- 1Laboratory of Animal Physiology, Department of Applied Molecular Biosciences, Graduate School of Bioagricultural Sciences, Nagoya University, Nagoya, Japan
- 2Institute of Transformative Bio-Molecules (WPI-ITbM), Nagoya University, Nagoya, Japan
- 3Avian Bioscience Research Center, Graduate School of Bioagricultural Sciences, Nagoya University, Nagoya, Japan
- 4Division of Seasonal Biology, Department of Environmental Biology, National Institute for Basic Biology, Okazaki, Japan
Most vertebrates living outside the tropical zone show robust physiological responses in response to seasonal changes in photoperiod, such as seasonal reproduction, molt, and migration. The highly sophisticated photoperiodic mechanism in Japanese quail has been used to uncover the mechanism of seasonal reproduction. Molecular analysis of quail mediobasal hypothalamus (MBH) revealed that local thyroid hormone activation within the MBH plays a critical role in the photoperiodic response of gonads. This activation is accomplished by two gene switches: thyroid hormone-activating (DIO2) and thyroid hormone-inactivating enzymes (DIO3). Functional genomics studies have shown that long-day induced thyroid-stimulating hormone (TSH) in the pars tuberalis (PT) of the pituitary gland regulates DIO2/3 switching. In birds, light information received directly by deep brain photoreceptors regulates PT TSH. Recent studies demonstrated that Opsin 5-positive cerebrospinal fluid (CSF)-contacting neurons are deep brain photoreceptors that regulate avian seasonal reproduction. Although the involvement of TSH and DIO2/3 in seasonal reproduction has been confirmed in various mammals, the light input pathway that regulates PT TSH in mammals differs from that of birds. In mammals, the eye is the only photoreceptor organ and light information received by the eye is transmitted to the pineal gland through the circadian pacemaker, the suprachiasmatic nucleus. Nocturnal melatonin secretion from the pineal gland indicates the length of night and regulates the PT TSH. In fish, the regulatory machinery for seasonal reproduction, from light input to neuroendocrine output, has been recently demonstrated in the coronet cells of the saccus vasculosus (SV). The SV is unique to fish and coronet cells are CSF-contacting neurons. Here, we discuss the universality and diversity of signal transduction pathways that regulate vertebrate seasonal reproduction.
Introduction
Animals that reproduce year-round (e.g., human beings and mice) are so-called non-seasonal breeders. However, in most animals living outside of tropical zones, gametogenesis occurs during a particular period of the year. This allows the animals to produce offspring in a favorable season. Such animals are called seasonal breeders. The timing of the breeding period is related to the length of the gestation or incubation period. Animals that mate in spring-summer (e.g., hamsters, quail, and medaka) are called long-day breeders, whereas those that mate in fall-winter (e.g., sheep, emu, and salmon) are called short-day breeders.
Involvement of the Mediobasal Hypothalamus in the Regulation of Seasonal Reproduction in Birds
The photoperiodic responses of seasonally breeding birds are so robust and rapid that they provide excellent models for the study of seasonal reproduction. Avian gonads change size seasonally, increasing or decreasing more than one hundred-fold within a few weeks. For example, when Japanese quail (Coturnix japonica) kept under short-day conditions are transferred to long-day conditions, an increase in plasma gonadotropin (luteinizing hormone: LH) concentration is observed by the end of the first long day and spermatogenesis is accomplished within 2 weeks (Nicholls et al., 1983). Because quail can be readily obtained from quail farms, it has been frequently used for the study of photoperiodism. Quail has been used as a model to explore the center that regulates seasonal reproduction. Lesions of the mediobasal hypothalamus (MBH), including the median eminence (ME) and infundibular nucleus (IN), or the dorsal MBH result in low plasma LH concentration and attenuate testicular growth under long-day conditions (Sharp and Follett, 1969; Davies and Follett, 1975). Electrical stimulation of the MBH increases plasma LH concentration (Konishi et al., 1987) and testicular growth (Ohta et al., 1984). Birds are receiving light information within the brain and local illumination of the MBH induces testicular development, suggesting the presence of deep brain photoreceptors within the MBH (Homma et al., 1979). In addition, expression of the neuronal activation marker, c-Fos, was observed within the ME and IN in response to a single long-day stimulus (Meddle and Follett, 1995, 1997). Therefore, the MBH is considered to be the center for seasonal reproduction in birds.
Local Thyroid Hormone Activation Driven by Pars Tuberalis Thyrotropin is the Key for Eliciting Photoperiodic Response in Birds
Lack of genome information had been a barrier to avian research for long time. However, differential subtractive hybridization analysis has revealed that long-day stimuli induce mRNA that encode type 2 deiodinase (DIO2) in the ependymal cells (ECs) (also known as tanycytes) lining the ventro-lateral walls of the third ventricle within the MBH (Yoshimura et al., 2003) (Figure 1). DIO2 is a thyroid hormone-activating enzyme that converts prohormone thyroxine (T4) to bioactive 3,5,3'-triiodothyronine (T3). Subsequently, long day suppression of type 3 deiodinase (DIO3) was reported. DIO3 is a thyroid hormone-inactivating enzyme that converts T4 and T3 to inactive metabolites rT3 and T2. These reciprocal gene switches, DIO2/3, appear to be the key for regulation of seasonal reproduction in quail (Yasuo et al., 2005). Indeed, T3 was up-regulated by these gene switches in the MBH under long-day conditions. In addition, ICV administration of T3 mimicked long day-induced testicular growth under short-day conditions and infusion of DIO2 inhibitor blocked testicular growth under long-day conditions (Yoshimura et al., 2003). It is well established that thyroid hormone is essential for brain development and is also critical for adult brain plasticity (Bernal, 2005). Indeed, T3 is reported to cause morphological changes in gonadotropin-releasing hormone (GnRH) nerve terminals and glial cells in the ME (Yamamura et al., 2006). Most GnRH nerve terminals are covered with glial cells and do not touch the basal lamina of the perivascular space of portal capillaries under short-day conditions. Under long-day conditions, however, many GnRH nerve terminals are in direct contact with the basal lamina. T3 implantation under short-day conditions mimics these morphological changes and results in testicular development (Yamamura et al., 2006). These findings suggest that local activation of thyroid hormone within the MBH is a critical event for the seasonal regulation of GnRH secretion.
