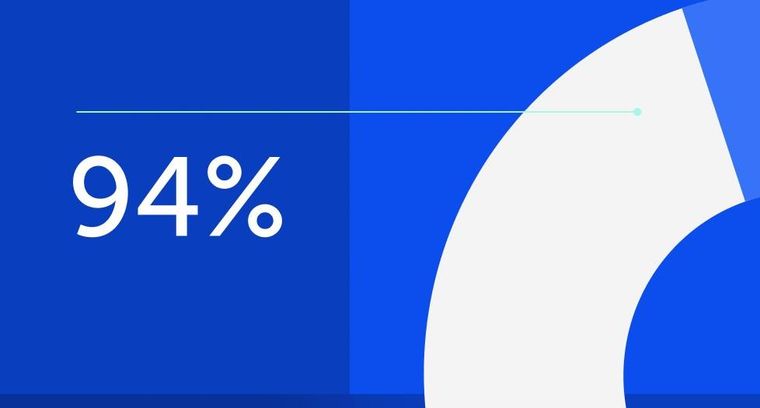
94% of researchers rate our articles as excellent or good
Learn more about the work of our research integrity team to safeguard the quality of each article we publish.
Find out more
REVIEW article
Front. Neurol., 11 April 2025
Sec. Neurorehabilitation
Volume 16 - 2025 | https://doi.org/10.3389/fneur.2025.1563681
Transcutaneous Electrical Acupoint Stimulation (TEAS) is a non-invasive therapeutic approach that combines modern electrophysiological technology with traditional Chinese medicine principles. Over the past few decades, TEAS has become a significant medical intervention during the perioperative period and its mechanisms have seen a continuous development. These mechanisms span across multiple aspects, including the regulation of the neuroendocrine network system, inhibition of oxidative stress, alleviation of immune suppression, regulation of endothelial function, and inhibition of coagulation and fibrinolysis system activation. This article aims to summarize and elaborate the mechanisms of TEAS in the perioperative period and to discuss current research challenges. Future studies should strive to optimize treatment protocols to promote the research on the mechanisms of TEAS in the perioperative period, subsequently offering patients safer and more effective treatment alternatives.
The perioperative period refers to the duration from decision to proceed with surgery to the completion of all related treatments, including preoperative, intraoperative, and postoperative phases (1). TEAS is a therapeutic approach that combines modern electrophysiological technologies with the principles traditional acupuncture. Moreover, the theoretical foundation of TEAS is rooted in the traditional Chinese medical concepts of meridians and acupuncture points, as further strengthened in the ancient medical text, Huangdi Neijing, in terms of its significance in sustaining physiological equilibrium. Currently, TEAS is widely used in the perioperative period, demonstrating considerable therapeutic efficacy. Specifically, a large amount of research have shown the application of TEAS in alleviating various perioperative symptoms, such as surgical pain, postoperative cognitive dysfunction (POCD), gastrointestinal issues, and sleep disturbances (2, 3), mainly used for preoperative rehabilitation, intraoperative anesthesia cooperation and postoperative complications treatment.
With the increasing understanding of its clinical efficacy, researchers have begun to focus more on the mechanisms of TEAS during the perioperative period. Studies have indicated that TEAS could inhibit inflammatory responses, modulate the release of endogenous analgesic substances, and modify the stress responses during the perioperative period (4–6). Nevertheless, a comprehensive review on this topic is still marginal. Therefore, to fill this research gap, this article aims to summarize and specify the mechanisms of TEAS in the perioperative period, so as to provide a theoretical basis and treatment strategies for promoting the clinical application of TEAS during the perioperative period.
Opiorphin, a natural opioid peptide found in the spinal cord, serves as a potent analgesic. In a randomized single-blind clinical trial, during the perioperative period, the application of TEAS could promotes the expression of opiorphin. Through the opioid system, TEAS inhibits the degradation of encephalin by neutral endopeptidase and aminopeptidase N in patients undergoing general anesthesia for colorectal surgery, thereby enhancing the analgesic effect of encephalin and achieving a sedative clinical outcome (4). What’s more, β-Endorphin (β-EP), widely distributed in the central nervous system, acts as an opioid receptor agonist and plays a crucial role in pain regulation. Its increase will enhance the body’s analgesic response. During the investigation on the analgesic effect of TEAS during labor, it was observed that TEAS could elevate the plasma β-EP levels and enhance analgesic effect (7).
Serotonin (5-hydroxytryptamine, 5-HT) and prostaglandin E2 are predominantly secreted by injured tissues and may directly act on nociceptive sensory neurons, significantly impacting pain generation and conduction of pain. Furthermore, the application of TEAS at a frequency of 2/100 Hz as an adjunct to anesthesia could inhibit the secretion of 5-HT and prostaglandin E2 by various cells in patients undergoing thoracic surgery. This reduces the transmission of noxious stimuli to the central nervous system, activates analgesic mechanisms, and facilitates the release of opioid peptides such as β-EP, thereby alleviating pain in the patients (5).
