- 1Faculty of Biology and Medicine, Institute of Sport Sciences, University of Lausanne, Lausanne, Switzerland
- 2National Centre of Nordic-Ski, Research and Performance, Premanon, France
- 3Applied Signal Processing Group, Ecole Polytechnique Fédérale de Lausanne, Lausanne, Switzerland
Heart rate variability (HRV) is non-invasive and commonly used for monitoring responses to training loads, fitness, or overreaching in athletes. Yet, the recording duration for a series of RR-intervals varies from 1 to 15 min in the literature. The aim of the present work was to assess the minimum record duration to obtain reliable HRV results. RR-intervals from 159 orthostatic tests (7 min supine, SU, followed by 6 min standing, ST) were analyzed. Reference windows were 4 min in SU (min 3–7) and 4 min in ST (min 9–13). Those windows were subsequently divided and the analyses were repeated on eight different fractioned windows: the first min (0–1), the second min (1–2), the third min (2–3), the fourth min (3–4), the first 2 min (0–2), the last 2 min (2–4), the first 3 min (0–3), and the last 3 min (1–4). Correlation and Bland & Altman statistical analyses were systematically performed. The analysis window could be shortened to 0–2 instead of 0–4 for RMSSD only, whereas the 4-min window was necessary for LF and total power. Since there is a need for 1 min of baseline to obtain a steady signal prior the analysis window, we conclude that studies relying on RMSSD may shorten the windows to 3 min (= 1+2) in SU or seated position only and to 6 min (= 1+2 min SU plus 1+2 min ST) if there is an orthostatic test. Studies relying on time- and frequency-domain parameters need a minimum of 5 min (= 1+4) min SU or seated position only but require 10 min (= 1+4 min SU plus 1+4 min ST) for the orthostatic test.
Introduction
Monitoring fatigue is essential for training optimization, notably for the identification of non-functional overreaching and overtraining (Meeusen et al., 2013). One of the non-invasive most commonly used tool is heart rate variability (HRV) because its changes largely depend on cardiac autonomic control, which is affected by physical training and fatigued state, for review see Buchheit (2014).
Numerous HRV test procedures have been developed to supervise athletes' follow-up: at rest either during sleep (Pichot et al., 2000; Garet et al., 2004) or awake (Schmitt et al., 2006; Plews et al., 2013), in supine and standing positions (Schmitt et al., 2013) or seated (Plews et al., 2017); with recording times varying from 1 to 15 min; during physical exercise (Sandercock and Brodie, 2006), or during the post-exercise recovery phase (Buchheit et al., 2007; Seiler et al., 2007; Hug et al., 2014). Moreover, some authors (Plews et al., 2012) rely on one HRV parameter only from the time domain: the root mean square of the successive differences (RMSSD), while others (Schmitt et al., 2016) prefer looking at multiple parameters from the time and frequency domains, which primarily are mean heart rate (mean HR), RMSSD, power in the low frequency band (LF), the high frequency band (HF), and the total power (LF+HF).
The combination of the time and frequency domains has allowed the identification of four different types of fatigue in the elite athletes, which is valuable in their training periodization (Schmitt et al., 2015b). However, the athletes have to perform a 13-min orthostatic test (7 min of recording supine followed by 6 min standing) on a very regular basis which might compromise their recovery (Schmitt et al., 2015a) or simply install a boring routine all around the year. To avoid problems of athlete compliance to complete HRV recordings, Plews et al. recommended either 5-min records (Plews et al., 2012) or recently a 1-min recording with devices as simple as photoplethysmographic (PPG) data captured at the finger level using a smartphone (Plews et al., 2017). Indeed, for RMSSD only, a 1-min window has been proposed as an alternative to the traditional 5-min window during rest or in post-exercise recovery conditions (Esco and Flatt, 2014; Pereira et al., 2016). However, none of the previous studies reported the impact of selecting a recording window as short as 1 min, on the reliability of LF, HF, and total power measurements.
With such variety in procedures, it remains particularly unclear how long a HRV recording should be in order to offer the best compromise between the quality and accuracy of the recording and the comfort for the athlete. The longer the recording time the more reliable the HRV parameters, the shorter the recording the more convenient for the athletes, especially when the recordings need to be performed on a regular basis (Plews et al., 2013). The aim of the present work is to assess the minimal acceptable recording duration at rest in the supine and the standing positions for mean HR, RMSSD, LF, HF, and total power; that is the duration below which there is a significant bias in the aforementioned HRV parameters, compared to the traditional window duration of several minutes.
