013). Effects of long-term etanercept treatment on anxiety- and depression-like neurobehaviors in rats. Physiol. Behav. 119, 145–148. doi: 10.1016/j.physbeh.2013.06.010
Bay-Richter, C., Linderholm, K. R., Lim, C. K., Samuelsson, M., Träskman-Bendz, L., Guillemin, G. J., et al. (2015). A role for inflammatory metabolites as modulators of the glutamate N-methyl-D-aspartate receptor in depression and suicidality. Brain Behav. Immun. 43, 110–117. doi: 10.1016/j.bbi.2014.07.012
Biesmans, S., Meert, T. F., Bouwknecht, J. A., Acton, P. D., Davoodi, N., De Haes, P., et al. (2013). Systemic immune activation leads to neuroinflammation and sickness behavior in mice. Mediators Inflamm. 271359. doi: 10.1155/2013/271359
Bjork, J. M., Grant, S. J., Chen, G., and Hommer, D. W. (2014). Dietary tyrosine/phenylalanine depletion effects on behavioral and brain signatures of human motivational processing. Neuropsychopharmacology 39, 595–604. doi: 10.1038/npp.2013.232
Blair, J. A., Barford, P. A., Morar, C., Pheasant, A. E., Hamon, C. G., Whitburn, S. B., et al. (1984). Tetrahydrobiopterin metabolism in depression. Lancet 2:163. doi: 10.1016/S0140-6736(84)91075-4
Blau, N. (2013). Sapropterin dihydrochloride for the treatment of hyperphenylalaninemias. Expert Opin. Drug Metab. Toxicol. 9, 1207–1218. doi: 10.1517/17425255.2013.804064
Boitard, C., Cavaroc, A., Sauvant, J., Aubert, A., Castanon, N., Laye, S., et al. (2014). Impairment of hippocampal-dependent memory induced by juvenile high-fat diet intake is associated with enhanced hippocampal inflammation in rats. Brain Behav. Immun. 40, 9–17. doi: 10.1016/j.bbi.2014.03.005
Brand, M. P., Hyland, K., Engle, T., Smith, I., and Heales, S. J. (1996). Neurochemical effects following peripheral administration of tetrahydropterin derivatives to the hph-1 mouse. J. Neurochem. 66, 1150–1156. doi: 10.1046/j.1471-4159.1996.66031150.x
Brandacher, G., Winkler, C., Aigner, F., Schwelberger, H., Schroecksnadel, K., Margreiter, R., et al. (2006). Bariatric surgery cannot prevent tryptophan depletion due to chronic immune activation in morbidly obese patients. Obes. Surg. 16, 541–548. doi: 10.1381/096089206776945066
Cancello, R., and Clement, K. (2006). Is obesity an inflammatory illness? Role of low-grade inflammation and macrophage infiltration in human white adipose tissue. BJOG 113, 1141–1147. doi: 10.1111/j.1471-0528.2006.01004.x
Capuron, L., and Castanon, N. (2017). Role of inflammation in the development of neuropsychiatric symptom domains: evidence and mechanisms. Curr. Top. Behav. Neurosci. 31, 31–44. doi: 10.1007/7854_2016_14
Capuron, L., Gumnick, J. F., Musselman, D. L., Lawson, D. H., Reemsnyder, A., Nemeroff, C. B., et al. (2002). Neurobehavioral effects of interferon-alpha in cancer patients: phenomenology and paroxetine responsiveness of symptom dimensions. Neuropsychopharmacology 26, 643–652. doi: 10.1016/S0893-133X(01)00407-9
Capuron, L., Lasselin, J., and Castanon, N. (2017). Role of adiposity-driven inflammation in depressive morbidity. Neuropsychopharmacology 42, 115–128. doi: 10.1038/npp.2016.123
Capuron, L., and Miller, A. H. (2011). Immune system to brain signaling: neuropsychopharmacological implications. Pharmacol. Ther. 130, 226–238. doi: 10.1016/j.pharmthera.2011.01.014
Capuron, L., Pagnoni, G., Demetrashvili, M. F., Lawson, D. H., Fornwalt, F. B., Woolwine, B., et al. (2007). Basal ganglia hypermetabolism and symptoms of fatigue during interferon-alpha therapy. Neuropsychopharmacology 32, 2384–2392. doi: 10.1038/sj.npp.1301362
Capuron, L., Pagnoni, G., Drake, D. F., Woolwine, B. J., Spivey, J. R., Crowe, R. J., et al. (2012). Dopaminergic mechanisms of reduced basal ganglia responses to hedonic reward during interferon alfa administration. Arch. Gen. Psychiatry 69, 1044–1053. doi: 10.1001/archgenpsychiatry.2011.2094
Capuron, L., Schroecksnadel, S., Feart, C., Aubert, A., Higueret, D., Barberger-Gateau, P., et al. (2011). Chronic low-grade inflammation in elderly persons is associated with altered tryptophan and tyrosine metabolism: role in neuropsychiatric symptoms. Biol. Psychiatry 70, 175–182. doi: 10.1016/j.biopsych.2010.12.006
Capuron, L., Su, S., Miller, A. H., Bremner, J. D., Goldberg, J., Vogt, G. J., et al. (2008). Depressive symptoms and metabolic syndrome: is inflammation the underlying link? Biol. Psychiatry 64, 896–900. doi: 10.1016/j.biopsych.2008.05.019
Carrillo-Mora, P., Mendez-Cuesta, L. A., Perez-De, La Cruz, V., Fortoul-van Der, Goes, T. I., et al. (2010). Protective effect of systemic L-kynurenine and probenecid administration on behavioural and morphological alterations induced by toxic soluble amyloid beta (25-35) in rat hippocampus. Behav. Brain Res. 210, 240–250. doi: 10.1016/j.bbr.2010.02.041
Castanon, N. (2015). “Animal models to study the role of kynurenine pathway in mood, behavior and cognition,” in Targeting the Broadly Pathogenic Kynurenine Pathway, ed. S. Mittal (Berlin: Springer), 323–337. doi: 10.1007/978-3-319-11870-3_25
Castanon, N., Lasselin, J., and Capuron, L. (2014). Neuropsychiatric comorbidity in obesity: role of inflammatory processes. Front. Endocrinol. 5:74. doi: 10.3389/fendo.2014.00074
Castanon, N., Luheshi, G., and Laye, S. (2015). Role of neuroinflammation in the emotional and cognitive alterations displayed by animal models of obesity. Front. Neurosci. 9:229. doi: 10.3389/fnins.2015.00229
Castanon, N., Medina, C., Mormede, C., and Dantzer, R. (2004). Chronic administration of tianeptine balances lipopolysaccharide-induced expression of cytokines in the spleen and hypothalamus of rats. Psychoneuroendocrinology 29, 778–790. doi: 10.1016/S0306-4530(03)00142-2
Chen, C. Y., Yeh, Y. W., Kuo, S. C., Liang, C. S., Ho, P. S., Huang, C. C., et al. (2018). Differences in immunomodulatory properties between venlafaxine and paroxetine in patients with major depressive disorder. Psychoneuroendocrinology 87, 108–118. doi: 10.1016/j.psyneuen.2017.10.009
