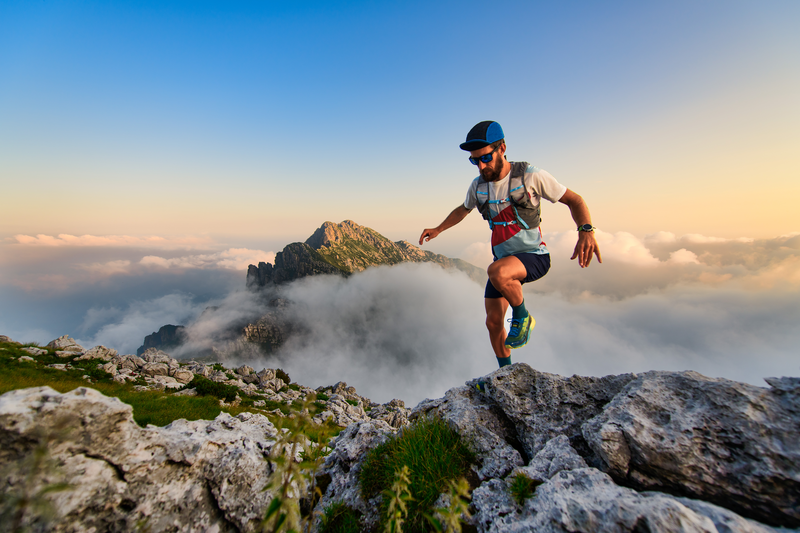
95% of researchers rate our articles as excellent or good
Learn more about the work of our research integrity team to safeguard the quality of each article we publish.
Find out more
REVIEW article
Front. Neurosci. , 10 November 2014
Sec. Neuropharmacology
Volume 8 - 2014 | https://doi.org/10.3389/fnins.2014.00361
This article is part of the Research Topic The Role of Cannabinoid Signaling in Emotional Processing: Implications for Neuropsychiatric Disorders View all 7 articles
Marijuana is the most widely used illicit drug among adolescents and young adults. Unique cognitive, emotional, and social changes occur during this critical period of development from childhood into adulthood. The adolescent brain is in a state of transition and differs from the adult brain with respect to both anatomy (e.g., neuronal connections and morphology) and neurochemistry (e.g., dopamine, GABA, and glutamate). These changes are thought to support the emergence of adult cerebral processes and behaviors. The endocannabinoid system plays an important role in development by acting on synaptic plasticity, neuronal cell proliferation, migration, and differentiation. Delta-9-tetrahydrocanabinol (THC), the principal psychoactive component in marijuana, acts as a partial agonist of the cannabinoid type 1 receptor (CB1R). Thus, over-activation of the endocannabinoid system by chronic exposure to CB1R agonists (e.g., THC, CP-55,940, and WIN55,212-2) during adolescence can dramatically alter brain maturation and cause long-lasting neurobiological changes that ultimately affect the function and behavior of the adult brain. Indeed, emerging evidence from both human and animal studies demonstrates that early-onset marijuana use has long-lasting consequences on cognition; moreover, in humans, this use is associated with a two-fold increase in the risk of developing a psychotic disorder. Here, we review the relationship between cannabinoid exposure during adolescence and the increased risk of neuropsychiatric disorders, focusing on both clinical and animal studies.
According to the Substance Abuse and Mental Health Services Administration, cannabis is the most commonly used drug of abuse among adolescents; in 2010, as many as 20% of all 16-year-olds surveyed reported using cannabis. Adolescence refers to the developmental time period between childhood and adulthood and is generally considered to occur from 12 to 17 years of age (Spear, 2000; Dahl, 2004). This period represents a critical phase in development, characterized by strong neurobehavioral plasticity and many maturation processes in the central nervous system, including myelination, synaptic pruning, volumetric growth, changes in receptor distribution, and programming of neurotrophic levels (Giedd et al., 1999; Spear, 2000; Bartzokis et al., 2001; Andersen, 2003). These changes occur primarily in the prefrontal cortex (PFC) and limbic regions (Chechik et al., 1999; Casey et al., 2000; Toga et al., 2006) and are believed to support the emergence of adult behavior and cognitive functions (Spear, 2000; Andersen, 2003).
Cannabinoid type 1 receptors (CB1R), type 2 receptors (CB2R), and their endogenous ligands (endocannabinoids; eCBs) anandamide (AEA) and 2-arachidonoyl glycerol (2-AG) are present and active during early brain development (Berghuis et al., 2005, 2007; Mulder et al., 2008). The eCB system modulates several neurodevelopmental processes, including the proliferation and differentiation of progenitor cells, neuronal migration, axonal guidance, fasciculation, positioning of cortical interneurons, neurite outgrowth, and morphogenesis (Harkany et al., 2007, 2008). In both adolescent humans and adolescent animals, the eCB system also undergoes functional development and changes (Rodriguez de Fonseca et al., 1993; Mato et al., 2003; Ellgren et al., 2008; Dalton and Zavitsanou, 2010; Eggan et al., 2010). In humans, CB1R expression increases dramatically from infancy to young adulthood in regions such as the frontal cortex, striatum and hippocampus (Mato et al., 2003). While these studies did not directly address specific phases of adolescence, studies in rodents have provided further information on changes in CB1R expression that may be regionally and temporally specific (Ellgren et al., 2008). CB1R expression increases progressively in the shell of the nucleus accumbens (NAcc) during adolescence, but decreases in the core of the NAcc during this same period (Ellgren et al., 2008). In the PFC, the expression of CB1R gradually decreases from mid-adolescence to late-adolescence with the greatest decreases observed in the cingulate, prelimbic, and infralimbic cortices (Ellgren et al., 2008; Heng et al., 2011). Concomitant to developmental changes in the CB1R, levels of AEA and 2-AG vary throughout adolescence in a region- and time-specific manner (Ellgren et al., 2008; Lee et al., 2013). There is an AEA spike in the NAcc during mid-adolescence; while in the PFC AEA progressively increases across adolescence (Ellgren et al., 2008). Conversely, levels of 2-AG are found to dramatically decline in the NAcc and PFC throughout adolescence (Ellgren et al., 2008). These distinct changes in CB1R and eCBs during adolescence suggest that this system plays both neurodevelopmental and morphogenic roles in the maturation of the central nervous system.
Given the importance of the eCB system in neurodevelopment, it is likely that externally induced changes in eCB signaling during adolescence can have profound long-term consequences on functioning in the adult brain. A large body of evidence obtained from both human studies and animal models suggests that exposure to cannabis during adolescence increases the likelihood of developing psychopathology in adulthood (Andreasson et al., 1987; Rubino et al., 2009b; Abush and Akirav, 2012; Renard et al., 2013).
The psychoactive effects of cannabis are due to the action of THC on CB1R receptors, which are present at high density in brain areas that play a role in processing emotional information, learning, and memory (e.g., the amygdala, PFC, and hippocampus) (Herkenham et al., 1990; Marsicano and Lutz, 1999). Perturbations in CB1R signaling in these brain regions have been linked to psychopathology and emotional dysregulation, which are common among neuropsychiatric disorders, including anxiety, depression, and schizophrenia (Degenhardt et al., 2003; Laviolette and Grace, 2006; Ahmad et al., 2013; Sanchez-Blazquez et al., 2014).
The aim of this review is to summarize published animal and human studies regarding the long-term consequences of cannabinoid exposure during adolescence, particularly the effects on cognitive functioning, emotional behavior, and the risk of developing a psychiatric disorder in adulthood. The relevant literature regarding the long-term consequences of cannabinoid exposure during adolescence in both human and animal studies is summarized in Table 1 and in Table 2, respectively.
Table 2. Summary of the effect of adolescent cannabinoid exposure on behavior in various rat strains.
Chronically using cannabis before the age of 17 causes more severe cognitive consequences compared to chronic use later in adolescence. Indeed, converging lines of evidence suggest that chronic use before the age of 17 is associated with deficits in working memory (Schweinsburg et al., 2008, 2010; Becker et al., 2010b), attention (Ehrenreich et al., 1999; Meier et al., 2012; Dougherty et al., 2013), decision-making (Dougherty et al., 2013), visual search (Huestegge et al., 2002), overall and verbal IQ (Pope et al., 1997; Meier et al., 2012), executive functioning (Medina et al., 2009; Becker et al., 2010a; Fontes et al., 2011; Solowij et al., 2012), visuospatial memory (Pope et al., 1997), cognitive inhibition (Fontes et al., 2011), and impulsivity (Dougherty et al., 2013). The magnitude of these deficits is proportional to the frequency, dose, and age at onset of use (Medina et al., 2007a; Schweinsburg et al., 2008, 2010; Becker et al., 2010a; Fontes et al., 2011; Meier et al., 2012).
Although the precise mechanism through which cannabis impairs cognition remains unknown, structural abnormalities have been measured in long-term, heavy cannabis users.
Magnetic resonance imaging (MRI) and positron emission tomography (PET) studies have reported reduced overall cortical gray matter and increased white matter volume in adolescent cannabis users compared to users who began using cannabis in adulthood (Amen and Waugh, 1998; Wilson et al., 2000). Cannabis use during adolescence has also been attributed to increased white matter diffusivity in the PFC compared to later use (Becker et al., 2010a). Functional MRI (fMRI) studies have revealed abnormal activation in the PFC and parietal brain regions of adolescent cannabis users (Jager et al., 2010; Becker et al., 2010a; Gruber et al., 2012). Other groups have also reported decreased cortical thickness in the right superior PFC, bilateral insula, and bilateral superior frontal cortices; increased cortical thickness in the lingual, temporal, inferior parietal, and paracentral regions (Lopez-Larson et al., 2011); and decreased volume in the right medial orbitofrontal cortex (Churchwell et al., 2010) and bilateral hippocampus (Yucel et al., 2008; Ashtari et al., 2011) of adolescent cannabis users without a comorbid psychiatric condition compared to adolescents who do not use cannabis. These structural changes have been associated with increased executive dysfunction (Medina et al., 2009; Churchwell et al., 2010) and verbal memory deficits (Ashtari et al., 2011). In addition, adolescent users of cannabis have reduced cerebral blood flow in the temporal, insular, and PFC regions, and these reductions in blood flow are associated with cognitive deficits (Jacobus et al., 2012). Moreover, converging evidence suggests the presence of abnormal activation patterns in the PFC, limbic region, parietal region, and cerebellum (Schweinsburg et al., 2008; Becker et al., 2010a; Lopez-Larson et al., 2012; Vaidya et al., 2012) in adolescent users of cannabis compared to subjects who do not use cannabis. Interestingly, using diffusion-weighted MRI and connectivity mapping, Zalesky et al. (2012) showed that axonal connectivity was impaired in the right fimbria and the corpus callosum, two structures that contain abundant levels of cannabinoid receptors in the developing brain (Romero et al., 1997; Molina-Holgado et al., 2002). Zalesky and colleagues also demonstrated that the age at which regular cannabis use begins is a key factor in determining the severity of the resulting microstructural changes in white matter (Zalesky et al., 2012).
Taken together, the above studies suggest that chronic cannabis use during adolescence can cause long-term structural changes that are associated with decreased neuronal efficiency in brain regions that play a central role in learning and memory.
