- 1The John B Pierce Laboratory, New Haven, CT, USA
- 2Department of Psychiatry, Yale University School of Medicine, New Haven, CT, USA
Evidence accumulates linking obesity and diabetes with cognitive dysfunction. At present the mechanism(s) underlying these associations and the relative contribution of diet, adiposity, and metabolic dysfunction are unknown. In this perspective key gaps in knowledge are outlined and an initial sketch of a neuropsychological profile is developed that points toward a critical role for dopamine (DA) adaptations in neurocognitive impairment secondary to diabetes and obesity. The precise mechanisms by which diet, metabolic dysfunction, and adiposity influence the DA system to impact cognition remains unclear and is an important direction for future research.
Neurocognitive Impairments in Type 2 Diabetes (T2D)
T2D is associated with cognitive decline, brain dysfunction, and dementia (Biessels et al., 2014; Koekkoek et al., 2015; Stoeckel et al., 2016). One recent study estimated that the combined overall relative risk for dementia is 73% higher in people with, compared to without T2D, indicating that between 1 in 10 and 1 in 15 incidences of dementia may be attributable to T2D (Biessels et al., 2014). Although glucose intolerance is diagnostic of T2D, a recent systematic review of 86 papers examining T2D and cognition only reported a weak association between glycaemia, and cognition (Geijselaers et al., 2015) and there is even less evidence for an association with other measures of peripheral glucose regulation and cognitive function (e.g., insulin concentration, insulin action, insulin resistance) (Geijselaers et al., 2015). Thus, although T2D is by definition associated with altered glucose metabolism, it is not clear that altered glucose metabolism contributes to cognitive change. The mechanism behind the link between cognitive dysfunction and T2D is therefore not clear.
Neurocognitive Deficits May Arise from Chronic Conditions Associated with T2D
The majority of human studies linking T2D to cognitive decline are performed in older individuals with long-standing diagnoses of diabetes (Stoeckel et al., 2016). This poses a problem for interpreting the pathophysiology of the link between T2D and cognition because individuals with chronic T2D exhibit a number of pathologies associated with cognitive decline such as damage to the blood brain barrier (BBB), neuroinflammation (Banks et al., 2012; Steculorum et al., 2014), cerebral atrophy, and small vessel disease (Biessels and Reijmer, 2014; Akrivos et al., 2015; Ramos-Rodriguez et al., 2016; Stranahan et al., 2016). The co-occurrence of these pathologies that are secondary to diabetes has led to controversy over whether it is T2D (Biessels and Reagan, 2015) or complications arising from T2D that leads to cognitive decline (De Felice and Ferreira, 2014). To rule-out confounds associated with the secondary complications of T2D it will be informative to study cognition in populations free from other chronic conditions and in populations prior to the onset of T2D. For example, it would be informative to characterize neurocognition in youth before and after the onset of prediabetes, since this population will be free from other chronic conditions that could influence cognitive function.
It is Unknown if Neurocognitive Deficits are Associated with T2D or Adiposity or Both
Perhaps the most important limitation of the current literature is the failure to disentangle effects of metabolic dysfunction on cognition from those of adiposity and diet. Obesity has been associated with altered brain structure and function in animal models and in metabolically and neurologically healthy adults and children (Elias et al., 2003; Reinert et al., 2013; Hsu and Kanoski, 2014; Yau et al., 2014; Bocarsly et al., 2015), while diets high in saturated fat and cholesterol are correlated with compromised cognitive flexibility and processing speed in pre-pubertal children after adjusting for age, sex, socioeconomic status, IQ, VO2max, and BMI (Khan et al., 2015b). Consumption of a high fat diet (HFD) can also negatively impact brain and brain function well-before obesity onset. For example, in animal models hypothalamic insulin resistance is observed following acute exposure to HFD before changes in adiposity occur (Clegg et al., 2011) and impaired performance on hippocampal-dependent tasks is observed after only 72-h access to a HFD when animals have actually lost weight, presumably due to neophobia (Kanoski and Davidson, 2010). These findings suggest that obesity can impact cognition independently from metabolic disease and that diet can impact metabolic function and cognition independently of obesity.
To date, studies have rarely taken obesity and diet into account when examining the relationship between T2D and cognition. For example, patients with T2D exhibit reduced activity in the default mode network (Musen et al., 2012), which has been associated with a wide range of neurological conditions and cognitive impairments (Browndyke et al., 2017; Contreras et al., 2017; Jockwitz et al., 2017; von Rhein et al., 2017) but BMI, which was higher in T2D, was not accounted for. Similarly, the putative confound of glucose intolerance is often not considered when examining the relationship between obesity and cognition. For example, a prospective study examining the impact of obesity on cognition excluded participants for many medical conditions likely to influence cognition, including stroke, dementia, myocardial infarction, and atrial fibrillation but NOT diabetes (Gunstad et al., 2010). They did however, include “glucose intolerance” in their mixed model regression analyses and found that this variable was related to cognitive impairments that also correlated with their adiposity measures (waist-hip ratio). In another study deficits in executive function and declarative memory were observed in 38 middle-aged adults with insulin resistance but without T2D compared to 54 age, gender, education but NOT BMI matched controls. Since the insulin resistance group had significantly higher BMI these deficits may be equally attributable to BMI (Bruehl et al., 2010).
Failure to account for confounds between diet, obesity, and metabolic dysfunction also pervade the animal literature. Rats prone to develop diabetes upon HFD are often used as a model of T2D (Levin and Routh, 1996). These models have been associated with deficits on the water maze (Li et al., 2002; Stranahan et al., 2008b), object recognition test (Stranahan et al., 2008a), contextual cue conditioning (Grillo et al., 2011), and discrimination and reversal learning (Kanoski et al., 2007, 2010). HFD has also been shown to increase inflammatory cytokines and impair neuroplasticity and learning and memory in the hippocampus (Erion et al., 2014). The extent to which adiposity or insulin resistance contributed to these observations is not known. However, impaired cognition is also observed with the streptozotocin (STX)-induced diabetic model, which impairs insulin production without increasing adiposity or requiring a high fat diet, indicating that metabolic dysfunction alone is sufficient to impair cognitive function (Stranahan et al., 2008a).
Importantly, when more than one variable is measured interactions between adiposity, diet, and impaired glucose tolerance are revealed. In obese humans without T2D, insulin sensitivity mediates the relationship between working memory-related activation in the right parietal cortex and BMI (Gonzales et al., 2010), while brain insulin action is selectively impaired in the prefrontal cortex in overweight and obese, but not diabetic adults compared to their lean counterparts (Kullmann et al., 2015), highlighting interactions between adiposity and glucose tolerance on brain function.
In summary, the relative contribution of diet, impaired glucose tolerance, and adiposity to neurocognitive impairment is largely unexplored and unknown.