Moreover, 5-HT plays a role in the regulation of gastrointestinal motility and several endocrine functions. Emetic molecules such as 5-HT which will increase under trauma or anesthesia, could directly stimulate the vomiting center of medulla oblongata, or activate the peripheral receptors in the gastrointestinal mucosa (8). A randomized controlled clinical trial proved that TEAS could inhibit the release of 5-HT in the central nervous system of patients undergoing gastric cancer surgery, resulting in improved POGD and reduced postoperative pain (8). Furthermore, TEAS might decrease the levels of vasoactive intestinal peptide, somatostatin, and neurotensin in patients undergoing gastrointestinal surgery, while simultaneously increasing the levels of motilin, gastrin, cholecystokinin, and ghrelin. These effects facilitate the recovery of gastrointestinal function and indirectly alleviate discomfort in patients after gastric cancer surgery (9, 10).
Increased levels of serum S-100β and neuron-specific enolase, along with a decrease in brain-derived neurotrophic factor, serve as indicators of neural cell injury and are closely associated with POCD (11). TEAS treatment at a frequency of 2/100 Hz could inhibit the levels of neuron-specific enolase and S100β protein in the serum of elderly patients undergoing total knee arthroplasty. What’s more, the level of brain-derived neurotrophic factor may be enhanced, improving neurometabolic function and having a positive effect on the prevention of POCD (12).
Tau protein, a microtubule-associated protein, is essential for maintaining the stability of microtubules within axons. Generally, after a single-port thoracoscopic lobectomy in elderly patients, the level of tau protein (p-tau) is abnormally raised. Consequently, the formation of neurofibrillary tangles within the brain parenchyma is accelerated, which is closely related to the formation of cognitive impairment. Accordingly, researchers have identified that TEAS is able to reduce serum p-tau levels in elderly patients, thereby improving POCD (13).
Melatonin is a facilitator of the memory process, and its reduction is associated with damage to the olfactory epithelial nerves (14). During the perioperative period, the use of sevoflurane anesthesia could lead to short-term olfactory recognition impairment, memory decline, and a decrease in melatonin levels (15). The application of TEAS in patients under sevoflurane anesthesia has been investigated to increase melatonin levels and aid in the recovery of olfactory memory. Furthermore, plasma melatonin plays a role in guiding sleep. A study on TEAS indicated that stimulation at Shenmen (HT7), Sanyinjiao (SP6), and Neiguan (PC6) can increase perioperative plasma melatonin concentrations among 30 living kidney donors, thereby reducing the incidence of postoperative sleep disturbances (16).
Autonomic imbalance during the perioperative period may lead to POGD, characterized by enhanced sympathetic excitability and reduced parasympathetic or vagal nerve activity postoperatively (17). The sympathetic nervous system inhibits gastric motility and emptying by releasing adrenaline, whereas the vagus nerve promotes gastric motility and emptying through the release of epinephrine (EPI) (18). Consequently, a decrease in digestive function may result from reduced parasympathetic nerve function weakening gastrointestinal peristalsis and increased sympathetic nerve excitability inhibiting gastrointestinal motility (17). Furthermore, heart rate variability analysis primarily reflects autonomic nervous regulation. Low-frequency power (LF) reflects sympathetic nervous activity, while high-frequency power (HF) reflects parasympathetic or vagal nerve activity. The LF/HF ratio indicates the balance between these two systems. The root mean square of successive R-R interval differences reflects parasympathetic nervous tension, while the standard deviation of 24-h R-R intervals reflects the combined effects of parasympathetic and sympathetic nervous activity (19). To sum up, through reducing LF/HF, increasing the patient’s 24-h R-R interval standard deviation, HF, and the root mean square of the successive R-R interval difference, TEAS could be utilized to reduce sympathetic nerve excitability in the early postoperative phase, restore parasympathetic nerve function, and ultimately prevent POGD (17, 20).
Early inflammatory responses during the perioperative period are risk factors for postoperative pulmonary complications, with patients undergoing surgeries closer to the chest and abdomen being more likely to develop such complications (6, 21). What’s more, inflammatory mediators such as tumor necrosis factor-alpha (TNF-α), interleukin-1 (IL-1), and C-reactive protein (CRP) are positively correlated with the degree of inflammation. Accordingly, TEAS could be adopted to reduce the level of inflammatory factors, thereby decreasing the severity of inflammation. In particular, the application of TEAS at Gegu (LI4), Neiguan (PC6), Houxi (SI3), and Zhigou (SJ6) could reduce IL-6 and IL-10 levels in patients undergoing thoracoscopic lung lobectomy, alleviating inflammatory responses caused by single-lung ventilation and preventing pulmonary complications (6). Similarly, TEAS at a frequency of 2/100 Hz may lower TNF-α, IL-6, and CRP levels in esophageal cancer patients, reducing the risk of postoperative pulmonary complications and inflammation (21). Additionally, laparoscopic colorectal surgery is frequently influenced by the stimulation of the inflammatory response, potentially resulting in postoperative complications, including impaired gastrointestinal function and intestinal paralysis (22). In a randomized, double-blind clinical trial, involving 30 colorectal cancer patients undergoing radical surgery, researchers applied TEAS at a frequency of 2 Hz. This treatment reduced the patients’ levels of TNF-α, IL-1, and IL-6, thereby mitigating the inflammatory response (23).