Anxiety disorders are the most common complications that arise from chronic heavy cannabis use. Whereas the lifetime prevalence for anxiety disorder on general population is estimated around 6–17% (Kedzior and Laeber, 2014), this prevalence is increased in cannabis users with a prevalence up to 20% (Reilly et al., 1998). A broad and recent meta-analysis (Kedzior and Laeber, 2014) shows that anxiety is significantly positively associated with the consumption (Odds ratio = 1.24) and misuse of cannabis (Odds ratio = 1.68). However, only a few studies have examined the relationship between adolescent cannabis use and long-term anxiety disorders. Cannabis uses during adolescence can double the risk of developing anxiety-related symptoms in adulthood, particularly if the onset of use was initiated before the age of 15. Moreover, girls are more likely than boys to develop these symptoms (Patton et al., 2002; Hayatbakhsh et al., 2007).
A recent study examined the relationship between cannabis use and mental health between the ages of 15 and 29. The authors found that heavy cannabis use during adolescence was associated with an increased risk of developing an anxiety disorder later in life, even if the individual no longer uses cannabis in adulthood (Degenhardt et al., 2013).
In many countries, a growing body of evidence supports an association between cannabis use and depression among young people. Among cannabis users, the prevalence of depressive disorders is 25%; approximately half of these depressive disorders are major depression, and the other half are severe mood disorders (Chabrol et al., 2008). This risk can increase by five-fold, depending on gender (women seem to be more susceptible than men) and the age at which cannabis use begins (Grant and Pickering, 1998; Green and Ritter, 2000).
A study conducted among Australians between 13 and 17 years of age found that adolescents who use cannabis are three times more likely to meet the criteria for depression later in life compared to adolescents who never used cannabis (Rey et al., 2002). Another study found that 30% of adolescents who chronically use cannabis between the ages of 15 and 17 develop depressive symptoms by the age of 21 (Fergusson et al., 2003). Van Laar and colleagues confirmed this observation and also reported that frequent cannabis use increases the risk of developing a depressive disorder (van Laar et al., 2007). In addition, Hayatbakhsh and colleagues demonstrated that adolescents under the age of 15 who frequently use cannabis are more likely to report symptoms of anxiety and depression in early adulthood, particularly when the researchers took into account cumulative exposure to cannabis and potentially significant confounding factors such as maternal smoking and alcohol consumption (Hayatbakhsh et al., 2007). Finally, a longitudinal study that was conducted in young Norwegians and followed over a 13-year period (from their early teens to their late teens) showed a dose-dependent relationship between chronic cannabis consumption and suicidal tendencies (i.e., thoughts and attempts) later in life (Pedersen, 2008). Taken together, these longitudinal studies suggest that early onset and regular use of cannabis increase the risk of depression later in life. However, some researchers suggest that both environmental factors and genetic predisposition play a role in this causal association (Lynskey et al., 2004; Vinod and Hungund, 2006). Indeed, Lynskey and colleagues observed that twins who were discordant for cannabis dependence were more likely to develop suicidal ideation or attempted suicide than their non-cannabis-dependent co-twins. They also reported that twins discordant for early marijuana initiation (before the age of 17) were more likely to attempt suicide than their non-early-cannabis use co-twins (Lynskey et al., 2004). Furthermore, post-mortem studies revealed that the density of CB1R was higher in the PFC of patients with depression who died by suicide than controls (Vinod and Hungund, 2006).
Finally, increased depressive symptoms in adolescent users of cannabis were shown to be associated with smaller global white matter volume (Medina et al., 2007b), suggesting that cannabis use during adolescence may disrupt white matter connections between brain regions that play a role in mood regulation.
These studies put forward that pre-existing genetic factors may predispose an individual to depression, and that these factors may be revealed by early cannabis use. Future studies are clearly needed in order to elucidate the neurobiological mechanisms that underlie the long-lasting effects of cannabis use on the eventual onset of depressive disorders.
The psychotic symptoms that have been associated with the use of cannabis (i.e., the so-called cannabis-induced psychosis) include a “loss of control,” thought disturbances, feelings of unreality, apprehension, fear and paranoia, depersonalization, dysphoria, difficulty concentrating, hallucinations, and other perceptual alterations (Hall and Degenhardt, 2000; Degenhardt and Hall, 2002). The first longitudinal study to demonstrate an association between adolescent cannabis use and schizophrenia in later life was conducted in young healthy Swedish subjects (Andreasson et al., 1987). The authors found that heavy cannabis use at age 18 led to a six-fold increase in the risk of developing schizophrenia 15 years later. In addition, an 11-year longitudinal study of subjects who did not present with pre-existing psychosis found that cannabis use was associated with an increased risk of developing schizophrenic symptoms (Arseneault et al., 2002). This study also revealed that early cannabis consumption (i.e., at age 15) increased the risk of developing schizophrenic symptoms at age 26 by a factor of four compared to cannabis consumption after age 18. According to the authors, 8–13% of the patients in their study might never have developed schizophrenic symptoms had they not used cannabis (Arseneault et al., 2004). This strong association between adolescent cannabis use and psychotic disorders later in life was confirmed by Stefanis and colleagues, who found that both positive and negative psychotic symptoms were more strongly associated with an onset of cannabis use before the age of 16, regardless of the frequency or duration of use (Stefanis et al., 2004).
There is still a debate on whether adolescent cannabis use triggers the onset of schizophrenia in genetically vulnerable individuals or whether individuals with pre-existing vulnerability for psychosis are more likely to use cannabis as a means of self-medication to alleviate some early psychotic symptoms (Dixon et al., 1990; Mueser et al., 1992; Ferdinand et al., 2005; Degenhardt et al., 2007). This proposed self-medication explanation does not account for the association between cannabis use during adolescence and the development of psychosis later in life, as this relationship is observed more often in the absence of psychological distress, and psychological distress does not necessarily predict cannabis use (Stefanis et al., 2004; Henquet et al., 2005).
In addition, there is overwhelming consensus within the literature citing a lack of evidence for the self-medication hypothesis, given that no relationship between early psychotic symptoms and an increased risk of later cannabis use has been reported (Patton et al., 2002; Tournier et al., 2003; Verdoux et al., 2003, 2005).
Yet, only a small minority of cannabis users develop psychotic symptoms. It is therefore likely that both environmental factors and genetic predisposition play a role in this causal association. Consistent with this notion, among patients with schizophrenia, hypersensitivity to the psychotomimetic effects of cannabis is associated with early cannabis exposure and a family history of psychosis (Arendt et al., 2008; Goldberger et al., 2010); moreover, a recent study of a large sample of students found that sensitivity to the psychotomimetic effects of cannabis appears to be an intrinsic feature present since the first exposure to cannabis (Krebs et al., 2014).
Studies in humans support CB1R dysfunction in certain brain regions of schizophrenic patients, specifically in the cortical regions that play a role in cognition and memory (i.e., the anterior and posterior cingulate cortices and the dorsolateral PFC), two functions that are severely compromised in schizophrenia. Indeed, post-mortem analyses revealed that the density of CB1R receptors was higher in the dorsolateral PFC and cingulate cortices (both anterior and posterior) of schizophrenic patients compared to controls, and these changes were apparently independent of recent pre-mortem cannabis use (Dean et al., 2001; Zavitsanou et al., 2004; Newell et al., 2006).
Clinical studies suggest that in addition to altering CB1R function, schizophrenic patients have changes in their eCB levels, particularly AEA. Indeed, AEA levels are higher in the cerebrospinal fluid of non-medicated paranoid schizophrenic patients than in healthy subjects, irrespective of recent cannabis use (Leweke et al., 1999). Interestingly, other lipid molecules, including oleoylethanolamide and palmitoylethanolamide, were not increased in these patients, excluding the likelihood of schizophrenia-related changes in general lipid signaling (Giuffrida et al., 2004).
Treating schizophrenic patients with classic antipsychotics (i.e., dopamine D2 receptor antagonists) lowers AEA levels to normal. Because paranoid schizophrenia is characterized primarily by predominantly positive symptoms due to hyper-dopaminergic neurotransmission (Oades et al., 2002), AEA has been suggested to play an adaptive role, counteracting the dopaminergic abnormalities in schizophrenia, thus reinforcing the existence of dysregulated AEA signaling in schizophrenia. Another study by the same group found that frequent cannabis use down-regulates AEA levels in the cerebrospinal fluid of schizophrenic patients, but not healthy controls (Leweke et al., 2007). These results indicate that frequent cannabis exposure may lead to the down-regulation of AEA signaling (by decreasing AEA biosynthesis and/or increasing AEA degradation) in the central nervous system of schizophrenic patients, but not healthy patients. This down-regulation of AEA might disrupt AEA's control over dopaminergic neurotransmission, thereby precipitating psychosis. Accordingly, alterations in AEA signaling might be an important component of the putative mechanism through which cannabis precipitates psychotic symptoms.
A growing body of data in recent years supports the notion that genetics plays a clear role in the association between early cannabis exposure and an increased risk of developing schizophrenia. Following the first published report of an association between a CNR1 receptor polymorphism and cannabis abuse in schizophrenia (Leroy et al., 2001), a second study of a Japanese cohort found that a polymorphism in the CNR1 gene (which encodes the CB1R receptor) may be associated with an increased risk of developing hebephrenic (i.e., disorganized) schizophrenia (Ujike et al., 2002). This form of schizophrenia is characterized primarily by negative symptoms that resemble the amotivational features commonly observed following chronic cannabis consumption. However, the current state of the literature does not suggest that this polymorphism would be a vulnerability factor for hebephrenic schizophrenia and further studies are needed to confirm CNR1 polymorphisms as a genetic risk for hebephrenic schizophrenia.
A more recent study evaluated interactions between CNR1 polymorphisms, heavy cannabis use, cerebral volume and cognitive function (Ho et al., 2011). Authors compared schizophrenia patients with heavy cannabis use and schizophrenia patients without heavy cannabis use. First, they observed that schizophrenia patients with cannabis abuse had smaller fronto-temporal white matter volumes than patients without heavy cannabis use. In addition, they found that schizophrenia patients with specific CNR1 polymorphisms (specifically rs12720071 SNP G-allele carriers) were more vulnerable to the impact of heavy cannabis use, as they showed greater white matter volume decrease and cognitive impairment than patients without heavy cannabis use. These results are suggestive of gene-environment interactions for conferring phenotypic abnormalities in schizophrenia (Ho et al., 2011).
The relationship between adolescent cannabis use and psychotic symptoms may also be attributed to a functional polymorphism in the catechol-O-methyltransferase (COMT) gene, which encodes an enzyme that degrades catecholamines such as dopamine (DA). The human COMT gene has two allelic variants that code for either a valine or a methionine at codon 158. The functional COMT valine 158 variant catabolizes synaptic DA faster than the COMT methionine 158 variant (Lachman et al., 1996). Caspi and colleagues found that carriers of the COMT valine 158 allele who use cannabis are more likely to exhibit psychotic symptoms and develop a schizophrenic disorder; in contrast, cannabis consumption had no such effect on individuals with two copies of the methionine variant (Caspi et al., 2005). Finally, increased COMT activity may underlie the decrease in synaptic DA levels that contributes to the development of cognitive disorders (Chen et al., 2004).
Polymorphisms in the AKT1 gene may play a role in psychosis induced by early cannabis use. AKT1 is a serine/threonine kinase that helps regulate dopaminergic signaling cascades. Cannabinoids can activate the AKT1 pathway via CB1R receptors. Indeed, Decoster and colleagues recently reported that a genetic variation in the AKT1 gene may mediate cannabis-associated effects on the expression of psychosis via a cannabinoid-regulated AKT1/GSK-3 signaling mechanism that lies downstream of the dopamine D2 receptor (Decoster et al., 2011).