Characterization of Glycemia
Another factor clouding the association between T2D and cognitive impairment is the use of a variety of methods to characterize glycemia, each of which reflect different, and sometimes independent, aspects of glucose metabolism (Geijselaers et al., 2015). Insulin sensitivity can be measured using a variety of techniques. HbA1c, which reflects the mean glucose concentration over a period of 8–12 weeks is the most frequently used measure. Fasting blood glucose concentrations are also frequently measured, which reflect nocturnal hepatic gluconeogenesis that is influenced by hepatic insulin sensitivity, but a recent review found that studies often fail to report whether measurements are taken at the same time of day (Geijselaers et al., 2015). Other measures include post-prandial glucose concentrations, reflecting insulin secretory responses and HOMA-IR to measure insulin resistance. HbA1c shows the strongest association to insulin resistance, followed by post-prandial measures. Fasting glucose, by contrast, seems to be unrelated to cognitive performance (Geijselaers et al., 2015). Interestingly, one study found that insulin resistance was related to declarative memory whereas HbA1c was associated with executive dysfunction (Bruehl et al., 2010), hinting at the possibility that the different measures are associated with distinct pathophysiological effects on the brain and highlighting the need for more comprehensive measures of glucose metabolism.
Neurocognitive Impairments May be Related to Central Rather than (or in Addition to) Peripheral Impairments in Glucose Tolerance
Insulin receptors are widely distributed in the brain, with the highest concentrations in the olfactory bulb, hypothalamus, cerebral cortex, cerebellum, and hippocampus (Havrankova et al., 1978; van Houten et al., 1979). Brain-specific deletion of the insulin receptor in mice results in glycogen synthase kinase 2 beta activation resulting in hyperphosphorylation of tau protein, a hallmark of early Alzheimer's Disease (AD) (Schubert et al., 2004). There is also evidence from animal studies that disrupted central insulin and insulin-like growth factor-1 (IGF-1) signaling may lead to disrupted neurotransmitter (e.g., dopamine) and astroglial cell function, brain endothelial cell function involved in formation and regulation of BBB, mitochondrial metabolism and oxidative stress, clearance of Aβ and/or amyloid fibrils, cholesterol synthesis in the brain (important for myelination and membrane function), glucose and lipid metabolism in select regions of the brain, and regulation of central energy balance, which could relate to both metabolic and neurocognitive dysfunction (Brüning et al., 2000; Convit et al., 2003; Schubert et al., 2004; Suzuki et al., 2010; Kleinridders et al., 2014; Stouffer et al., 2015). While these data suggest a likely role for central insulin resistance in impaired neurocognitive function, it is important to note that central insulin resistance has a complicated relationship with peripheral glycemic control (Ketterer et al., 2011). Central insulin resistance is thought to result from a combination of impaired insulin receptor signaling and decreases in the transport of insulin across the BBB (Banks et al., 2012), which can occur secondary to peripheral glucose intolerance (Niswender et al., 2003). Conversely, central insulin signaling contributes to peripheral glucose regulation (Brüning et al., 2000; Heni et al., 2014) to create a dynamic brain-gut axis regulating glucose metabolism. Importantly, however, central insulin resistance can occur independently from peripheral impairments in glucose tolerance. Post-mortem studies of brain tissue from patients with AD but not T2D, reveal disrupted brain insulin signaling (De Felice and Ferreira, 2014; Yarchoan and Arnold, 2014). Accordingly, treatment with intranasal insulin, which results in direct insulin transport from the nasal cavity to the CNS via intraneuronal and extraneuronal pathways (Reger and Craft, 2006), improves cognition in patients with (Reger et al., 2008; Craft et al., 2012) and without (Hallschmid et al., 2007, 2008) dementia. These findings underscore the importance of concurrent measures of peripheral and central insulin resistance.
One promising avenue for future research is in using intranasal insulin in combination with neuroimaging methodologies and neuropsychological testing to assess the role of central insulin resistance in neurocognition (Tschritter et al., 2006; Ketterer et al., 2011; Grichisch et al., 2012; Kullmann et al., 2013, 2015; Heni et al., 2014, 2016). For example, intranasal insulin decreases the blood oxygen dependent (BOLD) response in the hypothalamus and PFC increases BOLD response in the striatum (Schilling et al., 2014) and insular cortex (Heni et al., 2012) and increases brain energy levels (Jauch-Chara et al., 2012). Critically, these effects are blunted in obesity (Tschritter et al., 2006) with evidence that hypothalamic insulin resistance is driven by visceral fat and frontal insulin resistance by peripheral insulin sensitivity (Kullmann et al., 2015). Collectively, these findings indicate a complex relationship between peripheral glucose control and central insulin resistance and they raise the possibility that central insulin resistance contributes to cognitive impairment in concert with, or independently from peripheral impaired glucose tolerance.
Neurocognitive Deficits May be Domain-Specific and Differentially Influenced by Diet, Adiposity, and Metabolic Dysfunction
Although obesity and T2D are occasionally associated with global measures of brain atrophy (Enzinger et al., 2005; Gunstad et al., 2010; Raji et al., 2010; Brooks et al., 2013) and cognitive decline (Liang et al., 2014), many studies suggest that executive function is the domain most affected in both adults (Gunstad et al., 2007; Sabia et al., 2009; Fitzpatrick et al., 2013) (Volkow et al., 2009) and children (Convit et al., 2003; Reinert et al., 2013; Liang et al., 2014). For example, negative correlations are observed between BMI and performance on tasks of executive function but not episodic verbal memory (Volkow et al., 2009) with BMI negatively, and executive performance positively, correlated with baseline prefrontal glucose metabolism. Similarly, a recent meta-analysis of 21 studies concluded that obesity is associated with impairments in decision-making, planning and problem solving with less evidence for associations with verbal fluency and learning and memory (Fitzpatrick et al., 2013). Correspondingly, structural changes (Enzinger et al., 2005; Pannacciulli et al., 2006; Raji et al., 2010; Fotuhi et al., 2012; Bocarsly et al., 2015) and reduced brain connectivity (Musen et al., 2012) are observed in the parietal and prefrontal cortex (PFC), which are critical for executive function.
There is also strong evidence from animal work that HFD produces hippocampal insulin resistance (Biessels and Reagan, 2015) and damage (Hsu and Kanoski, 2014), resulting in impaired hippocampal-dependent cognitive functions (Kanoski and Davidson, 2011). Likewise, hippocampal atrophy is observed in obese humans (Raji et al., 2010) and altered hippocampal white matter connectivity is found in T2D (van Bussel et al., 2016). However, there are inconsistent findings with respect to alterations in hippocampal-dependent episodic memory tasks (Fitzpatrick et al., 2013). For example, significant deficits in working memory and in reinforcement learning are observed in the absence of episodic learning and memory impairment, in obese vs. healthy weight adults that are matched for age, gender, education, and IQ (Coppin et al., 2014). In contrast, impaired episodic memory and decreased hippocampal volume is observed as a function of glucose tolerance (Convit et al., 2003) and intranasal insulin increases the functional connectivity between the hippocampus and PFC in people with T2D (Zhang et al., 2015). Other studies report hippocampal-dependent impairments as a function of saturated fat intake (Francis and Stevenson, 2011) and central, but not whole body adiposity (Khan et al., 2015a). Collectively these data suggest that episodic memory may be affected by diet and metabolic dysfunction while being unrelated to BMI and whole body obesity.