Choi, Y. K., and Tarazi, F. I. (2010). Alterations in dopamine and glutamate neurotransmission in tetrahydrobiopterin deficient spr-/- mice: relevance to schizophrenia. BMB Rep. 43, 593–598. doi: 10.5483/BMBRep.2010.43.9.593
Colpo, G. D., Leboyer, M., Dantzer, R., Trivedi, M. H., and Teixeira, A. L. (2018). Immune-based strategies for mood disorders: facts and challenges. Expert Rev. Neurother. 18, 139–152. doi: 10.1080/14737175.2018.1407242
Corona, A. W., Norden, D. M., Skendelas, J. P., Huang, Y., O’Connor, J. C., Lawson, M., et al. (2013). Indoleamine 2,3-dioxygenase inhibition attenuates lipopolysaccharide induced persistent microglial activation and depressive-like complications in fractalkine receptor (CX(3)CR1)-deficient mice. Brain Behav. Immun. 31, 134–142. doi: 10.1016/j.bbi.2012.08.008
Curtius, H. C., Niederwieser, A., Levine, R. A., Lovenberg, W., Woggon, B., and Angst, J. (1983). Successful treatment of depression with tetrahydrobiopterin. Lancet 1, 657–658. doi: 10.1016/S0140-6736(83)91837-8
Cutler, J. A., Rush, A. J., McMahon, F. J., and Laje, G. (2012). Common genetic variation in the indoleamine-2,3-dioxygenase genes and antidepressant treatment outcome in major depressive disorder. J. Psychopharmacol. 26, 360–367. doi: 10.1177/0269881111434622
Daly, M. (2013). The relationship of C-reactive protein to obesity-related depressive symptoms: a longitudinal study. Obesity 21, 248–250. doi: 10.1002/oby.20051
Dantzer, R., O’Connor, J. C., Freund, G. G., Johnson, R. W., and Kelley, K. W. (2008). From inflammation to sickness and depression: when the immune system subjugates the brain. Nat. Rev. Neurosci. 9, 46–56. doi: 10.1038/nrn2297
Dantzer, R., and Walker, A. K. (2014). Is there a role for glutamate-mediated excitotoxicity in inflammation-induced depression? J. Neural Transm. 121, 925–932. doi: 10.1007/s00702-014-1187-1
Dassesse, D., Hemmens, B., Cuvelier, L., and Résibois, A. (1997). GTP-cyclohydrolase-I like immunoreactivity in rat brain. Brain Res. 777, 187–201. doi: 10.1016/S0006-8993(97)01111-6
de Cossio, L. F., Fourrier, C., Sauvant, J., Everard, A., Capuron, L., Cani, P. D., et al. (2017). Impact of prebiotics on metabolic and behavioral alterations in a mouse model of metabolic syndrome. Brain Behav. Immun. 64, 33–49. doi: 10.1016/j.bbi.2016.12.022
Dinel, A. L., André, C., Aubert, A., Ferreira, G., Laye, S., and Castanon, N. (2011). Cognitive and emotional alterations are related to hippocampal inflammation in a mouse model of metabolic syndrome. PLoS One 6:e24325. doi: 10.1371/journal.pone.0024325
Dinel, A. L., André, C., Aubert, A., Ferreira, G., Laye, S., and Castanon, N. (2014). Lipopolysaccharide-induced brain activation of the indoleamine 2,3-dioxygenase and depressive-like behavior are impaired in a mouse model of metabolic syndrome. Psychoneuroendocrinology 40, 48–59. doi: 10.1016/j.psyneuen.2013.10.014
Dobos, N., de Vries, E. F., Kema, I. P., Patas, K., Prins, M., Nijholt, I. M., et al. (2012). The role of indoleamine 2,3-dioxygenase in a mouse model of neuroinflammation-induced depression. J. Alzheimers Dis. 28, 905–915. doi: 10.3233/JAD-2011-111097
Dowlati, Y., Herrmann, N., Swardfager, W., Liu, H., Sham, L., Reim, E. K., et al. (2010). A meta-analysis of cytokines in major depression. Biol. Psychiatry 67, 446–457. doi: 10.1016/j.biopsych.2009.09.033
Duch, D. S., Woolf, J. H., Nichol, C. A., Davidson, J. R., and Garbutt, J. C. (1984). Urinary excretion of biopterin and neopterin in psychiatric disorders. Psychiatry Res. 11, 83–89. doi: 10.1016/0165-1781(84)90090-8
Eisenberger, N. I., Berkman, E. T., Inagaki, T. K., Rameson, L. T., Mashal, N. M., and Irwin, M. R. (2010). Inflammation-induced anhedonia: endotoxin reduces ventral striatum responses to reward. Biol. Psychiatry 68, 748–754. doi: 10.1016/j.biopsych.2010.06.010
Emery, C. F., Fondow, M. D., Schneider, C. M., Christofi, F. L., Hunt, C., Busby, A. K., et al. (2007). Gastric bypass surgery is associated with reduced inflammation and less depression: a preliminary investigation. Obes. Surg. 17, 759–763. doi: 10.1007/s11695-007-9140-0
Engler, H., Brendt, P., Wischermann, J., Wegner, A., Rohling, R., Schoemberg, T., et al. (2017). Selective increase of cerebrospinal fluid IL-6 during experimental systemic inflammation in humans: association with depressive symptoms. Mol. Psychiatry 22, 1448–1454. doi: 10.1038/mp.2016.264
Eskelund, A., Li, Y., Budac, D. P., Müller, H. K., Gulinello, M., Sanchez, C., et al. (2017). Drugs with antidepressant properties affect tryptophan metabolites differently in rodent models with depression-like behavior. J. Neurochem. 142, 118–131. doi: 10.1111/jnc.14043
Evans, D. L., Charney, D. S., Lewis, L., Golden, R. N., Gorman, J. M., Krishnan, K. R., et al. (2005). Mood disorders in the medically ill: scientific review and recommendations. Biol. Psychiatry 58, 175–189. doi: 10.1016/j.biopsych.2005.05.001
Favennec, M., Hennart, B., Caiazzo, R., Leloire, A., Yengo, L., Verbanck, M., et al. (2015). The kynurenine pathway is activated in human obesity and shifted toward kynurenine monooxygenase activation. Obesity 10, 2066–2074. doi: 10.1002/oby.21199
Felger, J. C., Li, L., Marvar, P. J., Woolwine, B. J., Harrison, D. G., Raison, C. L., et al. (2013a). Tyrosine metabolism during interferon-alpha administration: association with fatigue and CSF dopamine concentrations. Brain Behav. Immun. 31, 153–160. doi: 10.1016/j.bbi.2012.10.010
Felger, J. C., Mun, J., Kimmel, H. L., Nye, J. A., Drake, D. F., Hernandez, C. R., et al. (2013b). Chronic interferon-α decreases dopamine 2 receptor binding and striatal dopamine release in association with anhedonia-like behavior in nonhuman primates. Neuropsychopharmacology 38, 2179–2187. doi: 10.1038/npp.2013.115