Finally, a recent study of nearly 1200 young healthy students revealed that the psychotomimetic effects at first cannabis use were associated with CNR1 variants but not with COMT or AKT1 variants (Krebs et al., 2014). This finding supports the notion that individual variability in the psychotomimetic effect of cannabis may be attributed to specific genetic backgrounds that influence an individual's first response to cannabis, potentially revealing increased risk of developing psychosis later in life in those individuals (Krebs et al., 2014).
Taken together, these studies confirm that cannabis use—particularly during adolescence—can contribute to the emergence of psychotic disorders in genetically vulnerable individuals, supporting the “two-hit” hypothesis, which posits that both genetics and environmental factors encountered early in life increase the individual's risk of developing a psychiatric disorder (see below).
Animal models are useful tools for investigating the long-term behavioral effects of cannabis exposure during adolescence. To develop a suitable animal model, one must first take into consideration the obvious differences in developmental ontogeny between the animal species and human patients. Adolescence has a broad developmental border in both humans and animals. In rodents, adolescence ranges from postnatal day 28 (PND28) to PND42 (Spear, 2000), and it can be subdivided into specific phases such as early adolescence (beginning at PND28), mid-adolescence (beginning at PND38), and late adolescence (beginning at PND49) (Figure 1).
Figure 1. Schematic overview of the various stages of adolescence in rats. To model the effects of cannabinoid exposure at different developmental stages, rats can be treated chronically throughout the entire adolescent period (from PND28 through PND61) or during specific stages of adolescence, including early adolescence (beginning at PND28), mid-adolescence (beginning at PND38), or late adolescence (beginning at PND49). The long-term effects of adolescent cannabinoid exposure can then be measured in adulthood. PND, postnatal day.
The relevant literature regarding the long-term consequences of cannabinoid exposure during adolescence in rats is summarized in Table 2.
Chronically exposing adolescent rats (of either gender) to CB1R agonists (including THC, WIN55,212-2, and CP55,950) induces short-term memory impairment measured using the object recognition task (Schneider and Koch, 2003; O'Shea et al., 2006; Quinn et al., 2008; Schneider et al., 2008; Llorente-Berzal et al., 2013; Renard et al., 2013; Zamberletti et al., 2014). In contrast, chronic cannabinoid exposure to adult rats has no long-term significant effect on this cognitive function (Schneider and Koch, 2003; Quinn et al., 2008; Renard et al., 2013). However, one study demonstrated that chronic THC treatment in adult mice impairs performance in the object recognition task for 4 days following the last THC injection and these deleterious effects were shown to be prevented by a pre-treatment with Temsirolimus, an mTOR inhibitor, suggesting a role for mTOR in the THC-induced long-lasting memory impairment (Puighermanal et al., 2013).
Similarly, deficits in spatial working memory have been observed in adult rats that were exposed to CB1R agonists during adolescence; these deficits were detected using the radial maze task (Rubino et al., 2009a,b) and the object location task (Abush and Akirav, 2012; Renard et al., 2013). With respect to the potential neurobiological substrates that may underlie this phenomenon, these cognitive deficits have been correlated to reduced expression of several proteins in the hippocampus and in the PFC, including synaptic plasticity proteins (PSD95 and synaptophysin), cytoskeletal and structural proteins related to degenerative and oxidative changes, NMDA receptors, glutamic acid decarboxylase 67 (GAD 67), glial fibrillary acidic proteins (GFAP), and activity-regulated cytoskeletal-associated protein (Arc) (Quinn et al., 2008; Rubino et al., 2009a,b; Llorente-Berzal et al., 2013; Zamberletti et al., 2014). In addition, adult rats that were treated with cannabinoids during adolescence have reduced total dendritic length, arborization, and spine numbers in the dentate gyrus (Rubino et al., 2009b). Interestingly, exposure to cannabinoids during adolescence does not appear to induce long-lasting memory impairments in adult rats (measured using the Morris water maze) of either gender (Cha et al., 2007; Higuera-Matas et al., 2009). Overall, the deleterious cognitive effects of early cannabinoid exposure point to long-lasting impairments in short-term—rather than long-term—information processing. However, further research is needed in order to understand these differential changes in the temporal aspects of memory.
Taken together, these findings obtained from many animal studies support the results obtained from human studies and confirm that adolescence is a highly critical period with long-term downstream effects on cognitive processing.
With respect to anxiety, the results obtained from animal studies are less consistent than cognitive function, although this lack of consistency may be due to the specific developmental period in which cannabinoids are administered and/or the behavioral test used to measure anxiety. Chronic treatment with the cannabinoid agonist CP-55,940 from PND35 to PND45 decreased anxiety levels assessed using the open-field test and the elevated plus maze (Biscaia et al., 2003). However, no changes in plasma corticosterone levels were found in the adult rats, suggesting “normal” function and activity of the hypothalamo-hypophyso-cortico-adrenal axis. In addition, chronic treatment with various CB1R agonists (e.g., THC, CP-55,940, and WIN55,212-2) decreased anxiety levels in adult rats assessed using the social interaction test (O'Shea et al., 2006; Quinn et al., 2008; Zamberletti et al., 2014).
Conversely, treating rats chronically with CP-55,940 from PND28 to PND38 or with THC from PND28 to PND45 led to an increase in anxiety levels at adulthood, measured using the elevated plus maze (Higuera-Matas et al., 2009) or the open-field test, respectively (Llorente-Berzal et al., 2013). Similarly, chronically treating rats with high doses of WIN55,212-2 from PND30 to PND50 induced anxiety-like effects at adulthood, measured using the novelty-suppressed feeding test (Bambico et al., 2010).
Experimental studies revealed that exposing adolescent rats to cannabinoids leads to an immediate dysregulation of emotional processes and induces a depressive-like phenotype later in life. Treating adolescent (PND35–PND45) rats with WIN55,212-2 or THC led to decreased sucrose preference (a measure of anhedonia) in adulthood (Rubino et al., 2008; Bambico et al., 2010). Subjecting adolescent cannabinoid-treats rats to the forced-swim test also revealed a depressive phenotype, and these effects appear to be stronger in female rats (Rubino et al., 2008; Bambico et al., 2010; Zamberletti et al., 2014). These findings suggest that cannabinoid exposure during adolescence may affect the susceptibility to develop a mood disorder later in life, and these animal models appear to recapitulate the gender differences that are well-documented among human patients with depression. Investigating the neurobiological mechanisms that underlie the change in emotion induced by adolescent cannabinoid exposure, Rubino and colleagues found a decrease in CB1R expression in the amygdala, ventral tegmental area, and NAcc, thereby demonstrating a role for CB1R receptors in the regulation of emotional response (Rubino et al., 2008). These changes in CB1R expression were accompanied by changes in the levels of CREB protein in the hippocampus, NAcc, and PFC (Rubino et al., 2008). More recently, Bambico and colleagues reported that treating adolescent rats—but not adult rats—with WIN55,212-2 alters midbrain neuronal firing (Bambico et al., 2010). Specifically, adolescent cannabinoid treatment resulted in hyperactivity of noradrenergic neurons concomitant with hypoactivity of serotonergic neurons (Bambico et al., 2010). Such neuroadaptations are consistent with enhanced depressive-like behavior due to cannabinoid exposure during adolescence.
To date, animal studies regarding psychotic symptoms have focused on disruptions in **prepulse inhibition (PPI). Impaired PPI is widely accepted as an endophenotype of schizophrenia with high translational validity (Braff et al., 2001; Perry et al., 2001).
Chronically treating adolescent rats (PND40–PND65) with the cannabinoid agonist WIN55,212-2 induced long-lasting sensorimotor gating impairment in adulthood (i.e., 85 days after treatment), measured using the PPI test (Schneider and Koch, 2003; Wegener and Koch, 2009); in contrast, chronically treating adult rats did not affect PPI. These deficits were correlated with changes in basal neuronal activity (i.e., c-Fos activity) in the NAcc, amygdala, caudate putamen, and hippocampus (Wegener and Koch, 2009). In contrast, other groups reported no changes in PPI after chronically exposing adolescent rats (PND35–PND45) to THC (Bortolato et al., 2014). This discrepancy may be due to the adolescent period in which the rats were treated and/or the cannabinoid compound that was administered. These differences in results may also reflect differences in the genetic backgrounds of the rats used in these studies (for example, Bortolato and colleagues used Lewis rats, whereas Schneider and colleagues used Wistar rats). Indeed, strain differences have been found to play a critical role in modulating the acoustic startle reflex and sensorimotor gating in rats (Hsieh et al., 2006).
As discussed above, the “two-hit hypothesis” of schizophrenia posits that a prenatal genetic or environmental “first hit” increases the individual's vulnerability to a “second hit” later in life, thereby significantly increasing the risk of developing schizophrenic symptoms (Bayer et al., 1999). To test this hypothesis, COMT-knockout mice (the “first hit”) were exposed to THC during adolescence (the “second hit”); this two-hit approach induced long-lasting impairments in exploration, spatial working memory, and anxiety (O'Tuathaigh et al., 2010), as well as deficits in PPI, habituation to the acoustic startle response, sociability, and social novelty preference (O'Tuathaigh et al., 2012). Exposing adolescent COMT-knockout mice to THC also decreased the size of dopaminergic and GABAergic cells in the ventral tegmental area and PFC, respectively, and increased CB1R expression in the hippocampus (Behan et al., 2012). These results reinforce the notion that an individual's risk of developing schizophrenia may be influenced by interactions between genetic-based changes in DA catabolism and cannabis exposure. These finding also suggest how COMT deletion and adolescent cannabis use may work together to modulate the function of neurotransmitter systems implicated in schizophrenia.
Other studies have also revealed an interesting interaction between cannabis exposure and a mutation in the neuregulin 1 (Nrg1) gene. Mice that are heterozygous for the transmembrane domain Nrg1 mutant (Nrg1 HET mice) appeared to be more sensitive to the behavioral effects of acute THC exposure than their wild-type littermates (Boucher et al., 2007a; Long et al., 2010). Following chronic exposure to THC, Nrg HET mice developed tolerance to cannabinoid-induced hypothermia and locomotor suppression more rapidly than wild-type mice (Boucher et al., 2011). Conversely, wild-type mice (but not Nrg1 HET) mice developed tolerance to cannabinoid-induced anxiety, whereas Nrg1 HET mice showed a persistent anxiogenic response to chronic cannabinoid exposure. Cannabinoid exposure (either acute or chronic) also selectively induced the expression of Fos transcription factors in the lateral septum of Nrg1 HET mice, but not wild-type mice (Boucher et al., 2007b, 2011). In addition, chronically exposing adolescent Nrg1 HET mice to THC exacerbated their hyperlocomotor phenotype and induced differential effects in the density of NMDA, CB1, and 5-HT2A receptors in brain regions that are relevant to schizophrenia, particularly the hippocampus (Long et al., 2013). More recently, these researchers expanded their previous findings, reporting that chronic adolescent exposure to THC induces the differential expression of proteins involved in NMDA receptor trafficking to the synaptic membrane, lipid raft stabilization of synaptic NMDA receptors, and homeostatic responses that dampen excitotoxicity (Spencer et al., 2013). These observations suggest an interaction between the cannabinoid system and Nrg1 signaling and indicate that altered expression of the Nrg1 gene can modulate the behavioral consequences of THC exposure during adolescence.