This emerging neuropsychological profile provides an important insight into the pathophysiological mechanism that gives rise to neurocognitive impairment in obesity and T2D. Brain functions associated with diabetes and obesity tend to rely upon DA signaling (Figure 1). For example, the dopaminergic fronto-striatal loop plays a well-known role in working memory, cognitive flexibility, reinforcement learning, and incentive motivation (Frank and Fossella, 2011). It is also critical for response inhibition, the failure of which is associated with addictive like behaviors including overeating (Lokken et al., 2009; Maayan et al., 2011; Lee et al., 2013; Guo et al., 2014; Zhao et al., 2016). Finally, a role for DA in memory via a projection from the ventral tegmental area to the hippocampus has been described (Shohamy and Adcock, 2010). As reviewed above, these DA-dependent cognitive processes are altered in obesity/T2D, raising the possibility that a common pathway by which diet, adiposity and metabolic dysfunction might coalesce to impact cognition is by producing alterations in the DA system (Figure 1). Interestingly, although DA adaptations are considered integral in the development of compulsive behaviors and alterations in reward sensitivity they are not typically considered as a potential mechanism behind other neurocognitive complications in T2D and obesity.
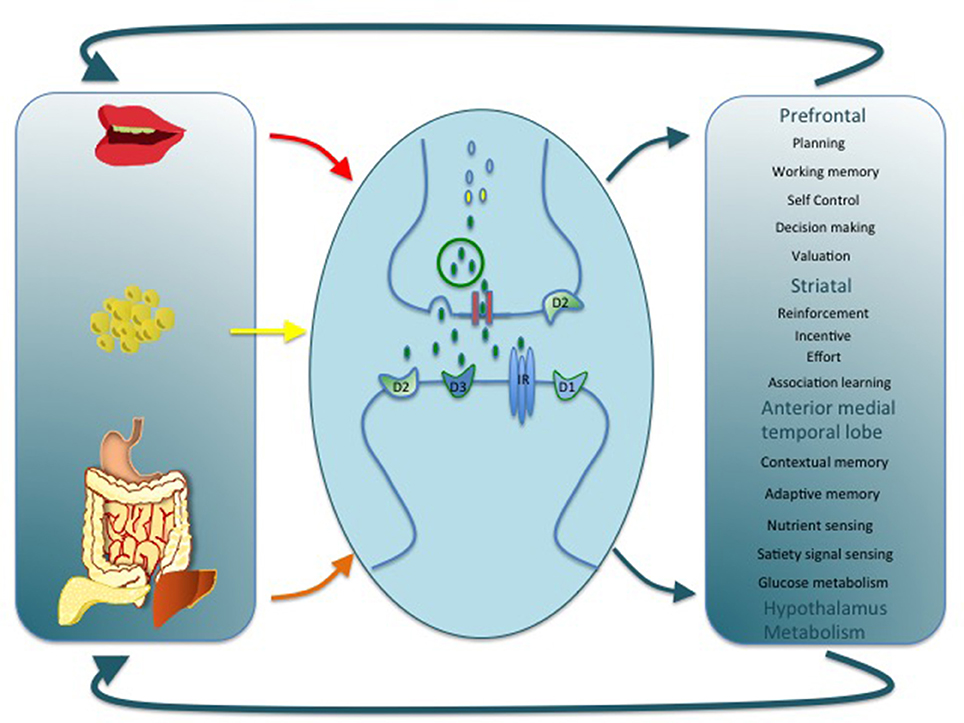
Figure 1. This cartoon depicts alterations in DA signaling as a common link by which diet, adiposity, and metabolic dysfunction might impact cognition, motivation, and energy balance. A variety of mechanisms at the cellular and molecular level could support this association by regulating pre and post-synaptic DA receptor expression, DA synthesis, release, and reuptake. Alterations at any level may in turn have a wide impact on brain function and provide a parsimonious explanation for a number of dysfunctions associated with obesity and T2D.
There are consistent findings in the animal literature that HFD, and adiposity alter DA signaling at the cellular, and molecular levels (Anderzhanova et al., 2007; Johnson and Kenny, 2010; van de Giessen et al., 2012, 2013; Sharma and Fulton, 2013; Tellez et al., 2013; Cansell et al., 2014; Adams et al., 2015; Woods et al., 2016), as well as mounting evidence for altered DA signaling in human obesity, especially reflected in changes in DA receptor density, (Wang et al., 2001, 2011; Dunn et al., 2010; Steele et al., 2010; Eisenstein et al., 2013, 2015; Guo et al., 2014; Cosgrove et al., 2015; Horstmann et al., 2015; Karlsson et al., 2015; Caravaggio et al., 2015b; Dang et al., 2016; Gaiser et al., 2016). Additionally, there is evidence that central and peripheral insulin resistance might impact DA function. Central insulin signaling, acting through the downstream modulator Akt, is a potent modulator of DA transporter (DAT) activity, which fine-tunes DA signaling at the synapse (Kleinridders et al., 2015), demonstrating a pathway by which central IR could influence the DA system. Insulin administration also suppresses DA release by clearing DA from the synapse and concomitantly reducing the rewarding properties of food (Figlewicz and Sipols, 2010). Likewise, peripheral insulin sensitivity is associated with reduced endogenous DA levels (Murzi et al., 1996; Caravaggio et al., 2015a) and peripheral glycemia with PFC-striatal-hippocampal functional connectivity (Page et al., 2013). Thus, diet, adiposity, and insulin resistance could each impact DA signaling with the potential for additive effects and interactions. Future work aiming to disambiguate the unique and interacting effects will therefore be an important step toward understanding neurocognitive impairment in T2D and obesity.
Summary
In summary, a consensus is emerging that obesity and diabetes are accompanied by cognitive impairments and brain dysfunction and that at least some of these effects are secondary to their onset. Multiple mechanisms have been proposed to underlie these associations but at present it is unclear which mechanism, or mechanisms, are critical. Also unclear is whether diet, obesity and metabolic dysfunction have distinct and/or converging pathways to neurocognitive impairment. However, work emerges to suggest that all three factors may influence DA signaling, which is provocative since the cognitive impairments that characterize diabetes and obesity uniformly rely upon the integrity of the DA system. It is therefore proposed that adaptations in DA signaling secondary to diet, adiposity and metabolic dysfunction underlie much of the neurocognitive impairment observed in diabetes and obesity.