In perioperative patients, stress reactions may result in the production of excessive reactive oxygen species, leading to cellular dysfunction and ultimately causing irreversible cell damage and death (24). During the process, patients may exhibit clinical manifestations such as blood pressure fluctuations and sleep disturbances, which may negatively affect postoperative recovery (25, 26). Additionally, the human body could generate various stress factors, including norepinephrine, EPI, adrenocorticotropic hormone, angiotensin II, cortisol (Cor), and CRP (27). The application of TEAS in patients undergoing laparoscopic cholecystectomy has been shown to significantly reduce the levels of NE, EPI, angiotensin II, CRP, and Cor, thereby alleviating the stress response (28, 29). Furthermore, the application of TEAS prior to anesthesia induction in hypertensive patients may mitigate the increase in adrenocorticotropic hormone and Cor, thereby aiding in the maintenance of circulatory stability and diminishing the stress response (25).
Secretory immunoglobulin A (SIgA) is an important salivary immune molecule that plays a key role in mucosal immunity. Trauma induces a stress response in the body, which alters mucosal immune function and increases SIgA concentration. Salivary amylase (sAA) is a specific indicator of stress. The level of sAA is directly proportional to the intensity of pain and the degree of discomfort caused by pain. Postoperative patients often experience varying degrees of tension and stress. During general anesthesia for colorectal surgery, the application of TEAS has been shown to reduce levels of SIgA, sAA, and Cor, effectively alleviating the stress response (4).
In the early postoperative period, patients frequently undergo sleep disturbances, which lead to an increase in reactive oxygen species and subsequently trigger oxidative stress. This process results in a decreased expression of nuclear factor erythroid 2-related factor 2 (Nrf2), a key regulator of oxidative stress. Nrf2 plays a crucial role in mitigating oxidative damage by activating antioxidant response elements and upregulating antioxidant enzymes such as superoxide dismutase (26). It has been demonstrated that Nrf2 is activated when TEAS is administered to patients undergoing surgery for gastrointestinal tumors. As a result, glutathione peroxidase 3 and superoxide dismutase could be expressed at higher levels. Additionally, TEAS could improve sleep quality, as evidenced by decreased Pittsburgh Sleep Quality Index and Athens Insomnia Scale scores. The mechanism may be related to the activation of the Nrf2/ARE signaling pathway and a reduction in the stress response (26).
Endothelin (ET) has vasoconstrictive and blood pressure-raising effects and can serve as an indicator of the intensity of the stress response (30). The application of TEAS during surgical anesthesia in elderly orthopedic patients could stabilize ET levels, thereby reducing intraoperative stress responses (31).
The activation and differentiation of T lymphocytes are crucial for the human body’s defense against infections and tumors (32). Cancer patients are commonly immunocompromised after surgical treatment, characterized by elevated CD8+ levels and decreased CD3+ and CD4+ levels. In a randomized controlled clinical trial, TEAS has been shown to reduce plasma CD8+ levels and increase plasma CD3+, CD4+, and the CD4+/CD8+ ratio, thereby enhancing the human body’s immune function (10, 33). In addition, the imbalance between T helper cells (Th1, Th2, Th17) and regulatory T cells (Treg) is mainly associated with immune dysregulation (34). Th1 cells counteract tumors by releasing immune cytokines such as γ-interferon (35). However, Th2 cells primarily participate in antibody-mediated immune responses. They collectively uphold the equilibrium of the immune system. Furthermore, Th17 cells and Treg cells have been defined as two distinct CD4+ T cell subsets derived from Th1 and Th2 cells (34). The expression levels of Th17-associated cytokines in patients with lung cancer were decreased following thoracotomy, which may have promoted an imbalance in numbers of Th1, Th2, Th17 and Treg cells, and contributed to postoperative immunosuppression. The use of TEAS during the perioperative period has been shown to increase Th1 and Th17 cell levels in lung cancer patients, promote the expression of related transcription factors, and enhance γ-interferon expression. Moreover, TEAS could reduce Th2 cells and decrease the expression of related transcription factors, partially restoring the balance of cell proportions, which may contribute to attenuating postoperative immunosuppression in lung cancer patients (35).
Alterations in the production and availability of endothelial-derived nitric oxide (NO), endothelin (ET), and von Willebrand factor (vWF) are referred to as endothelial dysfunction and are considered a critical initiating factor in coronary artery disease. Among these, ET-1 and NO are vasoactive substances that regulate vasoconstriction and vasodilation, respectively, whereas vWF serves as an auxiliary factor in hemostasis. After percutaneous coronary intervention (36), endothelial cells are damaged, leading to a decrease in NO secretion and an increase in the levels of ET-1 and vWF. The application of TEAS at a frequency of 4 Hz during the perioperative period has been shown to suppress ET-1 and vWF levels in percutaneous coronary intervention patients, increase serum NO levels, and effectively improve endothelial function, thereby reducing (36).
