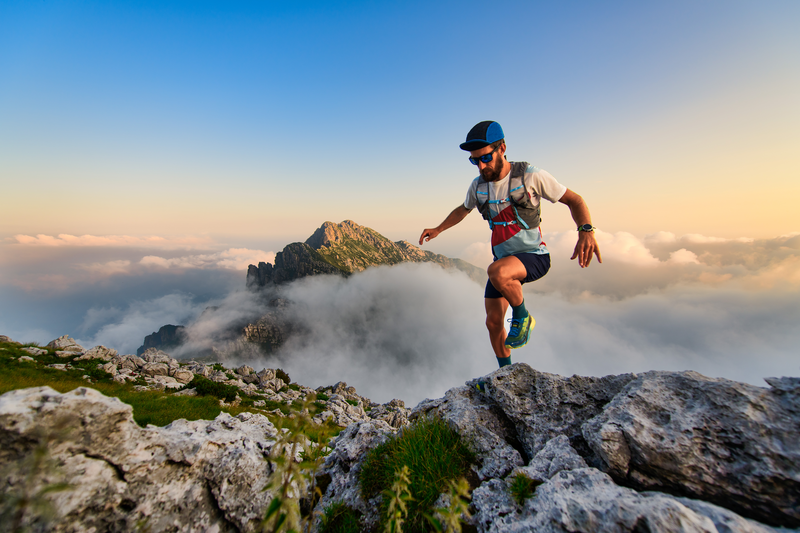
95% of researchers rate our articles as excellent or good
Learn more about the work of our research integrity team to safeguard the quality of each article we publish.
Find out more
PERSPECTIVE article
Front. Neurosci. , 28 June 2016
Sec. Neuropharmacology
Volume 10 - 2016 | https://doi.org/10.3389/fnins.2016.00294
This article is part of the Research Topic Structure-based drug design for diagnosis and treatment of neurological diseases View all 11 articles
Highlights:
• ASS2324 is a hybrid compound resulting from the juxtaposition of donepezil and the propargylamine PF9601N
• ASS2324 is a multi-target directed propargylamine able to bind to all the AChE/BuChE and MAO A/B enzymes
• ASS2324 shows antioxidant, neuroprotective and suitable permeability properties
• ASS2324 restores the scopolamine-induced cognitive impairment to the same extent as donepezil, and is less toxic
• ASS2324 prevents β-amyloid induced aggregation in the cortex of double transgenic mice
• ASS2324 is the most advanced anti-Alzheimer agent for pre-clinical studies that we have identified in our laboratories
The complex nature of Alzheimer's disease (AD) has prompted the design of Multi-Target-Directed Ligands (MTDL) able to bind to diverse biochemical targets involved in the progress and development of the disease. In this context, we have designed a number of MTD propargylamines (MTDP) showing antioxidant, anti-beta-amyloid, anti-inflammatory, as well as cholinesterase and monoamine oxidase (MAO) inhibition capacities. Here, we describe these properties in the MTDL ASS234, our lead-compound ready to enter in pre-clinical studies for AD, as a new multipotent, permeable cholinesterase/monoamine oxidase inhibitor, able to inhibit Aβ-aggregation, and possessing antioxidant and neuroprotective properties.
Alzheimer's disease (AD) is the most common neurodegenerative disease in the elderly (Karlawish, 2011). AD is characterized by progressive neuronal death resulting in severe cognitive impairment. Two distinctive hallmarks of AD are the presence of accumulated beta-amyloid (Aβ) plaques (Hamley, 2012) and hyperphosphorylated tau protein in the form of intracellular neurofibrillary tangles (NFT) (Wang et al., 2013). Although the precise etiology of AD is not yet known, there is a large consensus in describing it as a complex disorder caused by many factors, including loss of cholinergic transmission, protein misfolding and Aβ aggregation, oxidative stress, free radical formation (Rosini et al., 2014), and metal dyshomeostasis (Huang et al., 2004). AD pathology also involves dysfunctional neurotransmittters and synapse loss (Aisa et al., 2010; Villemagne and Chételat, 2016).
In the following sections we describe the currently accepted AD hypotheses, and our recent contribution to the development of new multipotent propargylamines for AD therapy.
Cholinergic neurotransmission modulates both cognitive function and cortical plasticity (Arendt and Bigl, 1986) and plays a significant role in the control of cerebral blood flow (Biesold et al., 1989), cortical activity (Détári et al., 1999), learning and memory (Deutsch, 1971). The first physiological evidence of the involvement of the cholinergic system in AD pathology was a reduction of the neurotransmitter acetylcholine (ACh), which constitutes the basis of the cholinergic hypothesis of AD (Deutsch, 1971), used to discover the first anti-AD agents. Acetylcholinesterase (AChE) is expressed in cholinergic neurons, its primary function being the rapid breakdown of ACh during the cholinergic neurotransmission. In addition to rapid breakdown by AChE, ACh can also be metabolized by butyrylcholinesterase (BuChE) (Mesulam et al., 2002) but with different kinetic behavior. Whereas AChE pre-dominates in neurons and exhibits high affinity for ACh, BuChE is present in endothelia, glia and neuronal cells with low affinity for ACh and high KM values (Soreq and Seidman, 2001).