Author Contributions
The author confirms being the sole contributor of this work and approved it for publication.
Funding
This work was supported by NIH NCI R01CA180030 awarded to DMS and Ivan de Araujo.
Conflict of Interest Statement
The author declares that the research was conducted in the absence of any commercial or financial relationships that could be construed as a potential conflict of interest.
Acknowledgments
I would like to thank Sonia Caprio and Hubert Priessl for comments on previous versions of this manuscript and Serge Luquet for guidance and contribution on the figure.
References
Adams, W. K., Sussman, J. L., Kaur, S., D'Souza, A. M., Kieffer, T. J., and Winstanley, C. A. (2015). Long-term, calorie-restricted intake of a high-fat diet in rats reduces impulse control and ventral striatal D2 receptor signalling - two markers of addiction vulnerability. Eur. J. Neurosci. 42, 3095–3104. doi: 10.1111/ejn.13117
Akrivos, J., Ravona-Springer, R., Schmeidler, J., LeRoith, D., Heymann, A., Preiss, R., et al. (2015). Glycemic control, inflammation, and cognitive function in older patients with type 2 diabetes. Int. J. Geriatr. Psychiatry 30, 1093–1100. doi: 10.1002/gps.4267
Anderzhanova, E., Covasa, M., and Hajnal, A. (2007). Altered basal and stimulated accumbens dopamine release in obese OLETF rats as a function of age and diabetic status. Am. J. Physiol. Regul. Integr. Comp. Physiol. 293, R603–R611. doi: 10.1152/ajpregu.00301.2007
Banks, W. A., Owen, J. B., and Erickson, M. A. (2012). Insulin in the brain: there and back again. Pharmacol. Ther. 136, 82–93. doi: 10.1016/j.pharmthera.2012.07.006
Biessels, G. J., and Reagan, L. P. (2015). Hippocampal insulin resistance and cognitive dysfunction. Nat. Rev. Neurosci. 16, 660–671. doi: 10.1038/nrn4019
Biessels, G. J., and Reijmer, Y. D. (2014). Brain changes underlying cognitive dysfunction in diabetes: what can we learn from MRI? Diabetes 63, 2244–2252. doi: 10.2337/db14-0348
Biessels, G. J., Strachan, M. W., Visseren, F. L., Kappelle, L. J., and Whitmer, R. A. (2014). Dementia and cognitive decline in type 2 diabetes and prediabetic stages: towards targeted interventions. Lancet. Diabetes Endocrinol. 2, 246–255. doi: 10.1016/s2213-8587(13)70088-3
Bocarsly, M. E., Fasolino, M., Kane, G. A., LaMarca, E. A., Kirschen, G. W., Karatsoreos, I. N., et al. (2015). Obesity diminishes synaptic markers, alters microglial morphology, and impairs cognitive function. Proc. Natl. Acad. Sci. U.S.A. 112, 15731–15736. doi: 10.1073/pnas.1511593112
Brooks, S. J., Benedict, C., Burgos, J., Kempton, M. J., Kullberg, J., Nordenskjold, R., et al. (2013). Late-life obesity is associated with smaller global and regional gray matter volumes: a voxel-based morphometric study. Int. J. Obes. 37, 230–236. doi: 10.1038/ijo.2012.13
Browndyke, J. N., Berger, M., Harshbarger, T. B., Smith, P. J., White, W., Bisanar, T. L., et al. (2017). Resting-state functional connectivity and cognition After major cardiac surgery in older adults without preoperative cognitive impairment: preliminary findings. J. Am. Geriatr. Soc. 65, e6–e12. doi: 10.1111/jgs.14534
Bruehl, H., Sweat, V., Hassenstab, J., Polyakov, V., and Convit, A. (2010). Cognitive impairment in nondiabetic middle-aged and older adults is associated with insulin resistance. J. Clin. Exp. Neuropsychol. 32, 487–493. doi: 10.1080/13803390903224928
Brüning, J. C., Gautam, D., Burks, D. J., Gillette, J., Schubert, M., Orban, P. C., et al. (2000). Role of brain insulin receptor in control of body weight and reproduction. Science 289, 2122–2125. doi: 10.1126/science.289.5487.2122
Cansell, C., Castel, J., Denis, R. G., Rouch, C., Delbes, A. S., Martinez, S., et al. (2014). Dietary triglycerides act on mesolimbic structures to regulate the rewarding and motivational aspects of feeding. Mol. Psychiatry 19, 1095–1105. doi: 10.1038/mp.2014.31
Caravaggio, F., Borlido, C., Hahn, M., Feng, Z., Fervaha, G., Gerretsen, P., et al. (2015a). Reduced insulin sensitivity is related to less endogenous dopamine at D-2/3 receptors in the ventral striatum of healthy nonobese humans. Int. J. Neuropsychopharmacol. 18:pyv014. doi: 10.1093/ijnp/pyv014
Caravaggio, F., Raitsin, S., Gerretsen, P., Nakajima, S., Wilson, A., and Graff-Guerrero, A. (2015b). Ventral striatum binding of a dopamine D2/3 receptor agonist but not antagonist predicts normal body mass index. Biol. Psychiatry 77, 196–202. doi: 10.1016/j.biopsych.2013.02.017
Clegg, D. J., Gotoh, K., Kemp, C., Wortman, M. D., Benoit, S. C., Brown, L. M., et al. (2011). Consumption of a high-fat diet induces central insulin resistance independent of adiposity. Physiol. Behav. 103, 10–16. doi: 10.1016/j.physbeh.2011.01.010
Contreras, J. A., Goni, J., Risacher, S. L., Amico, E., Yoder, K., Dzemidzic, M., et al. (2017). Cognitive complaints in older adults at risk for Alzheimer's disease are associated with altered resting-state networks. Alzheimer's Dement. 6, 40–49. doi: 10.1016/j.dadm.2016.12.004
Convit, A., Wolf, O. T., Tarshish, C., and de Leon, M. J. (2003). Reduced glucose tolerance is associated with poor memory performance and hippocampal atrophy among normal elderly. Proc. Natl. Acad. Sci. U.S.A. 100, 2019–2022. doi: 10.1073/pnas.0336073100
Coppin, G., Nolan-Poupart, S., Jones-Gotman, M., and Small, D. M. (2014). Working memory and reward association learning impairments in obesity. Neuropsychologia 65, 146–155. doi: 10.1016/j.neuropsychologia.2014.10.004
Cosgrove, K. P., Veldhuizen, M. G., Sandiego, C. M., Morris, E. D., and Small, D. M. (2015). Opposing relationships of BMI with BOLD and dopamine D2/3 receptor binding potential in the dorsal striatum. Synapse 69, 195–202. doi: 10.1002/syn.21809