A large number of studies have indicated that endothelial dysfunction and inflammatory responses could interact to form a vicious cycle, further exacerbating vascular damage and disease progression (36). Specifically, the inflammatory mediators act on the vascular endothelial cells, resulting in rearrangement of the actin filaments in the cytoskeleton, which subsequently induces cell contraction and morphological alterations (37). What’s more, the contraction of endothelial cells leads to the widening of intercellular gaps, which further facilitates the leakage of inflammatory mediators (38). In addition, CRP, as a highly sensitive and specific inflammatory marker, not only interacts with endothelial cells but also accelerates the vascular inflammatory process, leading to coronary plaque rupture. In the same clinical trial, TEAS not only regulated endothelial dysfunction but also modulated the levels of the inflammatory factor CRP. This suggests that TEAS may exert its therapeutic effects by simultaneously improving endothelial function and inhibiting the inflammatory response (36).
Additionally, endothelin (ET), as a vasoconstrictive peptide, is involved in the pathological processes of central nervous system damage. Calcitonin gene-related peptide (CGRP) could dilate cerebral vessels or counteract the vasoconstrictive effects of ET. ET and CGRP jointly maintain the vasomotor function of the cerebral vessels. Generally, after craniotomy, ET levels could be elevated, leading to an imbalance between CGRP and ET levels. Accordingly, the use of TEAS has demonstrated a reduction in ET levels among craniotomy patients, regulation of the CGRP/ET balance, and enhancement of microcirculation in brain tissue, thus offering protective effects against perioperative brain injury during neurosurgery (39).
When the damaged endothelial cells lose the physiological regulation mechanism of coagulation, the dynamic balance between coagulation, anticoagulation and fibrinolysis will be destroyed. This leads to hypercoagulability, intravascular microthrombosis, and disseminated intravascular coagulation (40). In this process, endothelial damage activates coagulation factor XII, triggering the intrinsic coagulation pathway. Endotoxins or tissue injury release tissue factor, which binds to and activates coagulation factor VII, thereby activating the extrinsic coagulation pathway and promoting thrombus formation (41). Furthermore, shortened prothrombin time, activated partial thromboplastin time, and elevated levels of D-dimer, fibrinogen, and cell aggregation are associated with an increased risk of thrombosis. The application of TEAS in perioperative patients for the prevention of deep vein thrombosis in the lower limbs could prolong prothrombin time and activate partial thromboplastin time, increase platelet count, and reduce D-dimer, fibrinogen, and red blood cell aggregation indices. These effects help alleviate the hypercoagulable state and improve blood circulation in the lower limbs (42, 43).
With extensive clinical research deepen, the therapeutic effects of TEAS during the perioperative period (3, 44, 45). To be specific, TEAS could be beneficial in several aspects, including anti-inflammatory, analgesic, stress-reducing, immune-enhancing effects, prevention of POCD, gastrointestinal regulation, and improvement of lower limb circulation (2). In addition, the mechanisms underlying these effects primarily focus on inhibiting inflammation, regulating the neuroendocrine system, modulating oxidative stress, alleviating immune suppression, improving endothelial function, and anticoagulation (A detailed summary is shown in Figure 1). These mechanisms play a crucial role in improving patient symptoms and enhancing overall prognosis during the perioperative period.
Figure 1. The mechanism of transcutaneous electrical acupoint stimulation in the perioperative period. OPI, Opiorphin protein; β-EP, β-Endorphin; 5-HT, 5-Hydroxytryptamine; PGE2, Prostaglandin E2; NSE, Neuron-specific enolase; S100β, Central nervous system specific protein β; p-tau, Phosphorylated Tau Protein; BDNF, Brain-derived neurotrophic factor; MT, Melatonin; SDNN, The standard deviation of the 24-h R-R interval; RMSSD, The root mean square of the adjacent R-R interval difference; HF, High-frequency power; LF, Low-frequency power; TNF-α, Tumor necrosis factor-α; IL-1, Interleukin-1; IL-6, Interleukin-6; IL-10, Interleukin-10; CRP, C-reactive protein; GPx-3, Glutathione peroxidase 3; SOD, Superoxide dismutase; NE, Norepinephrine; EPI, Epinephrine; AngII, Angiotensin-II; ACTH, Adrenocorticotropic hormone; Cor, Correlate; CRP, C-reactive protein; SIgA, Secretory immunoglobulin A; sAA, Salivary amylase; ET, Endothelin; Nrf2, Nuclearfactor erythroidderived 2-like 2; CD8+, Cluster of Differentiation 8 positive; CD3+, Cluster of Differentiation 3 positive; CD4+, Cluster of Differentiation 4 positive; Th17, Th17 cell; Th1, Th1 cell; IFN-γ, Interferon-γ; Th2, Th2 cell; ET-1, Endothelin-1; vWF, von Willebrand factor; NO, Nitric oxide; ET, Endothelin; PT - Prothrombin time; APTT, Activate partial thromboplastin time; PLT, Platelet count; FIB, Fibrinogen; AI, erythrocyte aggregation indices.