Materials and Methods
The RR-interval recordings of 159 orthostatic tests from three elite athletes (53 tests each) have been gathered (Schmitt et al., 2015b). Subjects 1 and 3 are males and subjects 2 is female. Each of the three athletes has won between one and four Olympic medals, either in swimming or biathlon, plus several other titles in international and national championships. The tests selected for the work represent a follow-up between four (subject 1) and 11 (subject 3) years for each athlete. The detailed procedure of the orthostatic test can be found in details elsewhere (Schmitt et al., 2013). Briefly, the orthostatic test relied on a 13-min RR-interval recording at rest with 7 min supine (SU) followed by 6 min standing (ST). The procedures were approved by the Necker Hospital Ethic Committee (Paris, France). All the subjects provided written, voluntary, informed consent. The data analyses are based on the RR-intervals between the 3rd and 7th min SU, and between the 9th and 13th min ST. Inside those two 4-min windows, the HRV analyses were repeated on the entire 4 min (0–4) as the reference analyses and then on eight different fractioned windows: the first min (0–1), the second min (1–2) the third min (2–3), the fourth min (3–4), the first 2 min (0–2), the last 2 min (2–4), the first 3 min (0–3), and the last 3 min (1–4). Analyses were performed separately for SU and ST. Measurement of the interval duration between two R-waves of the cardiac electrical activity was performed with a HR monitor (T6, Suunto®, Vantaa, Finland).
Heartbeats that are not originated from the sino-atrial node have been shown to have drastic effects on the outcome of HRV indexes (1996). To this end, the RR-intervals from the orthostatic tests were first analyzed to remove ectopic beats from the recordings using automatic and visual inspections of the RR series. Ectopic beats were then compensated by means of interpolation to