Felger, J. C., and Treadway, M. T. (2017). Inflammation effects on motivation and motor activity: role of dopamine. Neuropsychopharmacology 42, 216–241. doi: 10.1038/npp.2016.143
Fleming, P., Roubille, C., Richer, V., Starnino, T., McCourt, C., McFarlane, A., et al. (2015). Effect of biologics on depressive symptoms in patients with psoriasis: a systematic review. J. Eur. Acad. Dermatol. Venereol. 29, 1063–1070. doi: 10.1111/jdv.12909
Frenois, F., Moreau, M., O’Connor, J., Lawson, M., Micon, C., Lestage, J., et al. (2007). Lipopolysaccharide induces delayed FosB/DeltaFosB immunostaining within the mouse extended amygdala, hippocampus and hypothalamus, that parallel the expression of depressive-like behavior. Psychoneuroendocrinology 32, 516–531. doi: 10.1016/j.psyneuen.2007.03.005
Fu, X., Zunich, S. M., O’Connor, J. C., Kavelaars, A., Dantzer, R., and Kelley, K. W. (2010). Central administration of lipopolysaccharide induces depressive-like behavior in vivo and activates brain indoleamine 2,3 dioxygenase in murine organotypic hippocampal slice cultures. J. Neuroinflammation 7:43. doi: 10.1186/1742-2094-7-43
Fu, X. Y., Li, H. Y., Jiang, Q. S., Cui, T., Jiang, X. H., Zhou, Q. X., et al. (2016). Infliximab ameliorating depression-like behavior through inhibiting the activation of the IDO-HAAO pathway mediated by tumor necrosis factor-alpha in a rat model. Neuroreport 27, 953–959. doi: 10.1097/WNR.0000000000000637
Garbutt, J. C., Duch, D. S., Nichol, C. A., and Woolf, J. H. (1985). Urinary biopterin and neopterin excretion and pituitary-adrenal activity in psychiatric patients. Psychiatry Res. 16, 181–187. doi: 10.1016/0165-1781(85)90105-2
Gelman, B. B., Spencer, J. A., Holzer, C. E. III, and Soukup, V. M. (2006). Abnormal striatal dopaminergic synapses in National NeuroAIDS Tissue Consortium subjects with HIV encephalitis. J. Neuroimmune Pharmacol. 1, 410–420. doi: 10.1007/s11481-006-9030-6
Gibney, S. M., Fagan, E. M., Waldron, A. M., O’Byrne, J., Connor, T. J., and Harkin, A. (2014). Inhibition of stress-induced hepatic tryptophan 2,3-dioxygenase exhibits antidepressant activity in an animal model of depressive behavior. Int. J. Neuropsychopharmacol. 17, 917–928. doi: 10.1017/S1461145713001673
Gibney, S. M., McGuinness, B., Prendergast, C., Harkin, A., and Connor, T. J. (2013). Poly I:C-induced activation of the immune response is accompanied by depression and anxiety-like behaviours, kynurenine pathway activation and reduced BDNF expression. Brain Behav. Immun. 28, 170–181. doi: 10.1016/j.bbi.2012.11.010
Godbout, J. P., Moreau, M., Lestage, J., Chen, J., Sparkman, N. L., O’Connor, J., et al. (2008). Aging exacerbates depressive-like behavior in mice in response to activation of the peripheral innate immune system. Neuropsychopharmacology 33, 2341–2351. doi: 10.1038/sj.npp.1301649
Gossec, L., Steinberg, G., Rouanet, S., and Combe, B. (2015). Fatigue in rheumatoid arthritis: quantitative findings on the efficacy of tocilizumab and on factors associated with fatigue. The French multicentre prospective PEPS Study. Clin. Exp. Rheumatol. 33, 664–670.
Griffiths, C. E. M., Thaçi, D., Gerdes, S., Arenberger, P., Pulka, G., Kingo, K., et al. (2017). The EGALITY study: a confirmatory, randomized, double-blind study comparing the efficacy, safety and immunogenicity of GP2015, a proposed etanercept biosimilar, vs. the originator product in patients with moderate-to-severe chronic plaque-type psoriasis. Br. J. Dermatol. 176, 928–938. doi: 10.1111/bjd.15152
Haji, N., Mandolesi, G., Gentile, A., Sacchetti, L., Fresegna, D., Rossi, S., et al. (2012). TNF-(-mediated anxiety in a mouse model of multiple sclerosis. Exp. Neurol. 237, 296–303. doi: 10.1016/j.expneurol.2012.07.010
Hamer, M., Batty, G. D., Marmot, M. G., Singh-Manoux, A., and Kivimäki, M. (2011). Anti-depressant medication use and C-reactive protein: results from two population-based studies. Brain Behav. Immun. 25, 168–173. doi: 10.1016/j.bbi.2010.09.013
Harada, T., Kagamiyama, H., and Hatakeyama, K. (1993). Feedback regulation mechanisms for the control of GTP cyclohydrolase I activity. Science 260, 1507–1510. doi: 10.1126/science.8502995
Haroon, E., Miller, A. H., and Sanacora, G. (2017). Inflammation, glutamate, and glia: a trio of trouble in mood disorders. Neuropsychopharmacology 42, 193–215. doi: 10.1038/npp.2016.199
Haruki, H., Hovius, R., Pedersen, M. G., and Johnsson, K. (2016). Tetrahydrobiopterin biosynthesis as a potential target of the kynurenine pathway metabolite xanthurenic acid. J. Biol. Chem. 291, 652–657. doi: 10.1074/jbc.C115.680488
Hashimoto, R., Mizutani, M., Ohta, T., Nakazawa, K., and Nagatsu, T. (1994). Changes in plasma tetrahydrobiopterin levels of depressives in depressive and remission phases: reconfirmed by measurement with an internal standard. Neuropsychobiology 29, 57–60. doi: 10.1159/000119064
Henry, C. J., Huang, Y., Wynne, A. M., and Godbout, J. P. (2009). Peripheral lipopolysaccharide (LPS) challenge promotes microglial hyperactivity in aged mice that is associated with exaggerated induction of both pro-inflammatory IL-1beta and anti-inflammatory IL-10 cytokines. Brain Behav. Immun. 23, 309–317. doi: 10.1016/j.bbi.2008.09.002
Hirayama, M., Tsunoda, M., Yamamoto, M., Tsuda, T., and Ohno, K. (2016). Serum tyrosine-to-phenylalanine ratio is low in Parkinson’s disease. J. Parkinsons Dis. 6, 423–431. doi: 10.3233/JPD-150736
Hoge, E. A., Brandstetter, K., Moshier, S., Pollack, M. H., Wong, K. K., and Simon, N. M. (2009). Broad spectrum of cytokine abnormalities in panic disorder and posttraumatic stress disorder. Depress. Anxiety 26, 447–455. doi: 10.1002/da.20564
Homma, D., Katoh, S., Tokuoka, H., and Ichinose, H. (2013). The role of tetrahydrobiopterin and catecholamines in the developmental regulation of tyrosine hydroxylase level in the brain. J. Neurochem. 126, 70–81. doi: 10.1111/jnc.12287