The amyloid hypothesis postulates that neurodegeneration in AD is caused by abnormal accumulation of Aβ plaques in various areas of the brain (Evin and Weidemann, 2002). The Aβ senile plaques contain Aβ peptides with 39–43 amino acid residues, proteolytically derived from the sequential enzymatic action of β- and γ-secretases of transmembrane APP (Coulson et al., 2000). Within plaques, Aβ peptides in β-sheet conformation assemble and polymerise into fibrillar, protofibers and polymorphic oligomers (Selkoe, 1994). In vitro, the Aβ aggregation process is highly susceptible to pH, ionic strength of the solvent, purification process and temperature. Distinct oligomerization and assembly processes between Aβ1−40 and Aβ1−42 have been described (Bitan et al., 2003). While dimers and trimers are the most toxic forms of Aβ1−42, Aβ1−40 reaches equilibrium from monomers to tetramers. Recent findings have shown that soluble oligomeric species were able to disrupt synaptic function (Lambert et al., 1998) and support the belief that soluble dimeric species are highly toxic (Jin et al., 2011). However, direct Aβ-peptide neurotoxicity has been difficult to prove in animal models (Serrano-Pozo et al., 2013). Since the postulation of the amyloid hypothesis, a number, but a number of unsuccessful efforts have been undertaken in clinical research in order to develop novel drugs based on this concept.
Increased production of Reactive Oxygen Species (ROS) have been observed in AD (Praticò, 2008) and, consequently, elevated levels of oxidative markers including damage to proteins, lipids, carbohydrates, and nucleic acids. Antioxidant enzymes were also found to be increased in specific AD brain regions (Sultana et al., 2011). Not surprisingly, the oxidative stress (OS) hypothesis of AD has emerged as a key event in the progress of the disease. In addition, evidence suggests that secretion and deposition of Aβ within the neurons are compensatory measures taken by cells in effort to protect themselves against damage triggered by OS (Hayashi et al., 2007).
Cellular oxidative damage has also been linked to tau hyperphosphorylation and formation of NFTs (Lee et al., 2004). As a consequence, cells succumb to neurodegeneration exhibiting the distinctive cognitive impairment observed in AD patients (Zhu et al., 2007). Altogether, the primary role of OS in AD has been overwhelmingly confirmed, offering the chance to develop specific disease-modifying antioxidant approaches to cure or prevent the development of the disease.
The increased levels of ROS are reflected in a deregulated content of biometals such as iron, copper and zinc in the brain of AD patients. Recent findings point to brain OS as one of the earliest changes in AD pathogenesis that might play a central role in the disease progression (Lee et al., 2010). Redox-active metals are capable of stimulating free radical formation via the Fenton reaction. Biometals have also been shown to mediate Aβ toxicity in AD (Duce et al., 2010). It has been shown that Aβ peptide itself is a strong redox-active metalloprotein able to directly produce hydrogen peroxide and OH− in the presence of copper or iron, which, in turn, are enriched in the amyloid cores of senile plaques (Huang et al., 1999). Also, biometals can interact directly with Aβ peptide enhancing its self-aggregation and oligomerization at low physiological concentrations or at mildly acidic conditions (Huang et al., 1999). Moreover, metals can promote tau hyperphosphorylation and subsequent formation of NFTs inducing its aggregation upon interaction with Aβ (Yamamoto et al., 2002).
To date, only five drugs have ever been approved for AD therapy. Tacrine, rivastigmine, galantamine and donepezil are AChEI, whereas memantine is a NMDA receptor antagonist.
Tacrine, a competitive AChEI and the first drug to be approved for use in AD by the FDA in 1993, was withdrawn from the market in 2013 due to the high incidence of side effects, mostly derived from hepatotoxicity (Qizilbash et al., 1998). Rivastigmine, a non-selective pseudoreversible ChE inhibitor (Bullock and Lane, 2007), has been reported to have less side effects as well as positive benefit after administration to mild-to-moderate AD patients (Birks and Grimley Evans, 2015). Galantamine, a weak competitive reversible AChEI (Greenblatt et al., 1999) is also a potent allosteric modulator of nicotinic acetylcholine receptors—α4β2, α7/5-HT3, α3β4, and α6β4—in certain areas of the brain, and potentiates the effects of orthoesteric agonists (Dajas-Bailador et al., 2003; Akk and Steinbach, 2005). Donepezil is a brain-permeable reversible non-competitive ChE inhibitor approved for use in AD (Birks and Harvey, 2006) and currently the most widely prescribed drug for the treatment of this disease. Donepezil is highly selective for AChE over BuChE activity (405:1) (Nochi et al., 1995). Compared to other approved AChEI, donepezil is similarly effective in ameliorating cognitive and functional decline in AD with comparable safety and tolerability (Doody et al., 2014).
Memantine is a glutamatergic agent, the first and only NMDA receptor antagonist approved by FDA in 2003 for the treatment of moderate-to-severe AD and dementia. Memantine binds to NMDA receptors with a low-micromolar IC50 value, exhibits neuroprotective activities against Aβ toxicity (Hu et al., 2007), tau phosphorylation (Song et al., 2008), neuroinflammation (Willard et al., 2000), and oxidative stress (Figueiredo et al., 2013).
In the face of general neuronal loss, monoamine oxidase (MAO) inhibitors are used to preserve remaining levels of catecholamine neurotransmitters by inhibiting MAO A in neurons or MAO B in serotonergic neurons, glia and astrocytes. Since MAO B activity is increased in AD, MAO B inhibitors may be of potential therapeutic interest both to maintain neurotransmitter levels and to decrease hydrogen peroxide production (Mandel et al., 2005). For example, rasagiline and selegiline are propargylamines that irreversibly inhibit brain MAO B, but also show neuroprotective activities mainly due to their propargyl moiety (Zindo et al., 2015).
