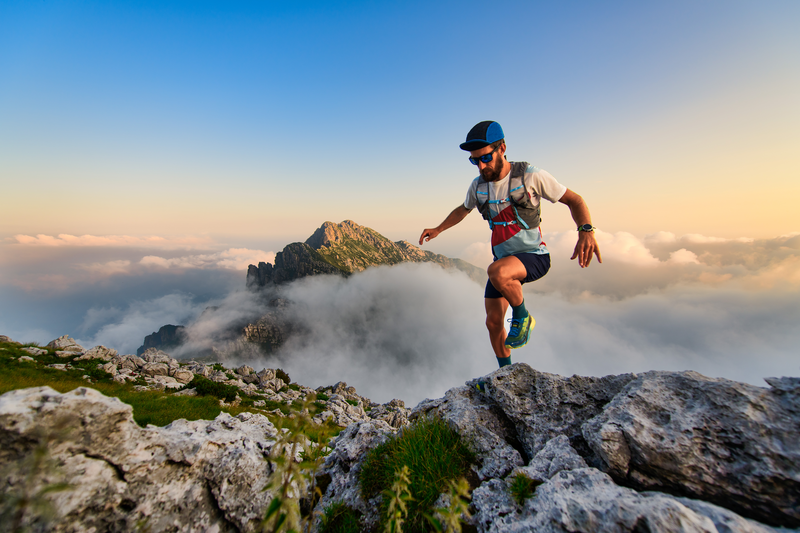
94% of researchers rate our articles as excellent or good
Learn more about the work of our research integrity team to safeguard the quality of each article we publish.
Find out more
ORIGINAL RESEARCH article
Front. Neurol.
Sec. Neuroepidemiology
Volume 16 - 2025 | doi: 10.3389/fneur.2025.1559484
The final, formatted version of the article will be published soon.
You have multiple emails registered with Frontiers:
Please enter your email address:
If you already have an account, please login
You don't have a Frontiers account ? You can register here
Background: Intracranial aneurysms (IAs) can lead to subarachnoid hemorrhage, a life-threatening event associated with high morbidity and mortality. Identifying individuals at elevated risk is crucial for guiding timely interventions and improving patient outcomes.In this retrospective cohort study, 850 patients who received interventional or surgical treatment for IAs between January 2018 and January 2024 were included.Demographic data (e.g., age, sex), lifestyle factors, and comorbidities were recorded.Hematologic, biochemical, and coagulation parameters were measured to evaluate their potential association with IA rupture. A univariate logistic regression was first conducted, followed by a multivariate logistic regression with a backward stepwise approach to derive the final predictive model. The model's performance was assessed using the area under the receiver operating characteristic curve (AUC), calibration curves, and decision curve analysis.Results: Younger age, female sex, higher neutrophil count, lower hematocrit, and elevated markers of inflammation and coagulation (including fibrinogen and D-dimer) emerged as key risk factors. Electrolyte imbalances, such as low potassium, and elevated lactate dehydrogenase were also significantly associated with rupture. The optimized model achieved an AUC of 0.815, with good calibration and clinical utility indicated by decision curve analysis.These findings highlight the interplay of demographic, inflammatory, metabolic, and coagulation parameters in determining rupture risk in patients with IAs.Incorporating these risk factors into clinical practice may enhance early detection, guide targeted prevention strategies, and ultimately improve outcomes for high-risk individuals.
Keywords: Intracranial aneurysms, Inflammatory markers, Metabolic biomarkers, Coagulation Factors, Predictive Modeling
Received: 21 Jan 2025; Accepted: 04 Mar 2025.
Copyright: © 2025 Zhang, Liu, Xu, Cai, Ba, Lin, Sun and Ye. This is an open-access article distributed under the terms of the Creative Commons Attribution License (CC BY). The use, distribution or reproduction in other forums is permitted, provided the original author(s) or licensor are credited and that the original publication in this journal is cited, in accordance with accepted academic practice. No use, distribution or reproduction is permitted which does not comply with these terms.
* Correspondence:
Liangzhi Ye, Wenzhou Central Hospital, Wenzhou, China
Disclaimer: All claims expressed in this article are solely those of the authors and do not necessarily represent those of their affiliated organizations, or those of the publisher, the editors and the reviewers. Any product that may be evaluated in this article or claim that may be made by its manufacturer is not guaranteed or endorsed by the publisher.
Research integrity at Frontiers
Learn more about the work of our research integrity team to safeguard the quality of each article we publish.