Horowitz, M. A., Wertz, J., Zhu, D., Cattaneo, A., Musaelyan, K., and Nikkheslat, N. (2014). Antidepressant compounds can be both pro- and anti-inflammatory in human hippocampal cells. Int. J. Neuropsychopharmacol. 18:yu076. doi: 10.1093/ijnp/pyu076
Hughes, A., and Kumari, M. (2017). Associations of C-reactive protein and psychological distress are modified by antidepressants, supporting an inflammatory depression subtype: Findings from UKHLS. Brain Behav. Immun. 66, 89–93. doi: 10.1016/j.bbi.2017.07.009
Ichinose, H., Ohye, T., Takahashi, E., Seki, N., Hori, T., Segawa, M., et al. (1994). Hereditary progressive dystonia with marked diurnal fluctuation caused by mutations in the GTP cyclohydrolase I gene. Nat. Genet. 8, 236–242. doi: 10.1038/ng1194-236
Jantaratnotai, N., Mosikanon, K., Lee, Y., and McIntyre, R. S. (2017). The interface of depression and obesity. Obes. Res. Clin. Pract. 11, 1–10. doi: 10.1016/j.orcp.2016.07.003
Jeon, S. W., and Kim, Y. K. (2017). Inflammation-induced depression: Its pathophysiology and therapeutic implications. J. Neuroimmunol. 313, 92–98. doi: 10.1016/j.jneuroim.2017.10.016
Jha, M. K., and Trivedi, M. H. (2018). Personalized antidepressant selection and pathway to novel treatments: clinical utility of targeting inflammation. Int. J. Mol. Sci. 19, 1–15. doi: 10.3390/ijms19010233
Kamata, M., Higuchi, H., Yoshimoto, M., Yoshida, K., and Shimizu, T. (2000). Effect of single intracerebroventricular injection of alpha-interferon on monoamine concentrations in the rat brain. Eur. Neuropsychopharmacol. 10, 129–132. doi: 10.1016/S0924-977X(99)00067-X
Kappelmann, N., Lewis, G., Dantzer, R., Jones, P. B., and Khandaker, G. M. (2018). Antidepressant activity of anti-cytokine treatment: a systematic review and meta-analysis of clinical trials of chronic inflammatory conditions. Mol. Psychiatry 23, 335–343. doi: 10.1038/mp.2016.167
Kawase, K., Kondo, K., Saito, T., Shimasaki, A., Takahashi, A., Kamatani, Y., et al. (2016). Risk factors and clinical characteristics of the depressive state induced by pegylated interferon therapy in patients with hepatitis C virus infection: a prospective study. Psychiatry Clin. Neurosci. 70, 489–497. doi: 10.1111/pcn.12424
Kazumori, H., Ishihara, S., Rumi, M. A., Ortega-Cava, C. F., Kadowaki, Y., and Kinoshita, Y. (2004). Transforming growth factor-alpha directly augments histidine decarboxylase and vesicular monoamine transporter 2 production in rat enterochromaffin-like cells. Am. J. Physiol. Gastrointest. Liver Physiol. 286, G508–G514. doi: 10.1152/ajpgi.00269.2003
Kekow, J., Moots, R. J., Emery, P., Durez, P., Koenig, A., Singh, A., et al. (2010). Patient-reported outcomes improve with etanercept plus methotrexate in active early rheumatoid arthritis and the improvement is strongly associated with remission: the COMET trial. Ann. Rheum. Dis. 69, 222–225. doi: 10.1136/ard.2008.102509
Kishi, T., Ichinose, H., Yoshimura, R., Fukuo, Y., Kitajima, T., Inada, T., et al. (2012). GTP cyclohydrolase 1 gene haplotypes as predictors of SSRI response in Japanese patients with major depressive disorder. J. Affect. Disord. 142, 315–322. doi: 10.1016/j.jad.2012.05.004
Kitagami, T., Yamada, K., Miura, H., Hashimoto, R., Nabeshima, T., and Ohta, T. (2003). Mechanism of systemically injected interferon-alpha impeding monoamine biosynthesis in rats: role of nitric oxide as a signal crossing the blood-brain barrier. Brain Res. 978, 104–114. doi: 10.1016/S0006-8993(03)02776-8
Klaus, F., Paterna, J. C., Marzorati, E., Sigrist, H., Götze, L., Schwendener, S., et al. (2016). Differential effects of peripheral and brain tumor necrosis factor on inflammation, sickness, emotional behavior and memory in mice. Brain Behav. Immun. 58, 310–326. doi: 10.1016/j.bbi.2016.08.001
Kloiber, S., Ising, M., Reppermund, S., Horstmann, S., Dose, T., Majer, M., et al. (2007). Overweight and obesity affect treatment response in major depression. Biol. Psychiatry 62, 321–326. doi: 10.1016/j.biopsych.2006.10.001
Knapp, S., and Irwin, M. (1989). Plasma levels of tetrahydrobiopterin and folate in major depression. Biol. Psychiatry 26, 156–162. doi: 10.1016/0006-3223(89)90019-X
Kocki, T., Wnuk, S., Kloc, R., Kocki, J., Owe-Larsson, B., and Urbanska, E. M. (2012). New insight into the antidepressants action: modulation of kynurenine pathway by increasing the kynurenic cid/3-hydroxykynurenine ratio. J. Neural. Transm. 119, 235–243. doi: 10.1007/s00702-011-0668-8
Köhler, O., Gasse, C., Petersen, L., Ingstrup, K. G., Nierenberg, A. A., Mors, O., et al. (2016). The effect of concomitant treatment with SSRIs and statins: a population-based study. Am. J. Psychiatry 173, 807–815. doi: 10.1176/appi.ajp.2016.15040463
Koshimura, K., Murakami, Y., Tanaka, J., and Kato, Y. (2000). The role of 6R-tetrahydrobiopterin in the nervous system. Prog. Neurobiol. 61, 415–438. doi: 10.1016/S0301-0082(99)00059-3
Krishna, S., Keralapurath, M. M., Lin, Z., Wagner, J. J., de La Serre, C. B., Harn, D. A., et al. (2015). Neurochemical and electrophysiological deficits in the ventral hippocampus and selective behavioral alterations caused by high-fat diet in female C57BL/6 mice. Neuroscience 297, 170–181. doi: 10.1016/j.neuroscience.2015.03.068
Kumai, T., Tateishi, T., Tanaka, M., Watanabe, M., Shimizu, H., and Kobayashi, S. (2000). Effect of interferon-alpha on tyrosine hydroxylase and catecholamine levels in the brain of rats. Life Sci. 67, 663–669. doi: 10.1016/S0024-3205(00)00660-3
Kunesová, M., Braunerová, R., Hlavatı, P., Tvrzická, E., Stanková, B., and Skrha, J. (2006). The influence of n-3 polyunsaturated fatty acids and very low calorie diet during a short-term weight reducing regimen on weight loss and serum fatty acid composition in severely obese women. Physiol. Res. 55, 63–72.