The lack of therapeutic effectiveness of the current drugs based on the single-target paradigm (León et al., 2013) for the treatment of AD prompted the search of MTDL, designed by molecular hybridization of different pharmacophoric moieties from well-known bioactive molecules, able to bind to multiple targets associated with AD. As a result, a number of standard natural or synthetic compounds, including donepezil, tacrine or rivastigmine (Samadi et al., 2011), curcumin (Malar and Devi, 2014), berberine (Jiang et al., 2011) or 8-hydroxyquinoline (Gomes et al., 2014) have been used for this purpose.
Based on this background, we have designed several MTD propargylamines (MTDP) for the potential treatment of AD. All these compounds bear the N-benzylpiperidine group present in donepezil and the N-propargylamine motif present in L-deprenyl (used in Parkinson's disease) and in PF9601N (Figure 1), a potent and selective MAO B inhibitor with neuroprotective effect demonstrated in vitro and in vivo using different experimental models (Pérez et al., 1999). The donepezil motif gives inhibition of the cholinesterases as well as the ability to inhibit the aggregation of Aβ via the acetylcholinesterase peripheral site, whereas the propargylamine inhibits MAO enzymes and also gives neuroprotection. Both scaffolds were linked by different heterocyclic ring systems, such as pyridine, indole or 8-hydroxyquinoline, affording diverse MTDP as promising drugs to be used in AD therapy (Figure 1). This strategy led to ASS234 (Figure 1) with the anti-cholinergic activity of donepezil, selective MAO A inhibition and neuroprotective properties (Bolea et al., 2011).
Next, with the aim of searching for improved MTDLs, two series of novel structurally derived compounds from ASS234 as multipotent donepezil-pyridyl and donepezil-indolyl hybrids were designed and pharmacologically assessed. Thus, the donepezil-pyridyl compound JMC1 (Figure 1) was identified as a very potent hAChE inhibitor (IC50 = 1.1 nM) and a moderate hBuChE inhibitor (IC50 = 0.6 μM) with total selectivity toward human MAO B (hMAO B) (Bautista-Aguilera et al., 2014b). The donepezil-indole JMC2 (Figure 1) exhibited the most interesting profile as a potent MAO A inhibitor (IC50 = 5.5 nM) moderately able to inhibit MAO B (IC50 = 150 nM), AChE (IC50 = 190 nM), and BuChE (IC50 = 830 nM) (Bautista-Aguilera et al., 2014a). Moreover, the kinetic analysis showed that JMC2 is a mixed-type AChE inhibitor able to span both the catalytic and peripheral sites (CAS and PAS) of this enzyme, a fact further confirmed by molecular modeling studies. Propargylamines JMC3 (Wang et al., 2014) and JMC4 (Wu et al., 2015) bear a N-benzylpiperidine moiety from donepezil and a 8-hydroxyquinoline group (Figure 1). JMC3 (Figure 1) was further characterized as an irreversible MAO and mixed-type ChE inhibitor in low micromolar range, and, in addition, it strongly complexed Cu (II), Zn (II), and Fe (III) (Wang et al., 2014). From theoretical ADMET analyses, JMC3 exhibited proper drug-likeness properties and good brain penetration suitable for CNS activity. JMC4 (Figure 1) showed similar inhibitory behavior as dual ChE/MAO inhibitor (Wu et al., 2015).
The propargylamine ASS234 (Figure 1), deserves further discussion. ASS234 is a very potent human MAO A and MAO B inhibitor with a IC50 values of 5.44 ± 1.74 and 177 ± 25 nM, respectively, inhibiting also both ChEs (IC50 (human AChE) = 0.81 ± 0.06 μM; IC50 (human BuChE) = 1.82 ± 0.14 μM) (Esteban et al., 2014) (Figure 2). In contrast, the reference compounds had only their single expected activity: donepezil was ineffective at inhibiting MAO activities, and PF9601N, while potently and selectively inhibiting MAO B displayed no interaction with the ChEs (Bolea et al., 2011). To sum up, ASS234 combines the best properties of donepezil and PF9601N, simultaneously inhibiting ChE to boost cholinergic transmission and MAO to raise catecholamine levels (Bolea et al., 2013).
Although ASS234 is a reversible inhibitor of both ChEs with micromolar affinity, it is a highly potent irreversible MAO A inhibitor, similar to clorgyline. Although the initial reversible binding parameter (Ki value of 0.4 μM) indicated that ASS234 has a lower affinity for MAO A than clorgyline (Ki value of 0.02 μM), and this was also reflected in the higher KI for the irreversible reaction, the full inactivation of MAO A was rapid. The crystal structure of hMAO B after inactivation by ASS234 highlighted the formation of a covalent adduct with the flavin N5 atom which, based on the spectral changes, occurs also with the MAO A cofactor (Esteban et al., 2014). Although the N-benzylpiperidine moiety is not fully visible in the electron density in the crystal structure at 1.8 Å resolution, the mass determinations demonstrate that ASS234 binds as the intact molecule to the MAO B active site, which rules out the possibility that the inhibitor may undergo degradation in the cellular context (Esteban et al., 2014).