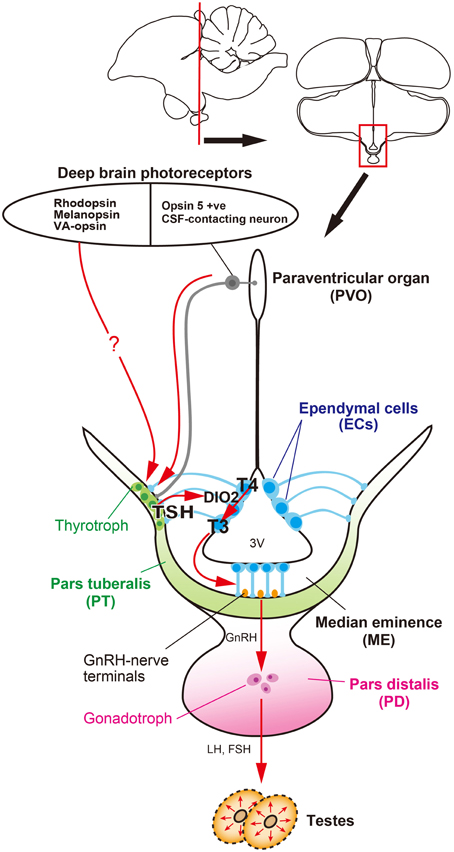
Figure 1. Signal transduction pathway regulating seasonal reproduction in birds. Light information received by deep brain photoreceptors is transmitted to the pars tuberalis (PT) of the pituitary gland, a regulatory hub for seasonal reproduction. Long day-induced thyrotropin (TSH) in the PT acts on ependymal cells to induce a thyroid hormone-activating enzyme, DIO2. The bioactive thyroid hormone, T3 is converted by DIO2 from the prohormone, T4. T3 regulates seasonal morphological changes in GnRH nerve terminals and glial processes, thereby regulating or modulating GnRH secretion.
The availability of genome sequences in avian species has provided an opportunity to employ a functional genomics approach to photoperiodism research. Using a functional genomics approach, long-day induction of TSHB mRNA, which encodes the β subunit of thyroid-stimulating hormone (TSH), was observed in the par tuberalis (PT) of the pituitary gland. This TSHB induction preceded DIO2/3 switching by about 4 h. Localization of TSH receptor (TSHR) was observed in the ECs where DIO2/3 are expressed, suggesting that PT TSH may act on the TSHR expressed in the ECs to regulate DIO2/3 switching. Indeed, ICV infusion of TSH drives DIO2/3 switching and testicular growth, even under short-day conditions (Nakao et al., 2008) (Figure 1). However, the transport system of PT TSH to the ECs remains unclear.
Involvement of Deep Brain Photoreceptors in Avian Seasonal Reproduction
Although the eye is the only photoreceptor organ in mammals, photoreceptive organs in non-mammalian vertebrates include eyes, pineal organs, and deep brain photoreceptors. Photo-capability in the deep brain was first demonstrated in European minnows, in which it controls changes in skin color (von Frisch, 1911). Subsequently, evidence of a deep brain photoreceptor that regulates seasonal reproduction in ducks was reported. Blind ducks continue to show photoperiodic responses, whereas enveloping the heads of ducks with black caps blocks testicular responses (Benoit, 1935). Moreover, injection of India ink under the scalp in pinealectomized sparrows abolishes the photoperiodic response (Menaker et al., 1970). Both pinealectomized and blinded quail are reported to undergo gonadal development in response to light cues (Siopes and Wilson, 1974). In addition, photo-stimulation of the hypothalamus using light fiber and light-emitting beads prompts testicular development in sparrows (Yokoyama et al., 1978) and Japanese quail (Homma et al., 1979). It has been confirmed that a broad spectrum of light penetrates into the brains of various vertebrate species (Hartwig and van Veen, 1979; Foster and Follett, 1985; Oishi and Ohashi, 1993).
Many groups have tried to identify deep brain photoreceptors. Several rhodopsin family proteins (e.g., rhodopsin (RH), melanopsin (OPN4), and vertebrate ancient (VA)-opsin) were reported to be localized in the avian deep brain region (Silver et al., 1988; Wada et al., 1998; Chaurasia et al., 2005; Halford et al., 2009). In addition, a novel opsin called Opsin 5 (OPN5: also known as neuropsin) was recently reported to be localized in the paraventricular organ (PVO) within the MBH (Nakane et al., 2010; Yamashita et al., 2010). This is intriguing because lesions around the PVO block the photoperiodic responses of gonads in Japanese quail (Sharp and Follett, 1969). Immunohistochemical analysis of OPN5 revealed its presence in the cerebrospinal fluid (CSF)-contacting neurons (Figure 2A). The CSF-contacting neurons in the PVO have long been a candidate deep brain photoreceptor because the retina and pineal organ evaginate from the diencephalon (around the third ventricle where the PVO is located) and the CSF-contacting neurons resemble photoreceptor cells in the developing retina (Vigh-Teichmann et al., 1980). Functional analysis demonstrated that OPN5 is a short-wavelength sensitive photopigment (Nakane et al., 2010; Yamashita et al., 2010) and long-day stimulation with short-wavelength light triggered testicular growth in eye-patched and pinealectomized quail (Nakane et al., 2010). Therefore, OPN5-expressing, CSF-contacting neurons in the PVO may be deep brain photoreceptors that are important for seasonal reproduction in birds (Figures 1, 2A).