calculate normal to normal (NN) intervals. From the NN-intervals, HRV parameters were extracted namely: mean HR, RMSSD, LF (0.04–0.15 Hz) HF, (0.15–0.40 Hz), and total power (LF + HF) in ms2 (Schmitt et al., 2015b). The spectral power was estimated using the Fast Fourier Transform on the resampled NN-intervals (4 Hz; Vesin et al., 2016). All procedures were carried out in agreement with the Task Force recommendations (1996).
The statistical analyses include correlation and Bland & Altman (B&A) plots between the reference window (0–4) and each of the tested windows both in SU or ST. Statistical significance was set at an alpha level of 0.05. The Kolmogorov-Smirnov test was used to assess normality of the data. All the parameters presented in this work were normally distributed. All computations were performed separately for SU and ST positions using MATLAB® (MathWorks, Natick, MA, USA).
Results
Table 1 contains the detailed statistical data for the systematic comparison for all the tested windows against the reference window, for the correlations and the B&A. Figure 1 shows the correlation and the B&A plots for LF in SU and ST, comparing the 1–4 window to the reference 0–4 window. The 1–4 window showed the closest characteristics to the reference window for LF. Figure 2 shows the same windows for RMSSD in SU and ST. Figure 3 shows the shortest acceptable window for RMSSD (0–2) in the supine position only.
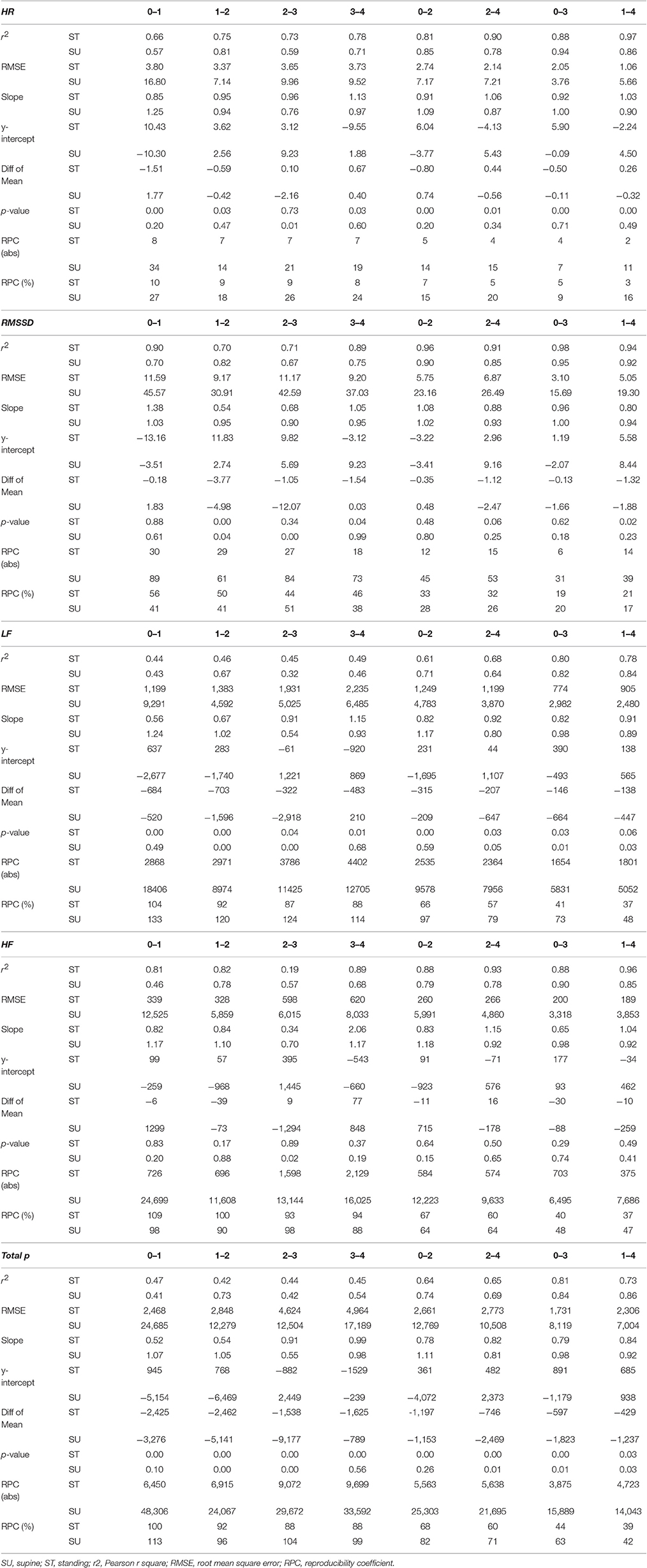
Table 1. Systematic correlation and Bland and Altman data for all windows comparison to the reference 0–4 window.
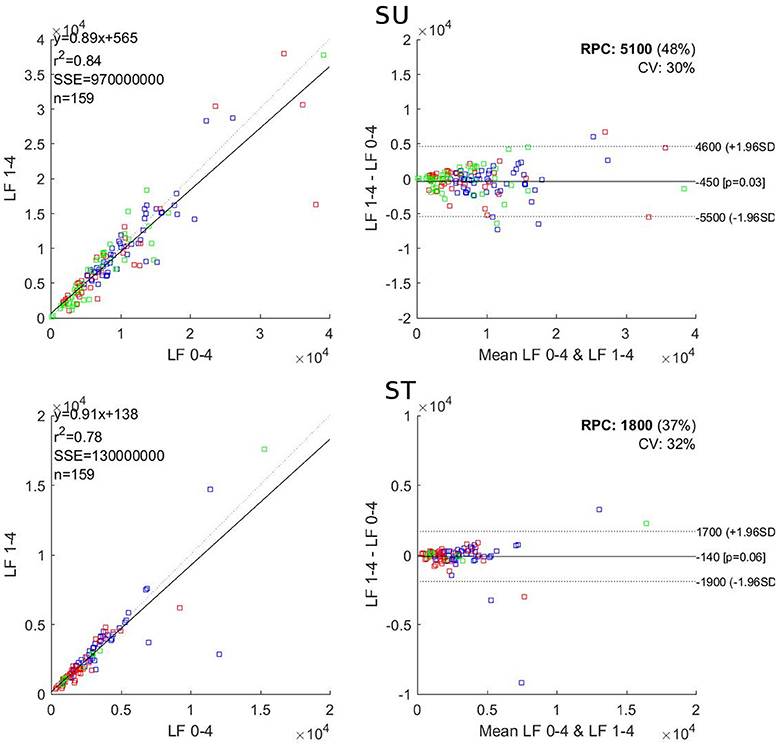
Figure 1. Correlation (Left) and Bland and Altman (Right) plots for comparison of LF in the 1–4 and the reference 0–4 windows. SU, supine; ST, standing; r2, Pearson r square; SSE, sum of square error; RPC, reproducibility coefficient; CV, coefficient of variation. Blue dots: Subject 1; red dots Subject 2; green dots: Subject 3.