Similarly, Schneider and Koch (2007) reported that adolescent cannabinoid exposure induces stronger deficits in both short-term memory and social behavior in rats that received a neonatal PFC lesion. In addition, exposure to THC during adolescence exacerbated (i) the short-term memory deficit in rats that were chronically pretreated with PCP (measured using the object recognition test) (Vigano et al., 2009), and (ii) the disruption in PPI in socially isolated rats (Malone and Taylor, 2006).
Taken together, these studies of the interaction between genetic factors and environmental factors help elucidate the putative mechanisms underlying the etiopathogenesis of schizophrenia and may facilitate the discovery of new therapeutic targets.
A considerable body of evidence obtained from both human studies and animal models suggests that cannabis use during adolescence increases the risk of developing a psychiatric disorder in adulthood, including anxiety, depression, and schizophrenia. The psychiatric disorders discussed in this review are multifactorial in origin, and the transition from adolescent cannabis use to subsequent psychiatric illness may also involve both genetic factors and environmental factors. The developmental period of initiation, the frequency, and the duration of cannabis use, as well as any underlying psychiatric pathology, may all play a critical role in the development of a psychiatric disorder. In addition, compelling evidence suggests that some adolescents are more susceptible to the long-term effects of cannabis use than others, and this may be due to differences in genetic vulnerability, including polymorphisms in the genes that play a role in the development of psychiatric diseases. However, whether early cannabis use is related to a pre-existing pathology that is exacerbated by drug use remains an open question.
Disrupting the eCB system during development may affect several neurotransmitter systems. Indeed, CB1R receptors are presynaptic and are expressed primarily in GABAergic interneurons and pyramidal neurons (Marsicano and Lutz, 1999; Manzoni and Bockaert, 2001; Morozov and Freund, 2003). By modulating the release of GABA and glutamate, CB1R receptors help prevent excess neuronal excitation and inhibition (Marsicano et al., 2003; Kano et al., 2009; Katona, 2009). Repeated excessive CB1R stimulation by THC during adolescence may shift the balance of GABAergic inhibitory input on pyramidal neurons. We can therefore speculate that exposure to cannabinoids during adolescence might interfere with the development of neuronal processes in the still-developing adolescent brain, thus leading to changes that ultimately affect brain connectivity, function, and behavior.
Longitudinal studies using neuroimaging and genetic approaches to evaluate adolescents prior to the start of chronic cannabis use are needed. In addition, studies using animal models are needed in order to further investigate the role of the endocannabinoid system in adolescence, as well as the molecular and neurochemical mechanisms that underlie the deleterious effects of cannabinoid exposure during adolescence. Such studies would greatly enhance our understanding of the propensity for adolescent cannabis use to facilitate the development of psychiatric disease later in life.
The authors declare that the research was conducted in the absence of any commercial or financial relationships that could be construed as a potential conflict of interest.
Abush, H., and Akirav, I. (2012). Short- and long-term cognitive effects of chronic cannabinoids administration in late-adolescence rats. PLoS ONE 7:e31731. doi: 10.1371/journal.pone.0031731
Pubmed Abstract | Pubmed Full Text | CrossRef Full Text | Google Scholar
Ahmad, T., Lauzon, N. M., de Jaeger, X., and Laviolette, S. R. (2013). Cannabinoid transmission in the prelimbic cortex bidirectionally controls opiate reward and aversion signaling through dissociable kappa versus mu-opiate receptor dependent mechanisms. J. Neurosci. 33, 15642–15651. doi: 10.1523/JNEUROSCI.1686-13.2013
Pubmed Abstract | Pubmed Full Text | CrossRef Full Text | Google Scholar
Amen, D. G., and Waugh, M. (1998). High resolution brain SPECT imaging of marijuana smokers with AD/HD. J. Psychoactive Drugs 30, 209–214. doi: 10.1080/02791072.1998.10399692
Pubmed Abstract | Pubmed Full Text | CrossRef Full Text | Google Scholar
Andersen, S. L. (2003). Trajectories of brain development: point of vulnerability or window of opportunity? Neurosci. Biobehav. Rev. 27, 3–18. doi: 10.1016/S0149-7634(03)00005-8
Pubmed Abstract | Pubmed Full Text | CrossRef Full Text | Google Scholar
Andreasson, S., Allebeck, P., Engstrom, A., and Rydberg, U. (1987). Cannabis and schizophrenia. A longitudinal study of Swedish conscripts. Lancet 2, 1483–1486. doi: 10.1016/S0140-6736(87)92620-1
Pubmed Abstract | Pubmed Full Text | CrossRef Full Text | Google Scholar
Arendt, M., Mortensen, P. B., Rosenberg, R., Pedersen, C. B., and Waltoft, B. L. (2008). Familial predisposition for psychiatric disorder: comparison of subjects treated for cannabis-induced psychosis and schizophrenia. Arch. Gen. Psychiatry 65, 1269–1274. doi: 10.1001/archpsyc.65.11.1269
Pubmed Abstract | Pubmed Full Text | CrossRef Full Text | Google Scholar
Arseneault, L., Cannon, M., Poulton, R., Murray, R., Caspi, A., and Moffitt, T. E. (2002). Cannabis use in adolescence and risk for adult psychosis: longitudinal prospective study. BMJ 325, 1212–1213. doi: 10.1136/bmj.325.7374.1212
Pubmed Abstract | Pubmed Full Text | CrossRef Full Text | Google Scholar
Arseneault, L., Cannon, M., Witton, J., and Murray, R. M. (2004). Causal association between cannabis and psychosis: examination of the evidence. Br. J. Psychiatry 184, 110–117. doi: 10.1192/bjp.184.2.110
Pubmed Abstract | Pubmed Full Text | CrossRef Full Text | Google Scholar
Ashtari, M., Avants, B., Cyckowski, L., Cervellione, K. L., Roofeh, D., Cook, P., et al. (2011). Medial temporal structures and memory functions in adolescents with heavy cannabis use. J. Psychiatr. Res. 45, 1055–1066. doi: 10.1016/j.jpsychires.2011.01.004
Pubmed Abstract | Pubmed Full Text | CrossRef Full Text | Google Scholar
Bambico, F. R., Nguyen, N. T., Katz, N., and Gobbi, G. (2010). Chronic exposure to cannabinoids during adolescence but not during adulthood impairs emotional behaviour and monoaminergic neurotransmission. Neurobiol. Dis. 37, 641–655. doi: 10.1016/j.nbd.2009.11.020
Bartzokis, G., Beckson, M., Lu, P. H., Nuechterlein, K. H., Edwards, N., and Mintz, J. (2001). Age-related changes in frontal and temporal lobe volumes in men: a magnetic resonance imaging study. Arch. Gen. Psychiatry 58, 461–465. doi: 10.1001/archpsyc.58.5.461
Pubmed Abstract | Pubmed Full Text | CrossRef Full Text | Google Scholar
Bayer, T. A., Falkai, P., and Maier, W. (1999). Genetic and non-genetic vulnerability factors in schizophrenia: the basis of the “two hit hypothesis.” J. Psychiatr. Res. 33, 543–548. doi: 10.1016/S0022-3956(99)00039-4
Becker, B., Wagner, D., Gouzoulis-Mayfrank, E., Spuentrup, E., and Daumann, J. (2010a). Altered parahippocampal functioning in cannabis users is related to the frequency of use. Psychopharmacology (Berl) 209, 361–374. doi: 10.1007/s00213-010-1805-z
Pubmed Abstract | Pubmed Full Text | CrossRef Full Text | Google Scholar
Becker, B., Wagner, D., Gouzoulis-Mayfrank, E., Spuentrup, E., and Daumann, J. (2010b). The impact of early-onset cannabis use on functional brain correlates of working memory. Prog. Neuropsychopharmacol. Biol. Psychiatry 34, 837–845. doi: 10.1016/j.pnpbp.2010.03.032
Pubmed Abstract | Pubmed Full Text | CrossRef Full Text | Google Scholar
Behan, A. T., Hryniewiecka, M., O'Tuathaigh, C. M., Kinsella, A., Cannon, M., Karayiorgou, M., et al. (2012). Chronic adolescent exposure to delta-9-tetrahydrocannabinol in COMT mutant mice: impact on indices of dopaminergic, endocannabinoid and GABAergic pathways. Neuropsychopharmacology 37, 1773–1783. doi: 10.1038/npp.2012.24
Pubmed Abstract | Pubmed Full Text | CrossRef Full Text | Google Scholar
Berghuis, P., Dobszay, M. B., Wang, X., Spano, S., Ledda, F., Sousa, K. M., et al. (2005). Endocannabinoids regulate interneuron migration and morphogenesis by transactivating the TrkB receptor. Proc. Natl. Acad. Sci. U.S.A. 102, 19115–19120. doi: 10.1073/pnas.0509494102
Pubmed Abstract | Pubmed Full Text | CrossRef Full Text | Google Scholar
Berghuis, P., Rajnicek, A. M., Morozov, Y. M., Ross, R. A., Mulder, J., Urban, G. M., et al. (2007). Hardwiring the brain: endocannabinoids shape neuronal connectivity. Science 316, 1212–1216. doi: 10.1126/science.1137406
Pubmed Abstract | Pubmed Full Text | CrossRef Full Text | Google Scholar
Biscaia, M., Marin, S., Fernandez, B., Marco, E. M., Rubio, M., Guaza, C., et al. (2003). Chronic treatment with CP 55,940 during the peri-adolescent period differentially affects the behavioural responses of male and female rats in adulthood. Psychopharmacology (Berl) 170, 301–308. doi: 10.1007/s00213-003-1550-7
Pubmed Abstract | Pubmed Full Text | CrossRef Full Text | Google Scholar
Bortolato, M., Bini, V., Frau, R., Devoto, P., Pardu, A., Fan, Y., et al. (2014). Juvenile cannabinoid treatment induces frontostriatal gliogenesis in Lewis rats. Eur. Neuropsychopharmacol. 24, 974–985. doi: 10.1016/j.euroneuro.2013.12.011
Pubmed Abstract | Pubmed Full Text | CrossRef Full Text | Google Scholar
Boucher, A. A., Arnold, J. C., Duffy, L., Schofield, P. R., Micheau, J., and Karl, T. (2007a). Heterozygous neuregulin 1 mice are more sensitive to the behavioural effects of Delta9-tetrahydrocannabinol. Psychopharmacology (Berl) 192, 325–336. doi: 10.1007/s00213-007-0721-3
Pubmed Abstract | Pubmed Full Text | CrossRef Full Text | Google Scholar
Boucher, A. A., Hunt, G. E., Karl, T., Micheau, J., McGregor, I. S., and Arnold, J. C. (2007b). Heterozygous neuregulin 1 mice display greater baseline and Delta(9)-tetrahydrocannabinol-induced c-Fos expression. Neuroscience 149, 861–870. doi: 10.1016/j.neuroscience.2007.08.020
Pubmed Abstract | Pubmed Full Text | CrossRef Full Text | Google Scholar
Boucher, A. A., Hunt, G. E., Micheau, J., Huang, X., McGregor, I. S., Karl, T., et al. (2011). The schizophrenia susceptibility gene neuregulin 1 modulates tolerance to the effects of cannabinoids. Int. J. Neuropsychopharmacol. 14, 631–643. doi: 10.1017/S146114571000091X
Pubmed Abstract | Pubmed Full Text | CrossRef Full Text | Google Scholar
Braff, D. L., Geyer, M. A., and Swerdlow, N. R. (2001). Human studies of prepulse inhibition of startle: normal subjects, patient groups, and pharmacological studies. Psychopharmacology (Berl) 156, 234–258. doi: 10.1007/s002130100810