Next, the presumed therapeutic potential of ASS234 was evaluated following its administration to a rat model of vascular dementia based on the permanent bilateral occlusion of the common carotid arteries with experimental vascular dementia to determine its impact on brain neurotransmitter systems. In this rat model, the administration of ASS234 for 5 days resulted in a potent and selective inhibition of MAO A activity in brain as well as a concurrent increase in concentrations of serotonin and the catecholamines, dopamine and noradrenaline (Stasiak et al., 2014). All these findings allow us to conclude that ASS234 is able to bind to multiple targets identifying it as an interesting MTDL molecule to be considered for therapeutic development against AD.
The mechanism by which ASS234 plays a neuroprotective role in AD pathology remains unclear. Recent evidence suggests that the Wingless-Type MMTV Integration Site (Wnt) signaling pathway is important in neuroprotection (Toledo et al., 2008), so we investigated whether ASS234 activated the Wnt signaling pathway (del Pino et al., 2014). Total RNA was extracted from SH-SY5Y cells incubated with ASS234 (5 μM) for 24 h and gene expression evaluated for some members of the Wnt1 class signal (Wnt1, Wnt2b, Wnt3a) which represent the “canonical” Wnt/β-catenin pathway, and for some members of the Wnt5a class signal (Wnt6, Wnt5a) which represent the “non-canonical” Wnt/PCP and Wnt/Ca2+ pathways. In ASS234-treated cells, gene expression of Wnt2b, Wnt5a, and Wnt6 was significantly increased. Ingenuity pathways analysis (IPA) identified a number of downstream genes regulated by the Wnt canonical pathways. One of these genes, PPARδ, a key gene related to neuroprotective effects against AD, was significantly increased by ASS234 treatment. From these results, we concluded that ASS234 induced canonical and non-canonical Wnt pathways, which presents another possible mechanism through which this compound can mediate its protective action. Knowing that the activation of Wnt signaling rescues memory loss and improves synaptic dysfunction in transgenic mice model of AD amyloid pathology, these findings indicate that ASS234 could be a novel promising drug for AD therapy.
In order to ascertain the suitability of MTDL ASS234 for pre-clinical studies, the obvious, preliminary and necessary “proof of concept” was assessed. First of all, we investigated the effect of a single-dose of ASS234 (0.62 mg/Kg) on cognition using the scopolamine test. Not surprisingly, scopolamine significantly decreased the exploratory preference for a novel object in the retention trial. Scopolamine-induced cognitive deficit is assessed as a decreased recognition index (RI) in comparison with non-treated and vehicle control groups. After a single dose of ASS234, a significant increase of the RI was observed, indicating reversal of memory impairment induced by scopolamine. Thus, the scopolamine-induced amnesia was reversed by concomitant administration of ASS234 (0.12 mM/kg) which in fact significantly improved the cognitive performance about 13.1%, suggesting that ASS234 exerts its therapeutic effect by enhancing natural memory processes.
Some preliminary studies have been carried out to assess the amyloid plaque burden and gliosis in the cortex of the ASS234 treated group of the transgenic AD model, APPswe/PS1ΔE9 tg mice. Daily administration of ASS234 for 16 weeks at a dose of (0.62 mg/Kg) resulted in apparent reduction in the number of neuritic plaques in the cerebral cortex and hippocampus in comparison to vehicle treated mice. Cortical plaque deposition was significantly decreased in tg mice given the ASS234 treatment compared to tg controls. As in the cortex, the Aβ plaque load in tg mice treated with ASS234 also decreased in the hippocampus, although statistical significance was not reached. These findings indicate that ASS234 has a greater effect upon plaque load in the cerebral cortex than in the hippocampus of APPswe/PS1ΔE9 tg mice.
Next, since microgliosis and astrocytosis, indicative of neuroinflammation, are prominent aspects of this AD mouse model, we proceed to identify the effect of ASS234 on neuroinflammation through the evaluation of the immunohistochemical distribution of the astrocyte marker protein GFAP and of the microglia/macrophage-specific protein iba-1. Significantly decreased GFAP and iba-1 immunostainings were observed in the cortex of the ASS234 treated tg mice compared with that of controls, suggesting a beneficial effect of ASS234 on neuroinflammation. All procedures with animals were carried out in accordance with European Communities Council Directive (2010/63/UE) on animal experiments under a protocol approved by the Animal Welfare Committee of the Cajal Institute (CSIC, Madrid, Spain) and by the Institutional Animal Ethics Committee of the Spain Council for Scientific Research (CSIC), adhering to the recommendations of the European Council and Spanish Department of Health for Laboratory Animals (R.D. 53/2013). A special effort was made to reduce the number of animals used in the study, and the number of animals assigned to each group was to be kept to a minimum necessary to achieve enough significance.
The promising results described above led us to assess the hepatotoxicity and metabolism of compound ASS234. Preliminary toxicity studies of ASS234 along with donepezil and tacrine were performed in parallel in the human cell line HepG2. The results showed that all three compounds reduced cell viability in a concentration-dependent manner, but at very high concentrations (100 and 300 μM) ASS234 exhibited lesser toxicity than the reference compounds, donepezil and tacrine.
The results presented in this review strongly reinforce the suitability of MTDLs as an appropriate pharmacological approach to be used in AD therapy. Amongst all the compounds tested, MTDL ASS234 particularly has emerged as an interesting lead compound for the design of novel MTDL with a good MAO/AChE inhibitory potency, a significant activity against amyloid aggregation, neuroprotective and anti-apoptotic properties, as well as potent antioxidant capacities, so may have a potential disease-modifying role in the treatment of AD. Given the strong correlation of neuroinflammation with amyloid burden, our results showing that ASS234 considerably reduces both amyloid burden and inflammation in the cerebral cortex of treated tg mice underline the beneficial action of ASS234 in slowing the progression of AD.