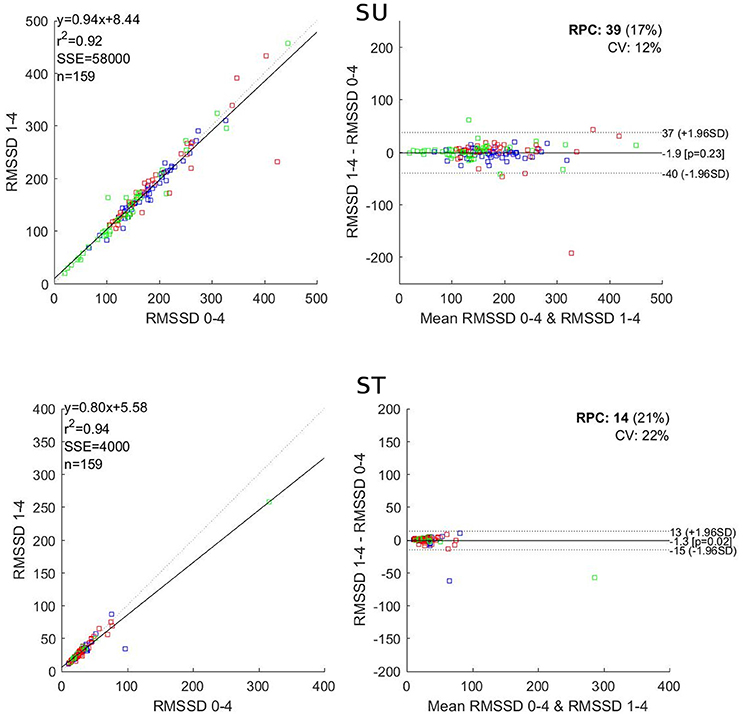
Figure 2. Correlation (Left) and Bland and Altman (Right) plots for comparison of RMSSD in the 1–4 and the reference 0–4 windows. SU, supine; ST, standing; r2, Pearson r square; SSE, sum of square error; RPC, reproducibility coefficient; CV, coefficient of variation. Blue dots: Subject 1; red dots Subject 2; green dots: Subject 3.
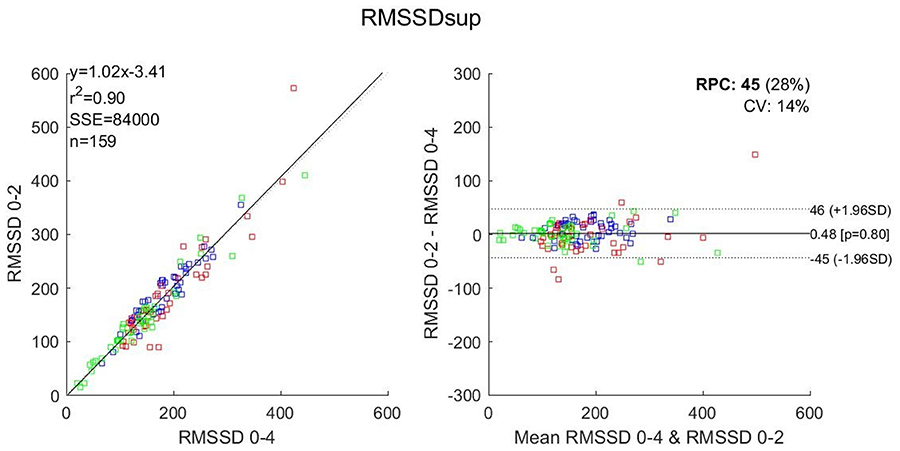
Figure 3. Correlation (Left) and Bland and Altman (Right) plots for comparison of RMSSD in the 0–2 and the reference 0–4 windows in the supine position. r2, Pearson r square; SSE, sum of square error; RPC, reproducibility coefficient; CV, coefficient of variation. Blue dots: Subject 1; red dots Subject 2; green dots: Subject 3.
For RMSSD, the 3-min windows showed r2 values ranging from 0.92 to 0.98, a mean bias between −1.88 and −0.13 and a significant difference for the 1–3 window in ST. The 2-min windows showed r2 values ranging from 0.85 to 0.96, a mean bias between −2.47 and 0.48 and showed no significant difference. The 1-min windows showed r2 values ranging from 0.67 to 0.90, a mean bias between −12.07 and 0.03 and significant differences for 1–2, 2–3, and 3–4 windows.
For LF, the 3-min windows showed r2 values ranging from 0.78 to 0.84, a mean bias between −664 and −138 and a significant difference for the 1–3 window in ST. The 2-min windows showed r2 values ranging from 0.61 to 0.71, a mean bias between −647 and −207 and showed significant difference for the 0–2 window in SU. The 1-min windows showed r2 values ranging from 0.32 to 0.67, a mean bias between −2,918 and 210 and significant differences for 0–1 and 3–4 windows.