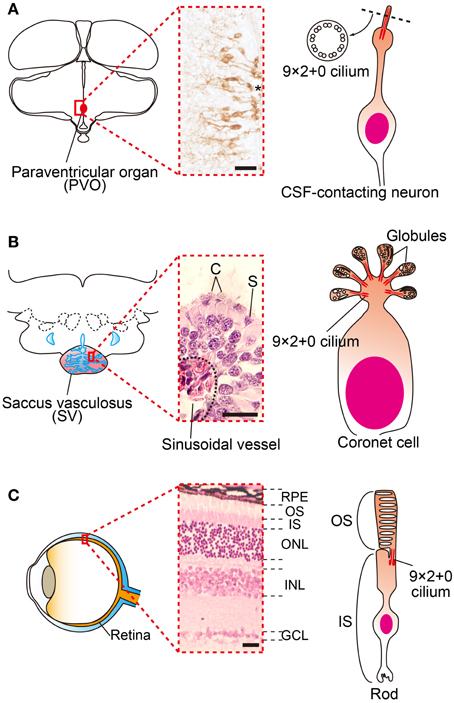
Figure 2. Family of cerebrospinal fluid (CSF)-contacting neurons. (A) Schematic drawings and picture of OPN5 positive CSF-contacting neurons in the paraventricular organ (PVO) of quail. (B) Schematic drawing and picture of coronet cells in the salmon saccus vasculosus (SV). The SV consists of coronet cells (C) and supporting cells (S). Globules of coronet cells are based on 9 × 2 + 0 cilia. (C) Schematic drawing and picture of a mammalian retina and photoreceptor. The outer segments of rod and cone cells are also based on 9 × 2 + 0 cilia. *third ventrile. RPE, retinal pigment epithelium, OS, outer segment, IS, inner segment, ONL, outer nuclear layer, INL inner nuclear layer, GCL, ganglion cell layer. Scale bars indicate 20 μm.
In summary, a series of quail studies have uncovered the signal transduction cascade that regulates seasonal reproduction, from photoreceptors to neuroendocrine output, in birds (Figure 1). That is, light information received by deep brain photoreceptors (e.g., OPN5, RH, OPN4, VA-opsin, etc.) is transmitted to the PT and long-day induced TSH secreted from the PT acts on TSHR to regulate DIO2/3 switching in the ECs. Bioactive T3 converted from T4 by DIO2 causes morphological changes in GnRH nerve terminals and glial processes in the ME, thereby regulating seasonal changes in GnRH secretion.
Signal Transduction Cascade for Seasonal Reproduction in Mammals
Thyroidectomy blocks the transition of seasonal reproductive state in sheep (Moenter et al., 1991), and it has been known for several decades that thyroid hormone is involved in the regulation of mammalian seasonality (Nicholls et al., 1988). However, its precise mode of action was unknown. After the discovery of photoperiodic DIO2/3 switching in birds, photoperiodic regulation of DIO2 and/or DIO3 within the MBH was reported in a number of mammalian species, such as hamsters (Watanabe et al., 2004, 2007; Revel et al., 2006; Barrett et al., 2007; Freeman et al., 2007; Yasuo et al., 2007a), rats (Yasuo et al., 2007b), mice (Ono et al., 2008) and even in short-day breeding sheep (Hanon et al., 2008) and goats (Yasuo et al., 2006). Therefore, local thyroid hormone activation within the MBH is considered to be central in the regulation of seasonal reproduction in mammals (Figure 3). However, in marked contrast with birds, the eye is the only photoreceptor organ. Light information is transmitted to the pineal gland through the circadian pacemaker, the suprachiasmatic nucleus (SCN). In mammals, photoperiodic information is decoded based on the duration of melatonin secretion by the pineal gland (Reiter, 1980; Yamazaki et al., 1999). Therefore, pinealectomy abolishes seasonal responses and melatonin administration mimics the effect of short photoperiod in mammals. Thus, melatonin is considered to play a deterministic role in mammalian seasonal reproduction (Reiter, 1980). Although melatonin controls DIO2/3 switching, melatonin receptors are absent in the ECs where DIO2/3 are expressed (Schuster et al., 2000; Song and Bartness, 2001). In contrast, melatonin receptors are densely expressed in the PT (Williams and Morgan, 1988; Wittkowski et al., 1988; Reppert et al., 1994; Klosen et al., 2002; Dardente et al., 2003). Therefore, it was predicted that TSH secreted from the PT may mediate the melatonin action to regulation of DIO2/3 switching in mammals. This hypothesis was tested using TSHR and melatonin receptor knockout mice (Ono et al., 2008; Yasuo et al., 2009). Melatonin administration had no effect on DIO2/3 switching in the TSHR and MT1 melatonin receptor null mice, whereas melatonin affected DIO2/3 switching in MT2 null mice. This suggests that melatonin acts on the MT1 melatonin receptor to regulate DIO2/3 switching through the TSH-TSHR signaling pathway in mammals (Figure 3).