Craft, S., Baker, L. D., Montine, T. J., Minoshima, S., Watson, G. S., Claxton, A., et al. (2012). Intranasal insulin therapy for Alzheimer disease and amnestic mild cognitive impairment: a pilot clinical trial. Arch. Neurol. 69, 29–38. doi: 10.1001/archneurol.2011.233
Dang, L. C., Samanez-Larkin, G. R., Castrellon, J. J., Perkins, S. F., Cowan, R. L., and Zald, D. H. (2016). Associations between dopamine D2 receptor availability and BMI depend on age. Neuroimage 138, 176–183. doi: 10.1016/j.neuroimage.2016.05.044
De Felice, F. G., and Ferreira, S. T. (2014). Inflammation, Defective insulin signaling, and mitochondrial dysfunction as common molecular denominators connecting Type 2 diabetes to Alzheimer disease. Diabetes 63, 2262–2272. doi: 10.2337/db13-1954
Dunn, J. P., Cowan, R. L., Volkow, N. D., Feurer, I. D., Li, R., Williams, D. B., et al. (2010). Decreased dopamine type 2 receptor availability after bariatric surgery: preliminary findings. Brain Res. 1350, 123–130. doi: 10.1016/j.brainres.2010.03.064
Eisenstein, S. A., Antenor-Dorsey, J. A., Gredysa, D. M., Koller, J. M., Bihun, E. C., Ranck, S. A., et al. (2013). A comparison of D2 receptor specific binding in obese and normal-weight individuals using PET with (N-[(11)C]methyl)benperidol. Synapse 67, 748–756. doi: 10.1002/syn.21680
Eisenstein, S. A., Bischoff, A. N., Gredysa, D. M., Antenor-Dorsey, J. A., Koller, J. M., Al-Lozi, A., et al. (2015). Emotional eating phenotype is associated with central dopamine D2 receptor binding independent of body mass index. Sci. Rep. 5:11283. doi: 10.1038/srep11283
Elias, M. F., Elias, P. K., Sullivan, L. M., Wolf, P. A., and D'Agostino, R. B. (2003). Lower cognitive function in the presence of obesity and hypertension: the Framingham heart study. Int. J. Obes. Relat. Metab. Disord. 27, 260–268. doi: 10.1038/sj.ijo.802225
Enzinger, C., Fazekas, F., Matthews, P. M., Ropele, S., Schmidt, H., Smith, S., et al. (2005). Risk factors for progression of brain atrophy in aging - Six-year follow-up of normal subjects. Neurology 64, 1704–1711. doi: 10.1212/01.WNL.0000161871.83614.BB
Erion, J. R., Wosiski-Kuhn, M., Dey, A., Hao, S., Davis, C. L., Pollock, N. K., et al. (2014). Obesity elicits interleukin 1-mediated deficits in hippocampal synaptic plasticity. J. Neurosci. 34, 2618–2631. doi: 10.1523/JNEUROSCI.4200-13.2014
Figlewicz, D. P., and Sipols, A. J. (2010). Energy regulatory signals and food reward. Pharmacol. Biochem. Behav. 97, 15–24. doi: 10.1016/j.pbb.2010.03.002
Fitzpatrick, S., Gilbert, S., and Serpell, L. (2013). Systematic review: are overweight and obese individuals impaired on behavioural tasks of executive functioning? Neuropsychol. Rev. 23, 138–156. doi: 10.1007/s11065-013-9224-7
Fotuhi, M., Do, D., and Jack, C. (2012). Modifiable factors that alter the size of the hippocampus with ageing. Nat. Rev. Neurol. 8, 189–202. doi: 10.1038/nrneurol.2012.27
Francis, H. M., and Stevenson, R. J. (2011). Higher reported saturated fat and refined sugar intake is associated with reduced hippocampal-dependent memory and sensitivity to interoceptive signals. Behav. Neurosci. 125, 943–955. doi: 10.1037/a0025998
Frank, M. J., and Fossella, J. A. (2011). Neurogenetics and pharmacology of learning, motivation, and cognition. Neuropsychopharmacology 36, 133–152. doi: 10.1038/npp.2010.96
Gaiser, E. C., Gallezot, J. D., Worhunsky, P. D., Jastreboff, A. M., Pittman, B., Kantrovitz, L., et al. (2016). Elevated dopamine D2/3 receptor availability in obese individuals: a PET imaging study with [11C](+)PHNO. Neuropsychopharmacology 41, 3042–3050. doi: 10.1038/npp.2016.115
Geijselaers, S. L., Sep, S. J., Stehouwer, C. D., and Biessels, G. J. (2015). Glucose regulation, cognition, and brain MRI in type 2 diabetes: a systematic review. Lancet. Diabetes Endocrinol. 3, 75–89. doi: 10.1016/s2213-8587(14)70148-2
Gonzales, M. M., Tarumi, T., Miles, S. C., Tanaka, H., Shah, F., and Haley, A. P. (2010). Insulin sensitivity as a mediator of the relationship between BMI and working memory-related brain activation. Obesity 18, 2131–2137. doi: 10.1038/oby.2010.183
Grichisch, Y., Çavusoglu, M., Preissl, H., Uludag, K., Hallschmid, M., Birbaumer, N., et al. (2012). Differential effects of intranasal insulin and caffeine on cerebral blood flow. Hum. Brain Mapp. 33, 280–287. doi: 10.1002/hbm.21216
Grillo, C. A., Piroli, G. G., Junor, L., Wilson, S. P., Mott, D. D., Wilson, M. A., et al. (2011). Obesity/hyperleptinemic phenotype impairs structural and functional plasticity in the rat hippocampus. Physiol. Behav. 105, 138–144. doi: 10.1016/j.physbeh.2011.02.028
Gunstad, J., Lhotsky, A., Wendell, C. R., Ferrucci, L., and Zonderman, A. B. (2010). Longitudinal examination of obesity and cognitive function: results from the Baltimore longitudinal study of aging. Neuroepidemiology 34, 222–229. doi: 10.1159/000297742
Gunstad, J., Paul, R. H., Cohen, R. A., Tate, D. F., Spitznagel, M. B., and Gordon, E. (2007). Elevated body mass index is associated with executive dysfunction in otherwise healthy adults. Compr. Psychiatry 48, 57–61. doi: 10.1016/j.comppsych.2006.05.001
Guo, J., Simmons, W. K., Herscovitch, P., Martin, A., and Hall, K. D. (2014). Striatal dopamine D2-like receptor correlation patterns with human obesity and opportunistic eating behavior. Mol. Psychiatry 19, 1078–1084. doi: 10.1038/mp.2014.102
Hallschmid, M., Benedict, C., Born, J., and Kern, W. (2007). Targeting metabolic and cognitive pathways of the CNS by intranasal insulin administration. Expert Opin. Drug Deliv. 4, 319–322. doi: 10.1517/17425247.4.4.319
Hallschmid, M., Benedict, C., Schultes, B., Born, J., and Kern, W. (2008). Obese men respond to cognitive but not to catabolic brain insulin signaling. Int. J. Obes. 32, 275–282. doi: 10.1038/sj.ijo.0803722