For HF, the 3-min windows showed r2 values ranging from 0.85 to 0.96, a mean bias between −259 and −30 and showed no significant difference. The 2-min windows showed r2 values ranging from 0.78 to 0.93, a mean bias between −178 and 715 and no significant difference. The 1-min windows showed r2 values ranging from 0.19 to 0.89, a mean bias between −1,294 and 1,299 and a significant difference for the 2–3 window in SU.
For total power, the 3-min windows showed r2 values ranging from 0.73 to 0.86, a mean bias between −1,823 and −429 and significant difference in all cases. The 2-min windows showed r2 values ranging from 0.64 to 0.74, a mean bias between −2,469 and −597 and significant differences for all cases except one. The 1-min windows showed r2 values ranging from 0.41 to 0.73, a mean bias between −9,177 and −789 and significant differences in all cases except two.
Discussion
In this work, we present a systematic comparison between an extended version of the orthostatic test, 13 min in total resulting in 4-min long analysis windows, and shortened analysis windows. Our main findings are that the analysis windows, when shortened, become less reliable in a significant manner for LF and total power. However, regarding RMSSD, shortening the analysis windows from 4 to 2 min is acceptable. In all regards 1-min windows led to deteriorated results.
The parameter most sensitive to window time reduction was LF. Cutting the first min of the 4-min reference window induced significant differences between the two windows (p < 0.05 SU; p ≈ 0.06 ST) and diminished the correlation coefficient (r2 = 0.84 SU; r2 = 0.78 ST) as seen in Figure 1. Yet, the 1–4 window should be the closest possible to the reference window as the subjects have been resting in a quiet environment, in supine position, for the last 4 min before the start point of that particular window. The LF band encompasses frequencies as low as 0.04 Hz, which means that, in 4 min, there is a maximum of only 9.6 full waveforms, which drops at 7.2 for 3 min. This drop appears sufficient to significantly alter the LF value when the window is shortened by 1 min. In the HF band the lowest selected frequency is 0.15 Hz, which means that over a 4-min window there is a maximum of 36 full waveforms, which drops at 27 for 3 min, a number still sufficient to keep good consistency between 3 and 4-min windows (r2 above 0.85 and no significant difference between the windows). The total power is negatively affected (poor r2 and significant differences both SU and ST) because LF is affected. Al Haddad et al. (2011) showed that the frequency domain parameters were less reproducible than the time domain ones during post-exercise recovery; which is in favor of an impaired LF when shortening the recording window.
A previously published study accurately categorizes the individual patterns of five HRV parameters in “no fatigue” and four types of fatigue (Schmitt et al., 2015b). These distinct patterns encompass increases and/or decreases in HR as well as in LF and HF components. These changes are differently sized in supine and standing positions, and sometimes directed contrariwise in each position. A main outcome of this previous work was that supine and standing HRV variables were fully independent, and non-commutable in the clustering of alterations from the individual normal “no fatigue” patterns (Schmitt et al., 2015a). So, LF is a major element of the HRV analysis and should be included on a regular basis, which makes the 4-min window mandatory. In the context of the entire orthostatic test this corresponds to 1 min resting supine followed by 4 min of recording plus 1 min standing followed by 4 min of recording, thus a total of 1+4+1+4 = 10 min.
However, we acknowledge that RMSSD measures are commonly recommended and therefore might have an effective practical usefulness to help the practitioner to identify a global “fatigue” level. In the perspective of reducing the duration of the orthostatic test to the drastic minimum, only using RMSSD, a 2-min window should be used; which in the context of the entire orthostatic test would make 1 min resting supine followed by 2 min of recording plus 1 min standing followed by 2 min of recording, thus a total of 1+2+1+2 = 6 min. Reducing the duration of the HRV data collection any further implies the suppression of the standing position, which would result in the supine only 1+2 = 3 min HRV test, as illustrated in Figure 3. Any test shorter than 3 min is dangerously exposed to imprecise computation of both the temporal and frequency domains which may result in the wrong diagnosis of the “fatigue” or “no fatigue” state. Accordingly, Munoz et al. (2015) studying a cohort of 3,387 adults, concluded that RMSSD should not be assessed using recordings shorter than 2 min, which is in accordance with the present work, focusing on elite athletes.