Although TEAS holds enormous promise for perioperative applications, its mechanism is still being developed. There still remains significant potential for advancement in mechanistic research. In particular, it could be manifested in the following aspects: (1) The mechanisms in the perioperative period typically involve the interconnection of inflammation, endothelial dysfunction, oxidative stress, and autonomic nervous system dysregulation. Studies have shown that increased sympathetic excitability may promote the occurrence of inflammation. However, current research on TEAS mechanisms tends to focus on a single aspect. Whether the suppression of perioperative inflammation by TEAS is closely related to autonomic nervous system regulation require further investigation. Clinically, trials of TEAS for perioperative patients may be designed to examine the impact of different autonomic nerve stimulation intensities on inflammation. Additionally, the pathological mechanisms of perioperative diseases are also associated with neuroinflammation, the release of harmful neurotransmitters, the activation mechanisms of microglia (46) and the expression of NLRP3 inflammasome (47). Specifically, microglia play a crucial role in neuroinflammation. In the state of neuroinflammation, they promote the release of inflammatory cytokines and trigger immune-inflammatory responses. NLRP3 inflammasome is one of the most typical inflammasomes involved in the inflammatory response, which may induce the activation of Caspase-1 and accelerate the maturation of pro-inflammatory cytokines such as IL-1β and IL-18. Nevertheless, whether TEAS could modulate these mechanisms remains to be explored in future studies. (2) The frequency of electrical stimulation in TEAS is a critical factor for its therapeutic effects, but there is still considerable debate regarding parameter selection. At present, commonly used frequencies in clinical studies include 2/100 Hz (15), 2 Hz (23), 100 Hz (48), and 4 Hz (36) (for more information on experimental design in literature, see supplementary documents). Some studies indicate that using a 2/100 Hz sparse-dense wave pattern during general anesthesia may better reduce stress responses in patients, increase postoperative motilin levels, and accelerate recovery (3). However, other studies reveal that 100 Hz TEAS is effective in alleviating postoperative nausea and vomiting symptoms in patients undergoing lung lobectomy (48). These parameters may have certain implications for mechanistic studies. In addition, variations in acupoint selection, stimulation parameters, sample sizes, and regional differences among research centers may also result in distinct physiological effects. Currently, there is a lack of standardized stimulation protocols in clinical practice. Given the inter-individual variability in parameter sensitivity, inconsistencies may exist in both the therapeutic efficacy of TEAS and its underlying mechanisms. Future research should aim to establish standardized parameters for TEAS in the perioperative period, so as to facilitate the clinical standardization of its application and clarify its mechanisms of action.
With the continuous integration and development of biomedical sciences, neuroscience, and electrophysiology, the application of TEAS in the perioperative period will become increasingly precise and efficient. Future research should focus on exploring the regulatory mechanisms of TEAS on neurotransmitter release during the perioperative period (49), the activation mechanisms of microglia, the expression of NLRP3 inflammasome, and the interactions between these mechanisms, among other aspects (50). Moreover, future studies should investigate the advantages of different perioperative conditions by employing various electrical stimulation frequencies of TEAS. By setting different electrical stimulation frequencies, the perioperative advantages of TEAS for various diseases could be explored. In addition, large-scale, multi-center clinical studies should be conducted to further standardize the clinical mechanism research of TEAS. These potential research directions will not only deepen our understanding of the mechanisms underlying TEAS but also provide safer, more effective, and convenient treatment options for perioperative patients, opening a new chapter of modern nerve electrical stimulation technology.
XJ: Conceptualization, Methodology, Project administration, Writing – original draft, Writing – review & editing. ML: Conceptualization, Methodology, Writing – original draft, Writing – review & editing, Project administration. YT: Resources, Writing – review & editing. JH: Resources, Writing – review & editing. XG: Writing – review & editing. CZ: Writing – review & editing. TL: Supervision, Writing – review & editing. YW: Supervision, Writing – review & editing. HW: Funding acquisition, Writing – review & editing.
The author(s) declare that financial support was received for the research and/or publication of this article. This study was supported by grants from the National Key R&D Program of China (2022YFC3500705).
The authors declare that the research was conducted in the absence of any commercial or financial relationships that could be construed as a potential conflict of interest.
The authors declare that no Gen AI was used in the creation of this manuscript.
All claims expressed in this article are solely those of the authors and do not necessarily represent those of their affiliated organizations, or those of the publisher, the editors and the reviewers. Any product that may be evaluated in this article, or claim that may be made by its manufacturer, is not guaranteed or endorsed by the publisher.