Havrankova, J., Roth, J., and Brownstein, M. (1978). Insulin receptors are widely distributed in the central nervous system of the rat. Nature 272, 827–829. doi: 10.1038/272827a0
Heni, M., Kullmann, S., Ahlqvist, E., Wagner, R., Machicao, F., Staiger, H., et al. (2016). Interaction between the obesity-risk gene FTO and the dopamine D2 receptor gene ANKK1/TaqIA on insulin sensitivity. Diabetologia 59, 2622–2631. doi: 10.1007/s00125-016-4095-0
Heni, M., Kullmann, S., Ketterer, C., Guthoff, M., Linder, K., Wagner, R., et al. (2012). Nasal insulin changes peripheral insulin sensitivity simultaneously with altered activity in homeostatic and reward-related human brain regions. Diabetologia 55, 1773–1782. doi: 10.1007/s00125-012-2528-y
Heni, M., Wagner, R., Kullmann, S., Veit, R., Mat Husin, H., Linder, K., et al. (2014). Central insulin administration improves whole-body insulin sensitivity via hypothalamus and parasympathetic outputs in men. Diabetes 63, 4083–4088. doi: 10.2337/db14-0477
Horstmann, A., Fenske, W. K., and Hankir, M. K. (2015). Argument for a non-linear relationship between severity of human obesity and dopaminergic tone. Obes. Rev. 16, 821–830. doi: 10.1111/obr.12303
Hsu, T. M., and Kanoski, S. E. (2014). Blood-brain barrier disruption: mechanistic links between Western diet consumption and dementia. Front. Aging Neurosci. 6:88. doi: 10.3389/fnagi.2014.00088
Jauch-Chara, K., Friedrich, A., Rezmer, M., Melchert, U. H., Scholand-Engler, G. H., Hallschmid, M., et al. (2012). Intranasal insulin suppresses food intake via enhancement of brain energy levels in humans. Diabetes 61, 2261–2268. doi: 10.2337/db12-0025
Jockwitz, C., Caspers, S., Lux, S., Eickhoff, S. B., Jütten, K., Lenzen, S., et al. (2017). Influence of age and cognitive performance on resting-state brain networks of older adults in a population-based cohort. Cortex 89, 28–44. doi: 10.1016/j.cortex.2017.01.008
Johnson, P. M., and Kenny, P. J. (2010). Dopamine D2 receptors in addiction-like reward dysfunction and compulsive eating in obese rats. Nat. Neurosci. 13, 635–641. doi: 10.1038/nn.2519
Kanoski, S. E., and Davidson, T. L. (2010). Different patterns of memory impairments accompany short- and longer-term maintenance on a high-energy diet. J. Exp. Psychol. Anim. Behav. Process. 36, 313–319. doi: 10.1037/a0017228
Kanoski, S. E., and Davidson, T. L. (2011). Western diet consumption and cognitive impairment: links to hippocampal dysfunction and obesity. Physiol. Behav. 103, 59–68. doi: 10.1016/j.physbeh.2010.12.003
Kanoski, S. E., Meisel, R. L., Mullins, A. J., and Davidson, T. L. (2007). The effects of energy-rich diets on discrimination reversal learning and on BDNF in the hippocampus and prefrontal cortex of the rat. Behav. Brain Res. 182, 57–66. doi: 10.1016/j.bbr.2007.05.004
Kanoski, S. E., Zhang, Y., Zheng, W., and Davidson, T. L. (2010). The effects of a high-energy diet on hippocampal function and blood-brain barrier integrity in the rat. J. Alzheimer's Dis. JAD 21, 207–219. doi: 10.3233/JAD-2010-091414
Karlsson, H. K., Tuominen, L., Tuulari, J. J., Hirvonen, J., Parkkola, R., Helin, S., et al. (2015). Obesity is associated with decreased mu-opioid but unaltered dopamine D2 receptor availability in the brain. J. Neurosci. 35, 3959–3965. doi: 10.1523/JNEUROSCI.4744-14.2015
Ketterer, C., Tschritter, O., Preissl, H., Heni, M., Haring, H. U., and Fritsche, A. (2011). Insulin sensitivity of the human brain. Diabetes Res. Clin. Pract. 93, S47–S51. doi: 10.1016/s0168-8227(11)70013-4
Khan, N. A., Baym, C. L., Monti, J. M., Raine, L. B., Drollette, E. S., Scudder, M. R., et al. (2015a). Central adiposity is negatively associated with hippocampal-dependent relational memory among overweight and obese children. J. Pediatr. 166, 302–308. doi: 10.1016/j.jpeds.2014.10.008
Khan, N. A., Raine, L. B., Drollette, E. S., Scudder, M. R., and Hillman, C. H. (2015b). The relation of saturated fats and dietary cholesterol to childhood cognitive flexibility. Appetite 93, 51–56. doi: 10.1016/j.appet.2015.04.012
Kleinridders, A., Cai, W., Cappellucci, L., Ghazarian, A., Collins, W. R., Vienberg, S. G., et al. (2015). Insulin resistance in brain alters dopamine turnover and causes behavioral disorders. Proc. Natl. Acad. Sci. U.S.A. 112, 3463–3468. doi: 10.1073/pnas.1500877112
Kleinridders, A., Ferris, H. A., Cai, W., and Kahn, C. R. (2014). Insulin action in brain regulates systemic metabolism and brain function. Diabetes 63, 2232–2243. doi: 10.2337/db14-0568
Koekkoek, P. S., Kappelle, L. J., van den Berg, E., Rutten, G. E. H. M., and Biessels, G. J. (2015). Cognitive function in patients with diabetes mellitus: guidance for daily care. Lancet Neurol. 14, 329–340. doi: 10.1016/S1474-4422(14)70249-2
Kullmann, S., Frank, S., Heni, M., Ketterer, C., Veit, R., Haring, H. U., et al. (2013). Intranasal insulin modulates intrinsic reward and prefrontal circuitry of the human brain in lean women. Neuroendocrinology 97, 176–182. doi: 10.1159/000341406
Kullmann, S., Heni, M., Veit, R., Scheffler, K., Machann, J., Haring, H. U., et al. (2015). Selective insulin resistance in homeostatic and cognitive control brain areas in overweight and obese adults. Diabetes Care 38, 1044–1050. doi: 10.2337/dc14-2319
Lee, Y., Chong, M. F., Liu, J. C., Libedinsky, C., Gooley, J. J., Chen, S., et al. (2013). Dietary disinhibition modulates neural valuation of food in the fed and fasted states. Am. J. Clin. Nutr. 97, 919–925. doi: 10.3945/ajcn.112.053801
Levin, B. E., and Routh, V. H. (1996). Role of the brain in energy balance and obesity. Am. J. Physiol. 271, R491–R500.