Lanquillon, S., Krieg, J. C., Bening-Abu-Shach, U., and Vedder, H. (2000). Cytokine production and treatment response in major depressive disorder. Neuropsychopharmacology 22, 370–379. doi: 10.1016/S0893-133X(99)00134-7
Lasselin, J., Magne, E., Beau, C., Ledaguenel, P., Dexpert, S., Aubert, A., et al. (2014). Adipose inflammation in obesity: relationship with circulating levels of inflammatory markers and association with surgery-induced weight loss. J. Clin. Endocrinol. Metab. 99, E53–E61. doi: 10.1210/jc.2013-2673
Laumet, G., Zhou, W., Dantzer, R., Edralin, J. D., Huo, X., Budac, D. P., et al. (2017). Upregulation of neuronal kynurenine 3-monooxygenase mediates depression-like behavior in a mouse model of neuropathic pain. Brain Behav. Immun. 66, 94–102. doi: 10.1016/j.bbi.2017.07.008
Lawson, M. A., Parrott, J. M., McCusker, R. H., Dantzer, R., Kelley, K. W., and O’Connor, J. C. (2013). Intracerebroventricular administration of lipopolysaccharide induces indoleamine-2,3-dioxygenase-dependent depression-like behaviors. J. Neuroinflammation 10:87. doi: 10.1186/1742-2094-10-87
Layé, S., Nadjar, A., Joffre, C., and Bazinet, R. P. (2018). Anti-inflammatory effects of Omega-3 fatty acids in the brain: physiological mechanisms and relevance to pharmacology. Pharmacol. Rev. 70, 12–38. doi: 10.1124/pr.117.014092
Leighton, S. P., Nerurkar, L., Krishnadas, R., Johnman, C., Graham, G. J., and Cavanagh, J. (2018). Chemokines in depression in health and in inflammatory illness: a systematic review and meta-analysis. Mol. Psychiatry 23, 48–58. doi: 10.1038/mp.2017.205
Lestage, J., Verrier, D., Palin, K., and Dantzer, R. (2002). The enzyme indoleamine 2,3-dioxygenase is induced in the mouse brain in response to peripheral administration of lipopolysaccharide and superantigen. Brain Behav. Immun. 16, 596–601. doi: 10.1016/S0889-1591(02)00014-4
Li, N., Liu, R. J., Dwyer, J. M., Banasr, M., Lee, B., Son, H., et al. (2011). Glutamate N-methyl-D-aspartate receptor antagonists rapidly reverse behavioral and synaptic deficits caused by chronic stress exposure. Biol. Psychiatry 69, 754–761. doi: 10.1016/j.biopsych.2010.12.015
Llorens-Martin, M., Jurado-Arjona, J., Fuster-Matanzo, A., Hernandez, F., Rabano, A., and Avila, J. (2014). Peripherally triggered and GSK-3beta-driven brain inflammation differentially skew adult hippocampal neurogenesis, behavioral pattern separation and microglial activation in response to ibuprofen. Transl. Psychiatry 4:e463. doi: 10.1038/tp.2014.92
Lovelace, M. D., Varney, B., Sundaram, G., Lennon, M. J., Lim, C. K., Jacobs, K., et al. (2017). Recent evidence for an expanded role of the kynurenine pathway of tryptophan metabolism in neurological diseases. Neuropsychopharmacology 112, 373–388. doi: 10.1016/j.neuropharm.2016.03.024
Luppino, F. S., de Wit, L. M., Bouvy, P. F., Stijnen, T., Cuijpers, P., Penninx, B. W., et al. (2010). Overweight, obesity, and depression: a systematic review and meta-analysis of longitudinal studies. Arch. Gen. Psychiatry 67, 220–229. doi: 10.1001/archgenpsychiatry.2010.2
Mangge, H., Summers, K. L., Meinitzer, A., Zelzer, S., Almer, G., Prassl, R., et al. (2014). Obesity-related dysregulation of the tryptophan-kynurenine metabolism: role of age and parameters of the metabolic syndrome. Obesity 22, 195–201. doi: 10.1002/oby.20491
Martín-Hernández, D., Tendilla-Beltrán, H., Madrigal, J. L. M., García-Bueno, B., Leza, J. C., and Caso, J. R. (2018). Chronic mild stress alters kynurenine pathways changing the glutamate neurotransmission in frontal cortex of rats. Mol. Neurobiol. doi: 10.1007/s12035-018-1096-7 [Epub ahead of print].
Mazarei, G., Budac, D. P., Lu, G., Lee, H., Moller, T., and Leavitt, B. R. (2013). The absence of indoleamine 2,3-dioxygenase expression protects against NMDA receptor-mediated excitotoxicity in mouse brain. Exp. Neurol. 249, 144–148. doi: 10.1016/j.expneurol.2013.08.007
Merali, Z., Brennan, K., Brau, P., and Anisman, H. (2003). Dissociating anorexia and anhedonia elicited by interleukin-1beta: antidepressant and gender effects on responding for “free chow” and “earned” sucrose intake. Psychopharmacology 165, 413–418. doi: 10.1007/s00213-002-1273-1
Moreau, M., André, C., O’Connor, J. C., Dumich, S. A., Woods, J. A., Kelley, K. W., et al. (2008). Inoculation of Bacillus Calmette-Guerin to mice induces an acute episode of sickness behavior followed by chronic depressive-like behavior. Brain Behav. Immun. 22, 1087–1095. doi: 10.1016/j.bbi.2008.04.001
Moreau, M., Lestage, J., Verrier, D., Mormède, C., Kelley, K. W., Dantzer, R., et al. (2005). Bacille Calmette-Guerin inoculation induces chronic activation of peripheral and brain indoleamine 2,3-dioxygenase in mice. J. Infect. Dis. 192, 537–544. doi: 10.1086/431603
Müller, N., Schwarz, M. J., Dehning, S., Douhe, A., Cerovecki, A., Goldstein-Muller, B., et al. (2006). The cyclooxygenase-2 inhibitor celecoxib has therapeutic effects in major depression: results of a double-blind, randomized, placebo controlled, add-on pilot study to reboxetine. Mol. Psychiatry 11, 680–684. doi: 10.1038/sj.mp.4001805
Müller, U., Steinberger, D., and Topka, H. (2002). Mutations of GCH1 in Dopa-responsive dystonia. J. Neural. Transm. 109, 321–328. doi: 10.1007/s007020200026
Murr, C., Grammer, T. B., Meinitzer, A., Kleber, M. E., März, W., and Fuchs, D. (2014). Immune activation and inflammation in patients with cardiovascular disease are associated with higher phenylalanine to tyrosine ratios: the ludwigshafen risk and cardiovascular health study. J. Amino Acids 2014:783730. doi: 10.1155/2014/783730
Murr, C., Widner, B., Wirleitner, B., and Fuchs, D. (2002). Neopterin as a marker for immune system activation. Curr. Drug Metab. 3, 175–187. doi: 10.2174/1389200024605082
Musselman, D. L., Lawson, D. H., Gumnick, J. F., Manatunga, A. K., Penna, S., Goodkin, R. S., et al. (2001). Paroxetine for the prevention of depression induced by high-dose interferon alfa. N. Engl. J. Med. 344, 961–966. doi: 10.1056/NEJM200103293441303
Nagatsu, I., Ichinose, H., Sakai, M., Titani, K., Suzuki, M., and Nagatsu, T. (1995). Immunocytochemical localization of GTP cyclohydrolase I in the brain, adrenal gland, and liver of mice. J. Neural Transm. Gen. Sect. 102, 175–188. doi: 10.1007/BF01281153
Nasser, A., Møller, L. B., Olesen, J. H., Konradsen Refsgaard, L., and Andreasen, J. T. (2014). Anxiety- and depression-like phenotype of hph-1 mice deficient in tetrahydrobiopterin. Neurosci. Res. 89, 44–53. doi: 10.1016/j.neures.2014.08.015
Nazimek, K., Kozlowski, M., Bryniarski, P., Strobel, S., Bryk, A., Myszka, M., et al. (2016). Repeatedly administered antidepressant drugs modulate humoral and cellular immune response in mice through action on macrophages. Exp. Biol. Med. 241, 1540–1550. doi: 10.1177/1535370216643769
Neurauter, G., Schröcksnadel, K., Scholl-Bürgi, S., Sperner-Unterweger, B., Schubert, C., Ledochowski, M., et al. (2008). Chronic immune stimulation correlates with reduced phenylalanine turnover. Curr. Drug Metab. 9, 622–627. doi: 10.2174/138920008785821738
Norden, D. M., McCarthy, D. O., Bicer, S., Devine, R. D., Reiser, P. J., Godbout, J. P., et al. (2015). Ibuprofen ameliorates fatigue- and depressive-like behavior in tumor-bearing mice. Life Sci. 143, 65–70. doi: 10.1016/j.lfs.2015.10.020