The Supplementary material for this article can be found online at: https://www.frontiersin.org/articles/10.3389/fneur.2025.1563681/full#supplementary-material
2. He, M, Hu, C, and Tian, W. Application of transcutaneous electrical acupoint stimulation in perioperative period. Acupunct Res. (2021) 46:800–3. doi: 10.13702/j.1000-0607.200977
3. Ren, S, Lu, H, Zhang, D, and Su, F. Postoperative analgesic effect of transcutaneous electrical acupoint stimulation on general anesthesia: a meta-analysis. J Clin Anesthesiol. (2022) 38:400–5. doi: 10.12089/jca.2022.04.013
4. Lin, Y, Yang, W, Li, Y, Li, Y, Tong, MW, Li, Y, et al. Mechanism of acupoint transcutaneous electric stimulation on analgesic anesthesia in the patients undergoing general anesthesia anorectal operation. Chin Acupunct Moxibust. (2017) 37:747–52. doi: 10.13703/j.0255-2930.2017.07.017
5. Shi, Y, Wang, T, Cao, X, and Han, L. Effects of transcutaneous Acupoint electrical stimulation assisted anesthesia on plasma levels of β-EP,5-HT, PGE2 and pain in patients undergoing thoracic surgery. J Liaoning Univ Tradit Chin Med. (2021) 23:190–3. doi: 10.13194/j.issn.1673-842x.2021.09.039
6. Kong, J, Feng, D, Chen, Y, Min, Y, Wang, J, and Lv, X. Effects of transcutaneous electrical acupoint stimulation on inflammation in patients undergoing video-assisted thoracoscopic lobectomy. J Tongji Univ (Med Sci). (2019) 40:321–5. doi: 10.16118/j.1008-0392.2019.03.011
7. Qi, W-H, Miao, W-J, Ji, Y-Z, Li, C, and Wang, J-H. The analgesic effect of transcutaneous electrical Acupoint stimulation on labor: a randomized control study. IJGM. (2021) 14:559–69. doi: 10.2147/IJGM.S291699
8. Zhou, X, Cao, S-G, Tan, X-J, Liu, X-D, Li, Z-Q, Kong, L-X, et al. Effects of transcutaneous electrical Acupoint stimulation (TEAS) on postoperative recovery in patients with gastric Cancer: a randomized controlled trial. CMAR. (2021) 13:1449–58. doi: 10.2147/CMAR.S292325
9. Zub-Pokrowiecka, A. Ghrelin and gastrin in advanced gastric cancer before and after gastrectomy. WJG. (2011) 17:449–58. doi: 10.3748/wjg.v17.i4.449
10. Ye, Z, Shi, P, Zhang, S, and Luo, D. Effect of percutaneous point electrical stimulation on pain and gastrointestinal peristalsis function in patients after surgery for gastric Cancer. New Chin Med. (2024) 56:160–4. doi: 10.13457/j.cnki.jncm.2024.08.027
11. Huo, Y, Yao, L, and Wang, G. Correlation of serum BDNF, BAFF and GFAP with the condition and cognitive function of epilepsy patients. Pract J Clin Med. (2022) 19:154–7.
12. Jin, X, Zhou, S, Zhou, Q, and Jiang, Y. Study on the effect of percutaneous acupoint electrical stimulation during perioperative period to prevent cognitive impairment after total knee arthroplasty in the elderly. Chin J Gen Pract. (2024) 22:235–9. doi: 10.16766/j.cnki.issn.1674-4152.003373
13. Chen, X, Cai, H, and Guo, J. Effect of TEAS combined with general anesthesia on early postoperative cognitive function in elderly patients undergoing single-port thoracoscopic lobectomy. Pak J Med Sci. (2022) 38:2118–24. doi: 10.12669/pjms.38.8.6927
14. Saravanan, B, Kundra, P, Mishra, SK, Surianarayanan, G, and Parida, PK. Effect of anaesthetic agents on olfactory threshold and identification – a single blinded randomised controlled study. Indian J Anaesth. (2018) 62:592. doi: 10.4103/ija.IJA_245_18
15. Huang, J, Gao, H, Cao, Y, Zhou, J, Ren, Y, Xi, T, et al. Effect of transcutaneous electrical acupoint stimulation on postoperative olfactory memory disorder and plasma melatonin in patients with general anesthesia of sevoflurane. Chin Acupunct Moxibust. (2020) 40:243–6. doi: 10.13703/j.0255-2930.20190326-k0010
16. Hou, Y, Han, M, Yang, C, Liu, H, Guan, X, Wu, Q, et al. Effect of transcutaneous electrical acupoint stimulation on the perioperative plasma melatonin levels and postoperative sleep quality in living kidney donors. J Clin Anesthesiol. (2022) 38:12–6. doi: 10.12089/jca.2022.01.003
17. Cai, Y, Lin, Y, Yin, S, Ding, Y, Wu, W, Mo, K, et al. Effect of transcutaneous electrical acupoint stimulation based on the theory of "qi ascending and descending and descending movement" on autonomic nervous system and gastrointestinal function in patients after general anesthesia laparoscopic cholecystectomy. Acupunct Res. (2024) 49:283–8. doi: 10.13702/j.1000-0607.20221402
18. Li, Y, Xu, Z, Wang, S, Dou, B, Li, Y, Yang, J, et al. Effect and mechanism of autonomic nerve-mediated acupuncture on functional dyspepsia. J Clin Acupunct Moxibust. (2021) 37:84–8. doi: 10.19917/j.cnki.1005-0779.021082
19. Hou, L, Rong, P, Li, L, Wei, W, Fang, J, Zhang, J, et al. Effects of transcutaneous auricular vagus nerve stimulation on autonomic nervous function in rats with functional dyspepsia. Acupunct Res. (2021) 46:663–70. doi: 10.13702/j.1000-0607.200965
20. Li, J, Zhao, W, Shao, X, Yang, A, Zhang, F, and Fang, J. Effect of transcutaneous electrical Acupoint stimulation on post-surgical gastrointestinal function, autonomic nerve activities and plasma brain-gut peptide levels in patients undergoing gastrointestinal surgery. Acupunct Res. (2016) 41:240–6. doi: 10.13702/j.1000-0607.2016.03.010
21. Luo, P, Ye, Y, Liu, F, and Sun, W. Effect of percutaneous Acupoint electrical stimulation on pulmonary complications in patients undergoing radical resection of esophageal carcinoma. Chin J Trad Med Sci Technol. (2023) 30:215–8.