Esco and Flatt (2014) proposed to reduce the recording windows to 1 min for RMSSD, despite significant differences vs. their reference 5-min window. Pereira et al. (2016) also found that a 1-min window was acceptable for RMSSD assessment, however their correlation coefficient was 0.85 (which for us is the edge of the acceptability). Moreover, they did not report whether the 1-min window induced significant difference when compared to the 5-min reference window. Additionally, when assessing the seasonal changes in futsal players, these authors relied on 5-min recordings rather than 1-min (Oliveira et al., 2013). In these studies as in most previous ones (Nakamura et al., 2017), the tested populations were very homogeneous, athletes, usually from a single team, taken at a given moment of their sport season; which is in favor a good reliability when reducing the recording duration, but which is clearly not representative of the intra-individual variations of the HRV parameters over longer periods (e.g., a whole year), potentially including several changes in fitness and fatigue states. In the present dataset, there are recordings corresponding to fatigue and non-fatigue states that were collected at various moments of the season, therefore corresponding to various training and competing modalities, including seasonal changes. In other words, our dataset encompasses a multitude of athletes' autonomic responses corresponding to an actual follow-up over several years and shows that under these circumstances the reliability of the 1-min window duration is questionable. Additionally, previous studies providing daily exercise prescription based on HRV over several weeks of follow-up also use windows of several minutes to assess the time domain parameters (Kiviniemi et al., 2010).
Several studies showed good reliability of ultra-short HRV recordings (<1 min) considering RMSSD. However, most of them focused on the post-exercise recovery phase (Goldberger et al., 2006; Al Haddad et al., 2011; Esco and Flatt, 2014; Gomes et al., 2017) which is not the purpose of the present study. Munoz et al. (2015) claimed that 10 s recordings, showing a r2 value of 0.728 compared to 3-min recordings is “substantial,” yet concluded that RMSSD should not be assessed using recordings lasting less than 2 min. To our knowledge, 10, 20, or 30 s recording windows have never been proposed as a procedure to ensure athletes' follow-up.
The European and American societies concerned (1996) recommend that the recording should last for at least 10 times the wavelength of the lower frequency bound of the investigated component, which is the case for our 4-min window encompassing 9.6 waveforms for the LF band. The societies also stated that the LF band requires the most extended recording duration with regard to the time domain parameters, which is in accordance with our present findings. Finally, the societies recommend 5-min recordings of a stationary system. The present work indicates that the last 4 min are sufficient for the window analysis, the first min being dedicated to the reaching of the stationary state of the subject.
In the orthostatic test originally designed by Schmitt et al. (2013), there are 3 min supine before the analysis window starts. This period is dedicated to let the respiratory rhythm settle to a basal rest level so that the respiratory sinus arrhythmia (RSA) does not drift during the HRV test, possibly jumping from HF to LF (and vice-versa) and consequently inducing misinterpretation of the frequency domain parameters (Saboul et al., 2014; Wessel et al., 2016). Although it is essential that the athletes be in a complete resting state when performing the HRV test (ideally in the morning upon waking up), we believe that reducing the time period before the start of the analysis window to 1 min is sufficient. However, future research is necessary to confirm the minimal duration needed. Also, future research should focus on the development of a tool for the extraction of the respiratory rate without a reference respiration signal (Mirmohamadsadeghi et al., 2016).
Conclusion
The present work shows that reducing the window duration for HRV analysis below 4 min negatively affects the outcomes for the low frequency band and the total power. Additionally, we found that reducing the window duration to 2 min is acceptable when RMSSD only is considered. 1-min windows significantly deteriorate the HRV analysis for all parameters. When both the time and the frequency domain parameters are considered we recommend 5 min supine followed by 5 min standing, the analyses being performed on the last 4-min in each position resulting in a 10-min orthostatic test. When RMSSD only is considered, 3 min supine seems the minimal duration or 3 min supine followed by 3 min standing should be sufficient, resulting in a 6-min orthostatic test.
Author Contributions
LS and GM designed the study; LS collected the data; NB, SY, and JV performed the signal processing and data analysis; NB drafted and wrote the article; NB prepared the figures and performed the statistical analysis; LS, SY, JV, and GM reviewed the manuscript. All authors approved the final version of the manuscript.
Funding
This study was performed in the framework of the Nano-Tera initiative supported by the Swiss National Science Foundation (SNSF).
Conflict of Interest Statement
The authors declare that the research was conducted in the absence of any commercial or financial relationships that could be construed as a potential conflict of interest.
