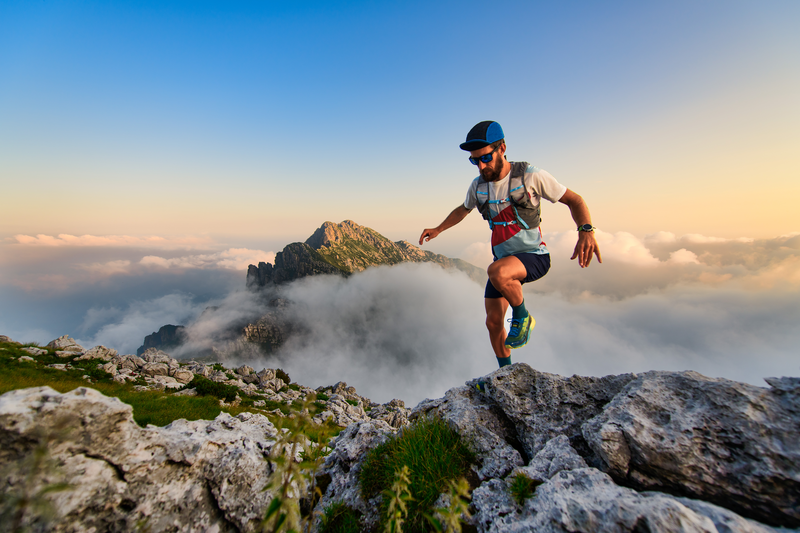
94% of researchers rate our articles as excellent or good
Learn more about the work of our research integrity team to safeguard the quality of each article we publish.
Find out more
PERSPECTIVE article
Front. Neurol.
Sec. Multiple Sclerosis and Neuroimmunology
Volume 16 - 2025 | doi: 10.3389/fneur.2025.1571605
The final, formatted version of the article will be published soon.
You have multiple emails registered with Frontiers:
Please enter your email address:
If you already have an account, please login
You don't have a Frontiers account ? You can register here
Multiple sclerosis (MS) is a chronic central nervous system disease characterized by neurodegeneration and inflammation. Neurofilament light chain (NfL), a protein released during axonal injury, has gained recognition as a potential biomarker for monitoring MS progression and treatment response. Evidence indicates that blood NfL (bNfL) offers a minimally invasive, costeffective tool for tracking neuroaxonal damage. Regular bNfL assessments can identify subclinical disease activity, guide treatment intensification, and support individualized care. However, bNfL level evaluation is currently not optimized in Italian clinical practice. This work examines the utility of bNfL monitoring in clinical practice, focusing on optimizing its use within specific patient profiles, especially in resource-limited settings. bNfL testing, particularly in targeted MS patient profiles, including stable patients exhibiting subclinical signs of disease activity, such as fatigue, and patients off-treatment, represents a promising adjunct for personalized disease management. Its integration into clinical practice, alongside MRI and clinical assessments, can enhance decision-making and improve care efficiency, especially in settings with limited MRI resources. Further research is needed to standardize testing protocols and establish disease-specific cutoffs.
Keywords: neurofilament light chain, blood NfL, Multiple Sclerosis, MS biomarker, clinical practice
Received: 05 Feb 2025; Accepted: 11 Mar 2025.
Copyright: © 2025 Centonze, Di Sapio, Brescia Morra, Colombo, Inglese, Paolicelli, Salvetti and Furlan. This is an open-access article distributed under the terms of the Creative Commons Attribution License (CC BY). The use, distribution or reproduction in other forums is permitted, provided the original author(s) or licensor are credited and that the original publication in this journal is cited, in accordance with accepted academic practice. No use, distribution or reproduction is permitted which does not comply with these terms.
* Correspondence:
Diego Centonze, University of Rome Tor Vergata, Roma, 00173, Lazio, Italy
Disclaimer: All claims expressed in this article are solely those of the authors and do not necessarily represent those of their affiliated organizations, or those of the publisher, the editors and the reviewers. Any product that may be evaluated in this article or claim that may be made by its manufacturer is not guaranteed or endorsed by the publisher.
Research integrity at Frontiers
Learn more about the work of our research integrity team to safeguard the quality of each article we publish.