O’Connor, J. C., André, C., Wang, Y., Lawson, M. A., Szegedi, S. S., Lestage, J., et al. (2009a). Interferon-gamma and tumor necrosis factor-alpha mediate the upregulation of indoleamine 2,3-dioxygenase and the induction of depressive-like behavior in mice in response to bacillus Calmette-Guerin. J. Neurosci. 29, 4200–4209. doi: 10.1523/JNEUROSCI.5032-08.2009
O’Connor, J. C., Lawson, M. A., André, C., Briley, E. M., Szegedi, S. S., Lestage, J., et al. (2009b). Induction of IDO by bacille Calmette-Guerin is responsible for development of murine depressive-like behavior. J. Immunol. 182, 3202–3212. doi: 10.4049/jimmunol.0802722
O’Connor, J. C., Lawson, M. A., Andre, C., Moreau, M., Lestage, J., Castanon, N., et al. (2009c). Lipopolysaccharide-induced depressive-like behavior is mediated by indoleamine 2,3-dioxygenase activation in mice. Mol. Psychiatry 14, 511–522. doi: 10.1038/sj.mp.4002148
O’Farrell, K., and Harkin, A. (2017). Stress-related regulation of the kynurenine pathway: relevance to neuropsychiatric and degenerative disorders. Neuropharmacology 112, 307–323. doi: 10.1016/j.neuropharm.2015.12.004
Ohashi, A., Saeki, Y., Harada, T., Naito, M., Takahashi, T., Aizawa, S., et al. (2016). Tetrahydrobiopterin supplementation: elevation of tissue biopterin levels accompanied by a relative increase in dihydrobiopterin in the blood and the role of probenecid-sensitive uptake in scavenging dihydrobiopterin in the liver and kidney of rats. PLoS One 11:e0164305. doi: 10.1371/journal.pone.0164305
Ormstad, H., Verkerk, R., and Sandvik, L. (2016). Serum phenylalanine, tyrosine, and their ratio in acute ischemic stroke: on the trail of a biomarker? J. Mol. Neurosci. 58, 102–108. doi: 10.1007/s12031-015-0659-6
Oxenkrug, G., Tucker, K. L., Requintina, P., and Summergrad, P. (2011). Neopterin, a marker of interferon-gamma-inducible inflammation, correlates with pyridoxal-5’-phosphate, waist circumference, HDL-cholesterol, insulin resistance and mortality risk in adult Boston community dwellers of puerto Rican origin. Am. J. Neuroprot. Neuroregen. 3, 48–52. doi: 10.1166/ajnn.2011.1024
Oxenkrug, G. F. (2011). Interferon-gamma-inducible kynurenines/pteridines inflammation cascade: implications for aging and aging-associated psychiatric and medical disorders. J. Neural Transm. 118, 75–85. doi: 10.1007/s00702-010-0475-7
Pan, L., McKain, B. W., Madan-Khetarpal, S., Mcguire, M., Diler, R. S., Perel, J. M., et al. (2011). GTP-cyclohydrolase deficiency responsive to sapropterin and 5-HTP supplementation: relief of treatment-refractory depression and suicidal behaviour. BMJ Case Rep. 2011:bcr0320113927. doi: 10.1136/bcr.03.2011.3927
Park, S. E., Dantzer, R., Kelley, K. W., and McCusker, R. H. (2011). Central administration of insulin-like growth factor-I decreases depressive-like behavior and brain cytokine expression in mice. J. Neuroinflammation 8:12. doi: 10.1186/1742-2094-8-12
Parrott, J. M., Redus, L., and O’Connor, J. C. (2016a). Kynurenine metabolic balance is disrupted in the hippocampus following peripheral lipopolysaccharide challenge. J. Neuroinflammation 13:124. doi: 10.1186/s12974-016-0590-y
Parrott, J. M., Redus, L., Santana-Coelho, D., Morales, J., Gao, X., and O’Connor, J. C. (2016b). Neurotoxic kynurenine metabolism is increased in the dorsal hippocampus and drives distinct depressive behaviors during inflammation. Transl. Psychiatry 6:e918. doi: 10.1038/tp.2016.200
Penninx, B. W., Milaneschi, Y., Lamers, F., and Vogelzangs, N. (2013). Understanding the somatic consequences of depression: biological mechanisms and the role of depression symptom profile. BMC Med. 11:129. doi: 10.1186/1741-7015-11-129
Ploder, M., Neurauter, G., Spittler, A., Schroecksnadel, K., Roth, E., and Fuchs, D. (2008). Serum phenylalanine in patients post trauma and with sepsis correlate to neopterin concentrations. Amino Acids 35, 303–307. doi: 10.1007/s00726-007-0625-x
Potvin, S., Stip, E., Sepehry, A. A., Gendron, A., Bah, R., and Kouassi, E. (2008). Inflammatory cytokine alterations in schizophrenia: a systematic quantitative review. Biol. Psychiatry 63, 801–808. doi: 10.1016/j.biopsych.2007.09.024
Prendergast, G. C., Malachowski, W. P., DuHadaway, J. B., and Muller, A. J. (2018). Discovery of IDO1 inhibitors: from bench to bedside. Cancer Res. 77, 6795–6811. doi: 10.1158/0008-5472.CAN-17-2285
Raison, C. L., Capuron, L., and Miller, A. H. (2006). Cytokines sing the blues: inflammation and the pathogenesis of depression. Trends Immunol. 27, 24–31. doi: 10.1016/j.it.2005.11.006
Raison, C. L., Dantzer, R., Kelley, K. W., Lawson, M. A., Woolwine, B. J., Vogt, G., et al. (2010). CSF concentrations of brain tryptophan and kynurenines during immune stimulation with IFN-alpha: relationship to CNS immune responses and depression. Mol. Psychiatry 15, 393–403. doi: 10.1038/mp.2009.116
Raison, C. L., Rutherford, R. E., Woolwine, B. J., Shuo, C., Schettler, P., Drake, D. F., et al. (2013). A randomized controlled trial of the tumor necrosis factor antagonist infliximab for treatment-resistant depression: the role of baseline inflammatory biomarkers. JAMA Psychiatry 70, 31–41. doi: 10.1001/2013.jamapsychiatry.4
Rapaport, M. H., Nierenberg, A. A., Schettler, P. J., Kinkead, B., Cardoos, A., Walker, R., et al. (2016). Inflammation as a predictive biomarker for response to omega-3 fatty acids in major depressive disorder: a proof-of-concept study. Mol. Psychiatry 21, 71–79. doi: 10.1038/mp.2015.22
Remus, J. L., and Dantzer, R. (2016). Inflammation models of depression in rodents: relevance to psychotropic drug discovery. Int. J. Neuropsychopharmacol. 19, 1–13. doi: 10.1093/ijnp/pyw028
Reus, G. Z., Jansen, K., Titus, S., Carvalho, A. F., Gabbay, V., and Quevedo, J. (2015). Kynurenine pathway dysfunction in the pathophysiology and treatment of depression: evidences from animal and human studies. J. Psychiatr. Res. 68, 316–328. doi: 10.1016/j.jpsychires.2015.05.007
Rosenblat, J. D., and McIntyre, R. S. (2018). Efficacy and tolerability of minocycline for depression: a systematic review and meta-analysis of clinical trials. J. Affect. Disord. 227, 219–225. doi: 10.1016/j.jad.2017.10.042
Rush, A. J., Trivedi, M. H., Wisniewski, S. R., Nierenberg, A. A., Stewart, J. W., Warden, D., et al. (2006). Acute and longer-term outcomes in depressed outpatients requiring one or several treatment steps: a STAR∗D report. Am. J. Psychiatry 163, 1905–1917. doi: 10.1176/ajp.2006.163.11.1905
Russi, P., Alesiani, M., Lombardi, G., Davolio, P., Pellicciari, R., and Moroni, F. (1992). Nicotinylalanine increases the formation of kynurenic acid in the brain and antagonizes convulsions. J. Neurochem. 59, 2076–2080. doi: 10.1111/j.1471-4159.1992.tb10097.x
Salazar, A., Gonzalez-Rivera, B. L., Redus, L., Parrott, J. M., and O’Connor, J. C. (2012). Indoleamine 2,3-dioxygenase mediates anhedonia and anxiety-like behaviors caused by peripheral lipopolysaccharide immune challenge. Horm. Behav. 62, 202–209. doi: 10.1016/j.yhbeh.2012.03.010
Sato, T., Suzuki, E., Yokoyama, M., Semba, J., Watanabe, S., and Miyaoka, H. (2006). Chronic intraperitoneal injection of interferon-alpha reduces serotonin levels in various regions of rat brain, but does not change levels of serotonin transporter mRNA, nitrite or nitrate. Psychiatry Clin. Neurosci. 60, 499–506. doi: 10.1111/j.1440-1819.2006.01538.x