From the safety point of view, the affinity of compound ASS234 for MAO A is of concern due to well known “cheese effect,” which occurs when tyramine enters the circulation and potentiates sympathetic cardiovascular activity by releasing noradrenaline, and should be considered in future developments of the molecule by analyzing tyramine potentiation and the ASS234 MAO selectivity in the brain. In sum, ASS234 has clearly overcome the “proof of concept,” and remains our most advanced anti-Alzheimer agent for pre-clinical studies targeted to find a new therapy for this devastating disease.
JM and MU wrote the manuscript. GE, IB, RR, AR, RM, MC, and LI corrected the manuscript.
The authors declare that the research was conducted in the absence of any commercial or financial relationships that could be construed as a potential conflict of interest.
MU and JM thank MINECO (Spain) for support (Grant SAF2012-33304; SAF2015-65586-R). RR, MU, GE, and JM thank EU (COST Action 1103) for support.
AD, Alzheimer's Disease; AChE, acetylcholinesterase; MAO, monoamine oxidase; MTDL, multi-target directed ligand.
Aisa, B., Gil-Bea, F. J., Solas, M., García-Alloza, M., Chen, C. P., Lai, M. K., et al. (2010). Altered NCAM expression associated with the cholinergic system in Alzheimer's disease. J. Alzheimer's Dis. 20, 659–668. doi: 10.3233/JAD-2010-1398
Akk, G., and Steinbach, J. H. (2005). Galantamine activates muscle-type nicotinic acetylcholine receptors without binding to the acetylcholine-binding site. J. Neurosci. 25, 1992–2001. doi: 10.1523/JNEUROSCI.4985-04.2005
Arendt, T., and Bigl, V. (1986). Alzheimer plaques and cortical cholinergic innervation. Neuroscience 17, 277–279. doi: 10.1016/0306-4522(86)90243-5
Bautista-Aguilera, O. M., Esteban, G., Bolea, I., Nikolic, K., Agbaba, D., Moraleda, I., et al. (2014a). Design, synthesis, pharmacological evaluation, QSAR analysis, molecular modeling and ADMET of novel donepezil-indolyl hybrids as multipotent cholinesterase/monoamine oxidase inhibitors for the potential treatment of Alzheimer's disease. Eur. J. Med. Chem. 75, 82–95. doi: 10.1016/j.ejmech.2013.12.028
Bautista-Aguilera, O. M., Esteban, G., Chioua, M., Nikolic, K., Agbaba, D., Moraleda, I., et al. (2014b). Multipotent cholinesterase/monoamine oxidase inhibitors for the treatment of Alzheimer's disease: design, synthesis, biochemical evaluation, ADMET, molecular modeling, and QSAR analysis of novel donepezil-pyridyl hybrids. Drug Des. Devel. Ther. 8, 1893–1910. doi: 10.2147/DDDT.S69258
Biesold, D., Inanami, O., Sato, A., and Sato, Y. (1989). Stimulation of the nucleus basalis of Meynert increases cerebral cortical blood flow in rats. Neurosci. Lett. 98, 39–44. doi: 10.1016/0304-3940(89)90370-4
Birks, J., and Harvey, R. J. (2006). Donepezil for dementia due to Alzheimer's disease. Cochrane Database Syst. Rev. CD001190. doi: 10.1002/14651858.CD001190.pub2
Birks, J. S., and Grimley Evans, J. (2015). Rivastigmine for Alzheimer's disease. Cochrane Database Syst. Rev. 4:CD001191. doi: 10.1002/14651858.CD001191.pub3
Bitan, G., Kirkitadze, M. D., Lomakin, A., Vollers, S. S., Benedek, G. B., and Teplow, D. B. (2003). Amyloid beta -protein (Abeta) assembly: abeta 40 and Abeta 42 oligomerize through distinct pathways. Proc. Natl. Acad. Sci. U.S.A. 100, 330–335. doi: 10.1073/pnas.222681699
Bolea, I., Gella, A., Monjas, L., Pérez, C., Rodríguez-Franco, M. I., Marco-Contelles, J., et al. (2013). Multipotent, permeable drug ASS234 inhibits Abeta aggregation, possesses antioxidant properties and protects from Abeta-induced apoptosis in vitro. Curr. Alzheimer Res. 10, 797–808. doi: 10.2174/15672050113109990151
Bolea, I., Juárez-Jimenez, J., de los Ríos, C., Chioua, M., Pouplana, R., Luque, F. J., et al. (2011). Synthesis, biological evaluation, and molecular modeling of donepezil and N-[(5-(benzyloxy)-1-methyl-1H-indol-2-yl)methyl]-N-methylprop-2-yn-1-amine hybrids as new multipotent cholinesterase/monoamine oxidase inhibitors for the treatment of Alzheimer's disease. J. Med. Chem. 54, 8251–8270. doi: 10.1021/jm200853t
Bullock, R., and Lane, R. (2007). Executive dyscontrol in dementia, with emphasis on subcortical pathology and the role of butyrylcholinesterase. Curr. Alzheimer Res. 4, 277–293. doi: 10.2174/156720507781077313
Coulson, E. J., Paliga, K., Beyreuther, K., and Masters, C. L. (2000). What the evolution of the amyloid protein precursor supergene family tells us about its function. Neurochem. Int. 36, 175–184. doi: 10.1016/S0197-0186(99)00125-4
Dajas-Bailador, F. A., Heimala, K., and Wonnacott, S. (2003). The allosteric potentiation of nicotinic acetylcholine receptors by galantamine is transduced into cellular responses in neurons: Ca2+ signals and neurotransmitter release. Mol. Pharmacol. 64, 1217–1226. doi: 10.1124/mol.64.5.1217