Li, X. L., Aou, S., Oomura, Y., Hori, N., Fukunaga, K., and Hori, T. (2002). Impairment of long-term potentiation and spatial memory in leptin receptor-deficient rodents. Neuroscience 113, 607–615. doi: 10.1016/S0306-4522(02)00162-8
Liang, J., Matheson, B. E., Kaye, W. H., and Boutelle, K. N. (2014). Neurocognitive correlates of obesity and obesity-related behaviors in children and adolescents. Int. J. Obes. 38, 494–506. doi: 10.1038/ijo.2013.142
Lokken, K. L., Boeka, A. G., Austin, H. M., Gunstad, J., and Harmon, C. M. (2009). Evidence of executive dysfunction in extremely obese adolescents: a pilot study. Surg. Obes. Relat. Dis. 5, 547–552. doi: 10.1016/j.soard.2009.05.008
Maayan, L., Hoogendoorn, C., Sweat, V., and Convit, A. (2011). Disinhibited eating in obese adolescents is associated with orbitofrontal volume reductions and executive dysfunction. Obesity 19, 1382–1387. doi: 10.1038/oby.2011.15
Murzi, E., Contreras, Q., Teneud, L., Valecillos, B., Parada, M. A., DeParada, M. P., et al. (1996). Diabetes decreases limbic extracellular dopamine in rats. Neurosci. Lett. 202, 141–144. doi: 10.1016/0304-3940(95)12232-X
Musen, G., Jacobson, A. M., Bolo, N. R., Simonson, D. C., Shenton, M. E., McCartney, R. L., et al. (2012). Resting-state brain functional connectivity is altered in type 2 diabetes. Diabetes 61, 2375–2379. doi: 10.2337/db11-1669
Niswender, K. D., Morrison, C. D., Clegg, D. J., Olson, R., Baskin, D. G., Myers, M. G., et al. (2003). Insulin activation of phosphatidylinositol 3-kinase in the hypothalamic arcuate nucleus - A key mediator of insulin-induced anorexia. Diabetes 52, 227–231. doi: 10.2337/diabetes.52.2.227
Page, K. A., Chan, O., Arora, J., Belfort-Deaguiar, R., Dzuira, J., Roehmholdt, B., et al. (2013). Effects of fructose vs glucose on regional cerebral blood flow in brain regions involved with appetite and reward pathways. JAMA 309, 63–70. doi: 10.1001/jama.2012.116975
Pannacciulli, N., Del Parigi, A., Chen, K., Le, D. S., Reiman, E. M., and Tataranni, P. A. (2006). Brain abnormalities in human obesity: a voxel-based morphometric study. Neuroimage 31, 1419–1425. doi: 10.1016/j.neuroimage.2006.01.047
Raji, C. A., Ho, A. J., Parikshak, N. N., Becker, J. T., Lopez, O. L., Kuller, L. H., et al. (2010). Brain structure and obesity. Hum. Brain Mapp. 31, 353–364. doi: 10.1002/hbm.20870
Ramos-Rodriguez, J. J., Infante-Garcia, C., Galindo-Gonzalez, L., Garcia-Molina, Y., Lechuga-Sancho, A., and Garcia-Alloza, M. (2016). Increased spontaneous central bleeding and cognition impairment in APP/PS1 mice with poorly controlled diabetes mellitus. Mol. Neurobiol. 53, 2685–2697. doi: 10.1007/s12035-015-9311-2
Reger, M. A., and Craft, S. (2006). Intranasal insulin administration: a method for dissociating central and peripheral effects of insulin. Drugs Today 42, 729–739. doi: 10.1358/dot.2006.42.11.1007675
Reger, M. A., Watson, G. S., Green, P. S., Wilkinson, C. W., Baker, L. D., Cholerton, B., et al. (2008). Intranasal insulin improves cognition and modulates beta-amyloid in early AD. Neurology 70, 440–448. doi: 10.1212/01.WNL.0000265401.62434.36
Reinert, K. R., Po'e, E. K., and Barkin, S. L. (2013). The relationship between executive function and obesity in children and adolescents: a systematic literature review. J. Obes. 2013:820956. doi: 10.1155/2013/820956
Sabia, S., Kivimaki, M., Shipley, M. J., Marmot, M. G., and Singh-Manoux, A. (2009). Body mass index over the adult life course and cognition in late midlife: the Whitehall II Cohort study. Am. J. Clin. Nutr. 89, 601–607. doi: 10.3945/ajcn.2008.26482
Schilling, T. M., Ferreira de Sá, D. S., Westerhausen, R., Strelzyk, F., Larra, M. F., Hallschmid, M., et al. (2014). Intranasal insulin increases regional cerebral blood flow in the insular cortex in men independently of cortisol manipulation. Hum. Brain Mapp. 35, 1944–1956. doi: 10.1002/hbm.22304
Schubert, M., Gautam, D., Surjo, D., Ueki, K., Baudler, S., Schubert, D., et al. (2004). Role for neuronal insulin resistance in neurodegenerative diseases. Proc. Natl. Acad. Sci. U.S.A. 101, 3100–3105. doi: 10.1073/pnas.0308724101
Sharma, S., and Fulton, S. (2013). Diet-induced obesity promotes depressive-like behaviour that is associated with neural adaptations in brain reward circuitry. Int. J. Obes. 37, 382–389. doi: 10.1038/ijo.2012.48
Shohamy, D., and Adcock, R. A. (2010). Dopamine and adaptive memory. Trends Cogn. Sci. 14, 464–472. doi: 10.1016/j.tics.2010.08.002
Steculorum, S. M., Solas, M., and Brüning, J. C. (2014). The paradox of neuronal insulin action and resistance in the development of aging-associated diseases. Alzheimers Dement. 10, S3–S11. doi: 10.1016/j.jalz.2013.12.008
Steele, K. E., Prokopowicz, G. P., Schweitzer, M. A., Magunsuon, T. H., Lidor, A. O., Kuwabawa, H., et al. (2010). Alterations of central dopamine receptors before and after gastric bypass surgery. Obes. Surg. 20, 369–374. doi: 10.1007/s11695-009-0015-4
Stoeckel, L. E., Arvanitakis, Z., Gandy, S., Small, D. M., Kahn, C. R., Pascual-Leone, A., et al. (2016). “White Paper” meeting summary and catalyst for future inquiry: complex mechanisms linking neurocognitive dysfunction to insulin resistance and other metabolic dysfunction. F1000Res. 5:353. doi: 10.12688/f1000research.8300.1
Stouffer, M. A., Woods, C. A., Patel, J. C., Lee, C. R., Witkovsky, P., Bao, L., et al. (2015). Insulin enhances striatal dopamine release by activating cholinergic interneurons and thereby signals reward. Nat. Commun. 6:8543. doi: 10.1038/ncomms9543