Savitz, J., Drevets, W. C., Smith, C. M., Victor, T. A., Wurfel, B. E., Bellgowan, P. S., et al. (2015a). Putative neuroprotective and neurotoxic kynurenine pathway metabolites are associated with hippocampal and amygdalar volumes in subjects with major depressive disorder. Neuropsychopharmacology 40, 463–471. doi: 10.1038/npp.2014.194
Savitz, J., Drevets, W. C., Wurfel, B. E., Ford, B. N., Bellgowan, P. S., Victor, T. A., et al. (2015b). Reduction of kynurenic acid to quinolinic acid ratio in both the depressed and remitted phases of major depressive disorder. Brain Behav. Immun. 46, 55–59. doi: 10.1016/j.bbi.2015.02.007
Sawada, M., Horikoshi, T., Masada, M., Akino, M., Sugimoto, T., Matsuura, S., et al. (1986). A sensitive assay of GTP cyclohydrolase I activity in rat and human tissues using radioimmunoassay of neopterin. Anal. Biochem. 154, 361–366. doi: 10.1016/0003-2697(86)90537-3
Schedlowski, M., Engler, H., and Grigoleit, J. S. (2014). Endotoxin-induced experimental systemic inflammation in humans: a model to disentangle immune-to-brain communication. Brain Behav. Immun. 35, 1–8. doi: 10.1016/j.bbi.2013.09.015
Schmidt, F. M., Kirkby, K. C., and Lichtblau, N. (2016). Inflammation and immune regulation as potential drug targets in antidepressant treatment. Curr. Neuropharmacol. 14, 674–687. doi: 10.2174/1570159X14666160115130414
Schwarcz, R., and Stone, T. W. (2017). The kynurenine pathway and the brain: challenges, controversies and promises. Neuropharmacology 112, 237–247. doi: 10.1016/j.neuropharm.2016.08.003
Shariq, A. S., Brietzke, E., Rosenblat, J. D., Barendra, V., Pan, Z., and McIntyre, R. S. (2018). Targeting cytokines in reduction of depressive symptoms: a comprehensive review. Prog. Neuropsychopharmacol. Biol. Psychiatry 83, 86–91. doi: 10.1016/j.pnpbp.2018.01.003
Sharma, S., and Fulton, S. (2013). Diet-induced obesity promotes depressive-like behaviour that is associated with neural adaptations in brain reward circuitry. Int. J. Obes. 37, 382–389. doi: 10.1038/ijo.2012.48
Shi, W., Meininger, C. J., Haynes, T. E., Hatakeyama, K., and Wu, G. (2004). Regulation of tetrahydrobiopterin synthesis and bioavailability in endothelial cells. Cell. Biochem. Biophys. 41, 415–434. doi: 10.1385/CBB:41:3:415
Silva-Adaya, D., Pérez-De, La Cruz, V., Villeda-Hernández, J., Carrillo-Mora, P., González-Herrera, I. G., et al. (2011). Protective effect of L-kynurenine and probenecid on 6-hydroxydopamine-induced striatal toxicity in rats: implications of modulating kynurenate as a protective strategy. Neurotoxicol. Teratol. 33, 303–312. doi: 10.1016/j.ntt.2010.10.002
Smith, K. J., Au, B., Ollis, L., and Schmitz, N. (2018). The association between C-reactive protein, Interleukin-6 and depression among older adults in the community: a systematic review and meta-analysis. Exp. Gerontol. 102, 109–132. doi: 10.1016/j.exger.2017.12.005
Steiner, J., Walter, M., Gos, T., Guillemin, G. J., Bernstein, H. G., Sarnyai, Z., et al. (2011). Severe depression is associated with increased microglial quinolinic acid in subregions of the anterior cingulate gyrus: evidence for an immune-modulated glutamatergic neurotransmission? J. Neuroinflammation 8:94. doi: 10.1186/1742-2094-8-94
Strawbridge, R., Arnone, D., Danese, A., Papadopoulos, A., Herane Vives, A., and Cleare, A. J. (2015). Inflammation and clinical response to treatment in depression: a meta-analysis. Eur. Neuropsychopharmacol. 25, 1532–1543. doi: 10.1016/j.euroneuro.2015.06.007
Sumi-Ichinose, C., Urano, F., Kuroda, R., Ohye, T., Kojima, M., Tazawa, M., et al. (2001). Catecholamines and serotonin are differently regulated by tetrahydrobiopterin. A study from 6-pyruvoyltetrahydropterin synthase knockout mice. J. Biol. Chem. 276, 41150–41160. doi: 10.1074/jbc.M102237200
Tashiro, T., Murakami, Y., Mouri, A., Imamura, Y., Nabeshima, T., Yamamoto, Y., et al. (2017). Kynurenine 3-monooxygenase is implicated in antidepressants-responsive depressive-like behaviors and monoaminergic dysfunctions. Behav. Brain Res. 317, 279–285. doi: 10.1016/j.bbr.2016.09.050
Thöny, B., Auerbach, G., and Blau, N. (2000). Tetrahydrobiopterin biosynthesis, regeneration and functions. Biochem. J. 347(Pt 1), 1–16.
Tien, L. T., Kaizaki, A., Pang, Y., Cai, Z., Bhatt, A. J., and Fan, L. W. (2013). Neonatal exposure to lipopolysaccharide enhances accumulation of (-synuclein aggregation and dopamine transporter protein expression in the substantia nigra in responses to rotenone challenge in later life. Toxicology 308, 96–103. doi: 10.1016/j.tox.2013.03.014
Toledo-Sherman, L. M., Prime, M. E., Mrzljak, L., Beconi, M. G., Beresford, A., Brookfield, F. A., et al. (2015). Development of a series of aryl pyrimidine kynurenine monooxygenase inhibitors as potential therapeutic agents for the treatment of Huntington’s disease. J. Med. Chem. 58, 115911–115983. doi: 10.1021/jm501350y
Toups, M. S., Myers, A. K., Wisniewski, S. R., Kurian, B., Morris, D. W., Rush, A. J., et al. (2013). Relationship between obesity and depression: characteristics and treatment outcomes with antidepressant medication. Psychosom. Med. 75, 863–872. doi: 10.1097/PSY.0000000000000000
Traki, L., Rostom, S., Tahiri, L., Bahiri, R., Harzy, T., Abouqal, R., et al. (2014). Responsiveness of the EuroQol EQ-5D and hospital anxiety and depression scale (HADS) in rheumatoid arthritis patients receiving tocilizumab. Clin. Rheumatol. 33, 1055–1060. doi: 10.1007/s10067-014-2609-z
Trender-Gerhard, I., Sweeney, M. G., Schwingenschuh, P., Mir, P., Edwards, M. J., Gerhard, A., et al. (2009). Autosomal-dominant GTPCH1-deficient DRD: clinical characteristics and long-term outcome of 34 patients. J. Neurol. Neurosurg. Psychiatry 80, 839–845. doi: 10.1136/jnnp.2008.155861
Tynan, R. J., Weidenhofer, J., Hinwood, M., Cairns, M. J., Day, T. A., and Walker, F. R. (2012). A comparative examination of the anti-inflammatory effects of SSRI and SNRI antidepressants on LPS stimulated microglia. Brain Behav. Immun. 26, 469–479. doi: 10.1016/j.bbi.2011.12.011
Tyring, S., Gottlieb, A., Papp, K., Gordon, K., Leonardi, C., Wang, A., et al. (2006). Etanercept and clinical outcomes, fatigue, and depression in psoriasis: double-blind placebo-controlled randomised phase III trial. Lancet 367, 29–35. doi: 10.1016/S0140-6736(05)67763-X
Valkanova, V., Ebmeier, K. P., and Allan, C. L. (2013). CRP, IL-6 and depression: a systematic review and meta-analysis of longitudinal studies. J. Affect. Disord. 150, 736–744. doi: 10.1016/j.jad.2013.06.004
Van Hove, J. L., Steyaert, J., Matthijs, G., Legius, E., Theys, P., Wevers, R., et al. (2006). Expanded motor and psychiatric phenotype in autosomal dominant Segawa syndrome due to GTP cyclohydrolase deficiency. J. Neurol. Neurosurg. Psychiatry 77, 18–23. doi: 10.1136/jnnp.2004.051664
Vécsei, L., Szalárdy, L., Fülöp, F., and Toldi, J. (2013). Kynurenines in the CNS: recent advances and new questions. Nat. Rev. Drug Discov. 12, 64–82. doi: 10.1038/nrd3793
Vichaya, E. G., Laumet, G., Christian, D. L., Grossberg, A. J., Estrada, D. J., Heijnen, C. B., et al. (2018). Motivational changes that develop in a mouse model of inflammation-induced depression are independent of indoleamine 2,3 dioxygenase. Neuropsychopharmacology doi: 10.1038/s41386-018-0075-z [Epub ahead of print].