del Pino, J., Ramos, E., Aguilera, O. M., Marco-Contelles, J., and Romero, A. (2014). Wnt signaling pathway, a potential target for Alzheimer's disease treatment, is activated by a novel multitarget compound ASS234. CNS Neurosci. Ther. 20, 568–570. doi: 10.1111/cns.12269
Détári, L., Rasmusson, D. D., and Semba, K. (1999). The role of basal forebrain neurons in tonic and phasic activation of the cerebral cortex. Prog. Neurobiol. 58, 249–277. doi: 10.1016/S0301-0082(98)00084-7
Deutsch, J. A. (1971). The cholinergic synapse and the site of memory. Science 174, 788–794. doi: 10.1126/science.174.4011.788
Doody, R. S., Thomas, R. G., Farlow, M., Iwatsubo, T., Vellas, B., Joffe, S., et al. (2014). Phase 3 trials of solanezumab for mild-to-moderate Alzheimer's disease. N. Engl. J. Med. 370, 311–321. doi: 10.1056/NEJMoa1312889
Duce, J. A., Tsatsanis, A., Cater, M. A., James, S. A., Robb, E., Wikhe, K., et al. (2010). Iron-export ferroxidase activity of beta-amyloid precursor protein is inhibited by zinc in Alzheimer's disease. Cell 142, 857–867. doi: 10.1016/j.cell.2010.08.014
Esteban, G., Allan, J., Samadi, A., Mattevi, A., Unzeta, M., Marco-Contelles, J., et al. (2014). Kinetic and structural analysis of the irreversible inhibition of human monoamine oxidases by ASS234, a multi-target compound designed for use in Alzheimer's disease. Biochim. Biophys. Acta 1844, 1104–1110. doi: 10.1016/j.bbapap.2014.03.006
Evin, G., and Weidemann, A. (2002). Biogenesis and metabolism of Alzheimer's disease Abeta amyloid peptides. Peptides 23, 1285–1297. doi: 10.1016/S0196-9781(02)00063-3
Figueiredo, C. P., Clarke, J. R., Ledo, J. H., Ribeiro, F. C., Costa, C. V., Melo, H. M., et al. (2013). Memantine rescues transient cognitive impairment caused by high-molecular-weight abeta oligomers but not the persistent impairment induced by low-molecular-weight oligomers. J. Neurosci. 33, 9626–9634. doi: 10.1523/JNEUROSCI.0482-13.2013
Gomes, L. M., Vieira, R. P., Jones, M. R., Wang, M. C., Dyrager, C., Souza-Fagundes, E. M., et al. (2014). 8-Hydroxyquinoline Schiff-base compounds as antioxidants and modulators of copper-mediated Abeta peptide aggregation. J. Inorg. Biochem. 139, 106–116. doi: 10.1016/j.jinorgbio.2014.04.011
Greenblatt, H. M., Kryger, G., Lewis, T., Silman, I., and Sussman, J. L. (1999). Structure of acetylcholinesterase complexed with (-)-galanthamine at 2.3 A resolution. FEBS Lett. 463, 321–326. doi: 10.1016/S0014-5793(99)01637-3
Hamley, I. W. (2012). The Amyloid beta peptide: a chemist's perspective. Role in Alzheimer's and fibrillization. Chem. Rev. 112, 5147–5192. doi: 10.1021/cr3000994
Hayashi, T., Shishido, N., Nakayama, K., Nunomura, A., Smith, M. A., Perry, G., et al. (2007). Lipid peroxidation and 4-hydroxy-2-nonenal formation by copper ion bound to amyloid-beta peptide. Free Radic. Biol. Med. 43, 1552–1559. doi: 10.1016/j.freeradbiomed.2007.08.013
Hu, M., Schurdak, M. E., Puttfarcken, P. S., El Kouhen, R., Gopalakrishnan, M., and Li, J. (2007). High content screen microscopy analysis of A beta 1-42-induced neurite outgrowth reduction in rat primary cortical neurons: neuroprotective effects of alpha 7 neuronal nicotinic acetylcholine receptor ligands. Brain Res. 1151, 227–235. doi: 10.1016/j.brainres.2007.03.051
Huang, X., Cuajungco, M. P., Atwood, C. S., Hartshorn, M. A., Tyndall, J. D., Hanson, G. R., et al. (1999). Cu(II) potentiation of alzheimer abeta neurotoxicity. Correlation with cell-free hydrogen peroxide production and metal reduction. J. Biol. Chem. 274, 37111–37116. doi: 10.1074/jbc.274.52.37111
Huang, X., Moir, R. D., Tanzi, R. E., Bush, A. I., and Rogers, J. T. (2004). Redox-active metals, oxidative stress, and Alzheimer's disease pathology. Ann. N.Y. Acad. Sci. 1012, 153–163. doi: 10.1196/annals.1306.012
Jiang, H., Wang, X., Huang, L., Luo, Z., Su, T., Ding, K., et al. (2011). Benzenediol-berberine hybrids: multifunctional agents for Alzheimer's disease. Bioorg. Med. Chem. 19, 7228–7235. doi: 10.1016/j.bmc.2011.09.040
Jin, M., Shepardson, N., Yang, T., Chen, G., Walsh, D., and Selkoe, D. J. (2011). Soluble amyloid beta-protein dimers isolated from Alzheimer cortex directly induce Tau hyperphosphorylation and neuritic degeneration. Proc. Natl. Acad. Sci. U.S.A. 108, 5819–5824. doi: 10.1073/pnas.1017033108
Karlawish, J. (2011). Addressing the ethical, policy, and social challenges of preclinical Alzheimer disease. Neurology 77, 1487–1493. doi: 10.1212/WNL.0b013e318232ac1a
Lambert, M. P., Barlow, A. K., Chromy, B. A., Edwards, C., Freed, R., Liosatos, M., et al. (1998). Diffusible, nonfibrillar ligands derived from Abeta1-42 are potent central nervous system neurotoxins. Proc. Natl. Acad. Sci. U.S.A. 95, 6448–6453. doi: 10.1073/pnas.95.11.6448