Pubmed Abstract | Pubmed Full Text | CrossRef Full Text | Google Scholar
Casey, B. J., Giedd, J. N., and Thomas, K. M. (2000). Structural and functional brain development and its relation to cognitive development. Biol. Psychol. 54, 241–257. doi: 10.1016/S0301-0511(00)00058-2
Pubmed Abstract | Pubmed Full Text | CrossRef Full Text | Google Scholar
Caspi, A., Moffitt, T. E., Cannon, M., McClay, J., Murray, R., Harrington, H., et al. (2005). Moderation of the effect of adolescent-onset cannabis use on adult psychosis by a functional polymorphism in the catechol-O-methyltransferase gene: longitudinal evidence of a gene X environment interaction. Biol. Psychiatry 57, 1117–1127. doi: 10.1016/j.biopsych.2005.01.026
Pubmed Abstract | Pubmed Full Text | CrossRef Full Text | Google Scholar
Cha, Y. M., Jones, K. H., Kuhn, C. M., Wilson, W. A., and Swartzwelder, H. S. (2007). Sex differences in the effects of delta9-tetrahydrocannabinol on spatial learning in adolescent and adult rats. Behav. Pharmacol. 18, 563–569. doi: 10.1097/FBP.0b013e3282ee7b7e
Pubmed Abstract | Pubmed Full Text | CrossRef Full Text | Google Scholar
Chabrol, H., Chauchard, E., and Girabet, J. (2008). Cannabis use and suicidal behaviours in high-school students. Addict. Behav. 33, 152–155. doi: 10.1016/j.addbeh.2007.04.029
Pubmed Abstract | Pubmed Full Text | CrossRef Full Text | Google Scholar
Chechik, G., Meilijson, I., and Ruppin, E. (1999). Neuronal regulation: a mechanism for synaptic pruning during brain maturation. Neural Comput. 11, 2061–2080. doi: 10.1162/089976699300016089
Pubmed Abstract | Pubmed Full Text | CrossRef Full Text | Google Scholar
Chen, J., Lipska, B. K., Halim, N., Ma, Q. D., Matsumoto, M., Melhem, S., et al. (2004). Functional analysis of genetic variation in catechol-O-methyltransferase (COMT): effects on mRNA, protein, and enzyme activity in postmortem human brain. Am. J. Hum. Genet. 75, 807–821. doi: 10.1086/425589
Pubmed Abstract | Pubmed Full Text | CrossRef Full Text | Google Scholar
Churchwell, J. C., Lopez-Larson, M., and Yurgelun-Todd, D. A. (2010). Altered frontal cortical volume and decision making in adolescent cannabis users. Front. Psychol. 1:225. doi: 10.3389/fpsyg.2010.00225
Pubmed Abstract | Pubmed Full Text | CrossRef Full Text | Google Scholar
Dahl, R. E. (2004). Adolescent development and the regulation of behavior and emotion: introduction to part VIII. Ann. N.Y. Acad. Sci. 1021, 294–295. doi: 10.1196/annals.1308.034
Pubmed Abstract | Pubmed Full Text | CrossRef Full Text | Google Scholar
Dalton, V. S., and Zavitsanou, K. (2010). Cannabinoid effects on CB1 receptor density in the adolescent brain: an autoradiographic study using the synthetic cannabinoid HU210. Synapse 64, 845–854. doi: 10.1002/syn.20801
Pubmed Abstract | Pubmed Full Text | CrossRef Full Text | Google Scholar
Dean, B., Sundram, S., Bradbury, R., Scarr, E., and Copolov, D. (2001). Studies on [3H]CP-55940 binding in the human central nervous system: regional specific changes in density of cannabinoid-1 receptors associated with schizophrenia and cannabis use. Neuroscience 103, 9–15. doi: 10.1016/S0306-4522(00)00552-2
Pubmed Abstract | Pubmed Full Text | CrossRef Full Text | Google Scholar
Decoster, J., van Os, J., Kenis, G., Henquet, C., Peuskens, J., De Hert, M., et al. (2011). Age at onset of psychotic disorder: cannabis, BDNF Val66Met, and sex-specific models of gene-environment interaction. Am. J. Med. Genet. B Neuropsychiatr. Genet. 156B, 363–369. doi: 10.1002/ajmg.b.31174
Pubmed Abstract | Pubmed Full Text | CrossRef Full Text | Google Scholar
Degenhardt, L., Coffey, C., Romaniuk, H., Swift, W., Carlin, J. B., Hall, W. D., et al. (2013). The persistence of the association between adolescent cannabis use and common mental disorders into young adulthood. Addiction 108, 124–133. doi: 10.1111/j.1360-0443.2012.04015.x
Pubmed Abstract | Pubmed Full Text | CrossRef Full Text | Google Scholar
Degenhardt, L., and Hall, W. (2002). Cannabis and psychosis. Curr. Psychiatry Rep. 4, 191–196. doi: 10.1007/s11920-002-0026-5
Pubmed Abstract | Pubmed Full Text | CrossRef Full Text | Google Scholar
Degenhardt, L., Hall, W., and Lynskey, M. (2003). Exploring the association between cannabis use and depression. Addiction 98, 1493–1504. doi: 10.1046/j.1360-0443.2003.00437.x
Pubmed Abstract | Pubmed Full Text | CrossRef Full Text | Google Scholar
Degenhardt, L., Tennant, C., Gilmour, S., Schofield, D., Nash, L., Hall, W., et al. (2007). The temporal dynamics of relationships between cannabis, psychosis and depression among young adults with psychotic disorders: findings from a 10-month prospective study. Psychol. Med. 37, 927–934. doi: 10.1017/S0033291707009956
Pubmed Abstract | Pubmed Full Text | CrossRef Full Text | Google Scholar
Dixon, L., Haas, G., Weiden, P., Sweeney, J., and Frances, A. (1990). Acute effects of drug abuse in schizophrenic patients: clinical observations and patients' self-reports. Schizophr. Bull. 16, 69–79. doi: 10.1093/schbul/16.1.69
Pubmed Abstract | Pubmed Full Text | CrossRef Full Text | Google Scholar
Dougherty, D. M., Mathias, C. W., Dawes, M. A., Furr, R. M., Charles, N. E., Liguori, A., et al. (2013). Impulsivity, attention, memory, and decision-making among adolescent marijuana users. Psychopharmacology (Berl) 226, 307–319. doi: 10.1007/s00213-012-2908-5
Pubmed Abstract | Pubmed Full Text | CrossRef Full Text | Google Scholar
Eggan, S. M., Mizoguchi, Y., Stoyak, S. R., and Lewis, D. A. (2010). Development of cannabinoid 1 receptor protein and messenger RNA in monkey dorsolateral prefrontal cortex. Cereb. Cortex 20, 1164–1174. doi: 10.1093/cercor/bhp179
Pubmed Abstract | Pubmed Full Text | CrossRef Full Text | Google Scholar
Ehrenreich, H., Rinn, T., Kunert, H. J., Moeller, M. R., Poser, W., Schilling, L., et al. (1999). Specific attentional dysfunction in adults following early start of cannabis use. Psychopharmacology (Berl) 142, 295–301. doi: 10.1007/s002130050892
Pubmed Abstract | Pubmed Full Text | CrossRef Full Text | Google Scholar
Ellgren, M., Artmann, A., Tkalych, O., Gupta, A., Hansen, H. S., Hansen, S. H., et al. (2008). Dynamic changes of the endogenous cannabinoid and opioid mesocorticolimbic systems during adolescence: THC effects. Eur. Neuropsychopharmacol. 18, 826–834. doi: 10.1016/j.euroneuro.2008.06.009
Pubmed Abstract | Pubmed Full Text | CrossRef Full Text | Google Scholar
Ferdinand, R. F., Sondeijker, F., van der Ende, J., Selten, J. P., Huizink, A., and Verhulst, F. C. (2005). Cannabis use predicts future psychotic symptoms, and vice versa. Addiction 100, 612–618. doi: 10.1111/j.1360-0443.2005.01070.x
Pubmed Abstract | Pubmed Full Text | CrossRef Full Text | Google Scholar
Fergusson, D. M., Horwood, L. J., and Swain-Campbell, N. R. (2003). Cannabis dependence and psychotic symptoms in young people. Psychol. Med. 33, 15–21. doi: 10.1017/S0033291702006402
Pubmed Abstract | Pubmed Full Text | CrossRef Full Text | Google Scholar
Fontes, M. A., Bolla, K. I., Cunha, P. J., Almeida, P. P., Jungerman, F., Laranjeira, R. R., et al. (2011). Cannabis use before age 15 and subsequent executive functioning. Br. J. Psychiatry 198, 442–447. doi: 10.1192/bjp.bp.110.077479
Pubmed Abstract | Pubmed Full Text | CrossRef Full Text | Google Scholar
Giedd, J. N., Blumenthal, J., Jeffries, N. O., Castellanos, F. X., Liu, H., Zijdenbos, A., et al. (1999). Brain development during childhood and adolescence: a longitudinal MRI study. Nat. Neurosci. 2, 861–863. doi: 10.1038/13158
Pubmed Abstract | Pubmed Full Text | CrossRef Full Text | Google Scholar
Giuffrida, A., Leweke, F. M., Gerth, C. W., Schreiber, D., Koethe, D., Faulhaber, J., et al. (2004). Cerebrospinal anandamide levels are elevated in acute schizophrenia and are inversely correlated with psychotic symptoms. Neuropsychopharmacology 29, 2108–2114. doi: 10.1038/sj.npp.1300558
Goldberger, C., Dervaux, A., Gourion, D., Bourdel, M. C., Loo, H., Laqueille, X., et al. (2010). Variable individual sensitivity to cannabis in patients with schizophrenia. Int. J. Neuropsychopharmacol. 13, 1145–1154. doi: 10.1017/S1461145710000647
Pubmed Abstract | Pubmed Full Text | CrossRef Full Text | Google Scholar
Grant, B. F., and Pickering, R. (1998). The relationship between cannabis use and DSM-IV cannabis abuse and dependence: results from the National Longitudinal Alcohol Epidemiologic Survey. J. Subst. Abuse 10, 255–264. doi: 10.1016/S0899-3289(99)00006-1
Pubmed Abstract | Pubmed Full Text | CrossRef Full Text | Google Scholar
Green, B. E., and Ritter, C. (2000). Marijuana use and depression. J. Health Soc. Behav. 41, 40–49. doi: 10.2307/2676359
Pubmed Abstract | Pubmed Full Text | CrossRef Full Text | Google Scholar
Gruber, S. A., Dahlgren, M. K., Sagar, K. A., Gonenc, A., and Killgore, W. D. (2012). Age of onset of marijuana use impacts inhibitory processing. Neurosci. Lett. 511, 89–94. doi: 10.1016/j.neulet.2012.01.039
Pubmed Abstract | Pubmed Full Text | CrossRef Full Text | Google Scholar
Hall, W., and Degenhardt, L. (2000). Cannabis use and psychosis: a review of clinical and epidemiological evidence. Aust. N.Z. J. Psychiatry 34, 26–34. doi: 10.1046/j.1440-1614.2000.00685.x
Pubmed Abstract | Pubmed Full Text | CrossRef Full Text | Google Scholar
Harkany, T., Guzman, M., Galve-Roperh, I., Berghuis, P., Devi, L. A., and Mackie, K. (2007). The emerging functions of endocannabinoid signaling during CNS development. Trends Pharmacol. Sci. 28, 83–92. doi: 10.1016/j.tips.2006.12.004
Pubmed Abstract | Pubmed Full Text | CrossRef Full Text | Google Scholar
Harkany, T., Keimpema, E., Barabas, K., and Mulder, J. (2008). Endocannabinoid functions controlling neuronal specification during brain development. Mol. Cell. Endocrinol. 286, S84–S90. doi: 10.1016/j.mce.2008.02.011
Pubmed Abstract | Pubmed Full Text | CrossRef Full Text | Google Scholar
Hayatbakhsh, M. R., Najman, J. M., Jamrozik, K., Mamun, A. A., Alati, R., and Bor, W. (2007). Cannabis and anxiety and depression in young adults: a large prospective study. J. Am. Acad. Child Adolesc. Psychiatry 46, 408–417. doi: 10.1097/chi.0b013e31802dc54d
Pubmed Abstract | Pubmed Full Text | CrossRef Full Text | Google Scholar
Heng, L., Beverley, J. A., Steiner, H., and Tseng, K. Y. (2011). Differential developmental trajectories for CB1 cannabinoid receptor expression in limbic/associative and sensorimotor cortical areas. Synapse 65, 278–286. doi: 10.1002/syn.20844
Pubmed Abstract | Pubmed Full Text | CrossRef Full Text | Google Scholar
Henquet, C., Krabbendam, L., Spauwen, J., Kaplan, C., Lieb, R., Wittchen, H. U., et al. (2005). Prospective cohort study of cannabis use, predisposition for psychosis, and psychotic symptoms in young people. BMJ 330, 11. doi: 10.1136/bmj.38267.664086.63