22. Yuan, L, Guo, J, Tang, W, Wang, J, Fu, G, and Guo, F. Effect of transcutaneous electrical acupoint stimulation on gastrointestinal function in perioperative period of laparoscopic intestinal surgery. J Clin Anesthesiol. (2017) 33:567–9.
23. Guo, J, Tang, W, Guo, F, Yang, L, Wang, J, Fu, G, et al. Transcutaneous electrical acupoint stimulation on inflammatory response and intestinal permeability in perioperative period of laparoscopic intestinal surgery. Chin Acupunct Moxibust. (2018) 38:1043–6. doi: 10.13703/j.0255-2930.2018.10.004
24. van der Pol, A, van Gilst, WH, Voors, AA, and van der Meer, P. Treating oxidative stress in heart failure: past, present and future. Eur J Heart Fail. (2019) 21:425–35. doi: 10.1002/ejhf.1320
25. Niu, C, Zhu, M, Zhang, C, and Zhang, J. Effect of transcutaneous electrical acupoint stimulation on perioperative stress responses in patients with essential hypertension. Jiangsu Med J. (2019) 45:817–9. doi: 10.19460/j.cnki.0253-3685.2019.08.019
26. Wang, J, Lu, F-F, Ge, M-M, Wang, L-W, Wang, G, Gong, G-W, et al. Transcutaneous electrical Acupoint stimulation improves postoperative sleep quality in patients undergoing laparoscopic gastrointestinal tumor surgery: a prospective, randomized controlled trial. Pain Ther. (2023) 12:707–22. doi: 10.1007/s40122-023-00493-2
27. Manou-Stathopoulou, V, Korbonits, M, and Ackland, GL. Redefining the perioperative stress response: a narrative review. Br J Anaesth. (2019) 123:570–83. doi: 10.1016/j.bja.2019.08.011
28. Tu, Q, Shi, J, Yu, H, Gu, S, Zhang, S, and Gan, J. Effect of percutaneous acupoint electrical stimulation on stress levels during the induction period of general anesthesia. Mod J Integr Trad Chin Western Med. (2019) 28:209–12.
29. Li, B, Zhao, Z, Liu, S, Chen, X, Shen, Y, and Chen, X. Effects of transcutaneous electrical acupoint stimulation on stress response and gastrointestinal function after laparoscopic cholecystectomy. J Clin Anesthesiol. (2023) 39:379–83.
30. Wang, L, Zhi, J, Wang, C, Lu, J, and Liu, Q. Influence of twirling reinforcement-reduction method of acupuncture on concentration of ET, NE and 5-HT in rats with stress-induced hypertension. J Beijing Univ Trad Chin Med. (2014) 37:681–5.
31. Xie, S. Application of transcutaneous electrical Acupoint stimulation to anesthesia for orthopedic surgery in the elderly. Shanghai J Acupunct Moxibust. (2020) 39:579–83. doi: 10.13460/j.issn.1005-0957.2020.05.0579
32. Ren, XF, Li, WZ, Meng, FY, and Lin, CF. Differential effects of propofol and isoflurane on the activation of T-helper cells in lung cancer patients. Anaesthesia. (2010) 65:478–82. doi: 10.1111/j.1365-2044.2010.06304.x
33. Wu, H, Meng, D, Wang, K, Quan, L, Kong, Q, Zhang, G, et al. The regulation of transcutaneous electrical acupoint stimulation on immune function in perioperative patients with lung cancer. Chin J Rehabil Med. (2014) 29:731–5.
34. Lin, W, Niu, Z, Zhang, H, Kong, Y, Wang, Z, Yang, X, et al. Imbalance of Th1/Th2 and Th17/Treg during the development of uterine cervical cancer. Int J Clin Exp Pathol. (2019) 12:3604–12.