Stranahan, A. M., Arumugam, T. V., Cutler, R. G., Lee, K., Egan, J. M., and Mattson, M. P. (2008a). Diabetes impairs hippocampal function through glucocorticoid-mediated effects on new and mature neurons. Nat. Neurosci. 11, 309–317. doi: 10.1038/nn2055
Stranahan, A. M., Hao, S., Dey, A., Yu, X., and Baban, B. (2016). Blood-brain barrier breakdown promotes macrophage infiltration and cognitive impairment in leptin receptor-deficient mice. J. Cereb. Blood Flow Metab. 36, 2108–2121. doi: 10.1177/0271678X16642233
Stranahan, A. M., Norman, E. D., Lee, K., Cutler, R. G., Telljohann, R. S., Egan, J. M., et al. (2008b). Diet-induced insulin resistance impairs hippocampal synaptic plasticity and cognition in middle-aged rats. Hippocampus 18, 1085–1088. doi: 10.1002/hipo.20470
Suzuki, R., Lee, K., Jing, E., Biddinger, S. B., McDonald, J. G., Montine, T. J., et al. (2010). Diabetes and insulin in regulation of brain cholesterol metabolism. Cell Metab. 12, 567–579. doi: 10.1016/j.cmet.2010.11.006
Tellez, L. A., Medina, S., Han, W., Ferreira, J. G., Licona-Limón, P., Ren, X., et al. (2013). A gut lipid messenger links excess dietary fat to dopamine deficiency. Science 341, 800–802. doi: 10.1126/science.1239275
Tschritter, O., Porubska, K., Preissl, H., Fritsche, A., and Haring, H. U. (2006). Cerebrocortical insulin resistance is related to visceral obesity and age, but is not independently correlated with leptin, adiponectin or the saturated long-chain fatty acids palmitate and stearate. Diabetes 55, A403–A404.
van Bussel, F. C., Backes, W. H., Hofman, P. A., van Boxtel, M. P., Schram, M. T., Stehouwer, C. D., et al. (2016). Altered hippocampal white matter connectivity in Type 2 diabetes mellitus and memory decrements. J. Neuroendocrinol. 28:12366. doi: 10.1111/jne.12366
van de Giessen, E., la Fleur, S. E., de Bruin, K., van den Brink, W., and Booij, J. (2012). Free-choice and no-choice high-fat diets affect striatal dopamine D2/3 receptor availability, caloric intake, and adiposity. Obesity 20, 1738–1740. doi: 10.1038/oby.2012.17
van de Giessen, E., la Fleur, S. E., Eggels, L., de Bruin, K., van den Brink, W., and Booij, J. (2013). High fat/carbohydrate ratio but not total energy intake induces lower striatal dopamine D2/3 receptor availability in diet-induced obesity. Int. J. Obes. 37, 754–757. doi: 10.1038/ijo.2012.128
van Houten, M., Posner, B. I., Kopriwa, B. M., and Brawer, J. R. (1979). Insulin-binding sites in the rat brain: in vivo localization to the circumventricular organs by quantitative radioautography. Endocrinology 105, 666–673. doi: 10.1210/endo-105-3-666
Volkow, N. D., Wang, G. J., Telang, F., Fowler, J. S., Goldstein, R. Z., Alia-Klein, N., et al. (2009). Inverse association between BMI and prefrontal metabolic activity in healthy adults. Obesity 17, 60–65. doi: 10.1038/oby.2008.469
von Rhein, D., Beckmann, C. F., Franke, B., Oosterlaan, J., Heslenfeld, D. J., Hoekstra, P. J., et al. (2017). Network-level assessment of reward-related activation in patients with ADHD and healthy individuals. Hum. Brain Mapp. doi: 10.1002/hbm.23522. [Epub ahead of print].
Wang, G. J., Geliebter, A., Volkow, N. D., Telang, F. W., Logan, J., Jayne, M. C., et al. (2011). Enhanced striatal dopamine release during food stimulation in binge eating disorder. Obesity 19, 1601–1608. doi: 10.1038/oby.2011.27
Wang, G. J., Volkow, N. D., Logan, J., Pappas, N. R., Wong, C. T., Zhu, W., et al. (2001). Brain dopamine and obesity. Lancet 357, 354–357. doi: 10.1016/S0140-6736(00)03643-6
Woods, C. A., Guttman, Z. R., Huang, D., Kolaric, R. A., Rabinowitsch, A. I., Jones, K. T., et al. (2016). Insulin receptor activation in the nucleus accumbens reflects nutritive value of a recently ingested meal. Physiol. Behav. 159, 52–63. doi: 10.1016/j.physbeh.2016.03.013
Yarchoan, M., and Arnold, S. E. (2014). Repurposing diabetes drugs for brain insulin resistance in Alzheimer Disease. Diabetes 63, 2253–2261. doi: 10.2337/db14-0287
Yau, P. L., Kang, E. H., Javier, D. C., and Convit, A. (2014). Preliminary evidence of cognitive and brain abnormalities in uncomplicated adolescent obesity. Obesity 22, 1865–1871. doi: 10.1002/oby.20801
Zhang, H., Hao, Y., Manor, B., Novak, P., Milberg, W., Zhang, J., et al. (2015). Intranasal insulin enhanced resting-state functional connectivity of hippocampal regions in type 2 diabetes. Diabetes 64, 1025–1034. doi: 10.2337/db14-1000
Keywords: dementia, diabetes, obesity, dopamine, cognition, executive function, memory, associative learning
Citation: Small DM (2017) Dopamine Adaptations as a Common Pathway for Neurocognitive Impairment in Diabetes and Obesity: A Neuropsychological Perspective. Front. Neurosci. 11:134. doi: 10.3389/fnins.2017.00134
Received: 28 November 2016; Accepted: 06 March 2017;
Published: 28 March 2017.
Edited by:
Riccarda Granata, University of Turin, ItalyReviewed by:
Emily E. Noble, University of Southern California, USAJacques Epelbaum, Institut National de la Santé et de la Recherche Médicale, France
Copyright © 2017 Small. This is an open-access article distributed under the terms of the Creative Commons Attribution License (CC BY). The use, distribution or reproduction in other forums is permitted, provided the original author(s) or licensor are credited and that the original publication in this journal is cited, in accordance with accepted academic practice. No use, distribution or reproduction is permitted which does not comply with these terms.
*Correspondence: Dana M. Small, dana.small@yale.edu