Pubmed Abstract | Pubmed Full Text | CrossRef Full Text | Google Scholar
Herkenham, M., Lynn, A. B., Little, M. D., Johnson, M. R., Melvin, L. S., De Costa, B. R., et al. (1990). Cannabinoid receptor localization in brain. Proc. Natl. Acad. Sci. U.S.A. 87, 1932–1936. doi: 10.1073/pnas.87.5.1932
Pubmed Abstract | Pubmed Full Text | CrossRef Full Text | Google Scholar
Higuera-Matas, A., Botreau, F., Miguens, M., Del, O. N., Borcel, E., Perez-Alvarez, L., et al. (2009). Chronic periadolescent cannabinoid treatment enhances adult hippocampal PSA-NCAM expression in male Wistar rats but only has marginal effects on anxiety, learning and memory. Pharmacol. Biochem. Behav. 93, 482–490. doi: 10.1016/j.pbb.2009.06.013
Pubmed Abstract | Pubmed Full Text | CrossRef Full Text | Google Scholar
Ho, B. C., Wassink, T. H., Ziebell, S., and Andreasen, N. C. (2011). Cannabinoid receptor 1 gene polymorphisms and marijuana misuse interactions on white matter and cognitive deficits in schizophrenia. Schizophr. Res. 128, 66–75. doi: 10.1016/j.schres.2011.02.021
Pubmed Abstract | Pubmed Full Text | CrossRef Full Text | Google Scholar
Hsieh, M. H., Swerdlow, N. R., and Braff, D. L. (2006). Effects of background and prepulse characteristics on prepulse inhibition and facilitation: implications for neuropsychiatric research. Biol. Psychiatry 59, 555–559. doi: 10.1016/j.biopsych.2005.07.032
Pubmed Abstract | Pubmed Full Text | CrossRef Full Text | Google Scholar
Huestegge, L., Radach, R., Kunert, H. J., and Heller, D. (2002). Visual search in long-term cannabis users with early age of onset. Prog. Brain Res. 140, 377–394. doi: 10.1016/S0079-6123(02)40064-7
Pubmed Abstract | Pubmed Full Text | CrossRef Full Text | Google Scholar
Jacobus, J., Goldenberg, D., Wierenga, C. E., Tolentino, N. J., Liu, T. T., and Tapert, S. F. (2012). Altered cerebral blood flow and neurocognitive correlates in adolescent cannabis users. Psychopharmacology (Berl) 222, 675–684. doi: 10.1007/s00213-012-2674-4
Pubmed Abstract | Pubmed Full Text | CrossRef Full Text | Google Scholar
Jager, G., Block, R. I., Luijten, M., and Ramsey, N. F. (2010). Cannabis use and memory brain function in adolescent boys: a cross-sectional multicenter functional magnetic resonance imaging study. J. Am. Acad. Child Adolesc. Psychiatry 49, 561–572. doi: 10.1016/j.jaac.2010.02.001
Pubmed Abstract | Pubmed Full Text | CrossRef Full Text | Google Scholar
Kano, M., Ohno-Shosaku, T., Hashimotodani, Y., Uchigashima, M., and Watanabe, M. (2009). Endocannabinoid-mediated control of synaptic transmission. Physiol. Rev. 89, 309–380. doi: 10.1152/physrev.00019.2008
Pubmed Abstract | Pubmed Full Text | CrossRef Full Text | Google Scholar
Katona, I. (2009). Endocannabinoid receptors: CNS localization of the CB(1) cannabinoid receptor. Curr. Top. Behav. Neurosci. 1, 65–86. doi: 10.1007/978-3-540-88955-7_3
Pubmed Abstract | Pubmed Full Text | CrossRef Full Text | Google Scholar
Kedzior, K. K., and Laeber, L. T. (2014). A positive association between anxiety disorders and cannabis use or cannabis use disorders in the general population–a meta-analysis of 31 studies. BMC Psychiatry 14:136. doi: 10.1186/1471-244X-14-136
Pubmed Abstract | Pubmed Full Text | CrossRef Full Text | Google Scholar
Krebs, M. O., Morvan, Y., Jay, T., Gaillard, R., and Kebir, O. (2014). Psychotomimetic effects at initiation of cannabis use are associated with cannabinoid receptor 1 (CNR1) variants in healthy students. Mol. Psychiatry 19, 402–403. doi: 10.1038/mp.2013.188
Pubmed Abstract | Pubmed Full Text | CrossRef Full Text | Google Scholar
Lachman, H. M., Morrow, B., Shprintzen, R., Veit, S., Parsia, S. S., Faedda, G., et al. (1996). Association of codon 108/158 catechol-O-methyltransferase gene polymorphism with the psychiatric manifestations of velo-cardio-facial syndrome. Am. J. Med. Genet. 67, 468–472.
Laviolette, S. R., and Grace, A. A. (2006). The roles of cannabinoid and dopamine receptor systems in neural emotional learning circuits: implications for schizophrenia and addiction. Cell. Mol. Life Sci. 63, 1597–1613. doi: 10.1007/s00018-006-6027-5
Pubmed Abstract | Pubmed Full Text | CrossRef Full Text | Google Scholar
Lee, T. T., Hill, M. N., Hillard, C. J., and Gorzalka, B. B. (2013). Temporal changes in N-acylethanolamine content and metabolism throughout the peri-adolescent period. Synapse 67, 4–10. doi: 10.1002/syn.21609
Pubmed Abstract | Pubmed Full Text | CrossRef Full Text | Google Scholar
Leroy, S., Griffon, N., Bourdel, M. C., Olie, J. P., Poirier, M. F., and Krebs, M. O. (2001). Schizophrenia and the cannabinoid receptor type 1 (CB1): association study using a single-base polymorphism in coding exon 1. Am. J. Med. Genet. 105, 749–752. doi: 10.1002/ajmg.10038
Pubmed Abstract | Pubmed Full Text | CrossRef Full Text | Google Scholar
Leweke, F. M., Giuffrida, A., Koethe, D., Schreiber, D., Nolden, B. M., Kranaster, L., et al. (2007). Anandamide levels in cerebrospinal fluid of first-episode schizophrenic patients: impact of cannabis use. Schizophr. Res. 94, 29–36. doi: 10.1016/j.schres.2007.04.025
Pubmed Abstract | Pubmed Full Text | CrossRef Full Text | Google Scholar
Leweke, F. M., Giuffrida, A., Wurster, U., Emrich, H. M., and Piomelli, D. (1999). Elevated endogenous cannabinoids in schizophrenia. Neuroreport 10, 1665–1669. doi: 10.1097/00001756-199906030-00008
Pubmed Abstract | Pubmed Full Text | CrossRef Full Text | Google Scholar
Llorente-Berzal, A., Puighermanal, E., Burokas, A., Ozaita, A., Maldonado, R., Marco, E. M., et al. (2013). Sex-dependent psychoneuroendocrine effects of THC and MDMA in an animal model of adolescent drug consumption. PLoS ONE 8:e78386. doi: 10.1371/journal.pone.0078386
Pubmed Abstract | Pubmed Full Text | CrossRef Full Text | Google Scholar
Long, L. E., Chesworth, R., Arnold, J. C., and Karl, T. (2010). A follow-up study: acute behavioural effects of Delta(9)-THC in female heterozygous neuregulin 1 transmembrane domain mutant mice. Psychopharmacology (Berl) 211, 277–289. doi: 10.1007/s00213-010-1896-6
Pubmed Abstract | Pubmed Full Text | CrossRef Full Text | Google Scholar
Long, L. E., Chesworth, R., Huang, X. F., McGregor, I. S., Arnold, J. C., and Karl, T. (2013). Transmembrane domain Nrg1 mutant mice show altered susceptibility to the neurobehavioural actions of repeated THC exposure in adolescence. Int. J. Neuropsychopharmacol. 16, 163–175. doi: 10.1017/S1461145711001854
Pubmed Abstract | Pubmed Full Text | CrossRef Full Text | Google Scholar
Lopez-Larson, M. P., Bogorodzki, P., Rogowska, J., McGlade, E., King, J. B., Terry, J., et al. (2011). Altered prefrontal and insular cortical thickness in adolescent marijuana users. Behav. Brain Res. 220, 164–172. doi: 10.1016/j.bbr.2011.02.001
Pubmed Abstract | Pubmed Full Text | CrossRef Full Text | Google Scholar
Lopez-Larson, M. P., Rogowska, J., Bogorodzki, P., Bueler, C. E., McGlade, E. C., and Yurgelun-Todd, D. A. (2012). Cortico-cerebellar abnormalities in adolescents with heavy marijuana use. Psychiatry Res. 202, 224–232. doi: 10.1016/j.pscychresns.2011.11.005
Pubmed Abstract | Pubmed Full Text | CrossRef Full Text | Google Scholar
Lynskey, M. T., Glowinski, A. L., Todorov, A. A., Bucholz, K. K., Madden, P. A., Nelson, E. C., et al. (2004). Major depressive disorder, suicidal ideation, and suicide attempt in twins discordant for cannabis dependence and early-onset cannabis use. Arch. Gen. Psychiatry 61, 1026–1032. doi: 10.1001/archpsyc.61.10.1026
Pubmed Abstract | Pubmed Full Text | CrossRef Full Text | Google Scholar
Malone, D. T., and Taylor, D. A. (2006). The effect of Delta9-tetrahydrocannabinol on sensorimotor gating in socially isolated rats. Behav. Brain Res. 166, 101–109. doi: 10.1016/j.bbr.2005.07.009
Pubmed Abstract | Pubmed Full Text | CrossRef Full Text | Google Scholar
Manzoni, O. J., and Bockaert, J. (2001). Cannabinoids inhibit GABAergic synaptic transmission in mice nucleus accumbens. Eur. J. Pharmacol. 412, R3–R5. doi: 10.1016/S0014-2999(01)00723-3
Pubmed Abstract | Pubmed Full Text | CrossRef Full Text | Google Scholar
Marsicano, G., Goodenough, S., Monory, K., Hermann, H., Eder, M., Cannich, A., et al. (2003). CB1 cannabinoid receptors and on-demand defense against excitotoxicity. Science 302, 84–88. doi: 10.1126/science.1088208
Pubmed Abstract | Pubmed Full Text | CrossRef Full Text | Google Scholar
Marsicano, G., and Lutz, B. (1999). Expression of the cannabinoid receptor CB1 in distinct neuronal subpopulations in the adult mouse forebrain. Eur. J. Neurosci. 11, 4213–4225. doi: 10.1046/j.1460-9568.1999.00847.x
Pubmed Abstract | Pubmed Full Text | CrossRef Full Text | Google Scholar
Mato, S., Del, O. E., and Pazos, A. (2003). Ontogenetic development of cannabinoid receptor expression and signal transduction functionality in the human brain. Eur. J. Neurosci. 17, 1747–1754. doi: 10.1046/j.1460-9568.2003.02599.x
Pubmed Abstract | Pubmed Full Text | CrossRef Full Text | Google Scholar
Medina, K. L., Hanson, K. L., Schweinsburg, A. D., Cohen-Zion, M., Nagel, B. J., and Tapert, S. F. (2007a). Neuropsychological functioning in adolescent marijuana users: subtle deficits detectable after a month of abstinence. J. Int. Neuropsychol. Soc. 13, 807–820. doi: 10.1017/S1355617707071032
Pubmed Abstract | Pubmed Full Text | CrossRef Full Text | Google Scholar
Medina, K. L., McQueeny, T., Nagel, B. J., Hanson, K. L., Yang, T. T., and Tapert, S. F. (2009). Prefrontal cortex morphometry in abstinent adolescent marijuana users: subtle gender effects. Addict. Biol. 14, 457–468. doi: 10.1111/j.1369-1600.2009.00166.x
Pubmed Abstract | Pubmed Full Text | CrossRef Full Text | Google Scholar
Medina, K. L., Nagel, B. J., Park, A., McQueeny, T., and Tapert, S. F. (2007b). Depressive symptoms in adolescents: associations with white matter volume and marijuana use. J. Child Psychol. Psychiatry 48, 592–600. doi: 10.1111/j.1469-7610.2007.01728.x
Pubmed Abstract | Pubmed Full Text | CrossRef Full Text | Google Scholar
Meier, M. H., Caspi, A., Ambler, A., Harrington, H., Houts, R., Keefe, R. S., et al. (2012). Persistent cannabis users show neuropsychological decline from childhood to midlife. Proc. Natl. Acad. Sci. U.S.A. 109, E2657–E2664. doi: 10.1073/pnas.1206820109
Pubmed Abstract | Pubmed Full Text | CrossRef Full Text | Google Scholar
Molina-Holgado, E., Vela, J. M., Arevalo-Martin, A., Almazan, G., Molina-Holgado, F., Borrell, J., et al. (2002). Cannabinoids promote oligodendrocyte progenitor survival: involvement of cannabinoid receptors and phosphatidylinositol-3 kinase/Akt signaling. J. Neurosci. 22, 9742–9753.