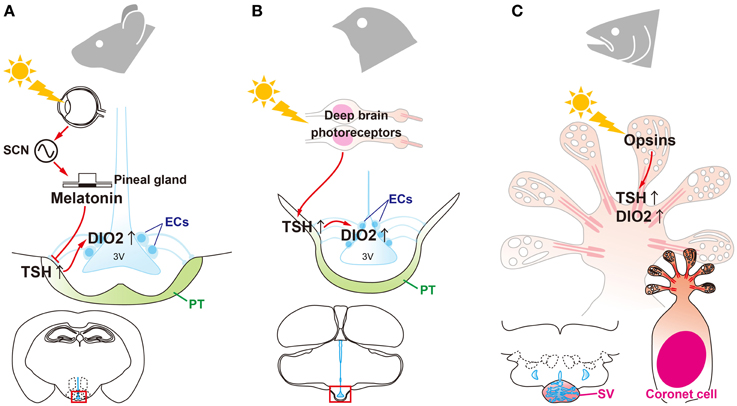
Figure 3. Universality and diversity of signal transduction pathways that regulate seasonal reproduction in vertebrates. (A) Eyes are the only photoreceptor organ in mammals. Light information is transmitted through the suprachiasmatic nucleus (SCN) to the pineal gland. Photoperiodic information is encoded by the pattern of melatonin secretion from the pineal gland. Melatonin regulates the “springtime hormone,” TSH, in the pars tuberalis (PT) of the pituitary gland. (B) In contrast to mammals, light information is directly received by deep brain photoreceptors in birds and is then transmitted to the PT to induce TSH. (C) In fish, all of the machinery required for seasonal reproduction (from photoreceptors to neuroendocrine output) is located in the saccus vasculosus (SV).
The RF-amides such as kisspeptin, a ligand for the G protein coupled receptor, GPR54, and RFamide-related peptide 3 (RFRP-3) are involved in the regulation of GnRH secretion (Clements et al., 2001; Kotani et al., 2001; Muir et al., 2001; Ohtaki et al., 2001; Clarke et al., 2008). Seasonal regulation of kisspeptin and RFRP-3 has been reported in hamsters (Revel et al., 2006, 2008). Administration of TSH to Djungarian and Syrian hamsters induces the expression of kisspeptin and RFRP-3 as well as gonadal development under short-day conditions (Klosen et al., 2013). T3 also provoked significant testicular growth and kisspeptin expression in Siberian hamsters (Phodopus sungorus) under short-day conditions (Henson et al., 2013). This suggests that long-day induces TSH and, following the activation of thyroid hormone by DIO2, regulates kisspeptin, RFRP-3 and the hypothalamic-pituitary-gonadal (HPG)-axis in mammalian species.
Signal Transduction Cascade for Seasonal Reproduction in Fish
Fish also show marked seasonal changes in physiology and behavior. Medaka (Oryzias latipes), are long-day seasonal breeders, and their gonads develop in response to elongated day-length (Koger et al., 1999). Salmonids, short-day seasonal breeders, show distinct photoperiodic responses, such as migration and parr-smolt transformation. Smoltification is closely linked to thyroid hormone (Robertson, 1949; Nishikawa et al., 1979). Although all fishes examined have had higher circulating levels of melatonin during the night than during the day, there are few reliable data consistent with a major physiological role for melatonin in the seasonal reproduction of fish (Urasaki, 1976; Garg, 1989; Masuda et al., 2005; Borg, 2010). This is in marked contrast to mammals, but is similar to birds. Fish do not have anatomically distinct PTs, a regulatory hub of seasonal reproduction in birds and mammals. Thus, the signal transduction pathway for fish seasonal reproduction remains unknown.
A recent study of masu salmon (Oncorhynchus masou masou) revealed that key elements for vertebrate seasonal reproduction, such as photopigments, TSH, TSHR, and DIO2, are expressed in the saccus vasculosus (SV). The SV is an organ only observed in fish and is located at the floor of the hypothalamus, posterior to the pituitary gland. Although its existence was first described in the 17th century (Collins, 1685), its physiological function remained a mystery for several centuries. In the SV, a folded EC layer makes a chamber that is directly connected to the third ventricle. Abundant sinusoidal vessels cover the whole external surface of the SV. The EC layer of the SV mainly consists of coronet cells and supporting cells (Sueiro et al., 2007). The coronet cells have morphologically specialized features; globules occupy the apical cellular structures of these cells (Figure 2B). Each globule has 9 × 2 + 0 cilia, as do photoreceptors in the retina (Figure 2C) and CSF-contacting neurons in the PVO (Figure 2A). The coronet cells also possess manifold primary vesicles (Jansen et al., 1982; Vigh and Vigh-Teichmann, 1998). Therefore, the coronet cells are considered to be CSF-contacting neurons.
Immunohistochemical analysis has revealed localization of photopigments (OPN4 and SWS1), TSH, and DIO2 in coronet cells (Nakane et al., 2013). The expression of these photoperiodic regulatory mechanisms within the SV implies that the SV plays a pivotal role as a seasonal sensor in fish. Indeed, isolated SVs respond to photoperiodic changes in in vitro and ablation of the SV prevents photoperiodically-induced gonadal development (Nakane et al., 2013). This suggests that coronet cells have multiple functions, including photoreception and neuroendocrine output (Figure 3).
Conclusion Remarks
The mechanisms of seasonal time measurement were a mystery for long time. However, recent studies have uncovered the signal transduction pathway that regulates seasonal reproduction in birds, mammals, and fish. These studies revealed the universality (i.e., signal transduction machineries) and diversity (responsible cells or organs) of these mechanisms among vertebrate species (Figure 3). This is similar to the structural and functional evolution of the pineal organ (Korf, 1994; Falcón et al., 2009). In non-mammalian vertebrates, the pinealocyte contains photoreceptors, the circadian clock, and neuroendocrine output in the form of melatonin. In marked contrast with non-mammalian vertebrates, the mammalian pinealocyte is specialized as a neuroendocrine organ for melatonin secretion. This is why the pineal organ is generally referred to as the pineal gland in mammals. As expressed by Ernst Haeckel's phrase “ontogeny recapitulates phylogeny,” the rat pineal gland responds to light during the postnatal period (Zweig et al., 1966; Tosini et al., 2000; Fukuhara and Tosini, 2003). Because multi-functionality is considered to be a general feature of ancient cell types (Arendt, 2008), coronet cells appear to be the ancestral vertebrate seasonal sensors.