Lee, H. P., Zhu, X., Casadesus, G., Castellani, R. J., Nunomura, A., Smith, M. A., et al. (2010). Antioxidant approaches for the treatment of Alzheimer's disease. Expert Rev. Neurother. 10, 1201–1208. doi: 10.1586/ern.10.74
Lee, Y. J., Jeong, S. Y., Karbowski, M., Smith, C. L., and Youle, R. J. (2004). Roles of the mammalian mitochondrial fission and fusion mediators Fis1, Drp1, and Opa1 in apoptosis. Mol. Biol. Cell 15, 5001–5011. doi: 10.1091/mbc.E04-04-0294
León, R., García, A. G., and Marco-Contelles, J. (2013). Recent advances in the multitarget-directed ligands approach for the treatment of Alzheimer's disease. Med. Res. Rev. 33, 139–189. doi: 10.1002/med.20248
Malar, D. S., and Devi, K. P. (2014). Dietary polyphenols for treatment of Alzheimer's disease-future research and development. Curr. Pharm. Biotechnol. 15, 330–342. doi: 10.2174/1389201015666140813122703
Mandel, S., Weinreb, O., Amit, T., and Youdim, M. B. H. (2005). Mechanism of neuroprotective action of the anti-Parkinson drug rasagiline and its derivatives. Brain Res. Rev. 48, 379–387. doi: 10.1016/j.brainresrev.2004.12.027
Mesulam, M., Guillozet, A., Shaw, P., and Quinn, B. (2002). Widely spread butyrylcholinesterase can hydrolyze acetylcholine in the normal and Alzheimer brain. Neurobiol. Dis. 9, 88–93. doi: 10.1006/nbdi.2001.0462
Nochi, S., Asakawa, N., and Sato, T. (1995). Kinetic study on the inhibition of acetylcholinesterase by 1-benzyl-4-[(5,6-dimethoxy-1-indanon)-2-yl]methylpiperidine hydrochloride (E2020). Biol. Pharm. Bull. 18, 1145–1147. doi: 10.1248/bpb.18.1145
Pérez, V., Marco, J. L., Fernández-Álvarez, E., and Unzeta, M. (1999). Relevance of benzyloxy group in 2-indolyl methylamines in the selective MAO-B inhibition. Br. J. Pharmacol. 127, 869–876. doi: 10.1038/sj.bjp.0702600
Praticò, D. (2008). Evidence of oxidative stress in Alzheimer's disease brain and antioxidant therapy: lights and shadows. Ann. N.Y. Acad. Sci. 1147, 70–78. doi: 10.1196/annals.1427.010
Qizilbash, N., Whitehead, A., Higgins, J., Wilcock, G., Schneider, L., and Farlow, M. (1998). Cholinesterase inhibition for Alzheimer disease: a meta-analysis of the tacrine trials. Dementia Trialists' Collaboration. JAMA 280, 1777–1782. doi: 10.1001/jama.280.20.1777
Rosini, M., Simoni, E., Milelli, A., Minarini, A., and Melchiorre, C. (2014). Oxidative stress in Alzheimer's disease: are we connecting the dots? J. Med. Chem. 57, 2821–2831. doi: 10.1021/jm400970m
Samadi, A., Valderas, C., de los Ríos, C., Bastida, A., Chioua, M., González-Lafuente, L., et al. (2011). Cholinergic and neuroprotective drugs for the treatment of Alzheimer and neuronal vascular diseases. ISynthesis, I., biological assessment, and molecular modelling of new tacrine analogues from highly substituted 2-aminopyridine-3-carbonitriles. Bioorg. Med. Chem. 19, 122–133. doi: 10.1016/j.bmc.2010.11.040
Selkoe, D. J. (1994). Alzheimer's disease: a central role for amyloid. J. Neuropathol. Exp. Neurol. 53, 438–447. doi: 10.1097/00005072-199409000-00003
Serrano-Pozo, A., Qian, J., Monsell, S. E., Frosch, M. P., Betensky, R. A., and Hyman, B. T. (2013). Examination of the clinicopathologic continuum of Alzheimer disease in the autopsy cohort of the National Alzheimer Coordinating Center. J. Neuropathol. Exp. Neurol. 72, 1182–1192. doi: 10.1097/NEN.0000000000000016
Song, M. S., Rauw, G., Baker, G. B., and Kar, S. (2008). Memantine protects rat cortical cultured neurons against beta-amyloid-induced toxicity by attenuating tau phosphorylation. Eur. J. Neurosci. 28, 1989–2002. doi: 10.1111/j.1460-9568.2008.06498.x
Soreq, H., and Seidman, S. (2001). Acetylcholinesterase–new roles for an old actor. Nat. Rev. Neurosci. 2, 294–302. doi: 10.1038/35067589
Stasiak, A., Mussur, M., Unzeta, M., Samadi, A., Marco-Contelles, J. L., and Fogel, W. A. (2014). Effects of novel monoamine oxidases and cholinesterases targeting compounds on brain neurotransmitters and behavior in rat model of vascular dementia. Curr. Pharm. Des. 20, 161–171. doi: 10.2174/13816128113199990026
Sultana, R., Mecocci, P., Mangialasche, F., Cecchetti, R., Baglioni, M., and Butterfield, D. A. (2011). Increased protein and lipid oxidative damage in mitochondria isolated from lymphocytes from patients with Alzheimer's disease: insights into the role of oxidative stress in Alzheimer's disease and initial investigations into a potential biomarker for this dementing disorder. J. Alzheimers Dis. 24, 77–84. doi: 10.3233/JAD-2011-101425
Toledo, E. M., Colombres, M., and Inestrosa, N. C. (2008). Wnt signaling in neuroprotection and stem cell differentiation. Prog. Neurobiol. 86, 281–296. doi: 10.1016/j.pneurobio.2008.08.001
Villemagne, V. L., and Chételat, G. (2016). Neuroimaging biomarkers in Alzheimer's disease and other dementias. Ageing Res. Rev. doi: 10.1016/j.arr.2016.01.004. [Epub ahead of print].