35. Wu, H, Wang, K, Li, G, Meng, D, Han, J, Wang, G, et al. Effects of transcutaneous acupoint electrical stimulation on the imbalance of Th1, Th2, Th17 and Treg cells following thoracotomy of patients with lung cancer. Exp Ther Med. (2016) 11:495–502. doi: 10.3892/etm.2015.2913
36. Zhao, F, Gao, Y, Cai, H, Song, S, Liu, X, and Liu, J. Effect of transcutaneous electric acupoints stimulation on vascular endothelial function and inflammatory factors after percutaneous coronary intervention. Acupunct Res. (2021) 46:684–9. doi: 10.13702/j.1000-0607.200784
37. Xu, N, and Chen, X. The functional changes of endothelial cells and their consequences in inflammatory processes. Int J Surg. (2005):21–5.
39. Wang, J, Ren, Q, Shen, C, Xie, W, Zheng, R, and Ni, J. Effect of transcutaneous Acupoint electrical stimulation on blood bioactive compounds involving cerebral injury during craniotomy. Acupunct Res. (2008) 33:26–30.
40. Kearon, C, Akl, EA, Comerota, AJ, Prandoni, P, Bounameaux, H, Goldhaber, SZ, et al. Antithrombotic therapy for VTE disease. Chest. (2012) 141:e419S–96S. doi: 10.1378/chest.11-2301
41. Li, Y, and Li, W. Disseminated intravascular coagulation etiology, pathogenesis and its prevention and treatment. Chin J Pract Pediatr. (2013) 28:669–72.
42. Zhou, Y, Gu, L, and Gu, Y. Observation of the clinical effect of percutaneous acupoint electrical stimulation in the prevention of deep vein thrombosis of the lower extremity in the perioperative period of rectal cancer. Mod Nurse. (2019) 26:69–71.
43. Shen, W, Wang, J, and Ji, M. The preventive effect of percutaneous acupoint electrical stimulation on the prevention of lower extremity deep vein thrombosis after orthopedic surgery. Chin J Trad Med Sci Technol. (2017) 24:207–8.
44. Ding, Y, Su, S, Lin, Y, Wei, Y, Shi, J, Gao, ZJ, et al. Effect of transcutaneous acupoint electrical stimulation at Neiguan (PC 6) on general anesthesia under preserving spontaneous breathing in thoracoscopic lobectomy. Chin Acupunct Moxibust. (2023) 43:282–6. doi: 10.13703/j.0255-2930.20220419-k0013
45. Fu, T, and Li, J. Progress in the mechanism and clinical application of transcutaneous acupoint electrical stimulation to improve postoperative gastrointestinal dysfunction. J Clin Anesthesiol. (2023) 39:528–31. doi: 10.12089/jca.2023.05.016
46. Chen, X, Shan, R, and Huang, C. The role of electroacupuncture in modulating microglial polarization for treatment of neuropathic pain. J Gannan Med Univ. (2024) 44:1156–62.
47. Cha, C, Li, Z, Wang, Y, and Luo, M. Sleep disorders and perioperative neurocognitive dysfunction. Med J Chin People's Liberat Army. (2024) 49:1099–104.
48. Huang, S, Peng, W, Tian, X, Liang, H, Jia, Z, Lo, T, et al. Effects of transcutaneous electrical acupoint stimulation at different frequencies on perioperative anesthetic dosage, recovery, complications, and prognosis in video-assisted thoracic surgical lobectomy: a randomized, double-blinded, placebo-controlled trial. J Anesth. (2017) 31:58–65. doi: 10.1007/s00540-015-2057-1
49. Zhou, J, Dong, L, and Zhu, Z. Advances in the effect of neuroinflammation on postoperative cognitive dysfunction. J Clin Anesthesiol. (2021) 37:1317–20.
Keywords: transcutaneous electrical acupoint stimulation, perioperative period, mechanism, surgery, rehabilitation
Citation: Jiang X, Li M, Tang Y, Hu J, Gai X, Zhang C, Li T, Wang Y and Wang H (2025) Research progress on the mechanism of transcutaneous electrical acupoint stimulation in the perioperative period. Front. Neurol. 16:1563681. doi: 10.3389/fneur.2025.1563681
Received: 20 January 2025; Accepted: 27 March 2025;
Published: 11 April 2025.
Edited by:
Hewei Wang, Fudan University, ChinaReviewed by:
Jian-Feng Tu, Beijing University of Chinese Medicine, ChinaCopyright © 2025 Jiang, Li, Tang, Hu, Gai, Zhang, Li, Wang and Wang. This is an open-access article distributed under the terms of the Creative Commons Attribution License (CC BY). The use, distribution or reproduction in other forums is permitted, provided the original author(s) and the copyright owner(s) are credited and that the original publication in this journal is cited, in accordance with accepted academic practice. No use, distribution or reproduction is permitted which does not comply with these terms.
*Correspondence: Hongfeng Wang, Y2N3aGZAMTI2LmNvbQ==
†These authors have contributed equally to this work and share first authorship
Disclaimer: All claims expressed in this article are solely those of the authors and do not necessarily represent those of their affiliated organizations, or those of the publisher, the editors and the reviewers. Any product that may be evaluated in this article or claim that may be made by its manufacturer is not guaranteed or endorsed by the publisher.
Research integrity at Frontiers
Learn more about the work of our research integrity team to safeguard the quality of each article we publish.