Morozov, Y. M., and Freund, T. F. (2003). Post-natal development of type 1 cannabinoid receptor immunoreactivity in the rat hippocampus. Eur. J. Neurosci. 18, 1213–1222. doi: 10.1046/j.1460-9568.2003.02852.x
Pubmed Abstract | Pubmed Full Text | CrossRef Full Text | Google Scholar
Mueser, K. T., Bellack, A. S., and Blanchard, J. J. (1992). Comorbidity of schizophrenia and substance abuse: implications for treatment. J. Consult. Clin. Psychol. 60, 845–856. doi: 10.1037/0022-006X.60.6.845
Pubmed Abstract | Pubmed Full Text | CrossRef Full Text | Google Scholar
Mulder, J., Aguado, T., Keimpema, E., Barabas, K., Ballester Rosado, C. J., Nguyen, L., et al. (2008). Endocannabinoid signaling controls pyramidal cell specification and long-range axon patterning. Proc. Natl. Acad. Sci. U.S.A. 105, 8760–8765. doi: 10.1073/pnas.0803545105
Pubmed Abstract | Pubmed Full Text | CrossRef Full Text | Google Scholar
Newell, K. A., Deng, C., and Huang, X. F. (2006). Increased cannabinoid receptor density in the posterior cingulate cortex in schizophrenia. Exp. Brain Res. 172, 556–560. doi: 10.1007/s00221-006-0503-x
Pubmed Abstract | Pubmed Full Text | CrossRef Full Text | Google Scholar
Oades, R. D., Klimke, A., Henning, U., and Rao, M. L. (2002). Relations of clinical features, subgroups and medication to serum monoamines in schizophrenia. Hum. Psychopharmacol. 17, 15–27. doi: 10.1002/hup.368
Pubmed Abstract | Pubmed Full Text | CrossRef Full Text | Google Scholar
O'Shea, M., McGregor, I. S., and Mallet, P. E. (2006). Repeated cannabinoid exposure during perinatal, adolescent or early adult ages produces similar longlasting deficits in object recognition and reduced social interaction in rats. J. Psychopharmacol. 20, 611–621. doi: 10.1177/0269881106065188
Pubmed Abstract | Pubmed Full Text | CrossRef Full Text | Google Scholar
O'Tuathaigh, C. M., Clarke, G., Walsh, J., Desbonnet, L., Petit, E., O'Leary, C., et al. (2012). Genetic vs. pharmacological inactivation of COMT influences cannabinoid-induced expression of schizophrenia-related phenotypes. Int. J. Neuropsychopharmacol. 15, 1331–1342. doi: 10.1017/S1461145711001581
Pubmed Abstract | Pubmed Full Text | CrossRef Full Text | Google Scholar
O'Tuathaigh, C. M., Hryniewiecka, M., Behan, A., Tighe, O., Coughlan, C., Desbonnet, L., et al. (2010). Chronic adolescent exposure to Delta-9-tetrahydrocannabinol in COMT mutant mice: impact on psychosis-related and other phenotypes. Neuropsychopharmacology 35, 2262–2273. doi: 10.1038/npp.2010.100
Pubmed Abstract | Pubmed Full Text | CrossRef Full Text | Google Scholar
Patton, G. C., Coffey, C., Carlin, J. B., Degenhardt, L., Lynskey, M., and Hall, W. (2002). Cannabis use and mental health in young people: cohort study. BMJ 325, 1195–1198. doi: 10.1136/bmj.325.7374.1195
Pubmed Abstract | Pubmed Full Text | CrossRef Full Text | Google Scholar
Pedersen, W. (2008). Does cannabis use lead to depression and suicidal behaviours? A population-based longitudinal study. Acta Psychiatr. Scand. 118, 395–403. doi: 10.1111/j.1600-0447.2008.01259.x
Pubmed Abstract | Pubmed Full Text | CrossRef Full Text | Google Scholar
Perry, W., Minassian, A., Feifel, D., and Braff, D. L. (2001). Sensorimotor gating deficits in bipolar disorder patients with acute psychotic mania. Biol. Psychiatry. 50, 418–424. doi: 10.1016/S0006-3223(01)01184-2
Pubmed Abstract | Pubmed Full Text | CrossRef Full Text | Google Scholar
Pope, H. G. Jr., Jacobs, A., Mialet, J. P., Yurgelun-Todd, D., and Gruber, S. (1997). Evidence for a sex-specific residual effect of cannabis on visuospatial memory. Psychother. Psychosom. 66, 179–184. doi: 10.1159/000289132
Pubmed Abstract | Pubmed Full Text | CrossRef Full Text | Google Scholar
Puighermanal, E., Busquets-Garcia, A., Gomis-Gonzalez, M., Marsicano, G., Maldonado, R., and Ozaita, A. (2013). Dissociation of the pharmacological effects of THC by mTOR blockade. Neuropsychopharmacology 38, 1334–1343. doi: 10.1038/npp.2013.31
Pubmed Abstract | Pubmed Full Text | CrossRef Full Text | Google Scholar
Quinn, H. R., Matsumoto, I., Callaghan, P. D., Long, L. E., Arnold, J. C., Gunasekaran, N., et al. (2008). Adolescent rats find repeated Delta(9)-THC less aversive than adult rats but display greater residual cognitive deficits and changes in hippocampal protein expression following exposure. Neuropsychopharmacology 33, 1113–1126. doi: 10.1038/sj.npp.1301475
Pubmed Abstract | Pubmed Full Text | CrossRef Full Text | Google Scholar
Reilly, D., Didcott, P., Swift, W., and Hall, W. (1998). Long-term cannabis use: characteristics of users in an Australian rural area. Addiction 93, 837–846. doi: 10.1046/j.1360-0443.1998.9368375.x
Pubmed Abstract | Pubmed Full Text | CrossRef Full Text | Google Scholar
Renard, J., Krebs, M. O., Jay, T. M., and Le, P. G. (2013). Long-term cognitive impairments induced by chronic cannabinoid exposure during adolescence in rats: a strain comparison. Psychopharmacology (Berl) 225, 781–790. doi: 10.1007/s00213-012-2865-z
Pubmed Abstract | Pubmed Full Text | CrossRef Full Text | Google Scholar
Rey, J. M., Sawyer, M. G., Raphael, B., Patton, G. C., and Lynskey, M. (2002). Mental health of teenagers who use cannabis. Results of an Australian survey. Br. J. Psychiatry 180, 216–221. doi: 10.1192/bjp.180.3.216
Pubmed Abstract | Pubmed Full Text | CrossRef Full Text | Google Scholar
Rodriguez de Fonseca, F., Ramos, J. A., Bonnin, A., and Fernandez-Ruiz, J. J. (1993). Presence of cannabinoid binding sites in the brain from early postnatal ages. Neuroreport 4, 135–138. doi: 10.1097/00001756-199302000-00005
Pubmed Abstract | Pubmed Full Text | CrossRef Full Text | Google Scholar
Romero, J., Garcia-Palomero, E., Berrendero, F., Garcia-Gil, L., Hernandez, M. L., Ramos, J. A., et al. (1997). Atypical location of cannabinoid receptors in white matter areas during rat brain development. Synapse 26, 317–323.