References
(1996). Heart rate variability. Standards of measurement, physiological interpretation, and clinical use. task force of the European Society of Cardiology and the North American Society of pacing and electrophysiology. Eur. Heart J. 17, 354#381. doi: 10.1093/oxfordjournals.eurheartj.a014868
Al Haddad, H., Laursen, P. B., Chollet, D., Ahmaidi, S., and Buchheit, M. (2011). Reliability of resting and postexercise heart rate measures. Int. J. Sports Med. 32, 598–605. doi: 10.1055/s-0031-1275356
Buchheit, M. (2014). Monitoring training status with HR measures: do all roads lead to Rome? Front. Physiol. 5:73. doi: 10.3389/fphys.2014.00073
Buchheit, M., Papelier, Y., Laursen, P. B., and Ahmaidi, S. (2007). Noninvasive assessment of cardiac parasympathetic function: postexercise heart rate recovery or heart rate variability? Am. J. Physiol. Heart Circ. Physiol. 293, H8–H10. doi: 10.1152/ajpheart.00335.2007
Esco, M. R., and Flatt, A. A. (2014). Ultra-short-term heart rate variability indexes at rest and post-exercise in athletes: evaluating the agreement with accepted recommendations. J. Sports Sci. Med. 13, 535–541.
Garet, M., Tournaire, N., Roche, F., Laurent, R., Lacour, J. R., Barthélémy, J. C., et al. (2004). Individual interdependence between nocturnal ANS activity and performance in swimmers. Med. Sci. Sports Exerc. 36, 2112–2118. doi: 10.1249/01.MSS.0000147588.28955.48
Goldberger, J. J., Le, F. K., Lahiri, M., Kannankeril, P. J., Ng, J., and Kadish, A. H. (2006). Assessment of parasympathetic reactivation after exercise. Am. J. Physiol. Heart Circ. Physiol. 290, H2446–H2452. doi: 10.1152/ajpheart.01118.2005
Gomes, R. L., Marques Vanderlei, L. C., Garner, D. M., Ramos Santana, M. D., de Abreu, L. C., and Valenti, V. E. (2017). Poincaré plot analysis of ultra-short-term heart rate variability during recovery from exercise in physically active men. J. Sports Med. Phys. Fitness. doi: 10.23736/S0022-4707.17.06922-5. [Epub ahead of print].
Hug, B., Heyer, L., Naef, N., Buchheit, M., Wehrlin, J. P., and Millet, G. P. (2014). Tapering for marathon and cardiac autonomic function. Int. J. Sports Med. 35, 676–683. doi: 10.1055/s-0033-1361184
Kiviniemi, A. M., Hautala, A. J., Kinnunen, H., Nissilä, J., Virtanen, P., Karjalainen, J., et al. (2010). Daily exercise prescription on the basis of HR variability among men and women. Med. Sci. Sports Exerc. 42, 1355–1363. doi: 10.1249/MSS.0b013e3181cd5f39
Meeusen, R., Duclos, M., Foster, C., Fry, A., Gleeson, M., Nieman, D., et al. (2013). Prevention, diagnosis, and treatment of the overtraining syndrome: joint consensus statement of the European College of Sport Science and the American College of Sports Medicine. Med. Sci. Sports Exerc. 45, 186–205. doi: 10.1249/MSS.0b013e318279a10a
Mirmohamadsadeghi, L., Bourdillon, N., and Vesin, J. (2016). “Cardio-respiratory characterization of the autonomic balance,” in Computing in Cardiology. (Vancouver, BC: IEEE).
Munoz, M. L., van Roon, A., Riese, H., Thio, C., Oostenbroek, E., Westrik, I., et al. (2015). Validity of (Ultra-)Short recordings for heart rate variability measurements. PLoS ONE 10:e0138921. doi: 10.1371/journal.pone.0138921
Nakamura, F. Y., Pereira, L. A., Esco, M. R., Flatt, A. A., Moraes, J. E., Cal Abad, C. C., et al. (2017). Intraday and interday reliability of ultra-short-term heart rate variability in rugby union players. J. Strength Cond. Res. 31, 548–551. doi: 10.1519/JSC.0000000000001514
Oliveira, R. S., Leicht, A. S., Bishop, D., Barbero-Álvarez, J. C., and Nakamura, F. Y. (2013). Seasonal changes in physical performance and heart rate variability in high level futsal players. Int. J. Sports Med. 34, 424–430. doi: 10.1055/s-0032-1323720
Pereira, L. A., Flatt, A. A., Ramirez-Campillo, R., Loturco, I., and Nakamura, F. Y. (2016). Assessing shortened field-based heart-rate-variability-data acquisition in team-sport athletes. Int. J. Sports Physiol. Perform. 11, 154–158. doi: 10.1123/ijspp.2015-0038