Vogelzangs, N., Duivis, H. E., Beekman, A. T., Kluft, C., Neuteboom, J., Hoogendijk, W., et al. (2012). Association of depressive disorders, depression characteristics and antidepressant medication with inflammation. Transl. Psychiatry 21:e79. doi: 10.1038/tp.2012.8
Walker, A. K., Budac, D. P., Bisulco, S., Lee, A. W., Smith, R. A., Beenders, B., et al. (2013). NMDA receptor blockade by ketamine abrogates lipopolysaccharide-induced depressive-like behavior in C57BL/6J mice. Neuropsychopharmacology 38, 1609–1616. doi: 10.1038/npp.2013.71
Werner, E. R., Gorren, A. C., Heller, R., Werner-Felmayer, G., and Mayer, B. (2003). Tetrahydrobiopterin and nitric oxide: mechanistic and pharmacological aspects. Exp. Biol. Med. 228, 1291–1302. doi: 10.1177/153537020322801108
Wiêdłocha, M., Marcinowicz, P., Krupa, R., Janoska-Jaździk, M., Janus, M., and Dêbowska, W. (2018). Effect of antidepressant treatment on peripheral inflammation markers - A meta-analysis. Prog. Neuropsychopharmacol. Biol. Psychiatry 80, 217–226. doi: 10.1016/j.pnpbp.2017.04.026
Woggon, B., Angst, J., Curtius, H. C., and Niederwieser, A. (1984). Unsuccessful treatment of depression with tetrahydrobiopterin. Lancet 2:1463. doi: 10.1016/S0140-6736(84)91648-9
Woo, Y. S., Seo, H. J., McIntyre, R. S., and Bahk, W. M. (2016). Obesity and its potential effects on antidepressant treatment outcomes in patients with depressive disorders: a literature review. Int. J. Mol. Sci. 17, 1–20. doi: 10.3390/ijms17010080
World Health Organization (2017). Depression and Other Common Mental Disorders: Global Health Estimates. Geneva: World Health Organization.
Wu, H. Q., Rassoulpour, A., and Schwarcz, R. (2007). Kynurenic acid leads, dopamine follows: a new case of volume transmission in the brain? J. Neural Transm. 114, 33–41. doi: 10.1007/s00702-006-0562-y
Wynne, A. M., Henry, C. J., Huang, Y., Cleland, A., and Godbout, J. P. (2010). Protracted downregulation of CX3CR1 on microglia of aged mice after lipopolysaccharide challenge. Brain Behav. Immun. 24, 1190–1201. doi: 10.1016/j.bbi.2010.05.011
Xie, W., Cai, L., Yu, Y., Gao, L., Xiao, L., He, Q., et al. (2014). Activation of brain indoleamine 2,3-dioxygenase contributes to epilepsy-associated depressive-like behavior in rats with chronic temporal lobe epilepsy. J. Neuroinflammation 11:41. doi: 10.1186/1742-2094-11-41
Zaminelli, T., Gradowski, R. W., Bassani, T. B., Barbiero, J. K., Santiago, R. M., Maria-Ferreira, D., et al. (2014). Antidepressant and antioxidative effect of Ibuprofen in the rotenone model of Parkinson’s disease. Neurotox. Res. 26, 351–362. doi: 10.1007/s12640-014-9467-y
Zangerle, R., Kurz, K., Neurauter, G., Kitchen, M., Sarcletti, M., and Fuchs, D. (2010). Increased blood phenylalanine to tyrosine ratio in HIV-1 infection and correction following effective antiretroviral therapy. Brain Behav. Immun. 24, 403–408. doi: 10.1016/j.bbi.2009.11.004
Zhao, X., Cao, F., Liu, Q., Li, X., Xu, G., Liu, G., et al. (2017). Behavioral, inflammatory and neurochemical disturbances in LPS and UCMS-induced mouse models of depression. Behav. Brain Res. doi: 10.1016/j.bbr.2017.05.064 [Epub ahead of print].
Zhu, C. B., Blakely, R. D., and Hewlett, W. A. (2006). The proinflammatory cytokines interleukin-1beta and tumor necrosis factor-alpha activate serotonin transporters. Neuropsychopharmacology 31, 2121–2131. doi: 10.1038/sj.npp.1301029
Zoga, M., Oulis, P., Chatzipanagiotou, S., Masdrakis, V. G., Pliatsika, P., Boufidou, F., et al. (2014). Indoleamine 2,3-dioxygenase and immune changes under antidepressive treatment in major depression in females. In Vivo 28, 633–638.
Keywords: inflammation, neuroinflammation, kynurenines, tetrahydrobiopterin (BH4), monoamines, depressive symptoms, antidepressant treatment, anti-inflammatory strategies
Citation: Vancassel S, Capuron L and Castanon N (2018) Brain Kynurenine and BH4 Pathways: Relevance to the Pathophysiology and Treatment of Inflammation-Driven Depressive Symptoms. Front. Neurosci. 12:499. doi: 10.3389/fnins.2018.00499
Received: 05 April 2018; Accepted: 03 July 2018;
Published: 24 July 2018.
Edited by:
Abed N. Azab, Ben-Gurion University of the Negev, IsraelReviewed by:
Anthony John Hannan, The Florey Institute of Neuroscience and Mental Health, AustraliaLuc Ver Donck, Janssen Research & Development, Belgium
Copyright © 2018 Vancassel, Capuron and Castanon. This is an open-access article distributed under the terms of the Creative Commons Attribution License (CC BY). The use, distribution or reproduction in other forums is permitted, provided the original author(s) and the copyright owner(s) are credited and that the original publication in this journal is cited, in accordance with accepted academic practice. No use, distribution or reproduction is permitted which does not comply with these terms.
*Correspondence: Nathalie Castanon, nathalie.castanon@inra.fr; nathalie.castanon@bordeaux.inra.fr