Rubino, T., Realini, N., Braida, D., Alberio, T., Capurro, V., Vigano, D., et al. (2009a). The depressive phenotype induced in adult female rats by adolescent exposure to THC is associated with cognitive impairment and altered neuroplasticity in the prefrontal cortex. Neurotox. Res. 15, 291–302. doi: 10.1007/s12640-009-9031-3
Pubmed Abstract | Pubmed Full Text | CrossRef Full Text | Google Scholar
Rubino, T., Realini, N., Braida, D., Guidi, S., Capurro, V., Vigano, D., et al. (2009b). Changes in hippocampal morphology and neuroplasticity induced by adolescent THC treatment are associated with cognitive impairment in adulthood. Hippocampus 19, 763–772. doi: 10.1002/hipo.20554
Pubmed Abstract | Pubmed Full Text | CrossRef Full Text | Google Scholar
Rubino, T., Vigano', D., Realini, N., Guidali, C., Braida, D., Capurro, V., et al. (2008). Chronic delta 9-tetrahydrocannabinol during adolescence provokes sex-dependent changes in the emotional profile in adult rats: behavioral and biochemical correlates. Neuropsychopharmacology 33, 2760–2771. doi: 10.1038/sj.npp.1301664
Pubmed Abstract | Pubmed Full Text | CrossRef Full Text | Google Scholar
Sanchez-Blazquez, P., Rodriguez-Munoz, M., and Garzon, J. (2014). The cannabinoid receptor 1 associates with NMDA receptors to produce glutamatergic hypofunction: implications in psychosis and schizophrenia. Front. Pharmacol. 4:169. doi: 10.3389/fphar.2013.00169
Pubmed Abstract | Pubmed Full Text | CrossRef Full Text | Google Scholar
Schneider, M., and Koch, M. (2003). Chronic pubertal, but not adult chronic cannabinoid treatment impairs sensorimotor gating, recognition memory, and the performance in a progressive ratio task in adult rats. Neuropsychopharmacology 28, 1760–1769. doi: 10.1038/sj.npp.1300225
Pubmed Abstract | Pubmed Full Text | CrossRef Full Text | Google Scholar
Schneider, M., and Koch, M. (2007). The effect of chronic peripubertal cannabinoid treatment on deficient object recognition memory in rats after neonatal mPFC lesion. Eur. Neuropsychopharmacol. 17, 180–186. doi: 10.1016/j.euroneuro.2006.03.009
Pubmed Abstract | Pubmed Full Text | CrossRef Full Text | Google Scholar
Schneider, M., Schomig, E., and Leweke, F. M. (2008). Acute and chronic cannabinoid treatment differentially affects recognition memory and social behavior in pubertal and adult rats. Addict. Biol. 13, 345–357. doi: 10.1111/j.1369-1600.2008.00117.x
Pubmed Abstract | Pubmed Full Text | CrossRef Full Text | Google Scholar
Schweinsburg, A. D., Brown, S. A., and Tapert, S. F. (2008). The influence of marijuana use on neurocognitive functioning in adolescents. Curr. Drug Abuse Rev. 1, 99–111. doi: 10.2174/1874473710801010099
Pubmed Abstract | Pubmed Full Text | CrossRef Full Text | Google Scholar
Schweinsburg, A. D., Schweinsburg, B. C., Medina, K. L., McQueeny, T., Brown, S. A., and Tapert, S. F. (2010). The influence of recency of use on fMRI response during spatial working memory in adolescent marijuana users. J. Psychoactive Drugs 42, 401–412. doi: 10.1080/02791072.2010.10400703
Pubmed Abstract | Pubmed Full Text | CrossRef Full Text | Google Scholar
Solowij, N., Jones, K. A., Rozman, M. E., Davis, S. M., Ciarrochi, J., Heaven, P. C., et al. (2012). Reflection impulsivity in adolescent cannabis users: a comparison with alcohol-using and non-substance-using adolescents. Psychopharmacology (Berl) 219, 575–586. doi: 10.1007/s00213-011-2486-y
Pubmed Abstract | Pubmed Full Text | CrossRef Full Text | Google Scholar
Spear, L. P. (2000). The adolescent brain and age-related behavioral manifestations. Neurosci. Biobehav. Rev. 24, 417–463. doi: 10.1016/S0149-7634(00)00014-2
Pubmed Abstract | Pubmed Full Text | CrossRef Full Text | Google Scholar
Spencer, J. R., Darbyshire, K. M., Boucher, A. A., Kashem, M. A., Long, L. E., McGregor, I. S., et al. (2013). Novel molecular changes induced by Nrg1 hypomorphism and Nrg1-cannabinoid interaction in adolescence: a hippocampal proteomic study in mice. Front. Cell. Neurosci. 7:15. doi: 10.3389/fncel.2013.00015
Pubmed Abstract | Pubmed Full Text | CrossRef Full Text | Google Scholar
Stefanis, N. C., Delespaul, P., Henquet, C., Bakoula, C., Stefanis, C. N., and van Os, J. (2004). Early adolescent cannabis exposure and positive and negative dimensions of psychosis. Addiction 99, 1333–1341. doi: 10.1111/j.1360-0443.2004.00806.x
Pubmed Abstract | Pubmed Full Text | CrossRef Full Text | Google Scholar
Toga, A. W., Thompson, P. M., and Sowell, E. R. (2006). Mapping brain maturation. Trends Neurosci. 29, 148–159. doi: 10.1016/j.tins.2006.01.007
Pubmed Abstract | Pubmed Full Text | CrossRef Full Text | Google Scholar
Tournier, M., Sorbara, F., Gindre, C., Swendsen, J. D., and Verdoux, H. (2003). Cannabis use and anxiety in daily life: a naturalistic investigation in a non-clinical population. Psychiatry Res. 118, 1–8. doi: 10.1016/S0165-1781(03)00052-0
Pubmed Abstract | Pubmed Full Text | CrossRef Full Text | Google Scholar
Ujike, H., Takaki, M., Nakata, K., Tanaka, Y., Takeda, T., Kodama, M., et al. (2002). CNR1, central cannabinoid receptor gene, associated with susceptibility to hebephrenic schizophrenia. Mol. Psychiatry 7, 515–518. doi: 10.1038/sj.mp.4001029
Pubmed Abstract | Pubmed Full Text | CrossRef Full Text | Google Scholar
Vaidya, J. G., Block, R. I., O'Leary, D. S., Ponto, L. B., Ghoneim, M. M., and Bechara, A. (2012). Effects of chronic marijuana use on brain activity during monetary decision-making. Neuropsychopharmacology 37, 618–629. doi: 10.1038/npp.2011.227
Pubmed Abstract | Pubmed Full Text | CrossRef Full Text | Google Scholar
van Laar, M., van Dorsselaer, S., Monshouwer, K., and de Graaf, R. (2007). Does cannabis use predict the first incidence of mood and anxiety disorders in the adult population? Addiction 102, 1251–1260. doi: 10.1111/j.1360-0443.2007.01875.x
Pubmed Abstract | Pubmed Full Text | CrossRef Full Text | Google Scholar
Verdoux, H., Gindre, C., Sorbara, F., Tournier, M., and Swendsen, J. D. (2003). Effects of cannabis and psychosis vulnerability in daily life: an experience sampling test study. Psychol. Med. 33, 23–32. doi: 10.1017/S0033291702006384
Pubmed Abstract | Pubmed Full Text | CrossRef Full Text | Google Scholar
Verdoux, H., Tournier, M., and Cougnard, A. (2005). Impact of substance use on the onset and course of early psychosis. Schizophr. Res. 79, 69–75. doi: 10.1016/j.schres.2004.12.008
Pubmed Abstract | Pubmed Full Text | CrossRef Full Text | Google Scholar
Vigano, D., Guidali, C., Petrosino, S., Realini, N., Rubino, T., Di, M., V., et al. (2009). Involvement of the endocannabinoid system in phencyclidine-induced cognitive deficits modelling schizophrenia. Int. J. Neuropsychopharmacol. 12, 599–614. doi: 10.1017/S1461145708009371
Pubmed Abstract | Pubmed Full Text | CrossRef Full Text | Google Scholar
Vinod, K. Y., and Hungund, B. L. (2006). Role of the endocannabinoid system in depression and suicide. Trends Pharmacol. Sci. 27, 539–545. doi: 10.1016/j.tips.2006.08.006
Pubmed Abstract | Pubmed Full Text | CrossRef Full Text | Google Scholar
Wegener, N., and Koch, M. (2009). Behavioural disturbances and altered Fos protein expression in adult rats after chronic pubertal cannabinoid treatment. Brain Res. 1253, 81–91. doi: 10.1016/j.brainres.2008.11.081
Pubmed Abstract | Pubmed Full Text | CrossRef Full Text | Google Scholar
Wilson, W., Mathew, R., Turkington, T., Hawk, T., Coleman, R. E., and Provenzale, J. (2000). Brain morphological changes and early marijuana use: a magnetic resonance and positron emission tomography study. J. Addict. Dis. 19, 1–22. doi: 10.1300/J069v19n01_01
Pubmed Abstract | Pubmed Full Text | CrossRef Full Text | Google Scholar
Yucel, M., Solowij, N., Respondek, C., Whittle, S., Fornito, A., Pantelis, C., et al. (2008). Regional brain abnormalities associated with long-term heavy cannabis use. Arch. Gen. Psychiatry 65, 694–701. doi: 10.1001/archpsyc.65.6.694
Pubmed Abstract | Pubmed Full Text | CrossRef Full Text | Google Scholar
Zalesky, A., Solowij, N., Yucel, M., Lubman, D. I., Takagi, M., Harding, I. H., et al. (2012). Effect of long-term cannabis use on axonal fibre connectivity. Brain 135, 2245–2255. doi: 10.1093/brain/aws136
Pubmed Abstract | Pubmed Full Text | CrossRef Full Text | Google Scholar
Zamberletti, E., Beggiato, S., Steardo, L. Jr., Prini, P., Antonelli, T., Ferraro, L., et al. (2014). Alterations of prefrontal cortex GABAergic transmission in the complex psychotic-like phenotype induced by adolescent delta-9-tetrahydrocannabinol exposure in rats. Neurobiol. Dis. 63, 35–47. doi: 10.1016/j.nbd.2013.10.028
Pubmed Abstract | Pubmed Full Text | CrossRef Full Text | Google Scholar
Zavitsanou, K., Garrick, T., and Huang, X. F. (2004). Selective antagonist [3H]SR141716A binding to cannabinoid CB1 receptors is increased in the anterior cingulate cortex in schizophrenia. Prog. Neuropsychopharmacol. Biol. Psychiatry 28, 355–360. doi: 10.1016/j.pnpbp.2003.11.005
Pubmed Abstract | Pubmed Full Text | CrossRef Full Text | Google Scholar
Keywords: cannabinoids, adolescence, long-term consequences, behavior, psychosis
Citation: Renard J, Krebs M-O, Le Pen G and Jay TM (2014) Long-term consequences of adolescent cannabinoid exposure in adult psychopathology. Front. Neurosci. 8:361. doi: 10.3389/fnins.2014.00361
Received: 24 June 2014; Accepted: 21 October 2014;
Published online: 10 November 2014.
Edited by:
Steven R. Laviolette, University of Western Ontario, CanadaReviewed by:
Luigia Trabace, University of Foggia, ItalyCopyright © 2014 Renard, Krebs, Le Pen and Jay. This is an open-access article distributed under the terms of the Creative Commons Attribution License (CC BY). The use, distribution or reproduction in other forums is permitted, provided the original author(s) or licensor are credited and that the original publication in this journal is cited, in accordance with accepted academic practice. No use, distribution or reproduction is permitted which does not comply with these terms.
*Correspondence: Thérèse M. Jay, Unités Mixtes de Recherche 894 Institut National de la santé et de la Recherche Médicale, Centre de Psychiatrie et Neurosciences, Centre de Psychiatrie et Neurosciences, 2ter rue d'Alésia, 75014 Paris, France e-mail:dGhlcmVzZS5qYXlAaW5zZXJtLmZy
Disclaimer: All claims expressed in this article are solely those of the authors and do not necessarily represent those of their affiliated organizations, or those of the publisher, the editors and the reviewers. Any product that may be evaluated in this article or claim that may be made by its manufacturer is not guaranteed or endorsed by the publisher.
Research integrity at Frontiers
Learn more about the work of our research integrity team to safeguard the quality of each article we publish.