Pichot, V., Roche, F., Gaspoz, J. M., Enjolras, F., Antoniadis, A., Minini, P., et al. (2000). Relation between heart rate variability and training load in middle-distance runners. Med. Sci. Sports Exerc. 32, 1729–1736. doi: 10.1097/00005768-200010000-00011
Plews, D. J., Laursen, P. B., Kilding, A. E., and Buchheit, M. (2012). Heart rate variability in elite triathletes, is variation in variability the key to effective training? A case comparison. Eur. J. Appl. Physiol. 112, 3729–3741. doi: 10.1007/s00421-012-2354-4
Plews, D. J., Laursen, P. B., Kilding, A. E., and Buchheit, M. (2013). Evaluating training adaptation with heart-rate measures: a methodological comparison. Int. J. Sports Physiol. Perform. 8, 688–691. doi: 10.1123/ijspp.8.6.688
Plews, D. J., Scott, B., Altini, M., Wood, M., Kilding, A. E., and Laursen, P. B. (2017). Comparison of heart rate variability recording with smart phone photoplethysmographic, polar H7 chest strap and electrocardiogram methods. Int. J. Sports Physiol. Perform. 14, 1–17. doi: 10.1123/ijspp.2016-0668
Saboul, D., Pialoux, V., and Hautier, C. (2014). The breathing effect of the LF/HF ratio in the heart rate variability measurements of athletes. Eur. J. Sport Sci. 14(Suppl. 1), S282–S288. doi: 10.1080/17461391.2012.691116
Sandercock, G. R. H., and Brodie, D. A. (2006). The use of heart rate variability measures to assess autonomic control during exercise. Scand. J. Med. Sci. Sports 16, 302–313. doi: 10.1111/j.1600-0838.2006.00556.x
Schmitt, L., Hellard, P., Millet, G. P., Roels, B., Richalet, J. P., and Fouillot, J. P. (2006). Heart rate variability and performance at two different altitudes in well-trained swimmers. Int. J. Sports Med. 27, 226–231. doi: 10.1055/s-2005-865647
Schmitt, L., Regnard, J., Auguin, D., and Millet, G. P. (2016). Monitoring training and fatigue with heart rate variability: case study in a swimming Olympic champion. J. Fitness Res. 5.
Schmitt, L., Regnard, J., Desmarets, M., Mauny, F., Mourot, L., Fouillot, J.-P., et al. (2013). Fatigue shifts and scatters heart rate variability in elite endurance athletes. PLoS ONE 8:e71588. doi: 10.1371/journal.pone.0071588
Schmitt, L., Regnard, J., and Millet, G. P. (2015a). Monitoring fatigue status with HRV measures in elite athletes: an avenue beyond RMSSD? Front. Physiol. 6:343. doi: 10.3389/fphys.2015.00343
Schmitt, L., Regnard, J., Parmentier, A. L., Mauny, F., Mourot, L., Coulmy, N., et al. (2015b). Typology of “fatigue” by heart rate variability analysis in elite nordic-skiers. Int. J. Sports Med. 36, 999–1007. doi: 10.1055/s-0035-1548885
Seiler, S., Haugen, O., and Kuffel, E. (2007). Autonomic recovery after exercise in trained athletes: intensity and duration effects. Med. Sci. Sports Exerc. 39, 1366–1373. doi: 10.1249/mss.0b013e318060f17d
Vesin, J. M., Yazdani, S., Mirmohamadsadeghi, L., and Bourdillon, N. (2016). “Extraction and analysis of short-time excursions in rr-interval time series,” in Computing in Cardiology. (Vancouver, BC: IEEE).
Keywords: accuracy, HRV, fatigue, RMSSD, LF-HF, athlete
Citation: Bourdillon N, Schmitt L, Yazdani S, Vesin J-M and Millet GP (2017) Minimal Window Duration for Accurate HRV Recording in Athletes. Front. Neurosci. 11:456. doi: 10.3389/fnins.2017.00456
Received: 08 June 2017; Accepted: 26 July 2017;
Published: 10 August 2017.
Edited by:
Mathias Baumert, University of Adelaide, AustraliaReviewed by:
Fábio Yuzo Nakamura, Universidade Estadual de Londrina, BrazilLinda F. Hayward, University of Florida, United States
Vitor E. Valenti, Universidade Estadual Paulista Júlio Mesquita Filho, Brazil
Copyright © 2017 Bourdillon, Schmitt, Yazdani, Vesin and Millet. This is an open-access article distributed under the terms of the Creative Commons Attribution License (CC BY). The use, distribution or reproduction in other forums is permitted, provided the original author(s) or licensor are credited and that the original publication in this journal is cited, in accordance with accepted academic practice. No use, distribution or reproduction is permitted which does not comply with these terms.
*Correspondence: Nicolas Bourdillon, nicolas.bourdillon@unil.ch