Conflict of Interest Statement
The authors declare that the research was conducted in the absence of any commercial or financial relationships that could be construed as a potential conflict of interest.
Acknowledgments
This work is supported by the Funding Program for Next Generation World Leading Researchers (NEXT Program) initiated by the Council for Science and Technology Policy (CSTP) (LS055) and JSPS KAKENHI grant number 26000013. WPI-ITbM is supported by World Premier International Research Center Initiative (WPI), MEXT, Japan.
References
Arendt, D. (2008). The evolution of cell types in animals: emerging principles from molecular studies. Nat. Rev. Genet. 9, 868–882. doi: 10.1038/nrg2416
Barrett, P., Ebling, F. J., Schuhler, S., Wilson, D., Ross, A. W., Warner, A., et al. (2007). Hypothalamic thyroid hormone catabolism acts as a gatekeeper for the seasonal control of body weight and reproduction. Endocrinology 148, 3608–3617. doi: 10.1210/en.2007-0316
Benoit, J. (1935). Le role des yeux dans l'action stimulante de la lumiere sure le developpement testiulaire chez le canard. CR. Soc. Bio. (Paris.) 118, 669–671.
Bernal, J. (2005). Thyroid hormones and brain development. Vitam. Horm. 71, 95–122. doi: 10.1016/S0083-6729(05)71004-9
Borg, B. (2010). “Photoperiodism in fishes,” in Photoperiodism: The Biological Calendar, eds R. J. Nelson, D. L. Denlinger, and D. E. Somers (New York, NY: Oxford University Press), 371–398.
Chaurasia, S. S., Rollag, M. D., Jiang, G., Hayes, W. P., Haque, R., Natesan, A., et al. (2005). Molecular cloning, localization and circadian expression of chicken melanopsin (Opn4): differential regulation of expression in pineal and retinal cell types. J. Neurochem. 92, 158–170. doi: 10.1111/j.1471-4159.2004.02874.x
Clarke, I. J., Sari, I. P., Qi, Y., Smith, J. T., Parkington, H. C., Ubuka, T., Iqbal, J., et al. (2008). Potent action of RFamide-related peptide-3 on pituitary gonadotropes indicative of a hypophysiotropic role in the negative regulation of gonadotropin secretion. Endocrinology 149, 5811–5821. doi: 10.1210/en.2008-0575
Clements, M. K., McDonald, T. P., Wang, R., Xie, G., O'Dowd, B. F., George, S. R., et al. (2001). FMRFamide-related neuropeptides are agonists of the orphan G-protein-coupled receptor GPR54. Biochem. Biophys. Res. Commun. 284, 1189–1193. doi: 10.1006/bbrc.2001.5098
Dardente, H., Klosen, P., Pévet, P., and Masson-Pévet, M. (2003). MT1 melatonin receptor mRNA expressing cells in the pars tuberalis of the European hamster: effect of photoperiod. J. Neuroendocrinol. 15, 778–786. doi: 10.1046/j.1365-2826.2003.01060.x
Davies, D. T., and Follett, B. K. (1975). Electrical stimulation of the hypothalamus and luteinizing hormone secretion in Japanese quail. J. Endocrinol. 67, 431–443. doi: 10.1677/joe.0.0670431
Falcón, J., Besseau, L., Fuentès, M., Sauzet, S., Magnanou, E., and Boeuf, G. (2009). Structural and functional evolution of the pineal melatonin system in vertebrates. Ann. N. Y. Acad. Sci. 1163, 101–111. doi: 10.1111/j.1749-6632.2009.04435.x
Foster, R. G., and Follett, B. K. (1985). The involvement of a rhodopsin-like photopigment in the photoperiodic response of the Japanese quail. J. Comp. Physiol. A 157, 519–528. doi: 10.1007/BF00615153
Freeman, D. A., Teubner, B. J., Smith, C. D., and Prendergast, B. J. (2007). Exogenous T3 mimics long day lengths in Siberian hamsters. Am. J. Physiol. Regul. Integr. Comp. Physiol. 292, 2368–2372. doi: 10.1152/ajpregu.00713.2006
Fukuhara, C., and Tosini, G. (2003). Induction of photosensitivity in cultured rat pineal affects Aa-nat regulation. Brain Res. Dev. Brain Res. 142, 219–221. doi: 10.1016/S0165-3806(03)00067-1
Garg, S. K. (1989). Effect of pinealectomy, eye enucleation, and melatonin treatment on ovarian activity and vitellogenin levels in the catfish exposed to short photoperiod or long photoperiod. J. Pineal. Res. 7, 91–104. doi: 10.1111/j.1600-079X.1989.tb00658.x
Halford, S., Pires, S. S., Turton, M., Zheng, L., González-Menéndez, I., Davies, W. L., et al. (2009). VA opsin-based photoreceptors in the hypothalamus of birds. Curr. Biol. 19, 1396–1402. doi: 10.1016/j.cub.2009.06.066
Hanon, E. A., Lincoln, G. A., Fustin, J. M., Dardente, H., Masson-Pévet, M., Morgan, P. J., et al. (2008). Ancestral TSH mechanism signals summer in a photoperiodic mammal. Curr. Biol. 18, 1147–1152. doi: 10.1016/j.cub.2008.06.076
Hartwig, H. G., and van Veen, T. (1979). Spectral characteristics of visible radiation penetrating into the brain and stimulating extraretinal photoreceptors. J. Comp. Physiol. 130, 277–282. doi: 10.1007/BF00614615
Henson, J. R., Carter, S. N., and Freeman, D. A. (2013). Exogenous T3 elicits long day-like alterations in testis size and the RFamides Kisspeptin and gonadotropin-inhibitory hormone in short-day Siberian hamsters. J. Biol. Rhythms 28, 193–200. doi: 10.1177/0748730413487974
Homma, K., Ohta, M., and Sakakibara, Y. (1979). “Photoinducible phase of the Japanese quail detected by direct stimulation of the brain,” in Biological Rhythms and their Central Mechanism, eds M. Suda, O. Hayaishi, and H. Nakagawa (Amsterdam: Elsevier, North-Holland Biomedical Press), 85–94.