Wang, J.-Z., Xia, Y.-Y., Grundke-Iqbal, I., and Iqbal, K. (2013). Abnormal hyperphosphorylation of tau: sites, regulation, and molecular mechanism of neurofibrillary degeneration. J. Alzheimer's Dis. 33, 123–139. doi: 10.3233/JAD-2012-129031
Wang, L., Esteban, G., Ojima, M., Bautista-Aguilera, O. M., Inokuchi, T., Moraleda, I., et al. (2014). Donepezil + propargylamine + 8-hydroxyquinoline hybrids as new multifunctional metal-chelators, ChE and MAO inhibitors for the potential treatment of Alzheimer's disease. Eur. J. Med. Chem. 80, 543–561. doi: 10.1016/j.ejmech.2014.04.078
Willard, L. B., Hauss-Wegrzyniak, B., Danysz, W., and Wenk, G. L. (2000). The cytotoxicity of chronic neuroinflammation upon basal forebrain cholinergic neurons of rats can be attenuated by glutamatergic antagonism or cyclooxygenase-2 inhibition. Exp. Brain Res. 134, 58–65. doi: 10.1007/s002210000446
Wu, M. Y., Esteban, G., Brogi, S., Shionoya, M., Wang, L., Campiani, G., et al. (2015). Donepezil-like multifunctional agents: design, synthesis, molecular modeling and biological evaluation. Eur. J. Med. Chem. doi: 10.1016/j.ejmech.2015.10.001. [Epub ahead of print].
Yamamoto, A., Shin, R. W., Hasegawa, K., Naiki, H., Sato, H., Yoshimasu, F., et al. (2002). Iron (III) induces aggregation of hyperphosphorylated tau and its reduction to iron (II) reverses the aggregation: implications in the formation of neurofibrillary tangles of Alzheimer's disease. J. Neurochem. 82, 1137–1147. doi: 10.1046/j.1471-4159.2002.t01-1-01061.x
Zhu, X., Lee, H. G., Perry, G., and Smith, M. A. (2007). Alzheimer disease, the two-hit hypothesis: an update. Biochim. Biophys. Acta 1772, 494–502. doi: 10.1016/j.bbadis.2006.10.014
Keywords: multi-target directed ligands, Alzheimer's disease, monoamine oxidases, cholinesterases, drugs
Citation: Marco-Contelles J, Unzeta M, Bolea I, Esteban G, Ramsay RR, Romero A, Martínez-Murillo R, Carreiras MC and Ismaili L (2016) ASS234, As a New Multi-Target Directed Propargylamine for Alzheimer's Disease Therapy. Front. Neurosci. 10:294. doi: 10.3389/fnins.2016.00294
Received: 18 March 2016; Accepted: 10 June 2016;
Published: 28 June 2016.
Edited by:
Andrew C. McCreary, Janssen Prevention Center, NetherlandsReviewed by:
Alfredo Meneses, Center for Research and Advanced Studies of the National Polytechnic Institute (CINVESTAV), MexicoCopyright © 2016 Marco-Contelles, Unzeta, Bolea, Esteban, Ramsay, Romero, Martínez-Murillo, Carreiras and Ismaili. This is an open-access article distributed under the terms of the Creative Commons Attribution License (CC BY). The use, distribution or reproduction in other forums is permitted, provided the original author(s) or licensor are credited and that the original publication in this journal is cited, in accordance with accepted academic practice. No use, distribution or reproduction is permitted which does not comply with these terms.
*Correspondence: José Marco-Contelles, aXFvYzIxQGlxb2cuY3NpYy5lcw==
Disclaimer: All claims expressed in this article are solely those of the authors and do not necessarily represent those of their affiliated organizations, or those of the publisher, the editors and the reviewers. Any product that may be evaluated in this article or claim that may be made by its manufacturer is not guaranteed or endorsed by the publisher.
Research integrity at Frontiers
Learn more about the work of our research integrity team to safeguard the quality of each article we publish.