Jansen, W. F., Burger, E. H., and Zandbergen, M. A. (1982). Subcellular localization of calcium in the coronet cells and tanycytes of the saccus vasculosus of the rainbow trout, Salmo gairdneri. Ann. N. Y. Acad. Sci. 117, 217–227.
Klosen, P., Bienvenu, C., Demarteau, O., Dardente, H., Guerrero, H., Pévet, P., et al. (2002). The mt1 melatonin receptor and RORb receptor are co-localized in specific TSH-immunoreactive cells in the pars tuberalis of the rat pituitary. J. Histochem. Cytochem. 50, 1647–1657. doi: 10.1177/002215540205001209
Klosen, P., Sébert, M. E., Rasri, K., Laran-Chich, M. P., and Simonneaux, V. (2013). TSH restores a summer phenotype in photoinhibited mammals via the RF-amides RFRP3 and kisspeptin. FASEB J. 27, 2677–2686. doi: 10.1096/fj.13-229559
Koger, C. S., Teh, S. J., and Hinton, D. E. (1999). Variations of light and temperature regimes and resulting effects on reproductive parameters in medaka (Oryzias latipes). Biol. Reprod. 61, 1287–1293. doi: 10.1095/biolreprod61.5.1287
Konishi, H., Foster, R. G., and Follett, B. K. (1987). Evidence for a daily rhythmicity in the acute release of LH in response to electrical stimulation in the Japanese quail. J. Comp. Physiol. A 161, 315–319. doi: 10.1007/BF00615251
Korf, H. W. (1994). The pineal organ as a component of the biological clock. Phylogenetic and ontogenetic considerations. Ann. N. Y. Acad. Sci. 31, 13–42. doi: 10.1111/j.1749-6632.1994.tb56818.x
Kotani, M., Detheux, M., Vandenbogaerde, A., Communi, D., Vanderwinden, J. M., Le Poul, E., et al. (2001). The metastasis suppressor gene KiSS-1 encodes kisspeptins, the natural ligands of the orphan G protein-coupled receptor GPR54. J. Biol. Chem. 276, 34631–34636. doi: 10.1074/jbc.M104847200
Masuda, T., Iigo, M., and Aida, K. (2005). Existence of an extra-retinal and extra-pineal photoreceptive organ that regulates photoperiodism in gonadal development of an Osmerid teleost, ayu (Plecoglossus altivelis). Comp. Biochem. Physiol. A. Mol. Integr. Physiol. 140, 414–422. doi: 10.1016/j.cbpb.2005.01.004
Meddle, S. L., and Follett, B. K. (1995). Photoperiodic activation of fos-like immunoreactive protein in neurones within the tuberal hypothalamus of Japanese quail. J. Comp. Physiol. A. 176, 79–89. doi: 10.1007/BF00197754
Meddle, S. L., and Follett, B. K. (1997). Photoperiodically driven changes in Fos expression within the basal tuberal hypothalamus and median eminence of Japanese quail. J. Neurosci. 17, 8909–8918.
Menaker, M., Roberts, R., Elliott, J., and Underwood, H. (1970). Extraretinal light perception in the sparrow. III. The eyes do not participate in photoperiodic photoreception. Proc. Natl. Acad. Sci. U.S.A. 67, 320–325. doi: 10.1073/pnas.67.1.320
Moenter, S. M., Woodfill, C. J., and Karsch, F. J. (1991). Role of the thyroid gland in seasonal reproduction: thyroidectomy blocks seasonal suppression of reproductive neuroendocrine activity in ewes. Endocrinology 128, 1337–1344. doi: 10.1210/endo-128-3-1337
Muir, A. I., Chamberlain, L., Elshourbagy, N. A., Michalovich, D., Moore, D. J., Calamari, A., et al. (2001). AXOR12, a novel human G protein-coupled receptor, activated by the peptide KiSS-1. J. Biol. Chem. 276, 28969–28975. doi: 10.1074/jbc.M102743200
Nakane, Y., Ikegami, K., Iigo, M., Ono, H., Takeda, K., Takahashi, D., et al. (2013). The saccus vasculosus of fish is a sensor of seasonal changes in day length. Nat. Commun. 4, 2108. doi: 10.1038/ncomms3108
Nakane, Y., Ikegami, K., Ono, H., Yamamoto, N., Yoshida, S., Hirunagi, K., et al. (2010). A novel mammalian neural tissue opsin (Opsin5) is a deep brain photoreceptor in birds. Proc. Natl. Acad. Sci. U.S.A. 107, 15264–15268. doi: 10.1073/pnas.1006393107
Nakao, N., Ono, H., Yamamura, T., Anraku, T., Takagi, T., Higashi, K., et al. (2008). Thyrotrophin in the pars tuberalis triggers photoperiodic response. Nature 452, 317–311. doi: 10.1038/nature06738
Nicholls, T. J., Follett, B. K., Goldsmith, A. R., and Pearson, H. (1988). Possible homologies between photorefractoriness in sheep and birds: the effect of thyroidectomy on the length of the ewe's breeding season. Reprod. Nutr. Dev. 28, 375–385. doi: 10.1051/rnd:19880304
Nicholls, T. J., Follett, B. K., and Robinson, J. E. (1983). A photoperiodic response in gonadectomized Japanese quail exposed to a single long day. J. Endocrinol. 97, 121–126. doi: 10.1677/joe.0.0970121
Nishikawa, K., Hirashima, T., Suzuki, S., and Suzuki, M. (1979). Changes in circulating L-thyroxine and L-triiodothyronine of the masu salmon, Oncorhynchus masou accompaning the smoltification, measured by radioimmunoassay. Endocrinol. Jpn. 26, 731–735. doi: 10.1507/endocrj1954.26.731
Ohta, M., Wada, M., and Homma, K. (1984). Induction of rapid testicular growth in quail by phasic electrical stimulation of the hypothalamic photosensitive area. J. Comp. Physiol. A 154, 583–589. doi: 10.1007/BF00610171
Ohtaki, T., Shintani, Y., Honda, S., Matsumoto, H., Hori, A., Kanehashi, K., et al. (2001). Metastasis suppressor gene KiSS-1 encodes peptide ligand of a G-protein-coupled receptor. Nature 411, 613–617. doi: 10.1038/35079135
Oishi, T., and Ohashi, K. (1993). Effects of wavelengths of light on the photoperiodic gonadal response of blinded-pinealectomized Japanese quail. Zoolog. Sci. 10, 757–762.
Ono, H., Hoshino, Y., Yasuo, S., Watanabe, M., Nakane, Y., Murai, A., et al. (2008). Involvement of thyrotropin in photoperiodic signal transduction in mice. Proc. Natl. Acad. Sci. U.S.A. 105, 18238–18242. doi: 10.1073/pnas.0808952105
Reiter, R. J. (1980). The pineal and its hormones in the control of reproduction in mammals. Endocr. Rev. 1, 109–131. doi: 10.1210/edrv-1-2-109
Reppert, S. M., Weaver, D. R., and Ebisawa, T. (1994). Cloning and characterization of a mammalian melatonin receptor that mediates reproductive and circadian responses. Neuron 13, 1177–1185. doi: 10.1016/0896-6273(94)90055-8
Revel, F. G., Saboureau, M., Pévet, P., Mikkelsen, J. D., and Simonneaux, V. (2006). Melatonin regulates type 2 deiodinase gene expression in the Syrian hamster. Endocrinology 147, 4680–4687. doi: 10.1210/en.2006-0606
Revel, R. G., Saboureau, M., Pévet, P., Simonneaux, V., and Mikkelsen, J. D. (2008). RFamide-related peptide gene is a melatonin-driven photoperiodic gene. Endocrinology 149, 902–912. doi: 10.1210/en.2007-0848
Robertson, O. H. (1949). Production of the silvery smolt stage in rainbow trout by intramuscular injection of mammalian thyroid extract and thyrotropic hormone. J. Exp. Zool. 110, 337–355 doi: 10.1002/jez.1401100303
Schuster, C., Gauer, F., Guerrero, H., Lakhdar-Ghazal, N., Pévet, P., and Masson-Pévet, M. (2000). Photic regulation of mt1 melatonin receptors in the Siberian hamster pars tuberalis and suprachiasmatic nuclei: involvement of the circadian clock and intergeniculate leaflet. J. Neuroendocrinol. 12, 207–216. doi: 10.1046/j.1365-2826.2000.00039.x
Sharp, P. J., and Follett, B. K. (1969). The effect of hypothalamic lesions on gonadotrophin release in Japanese quail (Coturnix coturnix japonica). Neuroendocrinology 5, 205–218. doi: 10.1159/000121861
Silver, R., Witkovsky, P., Horvath, P., Alones, V., Barnstable, C. J., and Lehman, M. N. (1988). Coexpression of opsin- and VIP-like-immunoreactivity in CSF-contacting neurons of the avian brain. Cell Tissue Res. 253, 189–198. doi: 10.1007/BF00221754
Siopes, T. D., and Wilson, W. O. (1974). Extraocular modification of photoreception in intact and pinealectomized coturnix. Poult. Sci. 53, 2035–2041. doi: 10.3382/ps.0532035
Song, C. K., and Bartness, T. J. (2001). CNS sympathetic outflow neurons to white fat that express MEL receptors may mediate seasonal adiposity. Am. J. Physiol. Regul. Integr. Comp. Physiol. 281, R666–R672.
Sueiro, C., Carrera, I., Ferreiro, S., Molist, P., Adrio, F., Anadón, R., et al. (2007). New insights on saccus vasculosus evolution: a developmental and immunohistochemical study in Elasmobranchs. Brain Behav. Evol. 70, 187–204. doi: 10.1159/000104309
Tosini, G., Doyle, S., Geusz, M., and Menaker, M. (2000). Induction of photosensitivity in neonatal rat pineal gland. Proc. Natl. Acad. Sci. U.S.A. 97, 11540–11544. doi: 10.1073/pnas.210248297
Urasaki, H. (1976). The role of pineal and eyes in the photoperiodic effect on the gonad of the medaka, Oryzias latipes. Chronobiologia 3, 228–234.
Vigh, B., and Vigh-Teichmann, I. (1998). Actual problems of the cerebrospinal fluid-contacting neurons. Microsc. Res. Tech. 41, 57–83.
Vigh-Teichmann, I., Röhlich, P., Vigh, B., and Aros, B. (1980). Comparison of the pineal complex, retina and cerebrospinal fluid contacting neurons by immunocytochemical antirhodopsin reaction. Z. Mikrosk. Anat. Forsch. 94, 623–640.
von Frisch, K. (1911). Beitrage zur physiologie der pigmentzellen in der fischhaut. Pflü. Arch. Gesammte. Physiol. Menschen. Tiere. 138, 319–387. doi: 10.1007/BF01680752
Wada, Y., Okano, T., Adachi, A., Ebihara, S., and Fukada, Y. (1998). Identification of rhodopsin in the pigeon deep brain. FEBS Lett. 424, 53–56. doi: 10.1016/S0014-5793(98)00138-0
Watanabe, M., Yasuo, S., Watanabe, T., Yamamura, T., Nakao, N., Ebihara, S., et al. (2004). Photoperiodic regulation of type 2 deiodinase gene in Djungarian hamster: Possible homologies between avian and mammalian photoperiodic regulation of reproduction. Endocrinology 145, 1546–1549. doi: 10.1210/en.2003-1593
Watanabe, T., Yamamura, T., Watanabe, M., Yasuo, S., Nakao, N., Dawson, A., et al. (2007). Hypothalamic expression of thyroid hormone-activating and -inactivating enzyme genes in relation to photorefractoriness in birds and mammals. Am. J. Physiol. Regul. Integr. Comp. Physiol. 292, 568–572. doi: 10.1152/ajpregu.00521.2006
Williams, L. M., and Morgan, P. J. (1988). Demonstration of melatonin-binding sites on the pars tuberalis of the rat. J. Endocrinol. 119, 1–3. doi: 10.1677/joe.0.119R001
Wittkowski, W., Bergmann, M., Hoffmann, K., and Pera, F. (1988). Photoperiod-dependent changes in TSH-like immunoreactivity of cells in the hypophysial pars tuberalis of the Djungarian hamster, Phodopus sungorus. Cell Tissue Res. 251, 183–187. doi: 10.1007/BF00215463
Yamamura, T., Yasuo, S., Hirunagi, K., Ebihara, S., and Yoshimura, T. (2006). T3 implantation mimics photoperiodically reduced encasement of nerve terminals by glial processes in the median eminence of Japanese quail. Cell Tissue Res. 324, 175–179. doi: 10.1007/s00441-005-0126-8
Yamashita, T., Ohuchi, H., Tomonari, S., Ikeda, K., Sakai, K., and Shichida, Y. (2010). Opn5 is a UV-sensitive bistable pigment that couples with Gi subtype of G protein. Proc. Natl. Acad. Sci. U.S.A. 107, 22084–22089. doi: 10.1073/pnas.1012498107
Yamazaki, S., Goto, M., and Menaker, M. (1999). No evidence for extraocular photoreceptors in the circadian system of the Syrian hamster. J. Biol. Rhythms 14, 197–201. doi: 10.1177/074873099129000605
Yasuo, S., Nakao, N., Ohkura, S., Iigo, M., Hagiwara, S., Goto, A., et al. (2006). Long-day suppressed expression of type 2 deiodinase gene in the mediobasal hypothalamus of the Saanen goat, a short-day breeder: Implication for seasonal window of thyroid hormone action on reproductive neuroendocrine axis. Endocrinology 147, 432–440. doi: 10.1210/en.2005-0507
Yasuo, S., Watanabe, M., Iigo, M., Nakamura, T. J., Watanabe, T., Takagi, T., et al. (2007b). Differential response of type 2 deiodinase gene expression tophotoperiod between photoperiodic Fischer 344 and nonphotoperiodic Wistar rats. Am. J. Physiol. Regul. Integr. Comp. Physiol. 292, 1315–1319. doi: 10.1152/ajpregu.00396.2006
Yasuo, S., Watanabe, M., Nakao, N., Takagi, T., Follett, B. K., Ebihara, S., et al. (2005). The reciprocal switching of two thyroid hormone-activating and -inactivating enzyme genes is involved in the photoperiodic gonadal response of Japanese quail. Endocrinology 146, 2551–2554. doi: 10.1210/en.2005-0057
Yasuo, S., Yoshimura, T., Ebihara, S., and Kolf, H. W. (2009). Melatonin transmits photoperiodic signals through the MT1 melatonin receptor. J. Neurosci. 29, 2885–2889. doi: 10.1523/JNEUROSCI.0145-09.2009
Yasuo, S., Yoshimura, T., Ebihara, S., and Korf, H. W. (2007a). Temporal dynamics of type 2 deiodinase expression after melatonin injections in Syrian hamsters. Endocrinology 148, 3608–3617. doi: 10.1210/en.2007-0497
Yokoyama, K., Oksche, A., Darden, T. R., and Farner, D. S. (1978). The sites of encephalic photoreception in phosoperiodic induction of the growth of the testes in the white-crowned sparrow. Zonotrichia leucophrys gambelii. Cell Tissue Res. 189, 441–467. doi: 10.1007/BF00209132
Yoshimura, T., Yasuo, S., Watanabe, M., Iigo, M., Yamamura, T., Hirunagi, K., et al. (2003). Light-induced hormone conversion of T4 to T3 regulates photoperiodic response of gonads in birds. Nature 426, 178–181. doi: 10.1038/nature02117
Keywords: circadian rhythm, pars tuberalis, saccus vasculosus, deep brain photoreceptor, thyroid-stimulating hormone, thyroid hormone, cerebrospinal fluid-contacting neuron, coronet cell
Citation: Nakane Y and Yoshimura T (2014) Universality and diversity in the signal transduction pathway that regulates seasonal reproduction in vertebrates. Front. Neurosci. 8:115. doi: 10.3389/fnins.2014.00115
Received: 20 February 2014; Paper pending published: 26 March 2014;
Accepted: 01 May 2014; Published online: 21 May 2014.
Edited by:
Ishwar Parhar, Monash University, MalaysiaReviewed by:
Valerie Simonneaux, Centre National de la Recherche Scientifique, FranceEric Fliers, University of Amsterdam, Netherlands
Copyright © 2014 Nakane and Yoshimura. This is an open-access article distributed under the terms of the Creative Commons Attribution License (CC BY). The use, distribution or reproduction in other forums is permitted, provided the original author(s) or licensor are credited and that the original publication in this journal is cited, in accordance with accepted academic practice. No use, distribution or reproduction is permitted which does not comply with these terms.
*Correspondence: Takashi Yoshimura, Institute of Transformative Bio-Molecules (WPI-ITbM), Nagoya University, Furo-cho, Chikusa-ku, Nagoya 464-8601, Japan e-mail:dGFrYXNoaXlAYWdyLm5hZ295YS11LmFjLmpw